Implications
In vitro production of embryos and other assisted reproductive techniques in cattle have shown significant progress in recent years. The combination of in vitro production with sexed semen and genomic selection is being successfully and widely used in North America, South America and Europe. Importantly, in 2016 the number of viable in vitro production embryos surpassed the number of transferable in vivo–produced embryos (multiple ovulation embryo transfer, MOET). This trend shows a shift among seedstock producers from traditional MOET toward IVP. These assisted reproductive techniques have been proven successful in the commercial field, assisting practitioners and cattle producers to improve reproductive performance, efficiency and genetic gain.
Introduction
The scientific and technological advances achieved during the past decades in animal reproduction have resultedin the development of a variety of tools commonly referred to as assisted reproductive technologies (ART). The primary focus of these tools is to maximize the number of offspring from genetically superior animals and disseminate germplasm worldwide (Berglund, Reference Berglund2008). Furthermore, ART allows for the exploitation of donors with compensable anatomical disabilities and sub-fertile conditions, for safeguarding germplasm from threatened species and domestic breeds and for reducing disease exposure and transmission.
While the number of in vivo–produced embryos that are collected and transferred worldwide seems to have stabilized in recent years, the transfer of embryos derived and transferred from in vitro production (IVP) continues to grow at an average annual growth rate of 12% (Figure 1a). In 2016, and for the first time in recorded history, the number of viable IVP embryos surpassed the number of transferable in vivo–produced embryos, based on data recorded by the International Embryo Transfer Society (IETS). However, this historic event is only based on the declared data submitted to IETS by volunteer participants, and as such it likely does not include the overall number (i.e., declared plus undeclared embryo production) of IVP and in vivo–produced embryos worldwide; therefore, it is within the realm of possibility that the actual event could have occurred even earlier. These trends show a shift among seedstock producers from traditional MOET and toward IVP (Figure 1a). Interestingly, this aforementioned shift is combined with a tendency toward increased efficiency of the IVP procedures (Figure 1b).

Figure 1 Evolution of Bos indicus and Bos taurus in vivo–produced embryos (MOET) and in vitro–produced (IVP) embryos worldwide (a) and embryo yield per collection/ovum pick-up (OPU) (b) as registered by the International Embryo Transfer Society (IETS, http://www.iets.org/comm_data.asp).
According to the annual embryo production statistics presented by the IETS in recent years, more than 400 000 bovine IVP embryos were transferred worldwide (Figure 1a). Of the total embryos transferred, around 80% of them were transferred fresh (Figure 2). This reflects the fact that embryos produced in vitro have lower cryotolerance than their in vivo counterparts. In the late 1990s, the quantity of frozen-thawed embryos (both in vivo and IVP) transferred was practically similar to fresh (non-cryopreserved) embryos. Subsequently, the ratio of fresh IVP embryos transferred increased, but in recent years (2014 to 2016) there had been an increase in the number of transfers of frozen-thawed IVP embryos, possibly due to the use of advanced formulated culture media without (or with low) serum content and/or with specific embryotrophic additives promoting embryo quality. Another potential reason behind the increase in frozen-thawed IVP embryo transfers may be due to the improved efficiency linked to the use of co-culture in IVP.

Figure 2 Percentage of fresh Bos indicus and Bos taurus in vivo–produced embryos (MOET) and in vitro–produced (IVP) embryos transferred worldwide registered by the IETS (http://www.iets.org/comm_data.asp).
Towards the end of the 1980s, the combination of the ultrasound-guided oocyte retrieval technique (Pieterse et al., Reference Pieterse, Kappen, Kruip and Taverne1988), usually referred to as ultrasound-guided ovum pickup (OPU) (Ward et al., Reference Ward, Lonergan, Enright and Boland2000), and IVP became available. Several European artificial insemination centers implemented OPU-IVP as the primary tool to multiply genetically high merit animals. In the USA the OPU-IVP technology combination was adopted on a commercial level in the late 1990s. By then, it was primarily used to produce embryos from ‘problem’ females that for different reasons could not generate viable embryos by conventional MOET. Under these circumstances, the commercial development of the technology was slow, whereas more recently, and fueled by the introduction of sexed semen (SS) and genomic selection (GS) of embryos (Johnson, Reference Johnson1995; Welch and Johnson, Reference Welch and Johnson1999), the commercial application of IVP has greatly increased (Sirard, Reference Sirard2018).
Recent IVP results (Gonzalez-Marin et al., Reference Gonzalez-Marin, Lenz, Gilligan, Evans, Gongora, Moreno and Vishwanath2017) and the fact that SS from more elite bulls is being made available both contribute to IVPs’ continued gain in the cattle market. A major factor that stands out for the increase in IVP can be attributed to the ‘reverse sorting’ technology, which consists of thawing a few straws of conventional non-sexed semen, then sex sorting the sperm using flow cytometry, followed by using the sexed sperm for IVP immediately thereafter (Morotti et al., Reference Morotti, Sanches, Pontes, Basso, Siqueira, Lisboa and Seneda2014). This method, perfected by Trans Ova Genetics, allowed for sex sorting almost any stored, cryopreserved bovine semen available.
New genetic tools led to improved animal selection by GS and allowed accelerated genetic gain and progress (Goddard et al., Reference Goddard, Hayes and Meuwissen2010). The combination of ART (MOET, IVP) and GS maximizes genetic gain (Ponsart et al., Reference Ponsart, Le Bourhis, Knijn, Fritz, Guyader-Joly, Otter, Lacaze, Charreaux, Schibler, Dupassieux and Mullaart2013) in cattle (Figure 3). In addition, the conjunction of biopsies obtained from non-implanted embryos or amniocentesis with GS in younger heifers has increased the genetic selection pressure even further (Kasinathan et al., Reference Kasinathan, Wei, Xiang, Molina, Metzger, Broek, Kasinathan, Faber and Allan2015). Although some limitations of these approaches have been found (e.g., extra cost and ethical considerations (Kasinathan et al., Reference Kasinathan, Wei, Xiang, Molina, Metzger, Broek, Kasinathan, Faber and Allan2015)), a recent study indicated that embryo biopsy does not affect the viability and pregnancy rate of IVP-derived embryo (de Sousa et al., Reference de Sousa, da Silva Cardoso, Butzke, Dode, Rumpf and Franco2017).
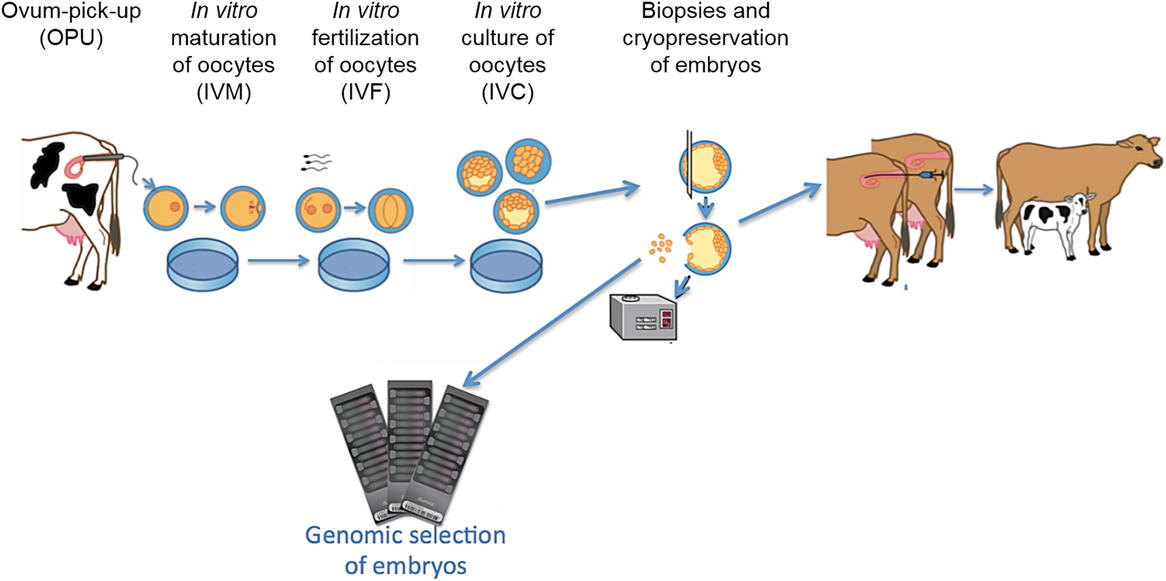
Figure 3 Flow of the process for Bos indicus and Bos taurus ovum pickup (OPU), in vitro maturation (IVM) of oocytes, in vitro fertilization (IVF), in vitro culture (IVC) of embryos, collection of biopsies, genomic selection using single nucleotide polymorphism (SNP) markers and cryopreservation.
Embryos can be derived by IVP from open-cycling heifers and cows as well as females that do not respond properly to superovulatory treatments, have abnormalities in their reproductive tract compromising gamete transport, or are in terminal conditions (age, health, accident). Likewise, pregnant animals during the first trimester of pregnancy, postpartum cows (lactation) and from pre-pubertal calves can be employed for IVP, as well as ovaries collected at abattoirs (Galli et al., Reference Galli, Duchi, Crotti, Turini, Ponderato, Colleoni, Lagutina and Lazzari2003).
The first calves obtained exclusively by IVP, that is, in vitro maturation (IVM) of oocytes, in vitro fertilization (IVF) as well as in vitro embryo development (IVD), were reported in the late 1980s (Goto et al., Reference Goto, Kajihara, Kosaka, Koba, Nakanishi and Ogawa1988). Significant progress has been made in understanding gamete/embryo requirements and metabolism allowing for formulation of better culture media to achieve a higher total production and embryo quality (Hansen, Reference Hansen2006) and a reduction in the occurrence of the large offspring syndrome (LOS) (Farin et al., Reference Farin, Crosier and Farin2001). Major advances in IVP today seek to improve overall performance at all stages: ovarian stimulation, oocyte recovery, maturation, fertilization, embryo development, freezing, transfer and pregnancy establishment.
Ovarian treatment and oocyte recovery
Oocytes for IVP can be obtained by (1) ultrasound-guided follicular aspiration from live animals or by ovariectomy or (2) postmortem follicular aspiration (abattoir-derived ovaries). In both scenarios, oocytes are aspirated from a heterogeneous pool of antral follicles, 2 to 8 mm in size, including follicles from both non-ovulatory and ovulatory follicular waves as well as dominant and subordinate follicles in these waves. Oocytes in the large dominant follicles will be in the process of the so-called pre-maturation or capacitation, building up their competence for final maturation, for fertilization and for sustaining embryonic development (Hyttel et al., Reference Hyttel, Fair, Callesen and Greve1997).
In the case of follicle aspiration (Bols et al., Reference Bols, Leroy and Viana2005) it is possible to obtain four to five usable (grades 1 and 2; (de Loos et al., Reference de Loos, van Vliet, van Maurik and Kruip1989)) oocytes per donor session (Hasler, Reference Hasler1998) from Bos taurus unstimulated females, while stimulation by FSH can increase oocyte recovery rates to 20 per Holstein donor session (Vieira et al., Reference Vieira, Rodrigues, Castro Netto, Guerreiro, Silveira, Freitas, Bragança, Marques, Sá Filho, Bó, Mapletoft and Baruselli2016). Donors (open, pregnant, pre-pubertal) can be subjected to follicular aspiration with a frequency of one to two times a week, with or without stimulation (Chaubal et al., Reference Chaubal, Ferre, Molina, Faber, Bols, Rezamand, Tian and Yang2007). During intensive OPU schemes (e.g., twice a week) special care must be taken into consideration, such as animal welfare, repetitive epidural anesthesia, ovary stroma integrity and adhesions (McEvoy et al., Reference McEvoy, Alink, Moreira, Watt and Powell2006). Consequently, more embryos can be produced from stimulated donors (Chaubal et al.,Reference Chaubal, Ferre, Molina, Faber, Bols, Rezamand, Tian and Yang2007). Only limited efforts have been devoted to enhancing the current synchronization and stimulation protocols for OPU donors.
In Bos indicus cattle, the use of FSH prior to OPU remains controversial. Some studies have shown an increase in the number of total oocytes recovered, viable oocytes, embryos produced and conception rates after transfer (Fernandes et al., Reference Fernandes, Miyauchi, Figueiredo, Palhão, Varago, Nogueira, Neves and Miyauchi2014), while others argue that FSH stimulation reduces cleavage, blastocyst and hatching rates (Monteiro et al., Reference Monteiro, Ferreira, Potiens, Eberhardt, Trinca and Barros2010). Importantly, there are cases reported for Bos indicus in which FSH stimulation prior to OPU also has resulted in positive effects on IVP and the resulting pregnancy rates (Cavalieri et al., Reference Cavalieri, Morotti, Seneda, Colombo, Andreazzi, Emanuelli and Rigolon2017). It should be noted though that generally in the field (on-farm situations), practitioners do not synchronize and stimulate Bos indicus donors due to cost-effectiveness outcome, as Bos indicus generally produces more oocytes per aspiration without synchronization nor stimulation compared to Bos taurus, 15.1 ± 1.9 v. 7.8 ± 0.8, respectively (Mean ± SD) (Fernandes et al., Reference Fernandes, Miyauchi, Figueiredo, Palhão, Varago, Nogueira, Neves and Miyauchi2014). Even though a significant difference was found in the control v. the synchronized groups for both Bos indicus and Bos taurus, FSH stimulation was only significantly effective in Bos taurus donors (Fernandes et al., Reference Fernandes, Miyauchi, Figueiredo, Palhão, Varago, Nogueira, Neves and Miyauchi2014), highlighting the need to consider cost/biological success as a decisive factor in determining which method is used.
To modify the oocyte quality within the follicle it is necessary to manipulate follicular dynamics and function through hormonal treatments (Merton et al., Reference Merton, de Roos, Mullaart, de Ruigh, Kaal, Vos and Dieleman2003) and/or dietary regimes (Webb et al., Reference Webb, Garnsworthy, Gong and Armstrong2004). Several approaches have been suggested to improve the number of follicles and oocyte quality (measured as the rate of viable embryos) in OPU programs. The strategies include: (1) donor stimulation with gonadotropins (Sendag et al., Reference Sendag, Cetin, Alan, Hadeler and Niemann2008), (2) partial in vivo pre-maturation, termed ‘coasting’ (Nivet et al., Reference Nivet, Bunel, Labrecque, Belanger, Vigneault, Blondin and Sirard2012) and (3) dietary supplementation with energy concentrates rich in fatty acids (Dunning et al., Reference Dunning, Russell and Robker2014).
Notably, ultrasound screen resolution and probe characteristics have a great impact on oocyte recovery efficiency (Bols et al., Reference Bols, Leroy, Vanholder and Van Soom2004) and, perhaps more importantly, in reducing the risk of causing unnecessary damage to the ovarian tissue. Newer ultrasound machines show small diameter follicles (2 to 3 mm) which may not be ideal for retrieving oocytes, but still suitable for selecting donors based on the ovarian antral follicle count (Silva-Santos et al., Reference Silva-Santos, Santos, Koetz Junior, Morotti, Siloto, Marcantonio, Urbano, Oliveira, Lima and Seneda2014). Cumulus oocyte complex (COC) retrieval is highly correlated with embryo production and the number of pregnancies (Watanabe et al., Reference Watanabe, Souza, Mingoti, Ferreira, Oliveira Santana Batista, Dayan, Watanabe, Meirelles, Nogueira, Ferraz and Baruselli2017), but not with pregnancy rate (Feres et al., Reference Feres, Siqueira, Palhao, Dos Santos, Brandao and Viana2018). Newer Doppler ultrasonography features allow follicular blood flow evaluation, which can be used to prevent blood contamination in the collection tube. Laparoscopic ovum pickup (LOPU) has recently gained interest among commercial IVP companies as a viable tool to recover COCs from calves at 2 to 6-months of age (Baldassarre et al., Reference Baldassarre, Currin, Michalovic, Bellefleur, Gutierrez, Mondadori, Glanzner, Schuermann, Bohrer, Dicks, Lopez, Grand, Vigneault, Blondin, Gourdon and Bordignon2018). This technique, mainly used in small ruminants (Cognié et al., Reference Cognié, Baril, Poulin and Mermillod2003), could contribute to accelerate dissemination of superior genetics, further encouraging the genetic improvement through top genomic young animals.
Oocyte maturation
Oocyte maturation includes nuclear as well as cytoplasmic changes in the developing gamete. Nuclear oocyte maturation refers to the progression of meiosis from the prophase of the first meiotic division to the metaphase of the second meiotic division (MII) at the time of ovulation. Along with nuclear maturation a series of changes in organelles, proteins and transcripts take place in the oocyte constituting the cytoplasmic oocyte maturation (Hyttel et al., Reference Hyttel, Fair, Callesen and Greve1997). Meiosis arrests at MII until fertilization, when it resumes and completes the segregation of the second polar body (Sirard, Reference Sirard2001).
Under in vitro conditions, oocytes will normally complete nuclear maturation to MII within 20 to 24 h and then will be ready for fertilization (Leibfried-Rutledge et al., Reference Leibfried-Rutledge, Critser, Eyestone, Northey and First1987). Along with the nuclear maturation, the oocytes complete a cytoplasmic maturation that mimics the cytoplasmic maturation seen under in vivo conditions although the aberrancies in the precise migration of the cortical granules of significance for preventing polyspermic fertilization have been noted in vitro (Hyttel et al., Reference Hyttel, Xu, Smith and Greve1986). The heterogeneous source of the aspirated immature oocytes retrieved from ovarian follicles at different phases of the follicular growth can result in compromised developmental competence due to improper completion of the cytoplasmic oocyte maturation (Mermillod et al., Reference Mermillod, Oussaid and Cognie1999). Around 85% to 90% of the cultured immature oocytes will reach MII at the end of IVM under proper conditions. Although this success rate may be interpreted as satisfactory, oocyte cytoplasmic maturation and full acquisition of developmental competence are in many cases not automatically accompanying the nuclear maturation and may account for fertilization and/or development deficiencies (Watson, Reference Watson2007). In this regard, oocytes matured in vivo yield more blastocysts compared to their IVM counterparts (Sirard and Blondin, Reference Sirard and Blondin1996). Oocyte quality/competence represents a major factor in determining blastocyst development rates (Krisher, Reference Krisher2004). Improving the direct assessment of nuclear and cytoplasmic maturation by non-invasive methods could allow for future selection of the competent oocytes (Fulka et al., Reference Fulka, First and Moor1998).
The major research line in this field is the concept of in vitro prematuration, maintaining the oocytes at the germinal vesicle (GV) stage in culture to allow them to end their differentiation before meiotic resumption. Indeed, meiotic resumption induces chromosome condensation and, in turn, arrest of transcription that may block the synthesis of maternal messenger RNA (mRNA) that will be necessary for embryo development before embryonic genome activation. It is possible to block meiotic resumption of cattle oocytes by specific inhibitors of the M-Phase Promoting factor (MPF), involved in GVBD, chromosome condensation and spindle formation; however, inhibiting meiosis for 24 to 48 h did not increase oocyte competence (Mermillod et al., Reference Mermillod, Tomanek, Marchal and Meijer2000). More recently, the concept of stimulated physiological oocyte maturation (SPOM) emerged as a promising approach to improve IVM (Albuz et al., Reference Albuz, Sasseville, Lane, Armstrong, Thompson and Gilchrist2010). This method combines the meiotic inhibition for 12 h by drugs increasing the oocyte cAMP level and stimulation of maturation by FSH, resulting in slower maturation (6 h longer) and increased development rate and quality after IVF. Even if this methodology is still controversial (Guimarães et al., Reference Guimarães, Pereira, Leme and Dode2015), it represents a promising direction for further research.
Inside the follicle, oocyte and surrounding somatic cells are in constant interaction through gap junction and factors transmitted by the follicular fluid (FF). These interactions are determining factors for the success of oocyte differentiation, leading to developmental competence. Many compounds found in FF, including growth factors produced by granulosa cells or by the oocyte itself, have been described (Rodgers and Irving-Rodgers, Reference Rodgers and Irving-Rodgers2010). More recently, extracellular vesicles (EVs), including exosomes, have been described in the FF (Di Pietro, Reference Di Pietro2016). These EVs originate from somatic cells and the oocyte and contain a diversified cargo, including proteins, mRNA, microRNA and lipids (Santonocito et al., Reference Santonocito, Vento, Guglielmino, Battaglia, Wahlgren, Ragusa, Barbagallo, Borzi, Rizzari, Maugeri, Scollo, Tatone, Valadi, Purrello and Di Pietro2014). These exosomes are involved in oxidative attack protection (Saeed-Zidane et al., Reference Saeed-Zidane, Linden, Salilew-Wondim, Held, Neuhoff, Tholen, Hoelker, Schellander and Tesfaye2017), miRNA signaling, inducing transcription modulation in early embryos (Gross et al., Reference Gross, Kropp and Khatib2017) and regulating cumulus expansion (Hung et al., Reference Hung, Hong, Christenson and McGinnis2015). Altogether, a further study of FF exosomes may allow for the improvement of IVP results (da Silveira et al., Reference da Silveira, Andrade, Del Collado, Sampaio, Sangalli, Silva, Pinaffi, Jardim, Cesar, Nogueira, Cesar, Coutinho, Pereira, Perecin and Meirelles2017) and help to define markers of oocyte quality.
Fertilization
After completion of IVM, oocytes are co-incubated with spermatozoa for up to 18 to 24 h while undergoing IVF. The sperm–oocyte interaction in the fertilization dish occurs in a microenvironment of 50 to 100 µl for low numbers of OPU donor oocytes, and usually a microenvironment of >400 µl for abattoir mass-collected oocytes (Gordon, Reference Gordon and Gordon2003). Usually the spermatozoa are washed and selected using swim-up or density gradient centrifugation procedures to remove freezing media, seminal plasma, debris and dead spermatozoa and to select the more motile fraction. The spermatozoa must also be treated with capacitating factors in order to acquire the ability to penetrate the zona pellucida (ZP) of the oocyte (Parrish et al., Reference Parrish, Susko-Parrish, Leibfried-Rutledge, Critser, Eyestone and First1986). The minimum number of spermatozoa required per oocyte is not well defined due to a large variation between bulls and breeds although generally a concentration of 1 to 2 million spermatozoa ml−1 is commonly used for IVF (Ward et al., Reference Ward, Enright, Rizos, Boland and Lonergan2002). Therefore, large numbers of oocytes can be fertilized using conventional frozen semen as compared with the traditional 2.1 million sexed spermatozoa per straw. Fertilization efficiency can be optimized, even at lower sperm concentrations of <0.5 × 106/ml, by selecting a high fertility bull or multiple bulls (pooled; Lu and Polge, Reference Lu and Polge1992) and/or adjusting capacitating factor concentration, drop size and oocyte density (Van Soom et al., Reference Van Soom, Van Vlaenderen, Mahmoudzadeh and de Kruif1991). In some cases, such as commercial IVP programs, sire selection by lab personnel is not possible or at least restricted by customer choice. Despite this reality, a main factor to consider is the motile sperm : oocyte ratio, which can be as low as 600 : 1 (An et al., Reference An, Chaubal, Liu, Chen, Nedambale, Xu, Xue, Moreno, Tao, Presicce and Du2017) to 5000 : 1 (Tanghe et al., Reference Tanghe, Van Soom, Talebkhan Garoussi, Mintiens and de Kruif2000). In the case of sexed sperm the sperm : oocyte ratio should be a little higher ranging from at least 600 to 1200 : 1 (Xu et al., Reference Xu, Guo, Su, Nedambale, Zhang, Schenk, Moreno, Dinnyes, Ji, Tian, Yang and Du2006) to 1500 to 2250 : 1 (Lu and Seidel, Reference Lu and Seidel2004). Unfortunately, the aforementioned relevant information is not clearly detailed in scientific journal articles.
Fertilization rate, measured as the cleavage rate at 48 h post-insemination (and assuming parthenogenesis is not taking place), usually ranges between 70% and 85%. A major advantage of IVF is that it requires a small number of spermatozoa to fertilize the collected oocytes, which at least at a glance favors the utilization of SS that typically has a dose of around 2.1 million sperm v. Sexed Ultra with 4 million sperm per straw. Initially, commercially available SS from AI centers was used. More recently, a procedure called reverse sorting of sperm (as described earlier) was developed to sort conventionally frozen semen before use in IVF (de Graaf et al., Reference de Graaf, Evans, Maxwell, Cran and O’Brien2007). The feasibility of reverse sorting was demonstrated when used in a large-scale donor IVP program (Morotti et al., Reference Morotti, Sanches, Pontes, Basso, Siqueira, Lisboa and Seneda2014). This technological breakthrough allowed seedstock producers to produce sex-specific embryos through IVP from their top females with the best genetic merit sires. Table 1 summarizes the differences and similarities between reverse sorted and conventional SS. Presently, reverse sorting is only being used in IVP due to the small number of sexed sperm cells recovered (de Graaf et al., Reference de Graaf, Evans, Maxwell, Cran and O’Brien2007).
Table 1 Differences and similarities between Bos indicus and Bos taurus reverse sorted semen and conventional SS

SS = sexed semen; IVP = in vitro production; AI = artificial insemination.
Presently, a limited amount of scientific literature has been published regarding the use of SS in IVP. The data primarily describe the performance under regular IVF conditions and differences between bulls (An et al., Reference An, Chaubal, Liu, Chen, Nedambale, Xu, Xue, Moreno, Tao, Presicce and Du2017). SS has commonly been used under similar conditions and protocols as conventional semen, and this has probably contributed to the low rates of cleavage and embryo development (Zhang et al., Reference Zhang, Lu and Seidel2003). Therefore, penetration of the oocyte ZP appears to be a major obstacle when using SS (Lu et al., Reference Lu, Cran and Seidel1999; Lu and Seidel, Reference Lu and Seidel2004). Potential underlying causes of this impaired functionality may be low post-thaw survival and motility, impaired capacitation and hyperactivation, and problems with acrosome integrity and fluorescent dye–DNA interaction (Garner, Reference Garner2001).
Few reports have been sufficiently rigorous in comparing SS with conventional semen, taking factors as sire identity, ejaculate, sperm and heparin concentrations into account (Liu et al., Reference Liu, Hu, Sun, Hao, Liu, Zhao, Zhu and Du2015), whereas other articles do not allow for a proper comparison because the semen does not come from the same ejaculate (i.e., splitting the ejaculate and using portion for conventional semen and the other portion for SS) or this information is not clear (An et al., Reference An, Chaubal, Liu, Chen, Nedambale, Xu, Xue, Moreno, Tao, Presicce and Du2017). A couple of articles that specifically made this kind of a same sire sexed to conventional sperm comparison were conducted by Bermejo-Alvarez et al. (Reference Bermejo-Alvarez, Lonergan, Rath, Gutierrez-Adan and Rizos2010a, Reference Bermejo-Alvarez, Rizos, Rath, Lonergan and Gutierrez-Adan2010b). Bermejo-Alvarez et al. (Reference Bermejo-Alvarez, Rizos, Rath, Lonergan and Gutierrez-Adan2010b) found that gene-expression variation may play a significant role in gender-specific early embryo development, specifically transcriptional differences occurring during preimplantation and therefore not attributable to sex-specific hormonal actions. Bermejo-Alvarez et al. (Reference Bermejo-Alvarez, Lonergan, Rath, Gutierrez-Adan and Rizos2010a) revealed mRNA abundance differences between bulls, but not between X- and Y-sorted spermatozoa, although sex-related differences were observed between male and female blastocysts for three genes (GSTM3, DNMT3A and PGRMC1).
The use of SS in IVP has shown mixed results (Lu et al., Reference Lu, Cran and Seidel1999;Zhang et al., Reference Zhang, Lu and Seidel2003; Blondin et al., Reference Blondin, Beaulieu, Fournier, Morin, Crawford, Madan and King2009; Xu et al., Reference Xu, Chaubal and Du2009). This high variability could be explained based on different lab protocols and the luck of standardized IVF conditions for SS. During the flow cytometry sorting process, sperm is subjected to different treatments such as DNA staining, dilution effect, centrifugation forces, high pressure, electrical charge, laser emissions and cryopreservation (Garner et al., Reference Garner, Evans and Seidel2013). The cause of diminished functional capacity of sex-sorted spermatozoa seems to be most likely multifactorial and cumulative. Consequently, post-thaw survival, sperm integrity, acrosome status, membrane functionality, motility pattern, DNA quality and/or the accumulation of insults (‘addition effect’) could be affected or compromised. A distinguish characteristic of flow cytometric sorting of X and Y chromosome-bearing sperm is a marked reduction in post-thaw motility and average lifespan (Suh et al., Reference Suh, Schenk and Seidel2005). In order to compensate or attenuate these inefficiencies, different adjustments have been tested (gametes co-incubation time, sperm dose sperm and heparin concentration; Lu and Seidel, Reference Lu and Seidel2004; An et al., Reference An, Chaubal, Liu, Chen, Nedambale, Xu, Xue, Moreno, Tao, Presicce and Du2017). Nevertheless, the comparison between SS v. conventional unsorted semen could be inappropriate if sire identity (of proven fertility) and ejaculate batch is not clearly stated (Liu et al., Reference Liu, Hu, Sun, Hao, Liu, Zhao, Zhu and Du2015).
Before fertilization, sperm need to travel through the female genital tract to reach the oviduct. Microendoscopic studies in sheep showed that this transit is very fast (Druart et al., Reference Druart, Cognie, Baril, Clement, Dacheux and Gatti2009). During the path to fertilization, oviduct secretions prime the sperm, protect both gametes against ROS, facilitate the fertilization process and promote an adequate microenvironment for the embryo to grow (Li and Winuthayanon, Reference Li and Winuthayanon2017). Oviduct secretions have been recognized to have the capacity to modulate sperm function and enhance sperm motility, capacitation, acrosome reaction and sperm–zona binding interaction (Killian, Reference Killian2011). Furthermore, oviduct secretions may also contribute to increase monospermy fertilization through the modulation of the ZP, which affects sperm–oocyte interaction and, ultimately, contribute to ameliorate the control of polyspermy (Mondéjar et al., Reference Mondéjar, Martínez-Martínez, Avilés and Coy2013).
Once sperm reach the utero-tubal junction, sperm will be trapped and released progressively to reach the oocyte at the fertilization site. The use of an in vitro model of bovine oviduct epithelial cells (BOECs) cultured as monolayers showed that sperm bind spontaneously to the cells and are released by progesterone and that sperm that have been bound to BOECs and released by progesterone action displayed a higher fertilization potential than those of the control (Lamy et al., Reference Lamy, Corbin, Blache, Garanina, Uzbekov, Mermillod and Saint-Dizier2017). A comparative proteomic analysis of bound v. control sperm showed that a total of 27 proteins are captured by the sperm (Lamy et al., Reference Lamy, Nogues, Combes-Soia, Tsikis, Labas, Mermillod, Druart and Saint-Dizier2018). One study, in particular, used recombinant heparin binding proteins, that is, fertility associated antigen and type-2 tissue inhibitor of metalloproteinase, to try and increase the fertility of sperm (Alvarez-Gallardo et al., Reference Alvarez-Gallardo, Kjelland, Moreno, Welsh, Randel, Lammoglia, Perez-Martinez, Lara-Sagahon, Esperon-Sumano and Romo2013). These interesting discoveries may open new research lines to improve the fertilization potential of spermatozoa, and especially of those that are of poor quality such as low fertility bulls with high genetic interest or compromised SS.
Embryo development
In IVF the fertilized oocytes are submitted to IVD for 7 days until they reach the blastocyst stage. In general, 20% to 40% of the cultured presumptive zygotes will reach the blastocyst stage (Rizos et al., Reference Rizos, Clemente, Bermejo-Alvarez, De La Fuente, Lonergan and Gutiérrez-Adán2008). After reaching the blastocyst stage, embryo transfer is performed following similar procedures as with in vivo blastocysts, or embryos are cryopreserved. However, only high-quality IVP embryos are recommended for cryopreservation in order to achieve higher post-thaw viability results overall, which can help increase pregnancy rates in the case of direct embryo transfer protocols. The most popular freezing method for IVP embryos is still vitrification (Vajta, Reference Vajta2000), although slow freezing is seeing a resurgence in popularity (Bruyere et al., Reference Bruyere, Baudot, Guyader-Joly, Guerin, Louis and Buff2012). New trends point out that selecting the ‘best’ or most viable embryos that tolerate the cryopreservation process for direct transfer, achieve the highest pregnancy rates. Individual embryo oxygen consumption rate, key substrate metabolite consumption uptake analysis (pyruvate, glucose, amino acids, among others) and production of metabolites via cellular metabolism (i.e., lactate) could become a gold standard (Donnay et al., Reference Donnay, Partridge and Leese1999).
Considering the high maturation and cleavage rates achieved, the relatively low rates of embryonic development to the blastocyst stage present a problem (Merton et al., Reference Merton, de Roos, Mullaart, de Ruigh, Kaal, Vos and Dieleman2003). Between 20% and 40% of cultured presumptive zygotes will reach the blastocyst stage (Lonergan et al., Reference Lonergan, Rizos, Ward and Boland2001) (Figure 4). This phenomenon may be due to incomplete cytoplasmic oocyte maturation, inadequate culture conditions, leading to impaired embryonic genome activation, or DNA fragmentation (Sirard et al., Reference Sirard, Richard, Blondin and Robert2006). Although maturation conditions can influence oocyte developmental competence, Tesfaye et al. (Reference Tesfaye, Ghanem, Carter, Fair, Sirard, Hoelker, Schellander and Lonergan2009) demonstrated that differences in oocyte developmental capacity after IVM v. maturation in vivo were accompanied by distinct differences in transcript abundance of the surrounding cumulus cells, with 64 genes differentially expressed between the two groups. More specifically, genes associated with cumulus expansion and regulation of oocyte maturation were upregulated in in vivo–derived cumulus cells, whereas cumulus cells associated with IVM oocytes were enriched for genes involved in response to stress (Tesfaye et al., Reference Tesfaye, Ghanem, Carter, Fair, Sirard, Hoelker, Schellander and Lonergan2009). New insight is needed to determine real oocyte competence and subsequent factors affecting developmental competence such as its ability to fuse with sperm, pronuclei formation, initiation of cell division, compaction and inner cell allocation, and blastulation.

Figure 4 Bos indicus and Bos taurus in vitro–produced (IVP) embryo success in each successive step.
Notably, a new technique called high-resolution chromosome conformation capture (Hi-C) is shedding some light on how chromatin structure changes from the oocyte to the cleaving embryo and whether separate pronuclei, representative of the paternal and maternal genomes, are exhibiting normal epigenetic patterning at appropriate stages of development (Akst, Reference Akst2017). This procedure could help elucidate where the failure in embryo development resides, that is, whether it is male- or female-derived, and steps to correct it could be implemented.
Initially, culture of bovine embryos was not performed in vitro but in the oviducts of various species (mice, rabbits, sheep and cattle, among the most popular; Lazzari et al., Reference Lazzari, Colleoni, Lagutina, Crotti, Turini, Tessaro, Brunetti, Duchi and Galli2010). In order to mimic the oviduct conditions, the first IVC included monolayers of BOECs for co-culture, which proved to be effective in producing high-quality freezable blastocysts (Abe and Hoshi, Reference Abe and Hoshi1997). The introduction of co-culture as embryo support aimed at (1) overcoming the early embryonic development arrest/blockage at the 8- to 16-cell stage, (2) increasing the blastocyst rate and (3) improving embryo quality and cryopreservation success (Orsi and Reischl, Reference Orsi and Reischl2007). The use of conditioned media from BOECs can mimic the co-culture effect, improving embryo development rate and quality (Eyestone and First, Reference Eyestone and First1989) and even serum-free conditioned media can be used in this view (Mermillod et al., Reference Mermillod, Vansteenbrugge, Wils, Mourmeaux, Massip and Dessy1993). In vivo, the oviduct fluid contains many proteins, including growth factors, heat shock proteins, free radical scavengers and enzymes, and its protein composition is evolving during the estrous cycle (Lamy et al., Reference Lamy, Nogues, Combes-Soia, Tsikis, Labas, Mermillod, Druart and Saint-Dizier2018). During culture, the BOECs are dedifferentiating and change their gene expression profile (Schmaltz-Panneau et al., Reference Schmaltz-Panneau, Locatelli, Uzbekova, Perreau and Mermillod2015), for instance, losing the expression of the P4 receptor and of oviduct specific glycoprotein (OVGP) which probably induces changes in their protein secretion profile and makes them unable to respond properly to hormonal stimulation. The oviduct fluid, as well as the BOEC conditioned media, has been shown to contain EVs, including a majority of exosomes (Alminana et al., Reference Alminana, Corbin, Tsikis, Alcantara-Neto, Labas, Reynaud, Galio, Uzbekov, Garanina, Druart and Mermillod2017). These EVs are able to cross the ZP and to be internalized by embryonic cells. In addition, the supplementation of the synthetic oviduct fluid (SOF) medium used for IVD improved the embryo development rate and quality. The identification of active factors in exosomes and their use in IVD or the use of exosomes themselves to help embryo development may represent an interesting research line for improving the quality of IVP embryos.
Culture conditions can influence embryo quality and cryotolerance (Rizos et al., Reference Rizos, Ward, Duffy, Boland and Lonergan2002). Different strategies have been used to compensate the current suboptimal IVP embryo development microenvironment. One of the most popular strategies involves the addition of supplements such as fetal bovine serum (FBS), serum replacements or bovine serum albumin (BSA). FBS, above certain concentrations (>5% v/v), may improve embryo production, but it has been associated with compromising cryotolerance (Rizos et al., Reference Rizos, Gutierrez-Adan, Perez-Garnelo, De La Fuente, Boland and Lonergan2003) and inducing LOS (Lazzari et al., Reference Lazzari, Wrenzycki, Herrmann, Duchi, Kruip, Niemann and Galli2002). When bovine zygotes were cultured in the ewe (Lazzari et al., Reference Lazzari, Colleoni, Lagutina, Crotti, Turini, Tessaro, Brunetti, Duchi and Galli2010), rabbit (Ectors et al., Reference Ectors, Thonon, Delval, Fontes, Touati, Beckers and Ectors1993), mouse (Rizos et al., Reference Rizos, Ramirez, Pintado, Lonergan and Gutierrez-Adan2010) or even the homologous bovine (Gad et al., Reference Gad, Hoelker, Besenfelder, Havlicek, Cinar, Rings, Held, Dufort, Sirard, Schellander and Tesfaye2012) oviduct, blastocyst quality, cryotolerance, embryonic genome activation and gene expression were similar to that of the in vivo–derived counterpart. Furthermore, the use of BOECs showed positive effects on embryo development and quality (Schmaltz-Panneau et al., Reference Schmaltz-Panneau, Locatelli, Uzbekova, Perreau and Mermillod2015). The composition of oviductal fluid (OF) is very complex (Li and Winuthayanon, Reference Li and Winuthayanon2017) and its addition to the culture phase enhanced embryo development rates and blastocyst quality assessed through cryotolerance, differential allocation of inner cell mass and trophectoderm cells, and gene expression (Lopera-Vasquez et al., Reference Lopera-Vasquez, Hamdi, Maillo, Gutierrez-Adan, Bermejo-Alvarez, Ramírez, Yáñez-Mó and Rizos2017).
The use of FBS has been demonstrated to cause severe fetal/calf deviations collectively referred to as LOS (Farin et al., Reference Farin, Crosier and Farin2001). The use of FBS as a culture additive remains controversial. Addition of serum at levels greater than 10% (v/v) may cause LOS; however, FBS seems to stimulate embryo development from day 4 to day 5 of culture (Thompson et al., Reference Thompson, Allen, McGowan, Bell, Lambert and Tervit1998; Holm et al., Reference Holm, Booth, Schmidt, Greve and Callesen1999) even at reduced concentrations (<3% v/v). Currently, serum-free media formulations based on the use of serum replacements or BSA can result in similar or even higher blastocyst rates and quality as compared to those including FBS (Stroebech et al., Reference Stroebech, Mazzoni, Pedersen, Freude, Kadarmideen, Callesen and Hyttel2015).
Importance of media for in vitro production of embryos
With the increasing commercial implementation of bovine embryo IVP worldwide, there is an increased focus on optimizing blastocyst yield and quality. Furthermore, an emphasized focus on regulatory restrictions concerning import/export of embryos cultured in media containing animal-based serum, due to the risk of spreading pathogens, has increased the desire to exclude serum from IVP media.
Although the commercial use of IVP embryos has been growing worldwide, there still exists a need to improve yield and quality in order to increase the pregnancy rate and achieve deep freezing for direct transfer. In addition, limited options concerning serum-free specially formulated bovine culture media are commercially available today that comply with the international standards and regulations defined by IETS (Stroebech et al., Reference Stroebech, Mazzoni, Pedersen, Freude, Kadarmideen, Callesen and Hyttel2015). The Danish company, EmbryoTrans Biotech ApS (Haslev, Denmark), has developed an entire portfolio of IVP media with synthetic serum replacement in which all steps from IVM through IVF and IVD until the blastocyst stage are performed in media without serum. The vast majority of bovine commercial and research IVP laboratories prepared all their own culture media in-house using commercially available mammalian cell culture solutions, such us Tissue Culture Medium 199 (TCM 199; Sigma-Aldrich, St. Louis, MO, USA), or Tyrode’s Albumin Lactate Pyruvate (TALP; Parrish et al., Reference Parrish, Susko-Parrish, Leibfried-Rutledge, Critser, Eyestone and First1986) and SOF (Holm et al., Reference Holm, Booth, Schmidt, Greve and Callesen1999) stocks with additives. In general, bovine IVP culture media required balanced salt solution (Earle’s salts for M199), sodium bicarbonate, essential and non-essential amino acids, l-glutamine, phosphatase, pyruvate, antibiotics, vitamins, EDTA, sugar (fructose or glucose) and other protector factors (i.e., antioxidants) and gamete/embryo performance promoters or nutrients (Gardner, Reference Gardner2008).
As a medium for IVD of embryos, SOF has been used as the most continuous culture medium system. However, an increasing interest to perform sequential culture has evolved at some of the commercial bovine laboratories. The concept of sequential media was specifically designed to meet the changing requirements of the embryo during this developmental period (Thompson and Peterson, Reference Thompson and Peterson2000) (Figure 5). The sequential media culture system is based on the concept that the embryo has different needs during its growth and that the ability to metabolize glucose is acquired progressively (Bavister, Reference Bavister1995). On the other hand, a monoculture medium system includes a single formulation with the need to ensure embryo development up to blastocyst stage. The science behind this approach is to allow the embryo to choose (‘à la carte’) the necessary nutrients to support full development (Gardner and Lane, Reference Gardner, Lane, Cibelli, Lanza, Campbell and West2002). Both strategies, sequential and monoculture medium systems, could be equally efficient (Macklon et al., Reference Macklon, Pieters, Hassan, Jeucken, Eijkemans and Fauser2002), where less pH and temperature disturbances may occur during a monoculture system culture (Swain, Reference Swain2010). The advantage of minimizing outside incubator manipulations may contribute to improved blastocyst formation performance and act as a compensation factor. The production of autocrine/paracrine beneficial factors by close-proximity embryos and the fact to maintaining constant culture conditions could contribute to an increased concentration of such autocrine/paracrine embryo promoting factors and increase their survival (Gopichandran and Leese, Reference Gopichandran and Leese2006).

Figure 5 Schematic representation of three approaches to culturing Bos indicus and Bos taurus embryos from the zygote to blastocyst stage.
Several bovine sequential embryo culture media have been presented in publications: Early-SOF/Late-SOF (Thompson, Reference Thompson2000), SOFC1/SOFC2 (Gandhi et al., Reference Gandhi, Lane, Gardner and Krisher2000), G1/G2 (Lane et al., Reference Lane, Gardner, Hasler and Hasler2003) and CDM-1/CDM-2 (Olson and Seidel, Reference Olson and Seidel2000). The two-step sequential media may involve the addition and/or subtraction of particular components of the basal medium formulation (Thompson et al., Reference Thompson, Allen, McGowan, Bell, Lambert and Tervit1998; Lane et al., Reference Lane, Gardner, Hasler and Hasler2003). Another variation in this two-step sequential media protocol is the use of two completely different culture media, that is, each with unique chemical components, for each developmental growing phase (KSOM/SOF) (Nedambale et al., Reference Nedambale, Dinnyes, Groen, Dobrinsky, Tian and Yang2004).
Single formulation (one-step) bovine embryo culture media such as CR1 (Rosenkrans and First, Reference Rosenkrans and First1994), SOF (Tervit et al., Reference Tervit, Whittingham and Rowson1972), BECM (Lim et al., Reference Lim, Mei, Chen, Godke and Hansel1999), KSOM (Liu and Foote, Reference Liu and Foote1995) and IVD101 (Abe and Hoshi, Reference Abe and Hoshi2003) usually come in two versions: without renewal (uninterrupted; Holm et al., Reference Holm, Booth, Schmidt, Greve and Callesen1999) or with renewal (interrupted) every 48 h to prevent the accumulation of toxic substances (Moore et al., Reference Moore, Rodriguez-Sallaberry, Kramer, Johnson, Wroclawska, Goicoa and Niasari-Naslaji2007). Figure 5 illustrates the temporal aspects often associated with these one-step v. two-step embryo culture media protocols. New bovine commercial ‘ready to use’ and ‘serum-free’ IVP media have been released and (Stroebech et al., Reference Stroebech, Mazzoni, Pedersen, Freude, Kadarmideen, Callesen and Hyttel2015) may contribute to more stable production systems, as they reduce the batch-to-batch variability of a laboratory-made medium. Advantages and disadvantages of the single monoculture media and sequential media formulations are presented in Table 2.
Table 2 Advantages and disadvantages of three approaches to culturing mammalian embryos from the zygote to blastocyst stage (Biggers and Summers, Reference Biggers and Summers2008)

Current modern benchtop incubators have switched from large volume capacity (>150 l) to mini-chambers (<500 ml). These new incubators offer multiple independent compartments each equipped with temperature, CO2 and O2 sensors enabling total control of each environment separately and providing faster recovery times (Kelly and Cho, Reference Kelly, Cho and Quinn2014). In addition, embryo developmental insight into rates of development and identification of morphological abnormalities occurring at given stages of development has been gained using real time-lapse embryo culture monitoring systems with built-in cameras installed inside the mini-chamber incubator (Kovacs, Reference Kovacs2014). Such systems combine embryo appearance (morphology) and timing of early embryo cleavage divisions (kinetics) into integrated computer software to identify viable embryos with high implantation potential (Munevver et al., Reference Munevver, Findikli, Bahceci and Schatten2017). The next generation embryo culture platforms will likely be based on microfluidic technology, changing from static to dynamic culture, automatic addition of specific chemicals at a pre-selected time, and allowing for gradual/partial changes in the medium formulation and thereby avoiding temperature and pH shocks (Wheeler and Rubessa, Reference Wheeler and Rubessa2017).
With the increased use of comprehensive chromosome screening in the human IVF field, the development of an efficient and practical blastocyst biopsy system is desired. Certainly, the culture environment and the manipulations of the embryo during culture period can impact its developmental and fertility capacity potential. Due to changes in embryo requirements during growth, the use of a specific media formulation similar to oviductal secretions may contribute to improving embryo yield performance. Under in vivo conditions, the developing embryo migrates from the oviduct to the uterine lumen where the fluid composition and gas atmosphere are likely different. Therefore, static culture systems may not be adequately re-creating the necessary embryo development environment. The dynamic systems, on the other hand, allow a gradual, precise and time-specific alteration of culture media, restore consumed nutrients, supply new nutrients and remove waste and toxic metabolic derivatives, offer the automation of certain processes (denudation), while facilitating basic gamete/embryo handling and reduced environmental stress (Wheeler and Rubessa, Reference Wheeler and Rubessa2017).
There is a remarkable knowledge platform of the necessary requirements at several stages of embryo development for achieving optimal developmental rates although much can still be learned from embryo culture systems that provide important insights in order to develop a completely defined and optimized media (Baltz, Reference Baltz2013). Novel devices, platforms and dynamic systems may offer a pathway toward the optimization of embryo culture conditions, attempting to maximize gamete competence, viability, cryotolerance and pregnancy rate (Zhao and Fu, Reference Zhao and Fu2017). Quality of in vitro–derived embryos is still inferior compared to those derived in vivo. However, culture of in vitro–produced bovine zygotes under in vivo (ewe or cow oviduct) conditions restores their quality similar to totally in vivo–produced embryos. Conversely, in vivo–produced bovine zygotes cultured in vitro typically produce blastocysts with a lower quality compared to fully in vivo–cultured blastocysts.
Viable gametes and embryos possess unique molecular profiles and ‘omics technologies’, including transcriptomics, proteomics and metabolomics, may be considered potential biomarkers and be utilized for embryo developmental and/or embryo viability selection (Fontanesi, Reference Fontanesi2016). In addition, the use of extracellular RNA analysis of the embryo culture media appears to be another promising embryo selection technology (Kropp and Khatib, Reference Kropp and Khatib2015). The combination of advanced energetic substrate metabolism analysis with real time-lapse embryo monitoring allows for continuous, non-invasive embryo observation and selection. Different prognostic factors (timing of the first cleavage, number of blastomeres, timing of morula compaction and inner cell allocation, timing of blastocoel formation, developmental arrest, blastocyst formation) could also help to identify the embryos with the highest chances of surviving cryopreservation, as well as for improving pregnancy rates. Although there is still much to be done, with regard to investigation and validation, several upfront advantages may increase IVF/IVP performance due to a drastic reduction in routine culture dish air exposure for recording specific information (cleavage, blastocyst formation, hatching) and elimination of subjective morphological embryo quality evaluation given the questionable viability predictive value.
Another interesting and recent technology has been successfully applied concerning the vitrification and ultra-rapid thawing of zebrafish embryos and coral larvae. The new vitrification method addresses the cryopreservation problem by using gold nanorods (GNRs) to assist with the thawing process. Khosla et al. (Reference Khosla, Wang, Hagedorn, Qin and Bischof2017) microinjected propylene glycol into zebrafish embryos along with GNRs, followed by vitrification in liquid nitrogen. They demonstrated the ability to rapidly thaw the zebrafish embryos rapidly (1.4 × 107°C/min) by irradiating the sample with a 1064 nm laser pulse for 1 ms. Recently, a similar procedure was conducted resulting in the first successful vitrification and post-thaw survival of coral larvae (Daly et al., Reference Daly, Zuchowicz, Nuñez Lendo, Khosla, Lager, Henley, Bischof, Kleinhans, Lin, Peters and Hagedorn2018). To our knowledge, this type of vitrification approach has not yet been applied to bovine oocytes and embryos, but the technique may hold promise under certain circumstances, for example, vitrification of immature oocytes of difficult-to-cryopreserve domestic and wild species or even cloned embryos.
Preconditioning follicles prior to oocyte retrieval through synchronization and stimulation can contribute to achieving the ultimate goal of reaching 100% blastocyst development under standard in vitro conditions (Nivet et al., Reference Nivet, Bunel, Labrecque, Belanger, Vigneault, Blondin and Sirard2012). This evidence also demonstrates that despite a suboptimal culture environment, the system does not handicap zygote performance when initial oocyte competence is not compromised. Furthermore, embryo formation is mainly affected by oocyte origin, while cryosurvival and gene expression can be altered by culture conditions regardless of the origin of the oocyte (Lonergan et al., Reference Lonergan, Rizos, Gutierrez-Adan, Fair and Boland2003).
Presently, bovine in vitro–derived embryos are less competent in terms of implanting and making a pregnancy, and more sensitive to the cryopreservation process compared with in vivo–frozen embryos (Nedambale et al., Reference Nedambale, Dinnyes, Groen, Dobrinsky, Tian and Yang2004). After the genomic era, embryos in different stages of development could be compared against in vivo in order to establish abnormalities in expression patterns. In vitro embryos are particularly sensitive to culture conditions at four-cell and morula stages (Gad et al., Reference Gad, Hoelker, Besenfelder, Havlicek, Cinar, Rings, Held, Dufort, Sirard, Schellander and Tesfaye2012). Nevertheless, we should expect gene expression, metabolism, performance and tolerance differences between in vitro–derived and in vivo embryos due to the fact that the in vitro environment is not exactly the same as the in vivo one. These differences observed between in vitro and in vivo embryos could be explained as an adaptive response to the environment and culture conditions. Certainly, the epigenetic effect in embryos as a consequence of culture conditions required adjustments (Wrenzycki, Reference Wrenzycki2016). Embryo selection prior to transfer remains as a subjective phenotype analysis performed by a skilled embryologist. Consequently, new non-invasive techniques to select viable embryos are required in order to predict in vitro developmental potential and implantation success (Van Soom et al., Reference Van Soom, Mateusen, Leroy and de Kruif2003). Therefore, cryoresistance evaluation was added as a viability test parameter (Massip and Leibo, Reference Massip, Leibo, Soom and Boerjan2002) along with many other biomarker-based methods to supplement the traditional morphological assessment (Rødgaard et al., Reference Rødgaard, Heegaard and Callesen2015). New approaches, such as morphokinetics (Sugimura et al., Reference Sugimura, Akai and Imai2017) and advanced genomic screening techniques, may become routine for embryo selection in a commercial IVP laboratory. In the near future, CRISPRCas-9 type–related technologies could assist in performing precise genome editing or epigenome editing for biomedical applications and bioreactors (Yum et al., Reference Yum, Youn, Choi and Jang2018).
Conclusions
There are still unresolved drawbacks of IVP that limit a wider implementation of the technology including reduced oocyte quality after IVM, lower embryo cryotolerance and reduced pregnancy rates. Communication between embryo and maternal environment is probably an important research target to understand and use maternal factors that can positively regulate embryo development. Novel improved sperm sexing technologies have been reported to result in better fertility and conception rates using artificial intelligence and potentially also IVP. Nevertheless, more data are needed to consolidate this promising research. The combination of OPU, IVP, SS and GS has proven successful in the commercial field in several countries, thereby permitting practitioners and cattle producers to improve reproductive performance, efficiency and genetic gain.
Acknowledgement
The writing process was supported by Innovation Fund Denmark (EliteOva).
Declaration of interest
None.
Ethics statement
None.
Software and data repository resources
None.