Introduction
Rabies is a neglected tropical disease [1] that has the highest mortality rate of all known infectious agents [Reference Radhakrishnan, Vanak, Nouvellet and Donnelly2]. Over 99% of cases of human rabies are caused by an infected dog bite [1], and as a result, the World Health Organization (WHO), Food and Agriculture Organization (FAO), and the World Organization for Animal Health (WOAH) have prioritised rabies as a model disease for a One Health approach. These agencies have launched the ‘United Against Rabies Forum’ which prioritises investments in rabies control, and coordinates global efforts to achieve zero human deaths from dog-mediated rabies by 2030 [3]. In this contribution, we use optimisation techniques to show that the most cost-effective vaccination strategies in countries with free-roaming dogs will include the use of oral rabies vaccine baits.
The majority of human rabies deaths occur in Africa and Asia, where free-roaming dogs are the primary means of transmission. India has a large free-roaming dog population, and probably accounts for 36% of human rabies deaths [4]. Rabies deaths are, in general, under-reported [Reference Warrell5], and until recently rabies was not a notifiable disease in India [6]. Therefore, the estimated number of deaths in India of 18,000–20,000 per year [4] is probably too low.
Timely post-exposure prophylaxis treatment (PEP) is almost 100% effective in preventing death [7], and treatment costs comprise ∼ 83% of the total rabies control budget in both Asia and Africa [Reference Knobel, Cleaveland, Coleman, Fèvre, Meltzer, Miranda, Shaw, Zinsstag and Meslin8]. About 29 million PEP are delivered each year [1], with 9 million in India [Reference Hampson, Coudeville, Lembo, Sambo, Kieffer, Attlan, Barrat, Blanton, Briggs, Cleaveland, Costa, Freuling, Hiby, Knopf, Leanes, Meslin, Metlin, Miranda, Müller, Nel, Recuenco, Rupprecht, Schumacher, Taylor, Vigilato, Zinsstag and Dushoff9]. The disease is disproportionately found in poor rural populations, for whom treatment costs, currently estimated at USD $108 including travel and loss of income, may be a heavy burden [1]. Most charitable donations associated with rabies control are commitments to contribute to the costs of PEP in endemic regions (e.g., [Reference Warrell5]).
However, the WHO suggests that dog vaccination is the most cost-effective strategy for preventing rabies in people, and reduces both human deaths and the need for PEP [1]. Programmes in Tanzania, the Philippines, and South Africa found that while costs per dog vaccinated varied (~$1.18 - $15.62 2012 USD), they were much lower than costs of PEP ($44.91 - $64.38 2012 USD) [Reference Elser, Hatch, Taylor, Nel and Shwiff10]. A recent cost comparison in Chad also suggests mass vaccination of dogs has approximately double the cost-effectiveness per DALY averted compared to PEP alone [Reference Mindekem, Lechenne, Naissengar, Oussiguéré, Kebkiba, Moto, Alfaroukh, Ouedraogo, Salifou and Zinsstag11]. Investment in dog vaccination, however, accounts for less than 1.5% of the global economic burden of the disease, and until recently, large-scale dog vaccination activities in India accounted for less than 0.5% of the estimated economic burden of the disease [Reference Hampson, Coudeville, Lembo, Sambo, Kieffer, Attlan, Barrat, Blanton, Briggs, Cleaveland, Costa, Freuling, Hiby, Knopf, Leanes, Meslin, Metlin, Miranda, Müller, Nel, Recuenco, Rupprecht, Schumacher, Taylor, Vigilato, Zinsstag and Dushoff9].
Annual vaccination of over 70% of the dog population can stop the transmission and eventually lead to the elimination of rabies if repeated over several years [Reference Coleman and Dye12], while other strategies, such as culling, are less effective [Reference Arief, Hampson, Jatikusumah, Widyastuti, Basri, Putra, Willyanto, Estoepangestie, Mardiana, Kesuma, Sumantra, Doherty, Salman, Gilbert and Unger13, Reference Morters, Restif, Hampson, Cleaveland, Wood and Conlan14]. Mass vaccination campaigns targeting dogs have been highly successful in many countries. For example, the USA was able to eliminate the canine rabies variant in the late 1970s and again in the 2000s [15]. Widely used strategies for dog vaccination include central point vaccination and door-to-door vaccination. In central point vaccination (CP), dog owners bring their pets to a central location such as a veterinarian’s office. Door-to-door (DD) strategies are where teams move from home to home to vaccinate dogs that can be handled by their owners. These two strategies have only been successful at large scales where most dogs are responsibly owned (e.g., Latin America [Reference Vigilato, Cosivi, Knöbl, Clavijo and Silva16] and the USA [15]).
In countries like India, where there are many free-roaming dogs that may not be owned [Reference Tiwari17], catch-vaccinate-release techniques (CVR) also have been employed. For animals that cannot be easily handled, CVR entails a team of people (4–7) capturing the animal in a net, injecting the vaccine, and then releasing it. For example, in 2013, the charity Mission Rabies (https://missionrabies.com/) conducted synchronised mass dog vaccination campaigns in 12 Indian cities using CVR and vaccinated 54,227 dogs [Reference Gibson, Wallace, Rahman, Bharti, Isloor, Lohr, Gamble, Mellanby, King and Day18]. However, there is no example of a large-scale national campaign that relies primarily on CVR. Wallace et al. [Reference Wallace, Cliquet, Fehlner-Gardiner, Fooks, Sabeta, Setién, Tu, Vuta, Yakobson, Yang, Brückner, Freuling, Knopf, Metlin, Pozzetti, Suseno, Shadomy, Torres, Vigilato, Abela-Ridder and Müller19] and Gibson et al. [Reference Gibson, Wallace, Rahman, Bharti, Isloor, Lohr, Gamble, Mellanby, King and Day18] suggest that the labour force required for such a campaign is prohibitively large.
Instead, several authors promote oral rabies vaccine bait handouts (ORV) as a key strategy in the control of canine rabies where there are large populations of free-roaming dogs [Reference Gibson, Yale, Vos, Corfmat, Airikkala-Otter, King, Wallace, Gamble, Handel, Mellanby, Bronsvoort and Mazeri20, Reference Rupprecht, Kuzmin, Yale, Nagarajan and Meslin21, Reference Wallace, Cliquet, Fehlner-Gardiner, Fooks, Sabeta, Setién, Tu, Vuta, Yakobson, Yang, Brückner, Freuling, Knopf, Metlin, Pozzetti, Suseno, Shadomy, Torres, Vigilato, Abela-Ridder and Müller19]. This method involves providing attractive oral rabies vaccine baits to animals that cannot be easily handled, observing consumption or removing the bait if rejected. The WHO has been recommending ORV as a complementary measure to reach inaccessible dogs since at least 1998 [22]. Moreover, oral baits dispersed in the environment have been used successfully in North America and Europe to control rabies in a variety of wildlife species, and have resulted in a net savings in disease control costs (e.g., [Reference Slate, Chipman, Rupprecht and DeLiberto23]). India formally endorsed the use of ORV for a WHO-recommended dog oral vaccine, SAG2, in 2007, but the costs of commercial vaccine baits exceeded funding [Reference Rupprecht, Kuzmin, Yale, Nagarajan and Meslin21].
It is likely, however, that the total costs of ORV vaccination of free-roaming dogs will be lower than those of CVR. Mission Rabies conducted a pilot test of oral baiting in Goa, India, in 2018, where they compared ORV and CVR using an empty bait construct [Reference Gibson, Yale, Vos, Corfmat, Airikkala-Otter, King, Wallace, Gamble, Handel, Mellanby, Bronsvoort and Mazeri20]. The fixed cost of ORV was one-quarter of CVR, and had a faster daily vaccination rate. ORV also increased the proportion of dogs accessible for vaccination across land use types, such as urban areas and rural villages. Further, staff reported that dogs were more likely to run away from CVR teams and alert nearby dogs by barking, while ORV teams reported that dogs were often attracted to the baits [Reference Gibson, Yale, Vos, Corfmat, Airikkala-Otter, King, Wallace, Gamble, Handel, Mellanby, Bronsvoort and Mazeri20].
Gibson et al. [Reference Gibson, Wallace, Rahman, Bharti, Isloor, Lohr, Gamble, Mellanby, King and Day18] used a spreadsheet tool, originally created by Wallace et al. [Reference Wallace, Undurraga, Gibson, Boone, Pieracci, Gamble and Blanton24], to calculate the costs of user-defined canine vaccination campaigns which included ORV for Indian cities. We show how a simple optimisation routine can instead use this same information to identify the best vaccination strategy for a target vaccination coverage, for dog populations with different proportions of free-roaming animals, and for a range of possible bait costs. This technique can suggest cheaper strategies that may not have been considered by practitioners. For the scenarios examined, we find including ORV almost always improves cost-effectiveness.
Methods
We used linear programming to determine the optimal combination of canine rabies vaccination methods that will minimise costs for a desired level of vaccination coverage, for a range of scenarios.
Structure and size of dog population
Following [Reference Gibson, Wallace, Rahman, Bharti, Isloor, Lohr, Gamble, Mellanby, King and Day18, Reference Wallace, Undurraga, Gibson, Boone, Pieracci, Gamble and Blanton24], we divided the total dog population into three categories: always confined (C), sometimes confined (SC), and never confined (NC). To determine if there were cost-savings of ORV, we compared optimal solutions where four different methods of vaccination were available (CP, DD, CVR, and ORV), or only the three standard methods (CP, DD, and CVR). The number of dogs and the proportion in each category in a region is most uncertain, so we varied the total population size and proportion of NC dogs to examine a range of scenarios. The usefulness of ORV will depend heavily on the number of NC dogs, and because of this, we merely divided the remaining population evenly between C and SC dogs.
Vaccination costs
For each scenario, we used per dog vaccination costs as input to an optimisation routine to identify strategies that minimised the final costs. Per dog vaccination costs were calculated from a selection of mean cost estimates proposed by [Reference Wallace, Undurraga, Gibson, Boone, Pieracci, Gamble and Blanton24] and specified for the city of Bangalore (see Appendix Bang Scen A - OBH - 11d.xlsx from [Reference Gibson, Wallace, Rahman, Bharti, Isloor, Lohr, Gamble, Mellanby, King and Day18]). In some cases, we introduced modifications (e.g., a doubling of the vehicle costs for CVR compared to DD/OVR which might be required with the larger team size). In other cases we estimated quantities that were not provided (see details in Appendix). Since there is considerable uncertainty regarding the exact price of oral baits for use in India [Reference Wallace, Cliquet, Fehlner-Gardiner, Fooks, Sabeta, Setién, Tu, Vuta, Yakobson, Yang, Brückner, Freuling, Knopf, Metlin, Pozzetti, Suseno, Shadomy, Torres, Vigilato, Abela-Ridder and Müller19], we allow this cost to vary from $0.50 to $5.50 in our calculations.
We determined personnel costs by setting the team size required for each method as 1, 2, 2, and 4 people for CP, DD, ORV, and CVR, respectively (see Appendix). We then calculated the per dog costs using vaccination rates of 30 dogs/team/day for CP, DD, and CVR methods from [Reference Gibson, Wallace, Rahman, Bharti, Isloor, Lohr, Gamble, Mellanby, King and Day18], which is within the range reported by other studies for high-density areas [Reference Gibson, Wallace, Rahman, Bharti, Isloor, Lohr, Gamble, Mellanby, King and Day18, Reference Monroe, Ludder, Dilius, Crowdis, Lohr, Cleaton, Gamble, Blanton, Etheart, Pieracci, Natal, Molina-Flores, Millien, Gibson and Wallace25]. We use either the same rate for ORV (Table 1) or a rate 50 of dogs/team/day that was used in [Reference Gibson, Wallace, Rahman, Bharti, Isloor, Lohr, Gamble, Mellanby, King and Day18], and which may reflect the faster handling rate of this method, or the tendency of dogs to cluster around the technicians [Reference Gibson, Yale, Vos, Corfmat, Airikkala-Otter, King, Wallace, Gamble, Handel, Mellanby, Bronsvoort and Mazeri20]. Using these estimates, we obtain a per dog vaccination cost of $1.03, $2.50, $5.36–$6.36, for CP, DD, and CVR methods, respectively, and a range of $1.75–$7.50 for ORV. We then used these costs for the optimisation procedure.
Table 1. Per dog vaccination costs calculated using estimates from [Reference Gibson, Wallace, Rahman, Bharti, Isloor, Lohr, Gamble, Mellanby, King and Day18] with differences noted in the Methods
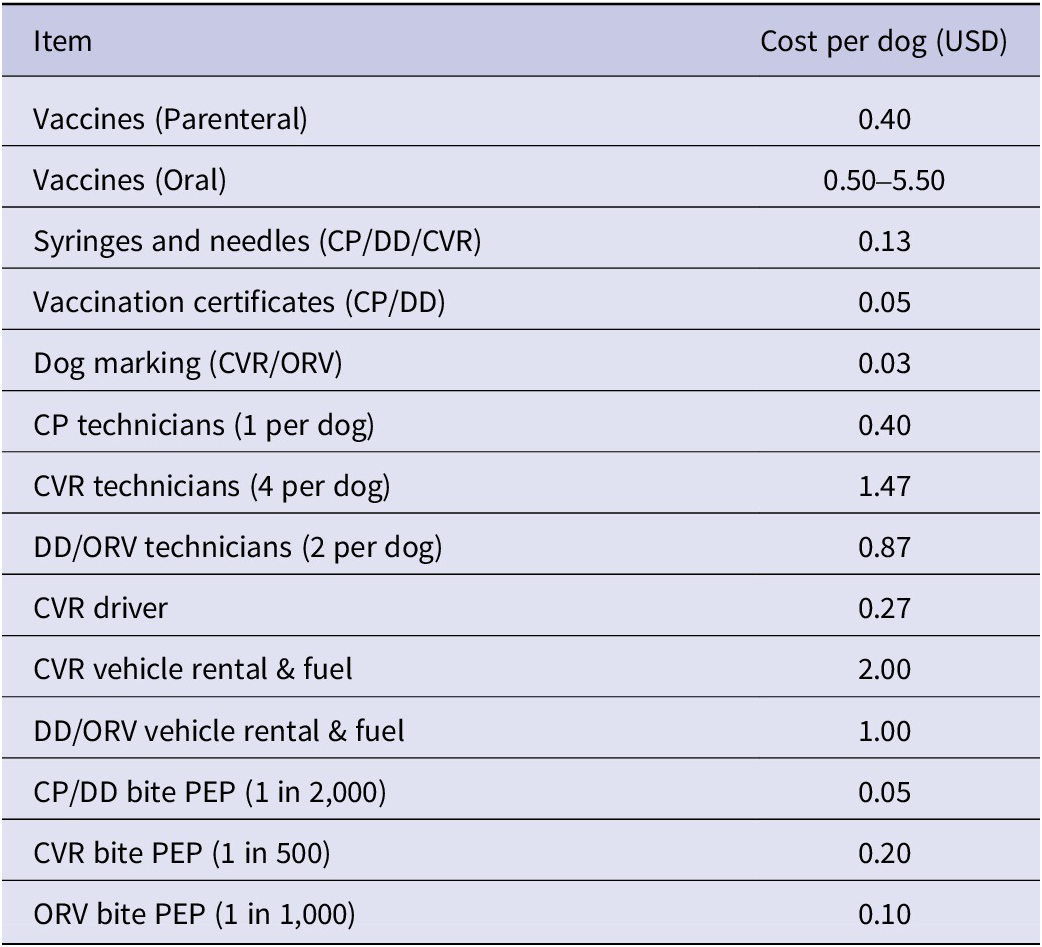
Vaccination coverage
The vaccination coverage achieved by these methods is determined by both vaccine effectiveness and accessibility of dogs to these various methods. Like Gibson et al. [Reference Gibson, Wallace, Rahman, Bharti, Isloor, Lohr, Gamble, Mellanby, King and Day18], we assume parenteral vaccination has the highest effectiveness at 100% chance of rabies immunity, while ORV provides only an 80% chance of immunity. Vaccine accessibility is the probability that a given vaccination method can reach a dog of a given category (see Appendix for more discussion), and was taken from example values in the spreadsheets provided by [Reference Gibson, Wallace, Rahman, Bharti, Isloor, Lohr, Gamble, Mellanby, King and Day18] (see Table 2). However, we also examined the effect of varying accessibility of NC dogs to ORV, and SC dogs to CP.
Table 2. Example of vaccination accessibility from [Reference Gibson, Wallace, Rahman, Bharti, Isloor, Lohr, Gamble, Mellanby, King and Day18]

To get the maximum possible vaccination coverage for a given method, we multiply the probability of being able to use a given vaccination method on each dog confinement category (Table 2) by vaccination effectiveness. For example, ORV can reach 79% of NC dogs (Table 2), but since it has an estimated 80% effectiveness once administered, this method has a maximum coverage of 63%. CVR has an accessibility of 64%, and parenteral vaccination provides a 100% immunity, so the maximum coverage rate remains quite similar at 64%.
Optimisation
To determine optimal vaccine delivery solutions, we use linear programming. This approach has been used for other similar healthcare problems (e.g., [Reference Earnshaw, Richter, Sorensen, Hoerger, Hicks, Engelgau, Thompson, Venkat, Williamson and Gregg26, Reference Diamante, Herrada, Lachica, Oguis and Alviola27]). Our task is an example of a linear transport problem with multiple constraints.
The objective function of a linear programming problem describes the main objective of the decision-maker. In this case, we wish to minimise the per dog costs of vaccination while maintaining a minimum level of vaccination coverage (see below Constraints to achieve rabies vaccination targets). To do this, we need to find the optimal number of dogs to vaccinate using each vaccination method.
Our objective function is as follows:

where cj is the cost for each vaccination method, as described above, and xij is the number of dogs vaccinated in each of the three categories, i, by one of the four methods j. Optimal solutions were found using lpSolve, an R [28] interface to the freely available software lp_solve (version 5.5, https://lpsolve.sourceforge.net/5.5/).
Constraints to achieve rabies vaccination targets
In addition to minimising costs, we added constraints to ensure the selected solution met a minimum vaccination target for disease transmission to be halted or at least slowed. For C and SC dog categories, we use 70% as the minimum annual vaccination coverage required for rabies control [Reference Coleman and Dye12]. We add this constraint as
$ \sum {x}_{ij}{v}_{ij}\ge 0.7{d}_i $
, where xij is the number of dogs in category i vaccinated by method j, di is the number of dogs in each category in the population, and vij is the maximum coverage of method j on dog category i, where this quantity includes both accessibility and vaccine effectiveness (see above Vaccination coverage).
Given the example vaccine effectiveness and accessibility of ORV and CVR provided by [Reference Gibson, Wallace, Rahman, Bharti, Isloor, Lohr, Gamble, Mellanby, King and Day18] for Bangalore, this 70% vaccination target cannot be met for NC dogs (although this coverage has been met in some studies [Reference Gibson, Wallace, Rahman, Bharti, Isloor, Lohr, Gamble, Mellanby, King and Day18]). However, rabies may have relatively low transmission rates, with reproductive number, R0, normally less than 2.5 [Reference Hampson, Dushoff, Cleaveland, Haydon, Kaare, Packer and Dobson29, Reference Rattanavipapong, Thavorncharoensap, Youngkong, Genuino, Anothaisintawee, Chaikledkaew and Meeyai30], such that in some populations lower vaccination coverage may be sufficient to substantially reduce economic and DALY impacts. For example, Fitzpatrick et al. [Reference Fitzpatrick, Shah, Pandey, Bilinski, Kakkar, Clark, Townsend, Abbas and Galvani31] predict an 88% reduction in annual human rabies deaths for an ongoing programme of canine vaccination that reaches ∼ 13% of the overall dog population in Tamil Nadu with an estimated R0 of 1.41. We therefore set the vaccination target for NC dogs at 60%, to fall slightly below the maximum possible coverage for CVR and ORV methods for the example values in [Reference Gibson, Wallace, Rahman, Bharti, Isloor, Lohr, Gamble, Mellanby, King and Day18].
Final costs
We generated optimal solutions (see example in Table 3) for each combination of oral bait price (ranging from $0.5 to $5.50), proportion of NC dogs (ranging from 0.05 to 0.99), over a range of total dog population sizes (5,000 - 150,000), with target vaccination thresholds of 70% for C and SC dogs, and 60% for NC dogs, where this lower value for NC dogs is close to the maximum possible for either ORV or CVR.
Table 3. Optimal vaccination strategy for a population of 50,000 dogs with 48% NC, oral bait cost of $2.50 with other costs as given in Table 1 and vaccination accessibility as given in Table 2

After an optimal vaccination strategy was identified, we then calculated the cost of a 30-day campaign to yield a final per dog cost. Since we assumed fixed costs did not vary significantly for different optimal strategies that used only CP, DD, and CVR, these were not included in the optimisation (see Appendix for a description of fixed costs, including the more general advertising costs). We include one additional cost for campaigns that employ ORV: $10,000 for an information campaign specific to oral baits. Therefore, a strategy which included ORV that was identified by the optimisation as having the lowest per dog vaccination cost could have a higher final per dog cost than other strategies.
Where solutions were possible (see above regarding Constraints to achieve rabies vaccination targets), we then compared the optimal solution obtained when the four different vaccination methods were available to that where only the three standard methods were used, in order to determine if costs were lowered by incorporating ORV.
Results
In general, the final cost per dog was reduced with use of ORV if there was a large number of NC dogs. For example, linear optimisation suggests that when there are 25,000 NC dogs in a total population of 50,000, ORV use will yield lower per dog costs until a fairly high oral bait cost of $3.85 when the parenteral vaccine costs $0.40 (Figure 1(a)). However, lower CVR vehicle costs will push this price threshold lower (Figure 1(b)).

Figure 1. Final per dog costs for vaccination campaigns with and without the use of oral rabies vaccine bait handout (ORV) for a dog population of 50,000, where the number of never-confined dogs (NC) is either 50% (blue lines) or 10% of the population (gold lines), and the catch-vaccinate-release (CVR) vehicle costs are either as given in Table 1 (a) or one-half this value (b). Horizontal dashed lines give the final per dog cost without ORV, while solid lines show how final per dog costs increase with oral bait cost. The intersection of the lines gives the bait cost at which the strategies have the same final per dog costs (indicated by a red dotted line).
We find a similar price threshold across a range of proportions of NC dogs in this fixed population size (Figure 2). Unless the oral bait price is greater than roughly 10x the price of the parenteral vaccine, or the proportion of NC dogs is less than ~0.2, ORV use gives lower costs. With a larger population size, or a higher proportion of NC dogs, this price threshold is even higher (e.g., $4.15 for 150,000 dogs, see Figure 3). However, for very small proportions of NC dogs (e.g., <0.1) there may be no cost advantage unless the total population is quite large.

Figure 2. Difference between final per dog costs for optimal vaccination strategies with and without oral rabies vaccine handout (ORV) for a total dog population of 50,000 with varying proportions of never-confined (NC) dogs and oral bait cost, and other costs as given in Table 1. Negative values indicate the reduction in final per dog cost when using ORV.

Figure 3. Maximum cost per bait at which there would no longer be a cost advantage of using oral rabies vaccine bait handout (ORV) for costs as given in Table 1, accessibility as in Table 2, and a range of total dog population sizes and different proportions of never-confined (NC) dogs. Vertical line shows outcomes for a population size of 50,000 dogs used for other figures.
We categorised the optimal solutions across the range of oral bait price and proportion of NC dogs for an intermediate fixed population size of 50,000 dogs. There are two major categories of solution for NC dogs, but CP vaccination is always suggested for C and SC dogs for the vaccination accessibilities in Table 2. If the oral bait cost is less than the price threshold, the optimal strategy is to always use ORV for NC dogs, and CVR otherwise.
Changes to ORV accessibility will change the optimal solution. Unsurprisingly, optimal solutions are more likely to include ORV for higher accessibility and lower cost (Figure 4). Interestingly, ORV is part of the optimal solution even when accessibility is low (e.g., 0.5) for moderate bait cost ($2.50). For example, as ORV accessibility ranges from 0.0 to 0.99 for a population of 50,000 dogs, we find three different optimal strategies: ORV alone when accessibility is greater than 0.75, a combination of ORV and CVR when accessibility is lower and oral bait cost is less than $3.85, or CVR alone for lower accessibility and higher costs.

Figure 4. Most cost-effective vaccination strategies for never-confined (NC) dogs with the constraint of 60% vaccination coverage. Shaded areas and text indicate whether the optimal strategy is to use oral rabies vaccine bait handout (ORV), catch-vaccinate-release (CVR), or a mixed strategy, as oral bait cost and OVR vaccination accessibility vary. Other costs as given in Table 1, and CVR accessibility is fixed at 0.64. We show the scenario where NC dogs comprise 50% of a total dog population of 50,000.
Similarly, the optimal strategy for other dog categories can vary with changes to vaccination method accessibility. When we allow the CP compliance rate for owners of SC dogs to vary, DD is preferred for low accessibility (Figure 5). We find there are even solutions where use of low-cost ORV may be optimal for this category, when the daily vaccination rate for this method is higher than DD.

Figure 5. Most cost-effective vaccination strategies for sometimes-confined (SC) dogs with the constraint of 70% coverage. Shaded areas and text indicate whether the optimal strategy is to use central point (CP), door-to-door (DD), oral rabies vaccine bait handout (ORV) or mixed strategies as oral bait cost and CP vaccination accessibility vary, where the ORV vaccination rate is faster than DD and CP at 50 dogs/team/day. We show the scenario where SC dogs comprise 50% of a total dog population of 50,000.
Discussion
A renewed commitment to achieving zero human deaths from dog-mediated rabies requires effective vaccination solutions. However, countries like India face the difficult problem of vaccinating large populations of free-roaming dogs. In cities like Bangalore, recent estimates suggest that the free-roaming population may be as large as 300,000 dogs (Worldwide Veterinary Service Centre, as reported by [Reference Sharma32]). Our analysis indicates that for dog populations like these, oral rabies vaccine bait handout (ORV) may minimise costs while still meeting reasonable vaccination coverage targets. Importantly, we find that ORV can offer significant cost-savings even when the baits themselves cost significantly more than parenteral vaccines, and have lower effectiveness.
Like other authors, we find that a mixed-methods vaccination strategy is required to achieve good coverage when there is a large number of free-roaming dogs [Reference Wallace, Undurraga, Gibson, Boone, Pieracci, Gamble and Blanton24]. However, unlike some of the user-defined strategies these authors examined, we find that strategies selected by the optimisation procedure also provide a cost advantage of using ORV. Using cost estimates from this previous work [Reference Wallace, Undurraga, Gibson, Boone, Pieracci, Gamble and Blanton24, Reference Gibson, Wallace, Rahman, Bharti, Isloor, Lohr, Gamble, Mellanby, King and Day18], we show that ORV becomes more cost-effective as the number of never-confined (NC) dogs increases because the only other feasible method for these dogs, catch-vaccinate-release (CVR), usually has higher personnel and equipment costs for the same vaccination coverage. We examined a wide range of per unit prices for oral baits, assuming that initially baits would be imported at high costs, but may have lower costs with future domestic manufacture. In 2020, Wallace et al. [Reference Wallace, Cliquet, Fehlner-Gardiner, Fooks, Sabeta, Setién, Tu, Vuta, Yakobson, Yang, Brückner, Freuling, Knopf, Metlin, Pozzetti, Suseno, Shadomy, Torres, Vigilato, Abela-Ridder and Müller19] suggested a price range of $2.00–$4.00 USD, while Gibson et al. [Reference Gibson, Wallace, Rahman, Bharti, Isloor, Lohr, Gamble, Mellanby, King and Day18] examined a range of $1.50–$2.50. We find that for some scenarios, even bait prices almost as high as 10x that of parenteral vaccines (~$3.85) can offer a cost-savings.
We note that all cost estimates included here are examples only, do not include fixed costs except for an ORV information campaign, and will likely vary widely from location to location. However, we expect the general trend of solutions to hold as long as the ratio of various costs remains similar. For example, reducing the vehicle costs of CVR by 50% still suggests that there is a bait price threshold below which ORV will be the best solution. However, if a particular cost category changes significantly relative to others, optimal strategies may change such that ORV is no longer part of the solution.
When it is suspected that the number of NC dogs is small (e.g., <10% of population of 50,000 dogs), it is less clear if ORV should be employed. Unfortunately, dog population estimates are usually poor. There is often little survey data, and estimates are frequently created by using a fixed proportion of the human population. Photo mark-recapture data collection seems quite promising (e.g., [Reference Tiwari, Robertson, O’Dea and Vanak33]) and could be used before the design of a vaccination campaign to estimate the size of free-roaming populations more accurately. Such methods may reveal that different areas in the same urban community have quite different population structures [Reference Bhalla, Kemmers, Vasques and Vanak34], so that more effective methods can be targeted for specific areas, again using optimisation (e.g., [Reference Diamante, Herrada, Lachica, Oguis and Alviola27]). However, for large numbers of NC dogs, it seems clear that ORV will usually be the best option.
Optimal solutions were determined in part by the accessibility of dogs to different methods because of the requirement to meet vaccination coverage targets. Accessibility estimates will also vary widely with location. While we found solutions that included an increased use of CVR as ORV accessibility decreased, we strongly suspect that low accessibility to ORV methods is correlated with low accessibility to CVR methods, except perhaps in the case of low bait palatability. We also note that it will be quite difficult to meet 70% vaccination targets for NC dogs with either of these methods, although the recent successes in Goa are inspiring [Reference Gibson, Yale, Corfmat, Appupillai, Gigante, Lopes, Betodkar, Costa, Fernandes, Mathapati and PM35]. Therefore, we suggest the efficacy of lower targets for reducing the human burden of disease should be further investigated.
Other vaccination accessibility changes can lead to optimal strategies that use ORV for different dog categories. In rural India, Tiwari [Reference Tiwari17] suggests that dogs are mostly ‘partially’ owned, meaning that a household may claim ownership but not consider themselves responsible for the animal’s vaccination and veterinary care, and it is possible there are similar patterns in urban centres. In Bangalore specifically, there is a higher density of free-roaming dogs in areas with a higher human density and lower average income. Households in this area are also more likely to feed free-roaming dogs [Reference Bhalla, Kemmers, Vasques and Vanak34]. This scenario both increases risks of rabies transmission and potentially makes it less likely that household-associated dogs will be transported to a central location for vaccination, or even encountered during door-to-door vaccination efforts. Owner-driven vaccination programmes used elsewhere (e.g., [Reference Vigilato, Cosivi, Knöbl, Clavijo and Silva16]) may not be as effective in these regions. For low CP compliance rates, or low DD probability, optimal solutions can employ low-cost ORV for these dogs.
Finally, methods not considered here may offer better cost-savings. For example, mobile CP methods, where vaccination centres on vehicles move through neighbourhoods, combined with DD, maybe a better option when ORV costs are high. For fractious dogs, or staff that have not been trained in injection, oral bait handout with a door-to-door access method is another option that may be a reasonable choice, and has been demonstrated to be highly effective [Reference Freuling, Busch, Shikongo, Silume, van der Westhuizen, Khaiseb, Shilongo and Müller36]. In the future, it may even be possible to combine oral contraceptives with the oral rabies vaccine bait, to simultaneously reduce population turnover.
In conclusion, pilot projects and analyses have previously suggested that oral baits will make a valuable contribution to India’s campaign to eliminate rabies [Reference Gibson, Yale, Vos, Corfmat, Airikkala-Otter, King, Wallace, Gamble, Handel, Mellanby, Bronsvoort and Mazeri20, Reference Gibson, Wallace, Rahman, Bharti, Isloor, Lohr, Gamble, Mellanby, King and Day18]. We use optimisation to demonstrate that even if oral baits are considerably more expensive and less effective than parenteral vaccinates, they may still reduce costs in most scenarios involving free-roaming dogs while providing very good vaccination coverage. We expect that charitable efforts aimed at providing low-cost ORV may be more cost-effective in reducing the human burden of disease than additional efforts directed at PEP.
Data availability statement
All code used to generate our results can be found on GitHub (see https://github.com/kcudding/rabies). In addition, the code requires spreadsheets in the supplementary material from [Reference Gibson, Wallace, Rahman, Bharti, Isloor, Lohr, Gamble, Mellanby, King and Day18] (http://www.mdpi.com/2414-6366/5/1/47/s1).
Author contribution
Conceptualization: K.C., W.H.B.M.; Project administration: W.H.B.M.; Writing – review & editing: K.C., W.H.B.M.; Formal analysis: K.C.; Investigation: K.C.; Methodology: K.C.; Software: K.C.; Visualization: K.C.; Writing – original draft: K.C.
Financial support
This study was funded by Rethink Priorities.
Competing interest
The authors declare none.
Appendix 1: Detailed methods for Cuddington and McAuliffe, Optimising rabies vaccination of dogs in India
Model formulation
We use linear optimisation on per dog vaccination costs to identify cost-minimising strategies with the constraint that the solution maintains the desired vaccination coverage, for a range of scenarios (see main text).
Detailed vaccination costs
Some costs for consumables in Gibson et al. [Reference Gibson, Wallace, Rahman, Bharti, Isloor and Lohr37] were already provided on a per dog cost (e.g., vaccines, syringes, certificates and dog marking). Costs that were not expressed per dog included vehicle rentals, equipment and staff wages that would scale with a given number of vaccinations (e.g., the costs of CVR include among other things, the cost of renting a van, the budgeted expense of treating employees that received a bite, and the salary of a driver).
We did not include fixed costs that we would expect for every vaccination campaign in the optimisation procedure. For example, we assumed that every campaign would have a fixed central location, such as an animal hospital, with refrigerated vaccine storage. Similarly, we assume that any vaccination campaign would include advertising and information efforts, and again, accounted for these as part of a fixed cost. CP vaccination would occur at this location, with animal technicians floating between injection practitioners, to be used as needed, as they do in a veterinary office. These technicians would therefore not be dedicated to a given team, and so were considered part of the fixed costs. However, it is certainly possible to instead assume a team size of two for CP vaccination: one vaccine injector and one animal handler. With this assumption the additional salary would be part of the variable costs, and would result in higher per dog costs for this method.
We calculated the per dog cost of personnel using estimated daily wages, team size and vaccination rates (see main text). Naturally these costs may vary wildly with location, but we confirmed that the rates used by Gibson et al. [Reference Gibson, Wallace, Rahman, Bharti, Isloor and Lohr37] (i.e. $12, $13, $11, $13 daily wage for technicians using CP, DD, CVR and OVR vaccination respectively, and $8 for the driver) were reasonable (about double the current minimum wage for skilled workers in the state of Karnataka [38]).
The per dog rate of other expenses such as CP/DD Bite PEP can be calculated from the rates provided (1 in 2,000) [Reference Gibson, Wallace, Rahman, Bharti, Isloor and Lohr37]. A separate rate for ORV was not provided, but we assumed the same cost as CP/DD PEP with a higher rate (1 in 1,000), but a lower rate than CVR PEP (1 in 500). We assumed that vaccination certificates would only be provided for CP and DD dogs, and dog marking (e.g., paint or similar [Reference Totton, Wandeler, Zinsstag, Bauch, Ribble and Rosatte39]) would be completed only for CVR and ORV dogs (see Table 1).
While DD and ORV vaccination requires a team of two and minimal equipment that can be transported on a small vehicle like a moped, CVR requires a lot of equipment and a vehicle that can transport 4–5 people. Therefore, unlike Gibson et al. [Reference Gibson, Wallace, Rahman, Bharti, Isloor and Lohr37], we differentiate the vehicle rental costs and assume that the larger vehicle for CVR will cost two times more ($30 USD/day) than a vehicle required for DD and OVR ($15/day), and will require twice the amount of gasoline. We believe this is an underestimate of required vehicle costs, since our informal online search of rental agencies suggests moped rentals will range from $3 to $7 USD per day, passenger cars $25–85 and larger minivan ~$100. Therefore, while not included in this analysis, if mopeds could indeed be used for DD and ORV, and a larger vehicle such as a minivan was needed for CVR, the costs would differ by a factor of 10. Note that when the faster vaccination rate of 50 dogs/team/day for ORV is used, the per dog cost of personnel and vehicle rental for DD and ORV methods will differ from Table 1.
Wallace et al. [Reference Wallace, Undurraga, Gibson, Boone, Pieracci and Gamble40] notes that the WHO suggests the use of oral baits requires information campaigns, as well as surveillance systems capable of detecting unintended vaccine exposures, which could add additional unknown costs to such a campaign. This recommendation for surveillance seems to relate more to the case where oral baits are broadcast in the environment rather than handed out to individual dogs. In addition, recent developments in these vaccines make adverse effects on accidental exposure very unlikely [Reference Yale, Lopes, Isloor, Head, Mazeri and Gamble41]. Nonetheless, we included one additional fixed cost for vaccination campaigns that included oral baits: $10,000 for dissemination regarding the deployment of oral baits. This value was based on estimates from Gibson et al. [Reference Gibson, Wallace, Rahman, Bharti, Isloor and Lohr37] for a more general advertising campaign, and included costs for 10,000 pieces of printed information and 30 days of radio or car loudspeaker announcements.
There are no differences in costs noted by these authors with respect to using the different methods for different classes of dogs. Thus, the cost of central point vaccination (CP) for a never-confined dog (NC), is given as the same for an always-confined © dog. The difference in using these methods for different categories is accounted for in an accessibility metric (see below Detailed vaccination method coverage).
Detailed vaccination method coverage
We use the vaccine accessibility values provided by Gibson et al. [Reference Gibson, Wallace, Rahman, Bharti, Isloor and Lohr37] for the city of Bangalore (Table 2), but (1) the vaccine accessibility values will depend on the particular location, and (2) their meaning lies in the exact method of implementation. For example, Gibson et al. [Reference Gibson, Wallace, Rahman, Bharti, Isloor and Lohr37] indicate that CP and DD campaigns will have a higher probability of reaching C and SC dogs, and estimate a 5% chance of vaccinating an always confined (C) dog with an oral bait handout. However, it is of course entirely possible to have a door-to-door campaign that employs oral baits instead of injections, and it may be cheaper and more effective to do so if getting trained personnel to administer injections is too costly, or the probability of owners responding to calls to attend CP vaccination locations is too low. We allow NC ORV and SC CP vaccine accessibility to vary in our analysis to address the first point, but have not included more hybrid strategies such as the use of oral baits in a door-to-door campaign.
In the main text we note that it is not possible to meet a 70% coverage target with the values provided by [Reference Gibson, Wallace, Rahman, Bharti, Isloor and Lohr37], and so set the coverage target for NC dogs at 60%. Another option is to set a 70% vaccination target for the combined transmission category of free-roaming dogs which includes both NC and SC dogs, as well as 70% of C dogs. Solutions that meet this constraint can only be found where NC dogs comprise <50% of the population, if only one vaccination attempt is made per dog, and the remaining dog population is divided evenly between SC and C dogs.
Optimisation with probabilistic constraints
We also investigated solutions where the probability of reaching the specified vaccination constraints was met with either a 20% probability or a 70% probability, on the grounds that lower probabilities of meeting vaccination targets may be sufficient for significant positive impact as suggested by Fitzpatrick et al. [Reference Fitzpatrick, Shah, Pandey, Bilinski, Kakkar and Clark42].
To incorporate a probabilistic approach to meeting vaccination targets, we use a chance constraint optimisation procedure [e.g., Reference Geletu, Klöppel, Zhang and Li43]. We impose the constraint that for each dog category, i,

where ζi is the number of vaccinated dogs required to achieve the desired vaccination coverage for that category (e.g., 0.7di), and p is a given probability.
Then, we can relax the probabilistic problem into the equivalent deterministic problem by using the appropriate probability density function and substituting the left-hand side of the constraint with a deterministic expression. If we assume that ζi is normally distributed with mean μi and variance σi 2, we can transform the constraint for each i into

where qp is the p-quantile of the standard normal distribution. We then assume that the dog population estimates were normally distributed with means as previously indicated and variance set as 20% of the mean. After this, we then solve as usual for a given p. However, we note that issues with convexity and stability can mean that small changes in the actual density function could cause major changes in the optimal solution.
However, introducing probabilistic constraints on meeting the vaccination targets did not introduce any differences in the optimal strategies. As expected, achieving a higher probability of success requires vaccinating more dogs. For instance, in a population of 50,000, we will need to vaccinate ~10% more dogs (5500–6400) to have a 70% probability of meeting our coverage targets, versus having only a 20% probability of doing so.