Green tea and black tea are derived from the leaves of Camellia sinensis L. (Theaceace). Green tea is produced by rapid heating of the leaves to preserve the colour and antioxidative compounds present in C. sinensis. Black tea is produced by a fermentation process, which is known to reduce the content of antioxidants(Reference Balentine, Wiseman and Bouwens1). Rooibos tea is produced from the leaves and stems of Aspalathus linearis Dahlg. (Leguminosae) and is a caffeine-free alternative to green and black tea. Tea is suggested to reduce mortality in CVD(Reference Hakim, Alsaif and Alduwaihy2, Reference Mukamal, Maclure and Muller3), such as acute myocardial infarction(Reference Mukamal, Maclure and Muller3). Several positive effects of tea on the cardiovascular system have been shown: reduction of atherosclerosis(Reference Basu and Lucas4) and lipid peroxidation(Reference Basu and Lucas4–Reference Tipoe, Leung and Hung7), anti-inflammatory properties(Reference O’Keefe, Gheewala and O’Keefe6, Reference Tipoe, Leung and Hung7) and improvement of endothelial function(Reference Kim, Kim and Choi8).
The effect of tea is suggested to be due to its high content of the flavonoid group catechins, i.e. epicatechin, epigallocatechin, epicatechingallate and epigallocatechingallate(Reference Ahmed, Rahman and Hasnain9–Reference Persson, Josefsson and Persson11). Epigallocatechingallate is the catechin most associated with the preventive effects of tea on CVD, due to its strong antioxidative properties(Reference Ahmed, Rahman and Hasnain9, Reference Miura, Chiba and Tomita10). In contrast to green and black tea, Rooibos tea contains dihydrochalcones, flavones and flavonols(Reference Bramati, Aquiland and Pietta12) but does not contain any catechins(Reference Persson, Josefsson and Persson11).
Angiotensin-converting enzyme (ACE) is a key enzyme in the renin–angiotensin aldosterone system (RAAS). ACE converts the inactive decapeptide angiotensin I, forming the active octapeptide angiotensin II. Angiotensin II is a main effector of the RAAS and seems to be involved in developing CVD. Angiotensin II binds to the angiotensin receptors AT1 and AT2. Activation of AT1 receptors is associated with endothelial dysfunction(Reference Nishimura13), vasoconstriction(Reference Nishimura13), cell proliferation(Reference Benson, Iguchi and Ho14), platelet aggregation(Reference Fogari and Zoppi15), inhibition of nitric oxide synthase(Reference Rush and Aultman16), aldosterone release(Reference Weiss, Sorescu and Taylor17), cognitive and behavioural processes such as depression, anxiety, enhanced learning and memory(Reference Birkenhäger and Staessen18–Reference McGuiness, Todd and Passmore20), dementia(Reference McGuiness, Todd and Passmore20) and increases in reactive oxygen species(Reference Reed, Kolz and Potter21).
The ACE gene polymorphic sites are an insertion/deletion (I/D) consisting of three genotypes: II and DD homozygotes and ID heterozygote(Reference Rigat, Hubert and Alhenc-Gelas22). The I/D polymorphism of the ACE gene determines individual serum levels of ACE(Reference Rigat, Hubert and Alhenc-Gelas22, Reference Tiret, Rigat and Visvikis23). The levels of ACE are two- to threefold higher in people with the DD genotype than in those with the II genotype, and people with the ID genotype have an intermediate level of ACE(Reference Rigat, Hubert and Alhenc-Gelas22, Reference Beohar, Damaraju and Prather24). The D allele of the ACE gene is proposed to correlate with CVD(Reference Cambien, Poirier and Lecerf25, Reference Samani, Thompson and O’Toole26). Hence, it seems that the DD genotype could be a risk factor for CVD(Reference Beohar, Damaraju and Prather24).
There are several interactions known between the RAAS and nitric oxide (NO). Angiotensin II and NO act as physiological antagonists concerning vascular tone and growth(Reference Millatt, Abdel-Rahman and Siragy27). NO has also been proposed as a negative regulator of angiotensin receptors, and endothelial angiotensin receptors may stimulate NO release(Reference Fernándes-Alfonso and González28). Furthermore, activation of the RAAS is believed to be involved in tolerance to organic nitrates(Reference Kurz, Hink and Nickenig29). NO is proposed to act as an ACE inhibitor in rats(Reference Ackermann, Fernández-Alfonso and Sánchez de Rojas30). It has also been shown that exogenous and endogenous NO can inhibit ACE in pigs and man in vitro and this NO-mediated ACE inhibition is additive with traditional ACE inhibitors like captopril, and of functional importance concerning angiotensin I-induced vasoconstriction and platelet aggregation(Reference Persson and Andersson31, Reference Persson, Whiss and Nyhlén32). This implies that NO not only has direct inhibitory effects on atherogenesis, but also indirect effects by decreasing the amount of angiotensin II.
In previous studies we found that green tea and black tea can inhibit ACE activity in human endothelial cells and that green tea, black tea and Rooibos tea can increase NO concentration from the same cells(Reference Persson, Josefsson and Persson11). Since there are no in vivo data supporting these effects, we wished to study the effect of green tea, black tea and Rooibos tea on ACE activity and NO concentration in healthy volunteers in vivo.
Materials and methods
Study subjects
The study protocol was approved by the regional Ethics Committee at Linköping University, Sweden (Dnr M56-07) and informed consent was obtained from the twenty non-nicotine-using healthy volunteers (ten males and ten females) who took part in the investigation. Their age ranged between 20 and 31 years with a mean value of 26 years. All subjects were healthy, as assessed by medical history and physical examination. Study participants did not use any kind of drugs (medical or herbal) two weeks prior to and during the entire sampling period. Intake of beverages or foods containing high amounts of catechins, the flavonol quercetin or anthocyanins (i.e. chokeberries, aubergine, blackberries, bilberries, elderberries, raspberries, strawberries, coffee, cacao/chocolate, cherries, onions, plums, pears, radishes, red and black currants, red cabbage, black beans, cranberries, tea, wine, grapes and apples) was not allowed 48 h before the experiment. A list of foods not allowed was distributed to the participants when they registered to the study. The participants also answered a questionnaire concerning everyday intake of certain foods, i.e. vegetables, fruits and berries, containing the flavonoids catechins, quercetin and anthocyanins. Three subjects were excluded (one male and two female); two because of practical difficulties when collecting blood and one due to oral use of tobacco.
Study design
A randomized three-phase crossover design was used and the phases were separated by a period of 1–4 weeks. Forty-eight hours prior to each phase, the participants were not allowed intake of food or beverages containing high amounts of certain flavonoids (as mentioned above). The study subjects received 400 ml of green tea (Japanese Sencha), black tea (Indian Assam B.O.P.) or Rooibos tea (South Africa) in each phase. All experiments started at 08.00 hours and the subjects were not fasting, to resemble ordinary intake of tea. The tea was freshly prepared and cooled so the participants could drink it within approximately 2 min. The tea was prepared from green tea, Japanese Sencha imported by Charabang, Stockholm, Sweden; black tea, Indian Assam B.O.P. imported by Norrköping Kolonial, Sweden; and Rooibos tea imported by Norrköping Kolonial, Sweden. The teas were bought at Tebladet, Linköping, Sweden. Infusions were made with 10 g tea in 400 ml fresh-boiled water for 5 min with the green tea and the black tea, and for 10 min with the Rooibos tea, using a tea filter (Agatha’s Bester, Germany). The different boiling times were used to mimic the brewing of tea in households.
Sample collection
Data collected at each treatment were blood pressure, heart rate and venous blood samples for analyses of ACE activity, NO concentration and ACE genotype. Blood pressure, heart rate and blood samples for analyses of ACE activity and NO concentration were taken before drinking tea and after 30 min, 60 min and 3 h. Blood pressure and heart rate were measured in both supine and standing position, while blood samples were collected in supine position. Vacutainer tubes without anticoagulant were used to collect blood for serum-ACE and serum-nitrite analyses. After 2 h, the tubes were centrifuged at 1000 g for 20 min at 4°C. Serum was transferred to plastic tubes and ACE activity was analysed the same day, as described below. Remaining serum was frozen at −70°C until nitrite analysis. Vacutainer tubes with EDTA were used to collect blood for determination of polymorphism concerning the ACE genotype. The blood was transferred to sterile test tubes and frozen at −70°C until analysis.
Analytical methods
ACE activity was analysed using a commercial radioenzymatic assay (ACE-direct REA; Bühlmann Laboratories, Allschwil, Switzerland) with the following modifications. Blank and standard serum and the human serum were added to wells in a microtitre plate. The synthetic substrate [3H]hippuryl-glycyl-glycine was then added directly to all wells. The serum was incubated for 1 h at 37°C to allow for the ACE present to cleave the substrate to [3H]hippuric acid. Then serum and substrate was transferred from each well into scintillation vials containing 50 μl 1 m-HCl, to stop the enzymatic reaction. Scintillation liquid was added and the samples were counted in a scintillation counter.
Nitrite was analysed as a marker of NO concentration as proposed by Lauer et al.(Reference Lauer, Preik and Rassaf33). Nitrite concentration in the serum was analysed with a commercially available nitrite/nitrate assay: nitric oxide () assay kit obtained from R&D Systems, Abingdon, UK. The principle for the colorimetric detection of nitrite is the Griess reaction. In short, diazonium ions are produced when acidified nitrite reacts with sulfanilic acid. These diazonium ions form chromophore agents when reacting with N-(1-naphthyl)ethylenediamine. Optical density was determined with a Spectramax reader at 540 nm.
Genomic DNA was isolated from venous blood using QIAmp DNA Mini Kit (QIAGEN, Hilden, Germany). The ACE gene contains a polymorphism based on the absence and/or presence of a 287 bp non-sense DNA domain in intron 16, which results in three different genotypes: deletion/deletion homozygote (DD), insertion/insertion homozygote (II) and insertion/deletion heterozygote (ID). Three primers, 5′-CTG CAG GTG TCT GCA GCA TGT GC-3′, 5′-GAT TAC AGG CGT GAT ACA GTC ACT TTT-3′ and 5′-GCC ATC ACA TTC GTC AGA TCT GGT AG-3′ (Invitrogen Ltd, Paisley, UK), were used according to the method of Cheon et al.(Reference Cheon, Choi and Lee34).
For the PCR, a ‘ready-to-use’ reaction mixture (REDExtract-N-Amp Blood PCR ReadyMix; Sigma-Aldrich, St. Louis, MO, USA) was used in a total volume of 20 μl. Each primer was used at a concentration of 0.4 μm and for each reaction 2 μl DNA solution was added to the reaction mixture.
The PCR was performed in a Perkin Elmer Cetus DNA Thermal Cycler and started with 5 min of initial denaturation at 94°C. Then the samples underwent thirty cycles of amplification: 45 s of denaturation (94°C), 45 s of annealing (65°C) and 45 s of extension (72°C). The reaction was finished with 7 min of final extension (72°C) and hold at 4°C. The PCR products were separated by electrophoresis on a 2 % agarose gel, then stained with ethidium bromide and visualized with UV light. The PCR products detected with the primers are one 237 bp fragment for the deleted allele, and one 525 and one 155 bp fragment for the inserted allele.
Statistical analysis
Results are presented as means with their standard error. One unit (U) of ACE activity is defined as the amount of the enzyme required to release 1 μmole hippuric acid per minute and litre. Graph Pad Prism 5·0 (La Jolla, CA, USA) was used for statistical calculations. One-way ANOVA for repeated measures was performed followed by Dunnett’s post hoc test or Bonferroni’s post hoc test (questionnaire). Statistical significance was taken at P < 0·05.
Results
Angiotensin-converting enzyme activity
After oral intake of 400 ml green tea, black tea or Rooibos tea, significant inhibition of ACE activity was seen with the Rooibos tea after 30 min (P < 0·01) and after 60 min (P < 0·05). No significant inhibition was seen with the green tea or the black tea (Figs 1a, b and c). When the participants were grouped according to ACE genotype, a significant inhibition of ACE activity was seen with the green tea for the II genotype 30 min after intake of the tea (P < 0·05) and for the ID genotype 60 min after intake of the tea (P < 0·05; Fig. 2a). A significant inhibition of ACE activity was also seen with the Rooibos tea for the II genotype 60 min after intake of the tea (P < 0·05; Fig. 2c). No significant effect was seen with the black tea (Fig. 2b). No significant effect was seen with any of the teas for the DD genotype (Figs 2a to c). The basal level of ACE activity was significantly higher (P < 0·001) for the participants with the ID and DD genotypes compared with those with the II genotype (Fig. 3). No difference was seen between males and females in any of the groups.

Fig. 1 Angiotensin-converting enzyme (ACE) activity in serum before oral intake and 30, 60 min and 3 h after intake of 400 ml green tea (a, d), black tea (b, e) or Rooibos tea (c, f) in healthy volunteers (mean age 26 years, range 20–31 years), Sweden. The upper row (a, b, c) shows mean values with their standard errors represented by vertical bars; the lower row (d, e, f) shows values from each individual. Mean values were significantly different from those at baseline (one-way ANOVA for repeated measures, n 17): *P < 0·05, **P < 0·01

Fig. 2 Angiotensin-converting enzyme (ACE) activity in serum before oral intake and 30, 60 min and 3 h after intake of 400 ml green tea (a), black tea (b) or Rooibos tea (c) according to ACE genotype (II, ; ID,
; DD,
) in seventeen healthy volunteers (mean age 26 years, range 20–31 years), Sweden. Values are means with their standard errors represented by vertical bars. Mean values were significantly different from those at baseline (one-way ANOVA for repeated measures; II, n 6; ID, n 5; DD, n 6): *P < 0·05
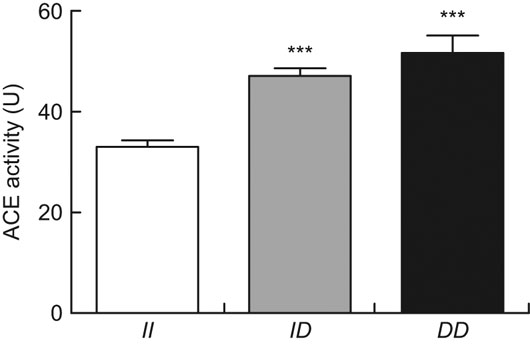
Fig. 3 Basal angiotensin-converting enzyme (ACE) activity in serum according to ACE genotype in healthy volunteers (mean age 26 years, range 20–31 years), Sweden. Values are means with their standard errors represented by vertical bars. Mean values were significantly different from those of the II genotype (one-way ANOVA; II, n 6; ID, n 5; DD, n 6): ***P < 0·001
Blood pressure and heart rate
Basal blood pressure (supine, standing; mmHg) was 121 (se 1·2)/71 (se 1·1), 122 (se 1·5)/74 (se 1·1). Blood pressure (supine, standing; mmHg) was 118 (se 1·4)/71 (se 1·1), 121 (se 1·8)/74 (se 1·3) after 30 min; 117 (se 1·5)/72 (se 1·0), 120 (se 1·8)/75 (se 1·3) after 60 min; and 116 (se 1·5)/71 (se 0·9), 119 (se 2·0)/74 (se 1·3) after 3 h. No significant change in blood pressure was seen after intake of any of the teas.
Basal heart rate (supine, standing; beats/min) was 75 (se 2), 83 (se 2). Heart rate (supine, standing; beats/min) was 65 (se 2), 71 (se 2) after 30 min; 63 (se 2), 71 (se 2) after 60 min; and 60 (se 2), 70 (se 2) after 3 h. No significant change in heart rate was seen after intake of any of the teas.
Nitric oxide concentration
After oral intake of 400 ml green tea, black tea or Rooibos tea, no significant effect on the NO concentration was seen (Fig. 4). After grouping the participants according to ACE genotype II, ID or DD, no significant effect was seen on NO concentration (data not shown).
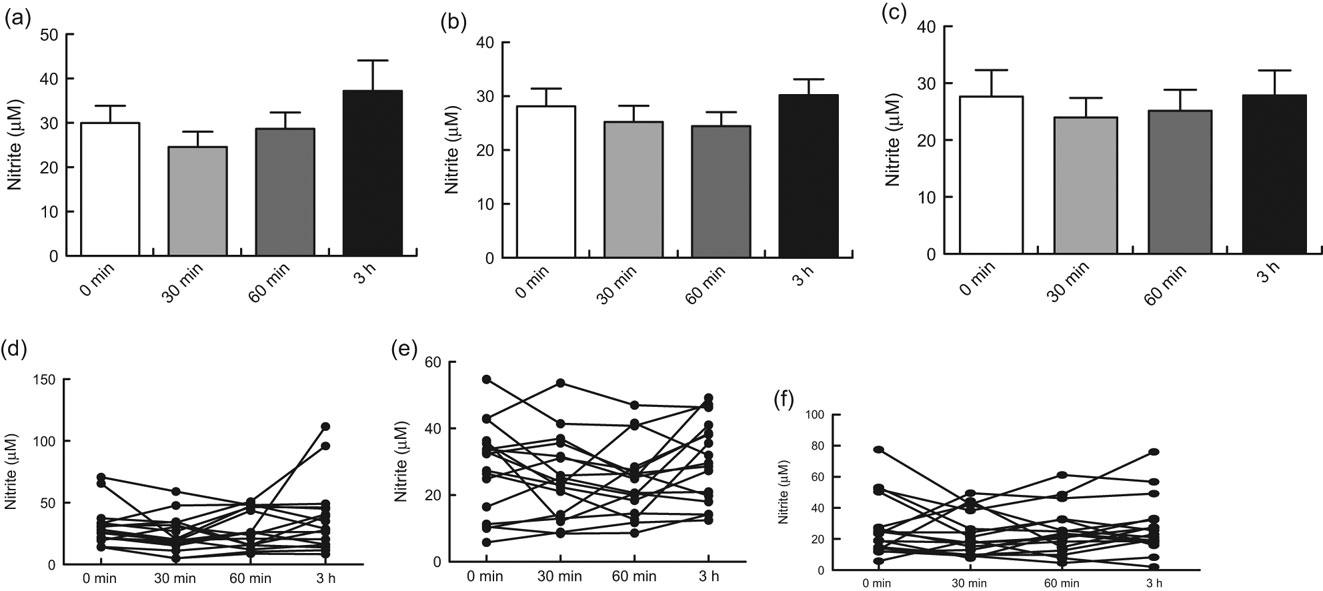
Fig. 4 Nitric oxide (NO) production (nitrite was analysed as a marker of NO concentration) in serum before oral intake and 30, 60 min and 3 h after intake of green tea (a, d), black tea (b, e) or Rooibos tea (c, f) in healthy volunteers (mean age 26 years, range 20–31 years), Sweden. The upper row (a, b, c) shows mean values with their standard errors represented by vertical bars; the lower row (d, e, f) shows values from each individual. There were no significant differences in NO production compared with baseline (one-way ANOVA for repeated measures, n 17)
The questionnaire
Average daily intake of green tea, black tea, Rooibos tea, coffee, vegetables and fruits is shown in Table 1. A significant (P < 0·01) difference was seen in intake of fruits and vegetables plus fruits between females and males (Table 1). The most consumed fruit were apples and bananas, and the most consumed vegetables were tomatoes. No differences in intake of apples, bananas and tomatoes were seen between males and females.
Table 1 Participants’ daily intake of flavonoid-containing foods from the questionnaireFootnote †: seventeen healthy volunteers (mean age 26 years, range 20–31 years), Sweden

Min, minimum; max, maximum.
† Intake based on answers to the following questions: (i) ‘How many cups of tea do you drink per day?’ (1 cup of tea is 250 ml); (ii) ‘How many cups of coffee do you drink per day?’ (1 cup of coffee is 150 ml); (iii) ‘How many times per day do you eat vegetables?’; and (iv) ‘How many times per day do you eat fruit?’ Mean values were significantly different from those of males: **P < 0·01.
Discussion
The current in vivo study showed a significant inhibition of ACE activity at 30 and 60 min after oral intake of Rooibos tea, while green tea and black tea showed no significant inhibition of ACE activity for the total group of participants (Figs 1a, b and c).
It was previously shown that green tea and black tea significantly inhibit ACE activity in human endothelial cells in vitro while Rooibos tea did not inhibit ACE activity in vitro (Reference Persson, Josefsson and Persson11). This discrepancy between the in vitro and in vivo studies may be due to either the content of the flavonoids or/and the metabolism of the components in the different teas, as there are differences in pharmacokinetics/metabolism between different flavonoids(Reference Karakaya35). The discrepancy between the studies could also be due to the bioavailability or/and to a possible different mechanism of action of the Rooibos tea compared with the green tea and the black tea.
The I/D polymorphism of the ACE gene is proposed to be important concerning serum levels of ACE(Reference Rigat, Hubert and Alhenc-Gelas22, Reference Tiret, Rigat and Visvikis23). As shown in Fig. 3, basal ACE activity of the participants showed a significant difference between the ACE II genotype and the ID and DD genotypes in accordance with the results of Rigat et al.(Reference Rigat, Hubert and Alhenc-Gelas22). The D allele of the ACE gene was previously proposed to be correlated with CVD(Reference Samani, Thompson and O’Toole26, Reference Cambien36). According to Fig. 2, the ACE II and ID genotypes seem to be more sensitive to ACE inhibition by the different teas than the DD genotype. A significant inhibition of ACE activity was seen for the II and ID genotypes with green tea and for the II genotype with Rooibos tea. The same pattern in ACE inhibitory action depending on ACE genotype has previously been shown with glyceryl trinitrate(Reference Persson, Säfholm and Andersson37). Hence, it seems that the DD genotype could be a risk factor for CVD, as previously proposed(Reference Beohar, Damaraju and Prather24). The difference between genotypes may also be a question of dosage. ID and even more the DD genotype might need higher amounts of flavonoids to affect ACE activity than the II genotype(Reference Danser, Batenburg and van den Meiracker38).
The effects of green and black tea on CVD are suggested to be due to their high content of catechins, i.e. epicatechin, epigallocatechin, epicatechingallate and epigallocatechingallate(Reference Persson, Josefsson and Persson11), while Rooibos tea does not contain any catechins(Reference Persson, Josefsson and Persson11) but dihydrochalcones, flavones and flavonols(Reference Bramati, Aquiland and Pietta12). The catechins especially epigallocatechingallate showed strong inhibitory effects on ACE activity in vitro (Reference Persson, Josefsson and Persson11). The slight inhibition of ACE activity seen by Rooibos tea in vitro might depend on its quercetin (flavonol) content, since quercetin exhibits an ACE inhibitory action in vitro (Reference Persson, Lindén and Andersson39). The two active sites of ACE contain Zn2+, and ability to bind the Zn2+ ion will inhibit ACE activity(Reference Berecek and Zhang40). ACE inhibition leads to less angiotensin II, and an increase of NO leads to down-regulation of AT1 receptors. Our previous in vitro study showed an increased NO concentration by green tea, black tea and Rooibos tea(Reference Persson, Josefsson and Persson11), but the current in vivo study did not show any significant effect on NO concentration after oral intake of any of the teas (Figs 4a, b and c). A possible explanation may be that we determined nitrite as a marker of NO, and nitrite is also a product of protein metabolism. As shown in Figs 1d to f and Figs 4d to f, the participants exhibited substantial individual variations in ACE activity as well as NO concentration and this fact may also influence the results. It must be taken into consideration that our in vivo study examined the effect on ACE activity and NO concentration after a single dose (one cup) of tea. The average inhibition of ACE activity 30 min after intake of Rooibos tea was 6 %, compared with 16 % inhibition of ACE activity after chronic intake of enalapril 10 mg(Reference Abrams, Davies and Gomez41). In the current study, no effect on blood pressure was seen after intake of tea. Since the RAAS is involved in long-term regulation of homeostasis, such an effect could not be expected. One previous study with 1 week’s intake of black tea showed a significant reduction in blood pressure and increase in flow-mediated vasodilation in healthy volunteers(Reference Grassi, Mulder and Draijer42). However, in a meta-analysis including the cardiovascular effects of black and green tea, no reduction in blood pressure was seen(Reference Hooper, Kroon and Rimm43). None of these studies included ACE activity among the investigated parameters. Further studies investigating regular long-term intake of tea remain to be performed.
Diet is of great importance to human health(Reference Allen, Carson and Kwik-Uribe44–Reference Patterson, Feightner and Garcia47). The prevention of CVD is attributed to a diversity of effects such as antioxidative(Reference Dragsted48), antithrombotic and anti-inflammatory properties, activation of endothelial nitric oxide synthase and inhibition of LDL oxidation(Reference Aron and Kennedy49–Reference Kaliora and Dedoussis53). Flavonoids, e.g. catechins found in green tea and black tea and flavonols found in Rooibos tea, are plant-derived antioxidants with alleged positive effect on the cardiovascular system(Reference Aron and Kennedy49, Reference Svarcova, Heinrich and Valentova54). However, the positive effect of fruit, vegetables and tea on CVD cannot always be solely explained by their antioxidative action. The present study shows an additional mechanism, flavonoids as ACE inhibitors.
The average intake of vegetables and fruit by the participants was approximately three times daily (Table 1). A significant difference was seen in frequency of intake of fruits and thereby in intake of vegetables plus fruits between females and males. Thus, according to this questionnaire, females consumed significantly more fruits than males. Recommendation from the Swedish National Food Administration is five times daily (or 500 g/d) for fruits and vegetables. According to the questionnaire, the most consumed fruits are apple and banana, and the most consumed vegetable is tomato. Apples, bananas and tomatoes also contain flavonoids, but at a much lower content than tea. Flavonoids, e.g. catechins found in tea, vegetables and fruits, are well known for their protective effect against CVD. Considering that, the intake of flavonoids among the subjects of the experiment seems to be generally low. Average daily intake of flavonoids is difficult to assess, it depends on season, availability, soil, climate and dietary habits.
Conclusion
In conclusion, oral intake of a single dose (400 ml) of Rooibos tea significantly inhibited ACE activity in healthy volunteers. When the participants were divided into subgroups according to ACE genotype, those with genotypes II and ID showed a significant inhibition of ACE activity after intake of green tea and Rooibos tea, while participants with ACE genotype DD seemed to be more difficult to affect with a single dose of tea. ACE inhibitors are the first-line treatment of hypertension and thus a common drug used for CVD. Intake of high amounts of flavonoids, i.e. tea, vegetables and fruits, might contribute to the prevention of CVD in the population and thereby prevent CVD mortality. These results suggest that green tea and Rooibos tea may have cardiovascular effects through inhibition of ACE activity. Additional experimental and clinical studies are needed in order to further elucidate these effects.
Acknowledgements
The study was supported by grants from Östergötlands County Council (LIO-8355) and the Cardiovascular Inflammation Research Centre (CIRC) at Linköping University. None of the authors have conflicts of interest. All the authors contributed to the design of the study. I.A.-L.P. performed the study, the analysis, interpreting the data and drafting the manuscript. S.H. was the physician responsible for the collection of blood samples. K.P. and R.G.G.A. conducted data analysis and interpretation. All of the authors read and approved the final manuscript. The authors acknowledge Marja Tjädermo and Ingela Jacobsson for excellent technical and clinical assistance.