Terror birds (Aves, Phorusrhacidae) comprise the most outstanding group of South American Cenozoic avifauna, and have been considered as apex predators in Cenozoic ecosystems (Ameghino Reference Ameghino1895; Andrews Reference Andrews1899; Alvarenga & Höfling Reference Alvarenga and Höfling2003; Blanco & Jones Reference Blanco and Jones2005; Bertelli et al. Reference Bertelli, Chiappe and Tambussi2007; Degrange et al. Reference Degrange, Tambussi, Moreno, Witmer and Wroe2010; Degrange Reference Degrange2012; Tambussi et al. Reference Tambussi, de Mendoza, Degrange and Picasso2012). Their terrestrial habits have been well established based on the reduction of their forelimbs (Alvarenga & Höfling Reference Alvarenga and Höfling2003; Degrange Reference Degrange2012), although it is possible that minor species were able to fly in a clumsy manner (Degrange Reference Degrange2012). One of the pillars for the hypothesis of a predatory mode of life for phorusrhacids is based on the morphology of their hind limbs (Fig. 1), which seem to be suitable for pursuing prey. However, this assumption has long been based solely on the premise that phorusrhacid hind limb bones are long, which is a feature that is present in several extant birds that do not run, but walk or hop. Although previous studies on the biomechanics of the hind limbs (e.g., Tambussi Reference Tambussi1997; Blanco & Jones Reference Blanco and Jones2005) and muscle reconstruction (Degrange Reference Degrange2012) pointed out that phorusrhacids were terrestrial birds with running abilities, a more extensive approach is needed.

Fig. 1 Phorusrhacid pelvis and hind limb: (A) Psilopterus bachmanni, YPM-PU 15904; (B) Psilopterus lemoinei, AMNH 9257 (pelvis) and YPM-PU 15402 (hind limb); (C) Procariama simplex, FM-P 14525; (D) Llallawavis scagliai, MMP 5050; (E) Mesembriornis milneedwardsi, MACN Pv 5944; (F) Patagornis marshi, NHMUK-A 516 (pelvis and femur) and AMNH 9264 (tibiotarsi and tarsometatarsus). Abbreviation: at = antitrochanter. Arrow indicates cranial part of the pelvis. Scale bars = 10 cm.
Gatesy & Dial (Reference Gatesy and Dial1996) developed the concept of locomotor modules to explain the origin and evolution of flight and the diversification of locomotor styles in modern birds. According to these authors, a locomotor module is a highly integrated anatomical subregion of the muscle-skeletal system, acting as a unit during locomotion due to independent neuromuscular control. The three locomotor modules recognised by these authors (wings, tail and hind limbs) are given different priority according to different lifestyles (Gatesy & Dial Reference Gatesy and Dial1996). However, Abourachid & Höfling (Reference Abourachid and Höfling2012) recognised that legs have a multi-purpose potential based on their three-segment configuration. This configuration allows the use of legs as propulsive, paddling, foraging or grooming tools.
Following on from these concepts, and in order to explore the relationship between terrestriality, running abilities and prey pursuit in terror birds, I use the quantitative approaches of traditional morphometrics and modern geomorphometrics to study the posterior appendicular module. I will explore whether morphometrics and/or qualitative features adequately reflect the different terrestrial locomotor strategies of extant birds. This type of analysis can be used to recognise skeletal features that are crucial for terrestrial locomotion in birds, and also to infer the locomotor styles of phorusrhacids. This is the first time that geomorphometrics has been applied to any terror bird, and the results represent a major step forward in the effort to place our understanding of the ecology of terror birds on a more quantitative footing.
1. Materials and methods
1.1. Anatomical nomenclature
The anatomical nomenclature follows Baumel et al. (Reference Baumel, King, Breazile, Evans and Vanden Berge1993), except where noted. Latin terminology is used for muscles and osteological structures, whilst the English equivalent is also used in the Discussion.
1.2. Traditional morphometrics: measurements
The phorusrhacid femur, tibiotarsus and tarsometatarsus were measured (Fig. 2; Table 1) and analysed using the classic multivariate analysis of morpho-functional and ecomorphological analyses: PCA and discriminant analysis. The proportion that each element contributes to the whole hind limb was also analysed using ternary plots. These approaches were chosen over geomorphometrics because hind limb elements provide few homologous points for description using landmarks.

Fig. 2 Measurements taken in birds' hind limbs studied herein. (A–D) femur: (A) cranial view; (B) lateral view; (C) proximal view; (D) distal view. (E–H) tibiotarsus: (E) cranial view; (F) lateral view; (G) proximal view; (H) distal view. (I–N) tarsometatarsus: (I) cranial view; (J) lateral view; (K) proximal view; (L) caudal view (hypotarsus measures); (M) cranial view (trochlear divarication); (N) distal view.
Measurements were taken using a 300 mm digital caliper with a resolution of 0.01 mm. No osteological reconstruction methods were used. As much of the material was very fragmentary, average individuals were created for each phorusrhacid species in order to use as many species as possible in the analysis. The average individual is made up from the average of each of the variables analysed (= measurement value/number of measurements carried out). All measurements are shown in .
Additionally, 22 ratios were constructed in order to obtain variables that describe the shape and features with a possible functional meaning, and to eliminate the arithmetic effect introduced by size in calculations. These are: femur, EA/EB, EC/ED, EC/EE, EF/EG, EI/EH; tibiotarsus, ZB/ZA, ZD/ZC, ZJ/ZD, ZC/ZE, ZF/ZG, ZH/ZI; tarsometatarsus, AA/AB, AA/AC, AD/AP, AD/AE, AJ/AK, AF/AG, AH/AI, AL/AM; whole hind limb, EB/ZB, ZB/AA, EB/AA.
Angular measurements (e.g., AL and AM) were converted to radians, and all measurements were then converted by applying a decimal-based logarithm in order to reduce heterocedasticity (i.e., dispersion associated with high values; Peters Reference Peters1983).
Principal component analyses (PCA) were carried out using a variance-covariance matrix. In order to check the contribution of body mass to the principal component 1 (the component that explains the most variation), a regression between the body mass and the contribution of each specimen to the component was performed. Dunning (Reference Dunning2008) was used as a reference for extant avian body masses. For the complete hind limb, a separate analysis of PCA was also performed using the ratios. A discriminant analysis using a correlation matrix was also performed on the tarsometatarsus (Campbell & Marcus Reference Campbell and Marcus1992) and the complete hind limb.
Two non-parametric discriminant analyses of the hind limb were carried out using a code written by Dr. A. Scarano (MLP), where the fda function of the mda package (Leisch et al. Reference Leisch, Hornik and Ripley2015) is used (using the MARS algorithm). The non-parametric method was chosen because the bird collection studied here is considered small (i.e., less than 250 specimens; A. Scarano pers. comm. 2011). In both analyses, a total of 67 individuals from different species were used to develop predictive models. To perform these analyses, three groups were distinguished: ‘waders’ (W) or wading birds; ‘ground birds’ (G) or land birds (including birds capable of walking, running, jumping or hopping); and ‘others’ (X), essentially a group including flying, arboreal, predatory and swimming birds. The assignment of each species to a group was carried out according to Zeffer et al. (Reference Zeffer, Johansson and Marmebro2003). This approach was used in order to explore the possibility that some phorusrhacids may have had wading habits.
The hind limb morphospace (a theoretical space that includes all morphological possibilities) of the phorusrhacids and other birds was also visualised using a ternary plot, in which the percentage contribution of each segment to the total length is plotted in one of the three axes that define a triangle. Measurements used were EB, ZB and AA, which were added (using raw data) to obtain the total length of the posterior member. The percentages were obtained by dividing each segment's length by the total hind limb length and multiplying the result by 100. Theoretically, any appendicular design can be plotted in this way, as long as none of its segments measure 0, thus avoiding the perimeter of the triangle (Gatesy & Middleton Reference Gatesy and Middleton1997; Middleton & Gatesy Reference Middleton and Gatesy2000). In the analyses performed here, the objective was to explore possible morphological similarities and discuss their functional and ecological implications.
All analyses were performed with Statistica v.6.0 and R v.2.13.1.
1.3. Geomorphometrics
It should be noted that classical morphometry has several problems: linear measurements are strongly correlated with size (Bookstein et al. Reference Bookstein, Chernoff, Elder, Humphries, Smith and Strauss1985) but, meanwhile, geomorphogeometric approaches have a clear protocol to remove the arithmetic effect of dimension (centroid size), whilst an analogue method using linear measurements is not so well-established. In addition, the homology of linear measurements is usually difficult to establish; the same measurements may represent very different forms and, finally, it is difficult to generate graphs representing the studied shape based on the linear measurements taken (Adams et al. Reference Adams, Rohlf and Slice2004). In addition, geomorphometric approaches are found to be very effective at the moment of capturing information about the shape of an organism (Zelditch et al. Reference Zelditch, Swiderski, Sheets and Fink2004).
Keeping these issues in mind, and also considering that the pelvis is a very complex structure (i.e., hard to represent through linear measurements), the terror birds' pelvises were analysed using 2D landmarks (homologous points), photographed from a dorsal and lateral view (Fig. 3; Table 2). X and y coordinates for each landmark were digitised using TpsDig 1.41 software (Rohlf Reference Rohlf2005). The resulting coordinates were subjected to a generalised procrustes analysis (GPA) to remove any information unrelated to shape (Rohlf & Slice Reference Rohlf and Slice1990; Zelditch et al. Reference Zelditch, Swiderski, Sheets and Fink2004). Localised shape changes were estimated using the partial warp scores (Bookstein Reference Bookstein1991), and once these values were obtained, a relative warps analysis (RWA) (Rohlf Reference Rohlf, Marcus, Bello and García-Valdecasas1993) was performed to examine the changes in shape of the pelvis using TpsRelw 1.35 (Rohlf Reference Rohlf2003) and MorphoJ v. 1.03c (Klingenberg Reference Klingenberg2011) software.

Fig. 3 Landmarks used in the study of birds' pelvis: (A) dorsal view; (B) lateral view.
Table 2 Pelvic landmarks used in the geomorphometric analysis (see Fig. 3)

The relative warps obtained were used to examine whether the morphological groups were consistent with the locomotor habit of the extant species.
1.4. Institutional abbreviations
AMNH, American Museum of Natural History, New York, USA; FM, Field Museum of Natural History, Chicago, USA; MACN, Museo Argentino de Ciencias Naturales “Bernardino Rivadavia”, Buenos Aires, Argentina; MLP, Museo de La Plata, Buenos Aires, Argentina; MMP, Museo Municipal de Ciencias Naturales Lorenzo Scaglia, Buenos Aires, Argentina; NHMUK, The Natural History Museum, London, UK; YPM, Yale Peabody Museum, New Haven, Connecticut, USA.
2. Results
2.1. Hind limb diversity: ternary plot
The studied group of birds includes 75 individuals of different species that occupy only 9 % of the total area of triangular space, distributed in an area equivalent with that of Gatesy & Middleton (Reference Gatesy and Middleton1997) (Fig. 4). No species are known to have femurs proportionally smaller than 27 % or bigger than 56 %. Tibiotarsus length varies between 37 % and 55 %, while the tarsometatarsus length is between 14 % and 45 %. In accordance with the proposal of Gatesy & Middleton (Reference Gatesy and Middleton1997), the tarsometatarsus is the largest contributor to the variation (31%), followed by the femur (29 %) and finally the tibiotarsus (18 %), which is the most conservative in its length. When plotted (Fig. 4A), the phorusrhacids are located next to the Otididae, Passeriformes, Polyborus plancus (Falconiformes), Tyto alba (Strigiformes) and Rheidae, and away from the Cariamidae and Sagittarius, to which they have traditionally been compared (e.g., Andrews Reference Andrews1899).

Fig. 4 Neornithes' hind limb proportions: (A) graph showing the area occupied by phorusrhacids; (B) simplified graphic with phorusrhacids subfamilies discriminated. Abbreviations: F = femur; Tbt = tibiotarsus; Tmt = tarsometatarsus.
The contribution of the femur, tibiotarsus and tarsometatarsus to the total length of the hind limb is identical in Psilopterinae and Patagornithinae, whilst in the Mesembriornithinae the tarsometatarsus is longer and the tibiotarsus is shorter. The femur of the Mesembriornithinae can be as long as in Psilopterinae–Patagornithinae, as in Mesembriornis or Llallawavis. In the latter, the tarsometatarsus is even longer, with a similar proportion to Rheidae (Fig. 4B).
2.2. Principal component analyses (PCA)
2.2.1. Femur
74 individuals from different species were included in the analysis. The first two components account for 99 % of the total variation. PC1 explains 98.5 % (Table 3; ). Whilst all variables have a strong contribution to this component, the cranial extension of the crista trochanterica (ED) and the cranio–caudal width of the condylus medialis (EH) have a slightly higher contribution. PC2 explains 0.5 % of the total variation in relation to femur length measurements (EA and EB) and the cranio–caudal diameter of corpus femoris (EG). In accordance with Campbell & Marcus (Reference Campbell and Marcus1992), birds are not separated by their locomotor habits, but rather by body size, represented here by body mass.
Table 3 Principal component analysis of the phorusrhacids and extant birds, analysed using femur, tibiotarsus, tarsometatarsus, complete hind limb and hind limb ratios measurements. Only the first five components are shown. For more details, see

PC1 is strongly influenced by body mass (R = 0.96; R2 = 0.93; P = 0.0000), and of the 98.5 % explained by this component, 93 % is explained by the mass of the animals analysed. It can be seen in Figure 5 that smaller birds are located to the right of the graph, whilst the largest are on the left. Phorusrhacids have negative values for PC1 and positive values for PC2. When plotted in a PC1–PC2 graph, phorusrhacids are separated into two groups (Fig. 5): Llallawavis, Mesembriornis and Patagornis are associated with terrestrial birds with cursor capacity such as Pterocnemia and Rhea; whilst the Psilopterinae Psilopterus lemoinei, P. bachmanni and Procariama simplex are grouped with flying birds, but with some connection to the terrestrial environment, whether waders or walkers (Grus, Ciconia, Aramus, Ardea and Penelope). Particularly striking is the membership to this group of the raptor Geranoaetus. PC1 distinguishes between the medium-sized phorusrhacids (Llallawavis, Mesembriornis and Patagornis) and the smallest ones (Psilopterinae).

Fig. 5 Principal component analysis of the femur: distribution of taxa in the morphospace defined by CP1 and CP2.
PC2 distinguishes the birds with stouter and shorter femurs (with negative values) from those with more slender and elongated femora (indicated by positive values). The Psilopterinae belong to this second group, whilst the medium-sized phorusrhacids have PC2 values closer to 0, thus indicating more robust femora. Emeus (Struthioniformes), traditionally considered as ‘graviportal’ (see Tambussi et al. Reference Tambussi, Picasso, Mosto, Ballent, Artabe and Tortello2010 for a discussion on the incorrect application of this term in birds), has negative values for both components.
2.2.2. Tibiotarsus
The analysis included 72 individuals from different species. The first two components account for 98.3 % of the total variation. PC1 explains 97 % of the variation (Table 3; ), mainly in relation to the latero-medial diameter of the corpus tibiotarsi (ZF), the cranio–caudal width of both condyli (ZH and ZI) and the cranial extension of the crista cnemialis cranialis (ZJ). Meanwhile, PC2 explains 1.3 % in relation to the tibiotarsi length measurements (ZA and ZB).
In the PC1–PC2 scatterplot (Fig. 6), a group formed of Patagornis, Llallawavis and terrestrial birds with cursor capacities (Pterocnemia, Rhea and Dromaius) can be separated from the group made up of Psilopterinae (P. lemoinei and P. simplex) and other terrestrial birds such as the Otididae Ardeotis kori, Ardeotis arabs and Otis tarda, and the Anseriformes Chauna torquata. These were all birds capable of flying (some are even migratory species from long distances), but that preferred to walk.

Fig. 6 Principal component analysis of the tibiotarsus: distribution of taxa in the morphospace defined by CP1 and CP2.
As with the femur, PC1 is strongly influenced by body mass (R = 0.96; R2 = 0.92; P = 0.0000): of the 97 % explained by PC1, 92 % is correlated with mass. The larger forms are located to the left of the graph, while the smaller are located to the right. In PC1, birds can also be distinguished according to their shaft and crista cnemialis cranialis: negative values correspond to birds with robust shafts and extended crista cnemialis cranialis.
PC2 allows the identification of birds according to the length of their tibiotarsus. Wading birds such as Phoenicopterus, Ciconia, Leptoptilos, Mycteria, Ardea and Aramus, and walkers such as Grus (which can also wade occasionally) and Sagittarius, have very long tibiotarsi (high positive values). Runners such as Cariamidae have positive values close to 0, indicating longer tibiotarsi than those of Phorusrhacidae. The smaller phorusrhacids have negative values close to 0, indicating the presence of longer tibiotarsi than those of the medium-sized terror birds.
2.2.3. Tarsometatarsus
76 individuals from different species were included in the analysis. The first two components account for 95.8 % of the total variation (Table 3; ). PC1 explains 88 % of the variation in tarsometatarsus length measurements (AA, AB and AC), whilst PC2 explains 7.8 % of length measurements (AA, AB and AC), latero–medial diameters (AF and AH) and divarication angles (AL, AM).
As in the case of tibiotarsus, in the PC1–PC2 plot (Fig. 7), a group composed of Patagornis, Llallawavis and terrestrial birds with cursor capacities (Pterocnemia, Rhea and Dromaius) can be observed, whereas P. lemoinei, P. bachmanni and P. simplex are grouped with land birds that prefer to walk, but have the ability to run, such as the Otididae Ardeotis kori, Ardeotis arabs and Otis tarda; although there are also other terrestrial wading birds associated, such as Chauna, Grus, Leptoptilos crumeniferus and L. dubius, and terrestrial walkers such as Sagittarius. The phorusrhacid Paraphysornis is associated with the Anseriformes Brontornis and the Struthioniformes Emeus. Phorusrhacos longissimus is not associated with any group, and is in between runners and ‘graviportal’ birds.

Fig. 7 Principal component analysis of the tarsometatarsus: distribution of taxa in the morphospace defined by CP1 and CP2.
Although PC1 is strongly influenced by body mass (R = 0.95; R2 = 0.90; P = 0.0000), it is less so than for the femur and tibiotarsus. Of the 95.8 % explained by PC1, 90 % is correlated with body mass. These results are consistent with Campbell & Marcus (Reference Campbell and Marcus1992), who stated that the tarsometatarsus is the bone that better reflects a bird's habit, since it is less influenced by mass. The more voluminous birds are located to the right, while the smaller are on the left of Figure 7.
With respect to PC2, positive values indicate long, slender tarsometatarsi with low angle divarication, whilst negative values indicate short, more robust tarsometatarsi shapes and a high divarication angle. In the case of phorusrhacid, the Psilopterinae, Llallawavis and Patagornis correspond to birds with long tarsometatarsi and with divarication angles which are lower than those of Paraphysornis, which has short tarsometatarsi with high divarication angles. Phorusrhacos has an intermediate length between Paraphysornis and Psilopterinae, but has a high divarication angle.
2.2.4. Complete hind limb
72 individuals from different species were analysed using a total of 33 measurements of the femur, tibiotarsus and tarsometatarsus. The first two components account for 96.5 % of the total variation (Table 3; ). PC1 explains 92.5 % of the variation related to the cranial extension of the crista trochanterica (ED), the cranio–caudal width of condyli (EH and EI), the latero–medial diameter of the corpus tibiotarsi (ZF), the cranial extension of the crista cnemialis cranialis (ZJ) and the tarsometatarsus length (AA, AB and AC). Meanwhile, PC2 explains 4 % of the variation of the tarsometatarsus length (AA, AB and AC) and divarication angles (AL and AM).
The PC1–PC2 plot (Fig. 8) shows that Patagornis marshi and Llallawavis scagliai are clearly grouped with landbirds with cursor capacities such as Dromaius, Rhea and Pterocnemia. P. simplex and P. lemoinei are associated with walker birds like the Otididae Ardeotis kori, Ardeotis arabs and Otis tarda, the Falconiformes Sagittarius, the Gruidae Grus, and the Ciconiidae Leptoptilos crumeniferus and L. dubius.

Fig. 8 Principal component analysis of the complete hind limb: distribution of taxa in the morphospace define d by CP1 and CP2.
PC1 is strongly influenced by body mass (R = 0.96; R2 = 0.92; P = 0.0000): of the 92.5 % explained by PC1, 92 % is correlated to body mass, whilst only 0.5 % is from shape. However, it should be noted that positive PC1 values indicate birds with projected femur condyli, cranially extended crista trochanterica, tibiotarsi with robust diaphysis, developed crista cnemialis cranealis and long tarsometatarsi.
As in the tarsometatarsus analysis, positive PC2 values indicate long, slender tarsometatarsi with a low divarication angle of the trochleae metatarsi. Phorusrhacids have values close to 0.
2.2.5. Hind limb ratios
Only by using the first seven components did the variation explained reach 90 % (Table 3; ). Due to the removal of the arithmetical effect of dimensions by using ratios, the first two components account only for 63.6 % of the total variation. PC1 explains 51.2 % of the variation in the ratios related to limb proportions (EB/AA and ZB/AA) and the robustness of the tarsometatarsus (AF/AG and AH/AI). PC2 explains 12.4 % of the variation related to hypotarsus development (AJ/AK), and PC3 explains 9.6 % in relation to the cranial development of the crista trochanterica (EC/ED), the relative development of the cristae cnemialis (ZJ/ZD) and the robustness of the tarsometatarsus (AJ/AK).
Phorusrhacids have values close to 0.4 for PC1 and PC2 (Fig. 9), and they appear to be associated with a very heterogeneous group consisting of birds such as Cariamidae, Grus, Sagittarius, Pitangus, Phoenicopterus, Podiceps and Ardeotis arabs, amongst others.
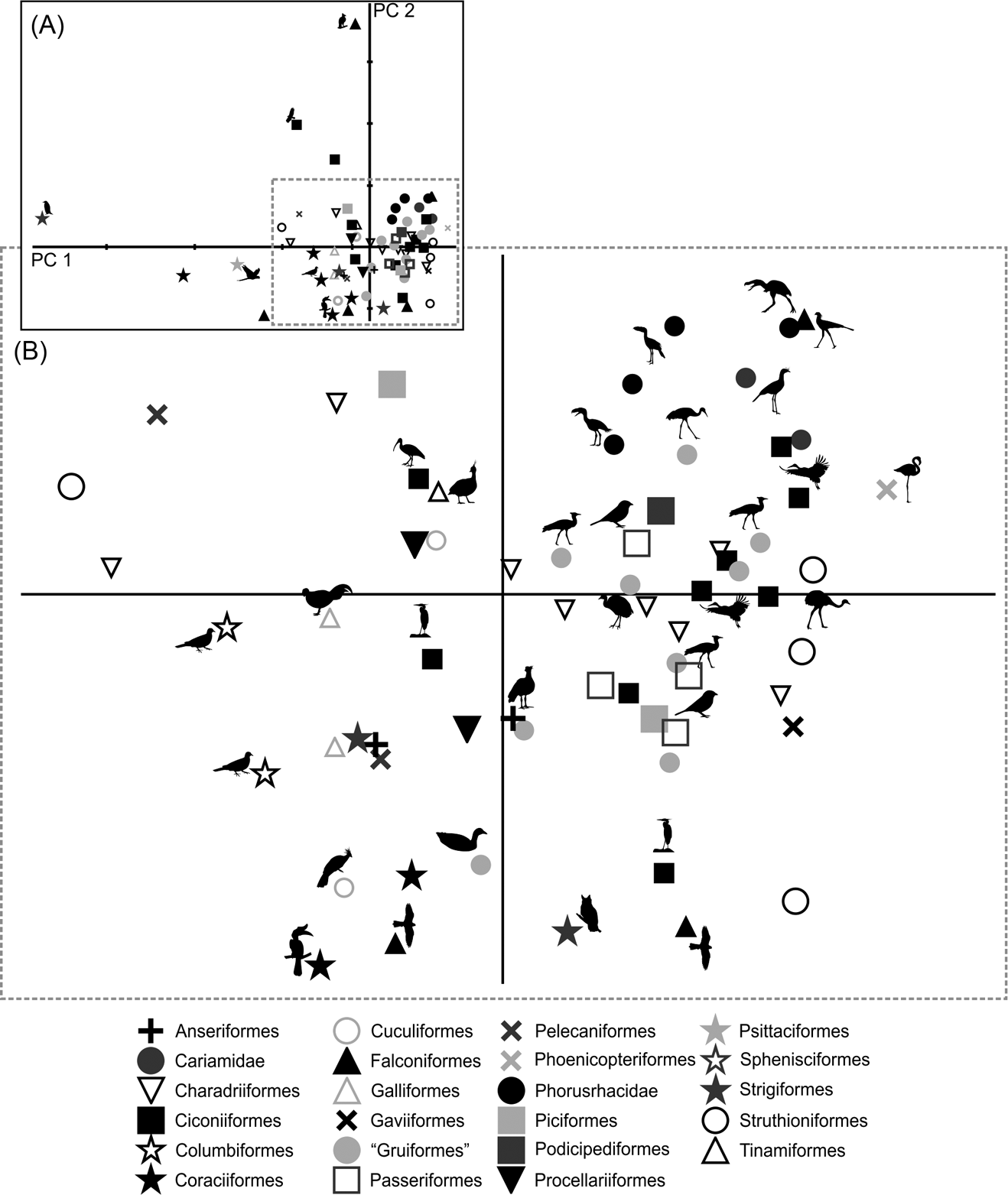
Fig. 9 Principal component analysis of the hind limb ratios: (A) distribution of taxa in the morphospace defined by CP1 and CP2; (B) detail of the principal area of taxa distribution in the morphospace.
PC1 separates birds with a shorter femora and a longer, slender tarsometatarsus. A positive PC2 value indicates a wider hypotarsus (high AJ/AK ratio), whilst negative values indicate a narrow hypotarsus (low AJ/AK ratio).
2.3. Discriminant analyses
2.3.1. Tarsometatarsus
The discriminant model using only the tarsometatarsus has an average probability of a correct classification of 67.3 % (model error: 0.20896; N = 67). The probability of a correct classification (CC) of each group is shown in Table 4. Probabilities that phorusrhacids belong to the groups used here are shown in Table 5.
Table 4 The probability of correct classification (CC) of each group based on the discriminant analysis performed on the tarsometatarsus

Abbreviations: G = ground birds; W = wading birds; X = “other birds”. Assignments according to Zeffer et al. (Reference Zeffer, Johansson and Marmebro2003)
Table 5 Phorusrhacids' probabilities of belonging to the groups used here based on the discriminant analysis made on the tarsometatarsus

Abbreviations: G = ground birds; W = wading birds; X = “other birds”
According to the tarsometatarsus measurements, phorusrhacids are more likely to belong to group G (ground birds; Fig. 10A) than to either of the other two groups, with a probability of 53 % (P. bachmanni) to 99 % (Phorusrhacos longissimus). Procariama, Patagornis, Llallawavis, Phorusrhacos and Paraphysornis have the highest probability of belonging to this group. The case of the Psilopterus species is not so categorical. Although the chances of this species being waders are very low, the likelihood of P. lemoinei belonging to the group made up of all other birds is not so low (21 %), and in the case of P. bachmanni it is almost 30 %. Furthermore, in an estimate of the probability of Brontornis belonging to any of the three groups, it is shown to have an 87 % chance of being a ground bird and a 12 % chance of belonging to group X.

Fig. 10 Discriminant analysis: (A) tarsometatarsus discriminant analysis, taxa distribution in morphospace defined by the discriminant variables 1 and 2; (B) Complete hind limb discriminant analysis, taxa distribution in morphospace defined by the discriminant variables 1 and 2. Phorusrhacids have been represented by silhouettes (which also indicate phorusrhacids' subfamily). Abbreviations: BB = Brontornis burmeisteri; G = ground birds; LL = Llallawavis scagliai; PA = Paraphysornis brasiliensis; PB = Psilopterus bachmanni; PH = Phorusrhacos longissimus; PL = Psilopterus lemoinei; PM = Patagornis marshi; PS = Procariama simplex; W = waders; X = “other birds”.
2.3.2. Hind limb
The complete hind limb model has an average probability of a correct classification of 60.26% (model error: 0.1791; N = 67). The probability of a correct classification (CC) for each group can be seen in Table 6, whilst the probability of phorusrhacids belonging to the groups studied here is shown in Table 7.
Table 6 The probability of correct classification (CC) of each group based on the discriminant analysis made on the hindlimb

Abbreviations: G = ground birds; W = wading birds; X = “other birds”. Assignments according to Zeffer et al. (Reference Zeffer, Johansson and Marmebro2003)
Table 7 Phorusrhacids' probabilities of belonging to the groups used here based on the discriminant analysis made on the hind limb

Abbreviations: G = ground birds; W = wading birds; X = “other birds”
Phorusrhacids should therefore belong to group G (Fig. 10B) of Zeffer et al. (Reference Zeffer, Johansson and Marmebro2003), with a 93 % probability for P. lemoinei and a 99 % probability for Patagornis and Llallawavis.
2.4. Pelvis geomorphometrics
2.4.1. Dorsal shape
69 individuals (including five phorusrhacids) were analysed using nine landmarks (Fig. 3A). The first three components account for 86.7 % of the total variation. PC1 explains 65.9 %, PC2 explains 14.2 % and PC3 explains 6.5 % (Table 8; ). Phorusrhacids are grouped with the birds which have a narrow, elongated pelvis, which includes terrestrial birds with cursor capacities such as Dromaius and Rhea. However, they are separated from other runners (Cariamidae) and from the walker birds (e.g., Otididae and Psophiidae) due to their longer pelvis, and from the aquatic birds Podiceps and Gavia, which have an even longer pelvis (Fig. 11). The exception is Andalgalornis, which has a pelvis which is similar in length to that of diving birds, but can be distinguished from them by the pelvis width (Fig. 12). The two morphotypes described by Degrange (Reference Degrange2012) are not separated.
Table 8 Principal component analysis of the pelvis in dorsal and lateral view. Only the first five components are shown. For more details, see

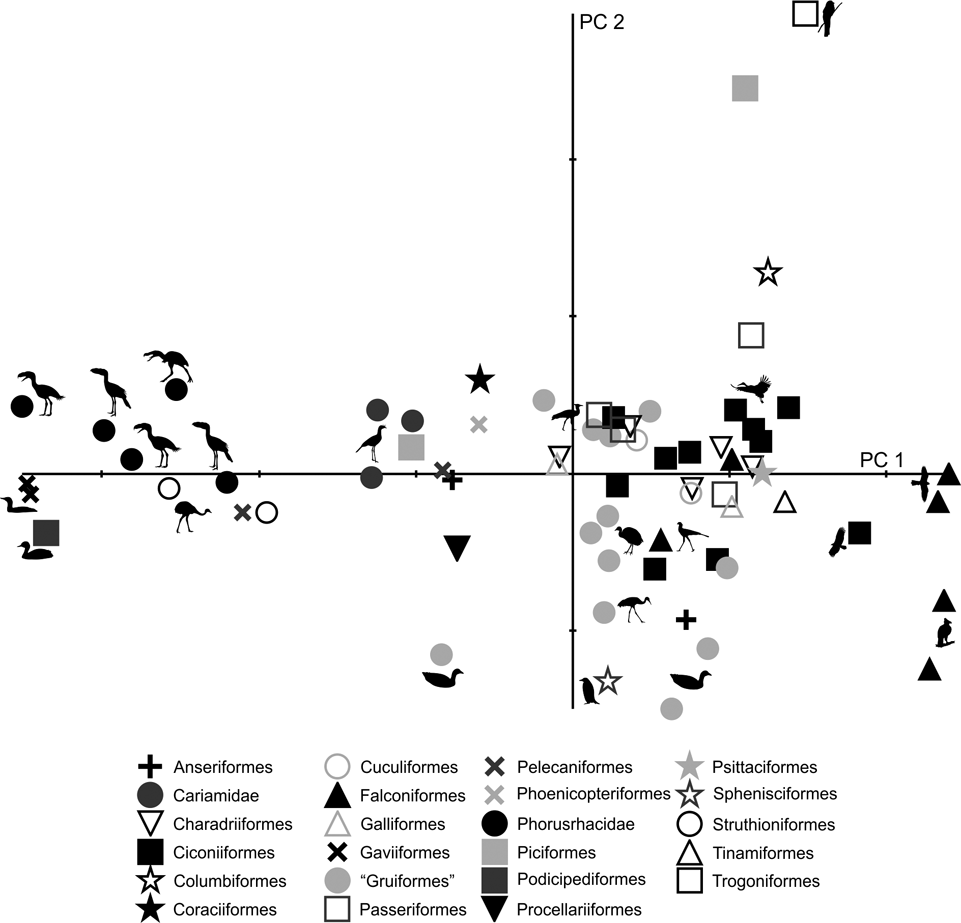
Fig. 11 Geomorphometric analysis of pelvis in dorsal view: distribution of taxa in the morphospace defined by CP1 and CP2.

Fig. 12 Deformation grid showing shape change of the pelvis (dorsal view) in different species.
PC1 is related to postacetabular length and pelvis width: negative values correspond to a narrow pelvis with a long postacetabular region, whereas positive values indicate a wide pelvis with a short postacetabular region. With some exceptions, this component distinguishes terrestrial birds with cursor capabilities (and swimming birds also) from non-cursorial terrestrial birds, and the non-cursorial terrestrial birds from waders. Cursorial birds have a very long postacetabular region, whilst the walking birds (Otididae and Psophiidae) have a shorter pelvis and a shorter postacetabular region, although to a lesser extent than the waders.
PC2 is dominated by pelvis width and preacetabular length: positive values indicate a wide pelvis with a short preacetabular region, whilst negative values indicate a narrow pelvis with a long preacetabular region. In this sense, phorusrhacids have a narrow pelvis with a relatively short preacetabular region.
2.4.2. Lateral shape
69 subjects (including five phorusrhacids) were analysed using 13 landmarks and 18 semi-landmarks (Fig. 3B). The first three components account for 71.94 % of the variation. PC1 explains 41.8 %, PC2 19.9 % and PC3 10.2 % (Table 8; ). Phorusrhacids share morphospace with Dromaius, Chunga, Cariama, Gavia, Podiceps, Phalacrocorax and Ramphastos, which are all birds with different locomotor habits, but with the peculiarity of having very long postacetabular regions (Figs 13, 14). No pelvic morphotypes were discerned.

Fig. 13 Geomorphometric analysis of pelvis in lateral view: distribution of taxa in the morphospace defined by CP1 and CP2.

Fig. 14 Deformation grid showing shape change of the pelvis (lateral view) in different species.
PC1 is related to the proportion between the extension of the postacetabular and preacetabular regions: negative values indicate a long preacetabular region and a short postacetabular region, whereas positive values indicate a short preacetabular region and a long postacetabular region. Phorusrhacids have a pelvis with a very long postacetabular region and a very short preacetabular region. This component separates phorusrhacids from terrestrial runners (Cariamidae) and walkers (Otididae) and from the waders, whose pelvis is even shorter.
PC2 is primarily related to the height of the postacetabular region, essentially through the extension of the processus terminalis ischii: positive values indicate a pelvis which is poorly extended ventrally, while negative values indicate a pelvis which is highly extended ventrally. Phorusrhacids have a high pelvis, but the processus terminalis ischii is poorly extended ventrally.
3. Discussion and conclusions
3.1. Locomotor habits and substrate preferences
The development of the anterior locomotor module of birds relaxed the pressures on the evolution of the posterior appendicular module, thus allowing a diversification of lifestyle habits (Gatesy & Middleton Reference Gatesy and Middleton1997), from those of the hyper-aerial birds (e.g., Apodidae) to those of the exclusively terrestrial birds (e.g., Rheidae). Although the basic functions of bird legs are always landing, taking-off and walking (Habib & Ruff Reference Habib and Ruff2008; Abourachid & Höfling Reference Abourachid and Höfling2012), between these extremes all birds have a wide variety of locomotor habits and occupy a wide variety of environments. Locomotor behaviour can also change according to the animal's activity. Flying birds are capable of walking, running, hopping, jumping, wading, swimming or diving. In his book on primates, Oxnard (Reference Oxnard1984) claimed that a fixed locomotor categorisation is impossible, since each animal uses a wide spectrum of locomotor modes. In extant birds, a simple direct observation can identify whether a bird is able to fly or not, although the ability to fly does not necessarily mean that it will be classified as a flying bird. Some birds are ubiquitous, while others are selective in choosing the environment they frequent. Examples are the Tinamidae, the Otididae, the Psophiidae and the Cariamidae, which, although they are able to fly, are considered terrestrial or land birds because most of their time is spent on the ground. Similarly, the Anatidae and Podicipedidae are also able to fly, but they are considered swimmers because they spend much of their time in the water. Complexity of animal behaviour is so broad that it becomes almost impossible to define unique locomotor habits. Moreover, as more is learned about animal ethology, the boundaries between behavioural categories are dissolved, thus defining a continuum of possible locomotor habits (Carrano Reference Carrano1999), precluding their strict classification. This scenario complicates the assumptions that can be made from appendicular morphology, since this does not necessarily have a straightforward relationship with locomotor diversity or a particular habit.
There is also confusion within published literature about what a locomotor habit is, and also about the substrate type and use. This is not exclusive to birds, and affects other vertebrates such as mammals (Toledo et al. Reference Toledo, Bargo, Cassini and Vizcaíno2012). For example, the term ‘arboreal’ (i.e., lives in trees) is often referred to as a locomotor habit in certain species of Passeriformes capable of flying, when the correct locomotor term is ‘climber’. Arboreal, in fact, refers to the preferred substrate. To explore an arboreal environment, birds use several types of locomotor abilities, such as walking on perches (e.g., psittaciforms), climbing branches for foraging (e.g., woodpeckers) and hopping on perches (passerines and toucans) (Abourachid & Höfling Reference Abourachid and Höfling2012). Even the term ‘predatory’ or ‘birds of prey’ is used as a mode of locomotion (e.g., Zeffer et al. Reference Zeffer, Johansson and Marmebro2003) for birds such as the Falconidae and Accipitridae, when in fact these birds travel through flight.
Despite this obscure scenario, some generalisations are possible; for example, a well developed posterior locomotor module is strongly linked to a terrestrial environment (Dial Reference Dial2003). This is the case in phorusrhacids, whose very well developed posterior locomotor module is accompanied by a reduction of the forelimbs, thus indicating that most of the phorusrhacids were obligate terrestrial birds, since they were unable to fly.
Terrestrial birds, or ‘ground birds’, as described by Zeffer et al. (Reference Zeffer, Johansson and Marmebro2003), are those that predominantly use their hind limbs for locomotion on the ground (i.e., the substrate), whether they hop, jump, walk or run (i.e., the locomotor mode). Birds move on the ground by walking, running and hopping (Hutchinson & Gatesy Reference Hutchinson and Gatesy2001; Alexander Reference Alexander2004), depending on their speed; at low speeds they walk, while at intermediate or faster speeds they can run or hop (Hayes & Alexander Reference Hayes and Alexander1983; Verstappen et al. Reference Verstappen, Aerts and van Damme2000; Verstappen & Aerts Reference Verstappen and Aerts2000). Wading birds (or waders), defined as those that feed while walking in waterlogged soils (i.e., wading), are technically terrestrial birds because their mode of locomotion is walking (Storer Reference Storer, Farner and King1971; Raikow Reference Raikow, King and McLelland1985). However, within the literature published on this subject, they are commonly separated from terrestrial birds (e.g., Zeffer et al. Reference Zeffer, Johansson and Marmebro2003).
With this in mind, it is evident that there is a mismatch between the assignments of a habitat through the observation of the anterior and posterior locomotor modules. As the locomotor habit or style and the substrate on which a bird lives are not easy to identify, many ecomorphological studies use the birds' ‘preferred’ locomotor habit and mode of locomotion.
The recognition of some locomotor patterns in recent species has also been attributed to extinct taxa based on qualitative comparisons of skeletal features (e.g., Hinić-Frlog & Motani Reference Hinić-Frlog and Motani2009). As previously stated, forelimb reduction in some species, associated with high body mass, indicates that most phorusrhacids were unable to fly (Ameghino Reference Ameghino1895; Andrews Reference Andrews1899; Sinclair & Farr Reference Sinclair, Farr and Scott1932; Alvarenga & Höfling Reference Alvarenga and Höfling2003; Chiappe & Bertelli Reference Chiappe and Bertelli2006). Several authors have stated that, beyond any doubt, phorusrhacids were cursorial birds (Alvarenga & Höfling Reference Alvarenga and Höfling2003; Blanco & Jones Reference Blanco and Jones2005; Chiappe & Bertelli Reference Chiappe and Bertelli2006) which were unable to fly (Alvarenga & Höfling Reference Alvarenga and Höfling2003), or that the smaller species were able to fly for very short distances in a clumsy manner (Tonni Reference Tonni1977; Tonni & Tambussi Reference Tonni and Tambussi1988; Tambussi & Noriega Reference Tambussi, Noriega and Arratia1996; Mourer-Chauviré et al. Reference Mourer-Chauviré, Tabuce, Mahboubi, Adaci and Bensalah2011; Degrange Reference Degrange2012; Degrange et al. Reference Degrange, Noriega and Vizcaíno2015). This indicates that they were obligate terrestrial birds (Degrange Reference Degrange2012). However, phorusrhacid terrestriality may include different non-exclusive styles such as jumping or hopping, walking, running or even wading. Although it has been established that the hind limb length defines the cursorial ability of phorusrhacids (e.g., Tambussi & Noriega Reference Tambussi, Noriega and Arratia1996; Alvarenga & Höfling Reference Alvarenga and Höfling2003; Blanco & Jones Reference Blanco and Jones2005), the fact that a very long tarsometatarsus is shared by cursorial, walking and wading birds means that we must question the sole use of hind limb metrics to reflect phorusrhacid's cursoriality.
3.2. Hind limb proportions
Limb proportions represent a key feature in the design of any limb (Gatesy & Middleton Reference Gatesy and Middleton1997; Middleton & Gatesy Reference Middleton and Gatesy2000; Gatesy et al. Reference Gatesy, Bäker and Hutchinson2009; Abourachid & Höfling Reference Abourachid and Höfling2012), and they have been used to categorise mammals specialised in racing, digging or weight support (Gregory Reference Gregory1912; Osborn Reference Osborn1929; Smith & Savage Reference Smith and Savage1956; Garland & Janis Reference Garland and Janis1993; Gebo & Rose Reference Gebo and Rose1993; Carrano Reference Carrano1997, Reference Carrano1999), and also as an indicator of cursoriality in dinosaurs (e.g., Osborn Reference Osborn1916; Holtz Reference Holtz1994; Ostrom Reference Ostrom1976; Coombs Reference Coombs1978; Sereno et al. Reference Sereno, Dutheil, Iarochene, Larsson, Lyon, Magwene, Sidor, Varricchio and Wilson1996) and even in birds (Gatesy & Middleton Reference Gatesy and Middleton1997; Zeffer et al. Reference Zeffer, Johansson and Marmebro2003). However, the actual information on locomotor habits that this can provide has been seriously questioned, particularly in the case of Neornithes. Several studies indicate that the ratios between the leg elements, or the hind limb total length, are not in themselves indicative of the type of locomotion (Gatesy & Middleton Reference Gatesy and Middleton1997; Zeffer et al. Reference Zeffer, Johansson and Marmebro2003; Habib & Ruff Reference Habib and Ruff2008). Hind limb proportions differ between bird species: the lengths of the femur, tibiotarsus and tarsometatarsus are proportionally different (Abourachid & Höfling Reference Abourachid and Höfling2012). It is also not new that birds with long legs can live in very different habitats. For example, the group LL (long-legged) (Campbell & Marcus Reference Campbell and Marcus1992) includes a very heterogeneous group of birds that share ‘only’ the great length of their legs; for example, Ciconiidae, Gruidae and Rheidae.
The critical analysis of the correlation between limb segment proportions and habitat use is also important (Abourachid & Höfling Reference Abourachid and Höfling2012). A running bird such as the greater rhea (Rhea americana) has a tarsometatarsus and a tibiotarsus equally as long as those of the secretary bird Sagittarius, which is not cursorial and therefore frequents grasslands, and of the flamingo Phoenicopterus, which is clearly a wading bird.
Patagornithinae and Psilopterinae hind limb proportions resemble those of terrestrial birds that walked like Otis (Otididae), whilst at the other end of the size range is the Passeriformes Turdus (Turdidae). By contrast, Mesembriornithinae proportions are similar to those of terrestrial cursorial birds, such as Rheidae. Interestingly, the seriemas Chunga and Cariama have different proportions to phorusrhacids (Fig. 4).
In a hypothetical Psilopterus–Rhea–Cariama series, there is a progressive increase in the relative length of the distal segments of the leg (tibiotarsus and tarsometatarsus), involving a progressive reduction in the relative length of the proximal segment (femur). Seriemas (Cariamidae), which can reach high speeds (40 km/h) and are considered agile runners (Gonzaga Reference Gonzaga, del Hoyo, Elliot and Sargatal1996), have a longer tibiotarsus than tarsometatarsus, short II and IV toes, and a longer third toe. Both Psophia (Psophiidae) and Otis (Otididae) have a similar hind limb proportion to that of Psilopterus, and can run and fly, although they more often walk (Collar Reference Collar, del Hoyo, Elliot and Sargatal1996; Sherman Reference Sherman, del Hoyo, Elliot and Sargatal1996).
To sum up, based solely on the proportions of hind limb elements, it is not possible to establish unequivocally that all phorusrhacids were birds with cursorial capacities.
3.3. PCA and discriminant analysis
In all of the PC analyses performed using linear and angular measurements (femur, tibiotarsus, tarsometatarsus and complete hind limb), PC1 is strongly influenced by body mass, and it can be seen that the larger birds appear in one extreme of the graphs (Figs 5–8). However, it is clear that a correlation between body mass and terrestriality exists, since larger birds with greater body mass tend to spend more time on the ground than in the air (e.g., Collar Reference Collar, del Hoyo, Elliot and Sargatal1996; Alexander Reference Alexander1998). There is a threshold value of body mass above which a bird cannot take off. This value is between 12–14 kg (Pennycuick Reference Pennycuick1989) and 16 kg (Pennycuick Reference Pennycuick2007). This is reflected in the graphs which match the distribution of larger size birds with those that are more associated with a terrestrial environment (whether they walk, run or wade).
In all analyses performed here, the Psilopterinae shared morphospace with more walker terrestrial birds such as Otididae (Otis and Ardeotis), but they are also associated with Leptoptilos crumeniferus, which is a wading bird according Zeffer et al. (Reference Zeffer, Johansson and Marmebro2003). Meanwhile, Patagornithinae and Mesembriornithinae are associated with Rheidae and Casuariidae, which are terrestrial birds with clearly cursorial capacities (e.g., Picasso Reference Picasso2010).
Concerning the femur, it is clear that body mass has a high influence on its morphology (Campbell & Marcus Reference Campbell and Marcus1992; Zeffer et al. Reference Zeffer, Johansson and Marmebro2003). However, PC2 sorts birds according to the shape of their femurs: from long and slender to short and robust. Patagornithinae and Mesembriornithinae have a similar morphology to Rheidae, whilst the Psilopterinae – which are similar in size to Otididade (PC1) – have longer and more slender femurs. Wading birds have more robust and shorter femurs (e.g., Phoenicopterus) or, on the contrary, more slender and thinner femurs (e.g., Ardea and Ciconia) than phorusrhacids.
Psilopterinae's tibiotarsus metrics are identical to those of the walkers Otididae, and quite different from those of runners such as Cariamidae, whilst the metrics for Patagornithinae and Mesembriornithinae are similar to those of Rheidae and Casuariidae. Psilopterinae have a cranial extension of the cristae cnemialis and a robustness of the shaft similar to typically wading birds, but the tibiotarsus is shorter. Mesembriornithinae and Patagornithinae have a greater cranial extension of the cristae cnemialis cranialis, and more robust and shorter tibiotarsi than wading birds.
Tarsometatarsus morphology is strongly influenced by the habits of an animal (Campbell & Marcus Reference Campbell and Marcus1992). However, it is evident in Figure 7 that waders, walkers and some runners (e.g., Cariamidae) have a very similar morphology; Psilopterinae are included in this association. Some waders, such as the flamingo, have a markedly longer tarsometatarsus than phorusrhacids, with less divergent trochleae metatarsi, whilst others such as Leptoptilos or Grus, have a very similar length and trochlear divarication. Mesembriornithinae and Patagornithinae have tarsometatarsi with similar morphometry to that of the cursorial Rheidae and Casuariidae. Paraphysornis and the Anseriformes Brontornis are associated with the moa Emeus, which is a fossil Palaeognathae considered ‘graviportal’, due to its short and robust tarsometatarsi with divergent trochleae. Phorusrhacos has shorter and more robust tarsometatarsi than Patagornithinae (Alvarenga & Höfling Reference Alvarenga and Höfling2003).
When analysing the complete hind limb, it is observed that Mesembriornithinae and Patagornithinae are associated with cursorial birds, but Psilopterinae are associated with walkers and waders. Even the extant Cariamidae appears to be associated with Mycteria and Ciconia, which are considered to be wading birds. This PCA, in particular, shows that the distinction between wading birds and terrestrial birds using hind limb metric variables is extremely difficult, and quite arbitrary. This is more evident when using rates, in which the segregation of the groups is not so clear. In other words, from a morphometric viewpoint, a wading bird can be a terrestrial bird and a terrestrial bird can be a wader (Fig. 10B). This makes more sense when we take into account the fact that terrestrial and wading birds differ in the environment or substrate that they frequent, but not in the locomotor habit or style, since both walk. This finding is reinforced by the reclassifications made in the discriminant analysis carried out here. For example, in Figure 10 it can be appreciated that there is no clear separation between wading birds and terrestrial birds. However, both analyses predicted that phorusrhacids correspond to the terrestrial bird group. The prediction probabilities are generally higher for membership to group G (‘ground birds’) and lower for group W (‘wading birds’). However, the discriminant analysis performed on the tarsometatarsus predicted that P. bachmanni was a ground bird with a probability of 53 %, but it has a probability of about 30 % of belonging to the heterogeneous group X (consisting of climbing birds, splatterers, divers and raptors), which may indicate that the hind limb of P. bachmanni is morphometrically generalist.
3.4. Pelvis morphometrics
The geomorphometric analysis performed showed that the pelvis, and particularly the postacetabular length, is a better indicator than the hind limb for discerning locomotor habits. With some exceptions (e.g., Ramphastidae), it can be stated that a long postacetabular region belongs to walking, running or diving birds. The seriemas (considered as runners) have a higher pelvis and a longer postacetabular region than Psophiidae and Otididae (considered as walking birds). In turn, these last examples have a much longer pelvis than the wading birds (with a very short postacetabular region). Phorusrhacids have a long, high pelvis with a very long postacetabular region. This combination is unique to these birds and clearly separates them from aquatic birds (such as Podicipedidae and Gaviidae), which also have a long postacetabular region. Postacetabular extension in phorusrhacids is related to a greater development of the hip extensor muscles (Degrange Reference Degrange2012), which is a feature of birds with cursorial capabilities (Picasso Reference Picasso2010).
4. Concluding remarks
Interpreting limb function represents a step forward in elucidating the palaeobiology of vertebrates (Vizcaíno et al. Reference Vizcaíno, Bargo, Fariña, Vizcaíno and Loughry2008, Reference Vizcaíno, Bargo, Kay, Fariña, Di Giacomo, Perry, Prevosti, Toledo, Cassini and Fernicola2010).
It is evident that appendicular morphometry is not 100 % reliable when distinguishing the different locomotor habits of birds. If hind limb morphology of a ground bird is in many cases metrically indistinguishable from that of a wading bird (and vice-versa), then why could not at least some of the phorusrhacids have been waders?
Undoubtedly, qualitative morphology remains the key when morphometry leads to ambiguous answers. Birds fly with forelimbs and move on land only with their hind limbs. In fact, in general, they can exhibit excellent performance and ground mobility regardless of their flight capabilities (Paul Reference Paul2002). If the hind limb of the Otididae (bustards) is analysed on its own, it can be incorrectly assumed that it is a terrestrial bird unable to fly; whilst, on the contrary, bustards are flying birds, and some species even migrate (Collar Reference Collar, del Hoyo, Elliot and Sargatal1996). However, it is true that cursoriality is associated with forelimb reduction (Coombs Reference Coombs1978; Kubo & Kubo Reference Kubo and Kubo2012). Ratites with cursorial capabilites have an elongated, narrow pelvis, which is a feature shared with diving birds, but they also have elongated tibiotarsi and tarsometatarsi, which is a characteristic shared with wading birds (that don't have an elongated pelvis), but not with divers. The preacetabular region is short compared with the postacetabular, and the height of the pelvis is higher in cursorial Ratites. In conclusion, the shape of the pelvis and hind limbs, coupled with the development of the cristae cnemialis and the shape and proportions of the toes, also gives us an idea of the mode of locomotion. Obviously, it is the joint study of the whole posterior locomotor module (pelvis+hind limb) and the anterior locomotor module which truly reveals the preferential mode of locomotion used by a bird.
The hind limbs act together with the pelvis, and it is precisely this whole structure (i.e., the posterior locomotor module) which better reflects the phorusrhacid's locomotor habit. The long, high pelvis, the caudally extended postacetabular region and the highly developed, laterally extended antitrochanter (a unique structure to birds related to body support and balance maintenance during biped locomotion; Hertel & Campbell Reference Hertel and Campbell2007) are indicators of terrestrial habit with cursorial capacities. In walking birds, the antitrochanter is also very well developed, but the pelvis is lower and less elongated. Wading birds have a low pelvis, with a very short postacetabular region and a poorly developed antitrochanter. Particularly in phorusrhacids, the lateral development of this structure reveals its relationship with the huge body mass of some of these animals; whilst its degree of verticalisation limits lateral movement of the femur, thus preventing abduction and maximising the cranio–caudal movements of the femur during locomotion.
A bird's feet are also indicative of its locomotor habit. It has been generalised that large birds associated with the terrestrial environment often have shorter digits and fewer toes (at least in the more specialised birds), thus minimising contact with the ground (Raikow Reference Raikow, King and McLelland1985). Wading birds in turn, are characterised by long toes, leading to an increase in surface contact with the swampy ground (Storer Reference Storer, Farner and King1971; Raikow Reference Raikow, King and McLelland1985). Some waders have interdigital membranes between toes II, III and IV (e.g., flamingos and ducks), whilst others have a very elongated toe I, which is not elevated and is in contact with the substrate (e.g., herons). Phorusrhacids have three relatively short toes II–IV (Jones Reference Jones2010; Degrange Reference Degrange2012) and a small, elevated toe I (Fig. 15), which is a feature related with terrestrial habits (Raikow 1985; del Hoyo et al. Reference del Hoyo, Elliot and Sargatal1996). They are therefore considered tridactyl, in the sense described by Raikow (1985). This type of foot minimises friction with the ground during a race and is not suitable for mobility in waterlogged soils.

Fig. 15 Phorusrhacid feet: (A) Psilopterus colzecus, MLP 76-VI-12-2, left foot; (B) Procariama simplex, MACN Pv 8225, right foot; (C) Procariama simplex, FM-P 14525, left foot; (D) Mesembriornis incertus, FM-P 14422, right foot; (E) Patagornis marshi, AMNH 9264, right foot. Shaded areas represent missing bones or parts of bones. Roman numerals indicate finger number. Scale bars = 1 cm.
In conclusion, phorusrhacids possess morphological attributes in the posterior appendicular complex that are associated with cursorial locomotion: the high pelvis and elongated postacetabular region; a very developed antitrochanter; elongated tibiotarsi and tarsometatarsi; the high development of the cristae cnemialis of the tibiotarsus; three relatively short digits pointing forward; and the elevated position of digit I, which does not touch the ground. This is consistent with the findings of Tambussi (Reference Tambussi1997), who demonstrated through an analysis of athletic capacity rates that some phorusrhacids had the same cursorial capacities as an ostrich. Cursoriality in large-sized birds is achieved with long strides through more elongated hind limbs, whilst smaller sized cursorial birds have shorter hind limbs, but a higher stride frequency (Storer Reference Storer, Farner and King1971; Gatesy & Biewener Reference Gatesy and Biewener1991; Abourachid & Renous Reference Abourachid and Renous2000; Picasso Reference Picasso2010). This shows that there is a limitation between size and, secondarily, leg length. The large sizes attained by some phorusrhacids, together with their elongated hind limbs, allowed them to develop long strides and thus succeed in a race.
Finally, to study limb adaptations in fossil birds, a more holistic study of the whole posterior locomotor module is necessary, with an emphasis on qualitative features, since morphometrics leaves some issues unresolved. A comparison with the wings is also needed, in order to make a more complete analysis of locomotor behaviour.
5. Acknowledgments
My warmest acknowledgment belongs to C.P. Tambussi for her constant support and advice during my PhD Thesis. A. Scarano helped selflessly in statistical subjects. My thanks to the following for allowing and facilitating the consultation of the collections under their management: L. Chiappe and K. Johnson from the Natural History Museum of Los Angeles County (Los Angeles); W. Simpson, J. Holstein and K. Lawson from the Field Museum of Natural History (Chicago); C. Norris, D. Brinkman and M. Fox from Yale Peabody Museum (New Haven); C. Mehling from the American Museum of Natural History (New York); A. Kramarz from the Museo Argentino de Ciencias Naturales “Bernardino Rivadavia” (Buenos Aires); M. Reguero from the Museo de La Plata (La Plata); and A. Dondas from the Museo Tradicional Lorenzo Scaglia (Mar del Plata). S. Chapman (The Natural History Museum, London) provided photos of the Phorusrhacidae deposited in the NHM. J. Noriega, C. Morgan and J. Desojo provided very useful comments and corrections. N. Toledo provided very enlightening discussions on locomotor habits and substrate preferences in different vertebrates. Gabrielle McLellan made substantial improvements to the English. This paper is part of my doctoral dissertation (National University of La Plata) realised at the División Paleontología Vertebrados (Museo de La Plata) and funded by a CONICET doctoral scholarship.