Introduction
Although genetically modified cotton expressing Bt toxin is effective in Australia in controlling Helicoverpa spp., farmers, scouts and researchers occasionally report surviving larvae of all sizes for short periods in all growing regions (Fitt, Reference Fitt2003; Whitburn and Downes, Reference Whitburn and Downes2009). Specifically, a survey conducted from 2005 to 2008 estimated that on average 15% of the area planted to Bollgard II, a Bt cotton expressing two cry toxin genes, carried larvae at or above the threshold levels recommended for applying a control spray (Wilson et al., Reference Wilson, Downes, Khan, Whitehouse, Baker, Grundy and Maas2013). Survival of larvae on Bt cotton is not necessarily due to physiological resistance (Lu et al., Reference Lu, Downes, Wilson, Gregg, Knight, Kauter and McCorkell2011). A number of potential alternative mechanisms may be responsible for the higher than expected survival, including poor gene expression in genetically modified plants (Lu et al., Reference Lu, Downes, Wilson, Gregg, Knight, Kauter and McCorkell2011), pest load or pressure due to climate suitability (Zalucki and Furlong, Reference Zalucki and Furlong2005), and/or behavioural mechanisms (Yang et al., Reference Yang, Johnson and Zalucki2008; Zalucki and Furlong, Reference Zalucki and Furlong2017) including behavioural resistance (Liu et al., Reference Liu, Xu, Zhu, Huang, Wang, Li, Gao, Zhou and Shen2010). Behavioural resistance could occur for instance, if larvae survive on plants due to where females placed eggs or by moving to find ‘safe havens’, thereby avoiding induced defences and minimizing exposure to constitutive defences (Perkins et al., Reference Perkins, Cribb, Brewer, Hanan, Grant, de Torres and Zalucki2013; Luong et al., Reference Luong, Zalucki, Cribb, Perkins and Downes2016), including Bt toxin (Downes and Mahon, Reference Downes and Mahon2012a, Reference Downes and Mahon2012b), and both mechanisms have a genetic basis.
When a host plant is unsuitable for larvae to settle and begin feeding, larvae often leave by dropping down from leaves (or other substrates) on a silk thread (Moore and Hanks, Reference Moore and Hanks2004). This dispersal-related behaviour of larval Lepidoptera has been referred to as ‘drop-off’, ‘spin-down’, ‘silking’ and ‘bungy jumping’ (Terry et al., Reference Terry, Bradley and Duyn1989; Zalucki et al., Reference Zalucki, Clarke and Malcolm2002; Moore and Hanks, Reference Moore and Hanks2004; Perović et al., Reference Perović, Johnson, Scholz and Zalucki2008). Larval dispersal may be different on Bt cotton plants compared to non-Bt cotton plants. Helicoverpa zea larvae move more rapidly and cover a greater vertical distance on Bt cotton than non-Bt cotton (Gore et al., Reference Gore, Leonard, Church and Cook2002); they moved away from Bollgard cotton terminals within 1 h, and <10% remained on cotton plants after 6 h. In addition, larvae of various Lepidoptera including H. zea ‘avoid’ Bt toxin in artificial diet (Gould and Anderson, Reference Gould and Anderson1991; Farrar and Ridgway, Reference Farrar and Ridgway1995; Stapel et al., Reference Stapel, Waters, Ruberson and Lewis1998; Gore et al., Reference Gore, Adamczyk and Blanco2005; Singh et al., Reference Singh, Rup and Koul2008) and Greenplate et al. (Reference Greenplate, Head, Penn and Kabuye1998) indicated that larvae avoid feeding on diet containing dried Bt cotton plant material. Yang et al. (Reference Yang, Johnson and Zalucki2008) suggested that larvae could survive if they could find squares/flowers but assumed that larvae behaved the same way on conventional and Bt cotton plants. Lu (Reference Lu2010), however, found that the survival of larvae did not correlate well with Bt toxin levels among the structures of Bollgard II® cotton plants.
Feeding behaviour may change in response to widespread adoption of Bt cotton as a result of behavioural resistance (Yang et al., Reference Yang, Johnson and Zalucki2008; Liu et al., Reference Liu, Xu, Zhu, Huang, Wang, Li, Gao, Zhou and Shen2010). Understanding the behaviour of larvae of Helicoverpa armigera that exhibit different physiological resistance to Bt toxins on Bt and non-Bt cotton plants is an important component of resistance management strategies for this insect. Here we study the drop-off behaviour of two H. armigera strains (Bt-resistant and -susceptible larvae) to determine whether they move the same way or differently in response to Bt and non-Bt cotton. This information will help address the question of how physiologically Bt-susceptible H. armigera can survive on Bt-cotton plants.
Materials and methods
Materials
Plants
Conventional cotton (Sicot 71 RRF) (here after non-Bt cotton) and a GM cotton, Bollgard II®, in the same cotton background (Sicot 71 BRF) (Bt cotton expressing Cry1Ac and Cry2Ab) were used to test the movement behaviour of newly-hatched larvae. At the time of the study, these were the most popular commercial varieties grown in Australia. Three seeds were sown in each pot (30 cm height and 25 cm diameter) in a standard potting mix (a mixture of sand, bark and peat moss). After germination, the largest seedling was selected to be grown, and the others were removed. Plants were maintained in a glasshouse at The University of Queensland (hereafter UQ) where the average temperature and relative humidity were 24 ± 6°C and 56 ± 10%, respectively. All plants were watered three times a week and supplied with general purpose Thrive soluble fertilizer (N:K:P:MgO at 16:9:12:2) every 4 weeks. The plants used in experiments were at flowering and/or squaring stages with nine or ten nodes from the top of plant, with flowers (fully opened), and squares present (fig. 1).
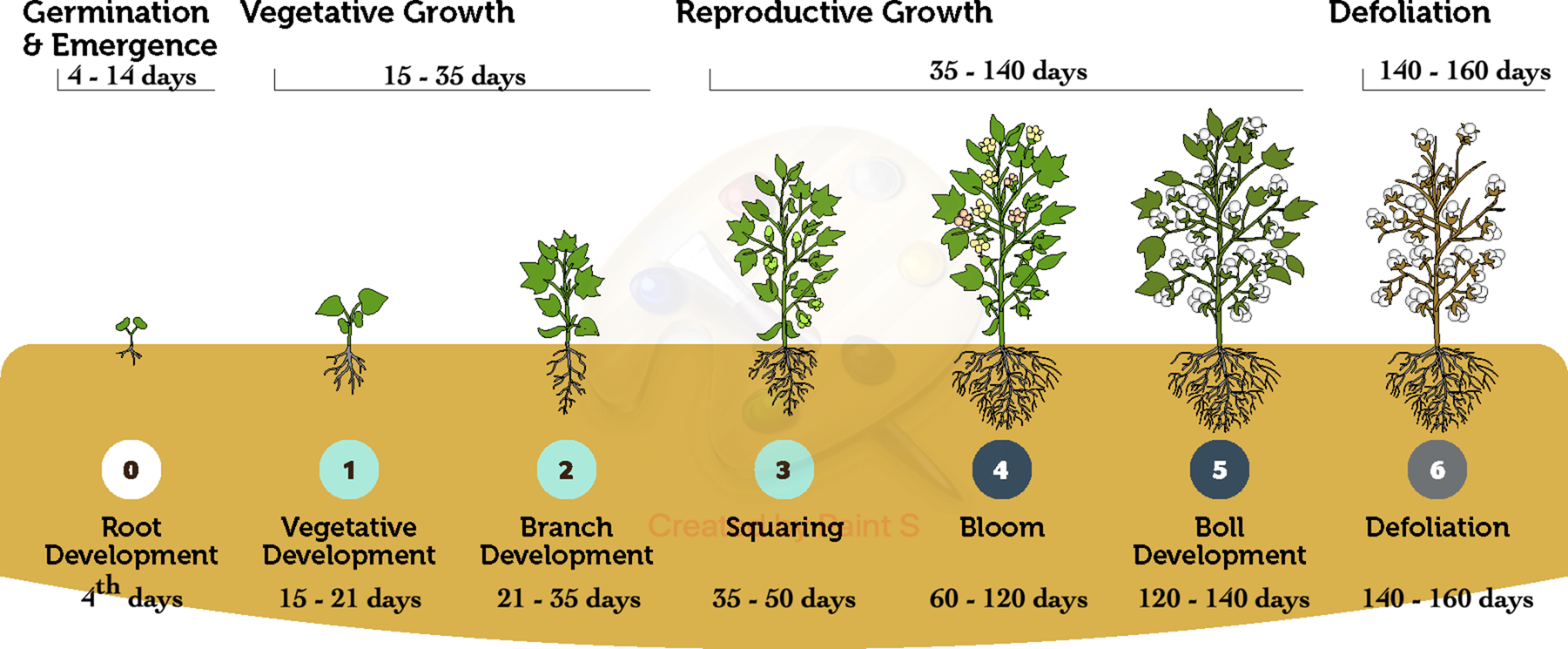
Figure 1. Growth stages and development of a cotton plant.
Insects
The H. armigera Bt-resistant strain used in this study (known as ‘SP15’) was established from a single mating pair collected as eggs on corn near Griffith, NSW, in December 2002. Progeny from the pair were subjected to an F2 screen (Andow and Alstad, Reference Andow and Alstad1998) and the SP15 colony was formed from F2 offspring that survived a discriminating dose (LD 95) (1 μg cm−2) of Cry2Ab (Mahon et al., Reference Mahon, Olsen, Garsia and Young2007). The F2 screens were performed with the specific intention of detecting resistance to Cry toxins in H. armigera. SP15 initially possessed a very restricted gene pool as it originated from a single isofemale line. Lepidopteran colonies suffer severe inbreeding depression rapidly leading to a loss of vigour that strongly influences the outcome of bioassays. Consequently, over the years since its isolation, SP15 has been outcrossed to the susceptible strain, GR, numerous times, to maintain fitness and to produce a strain that is near isogenic with the susceptible strain (Mahon et al., Reference Mahon, Olsen, Garsia and Young2007). Following each outcross, the colony was maintained without selection for one generation and then re-selected with 1–2 μg cm−2 Cry2Ab toxin as a diet surface treatment. Dried and ground corn (Zea mays L.) leaf material was used as a source of Cry2Ab toxin. Corn powder was provided by Monsanto (St Louis, USA) as a lyophilized leaf powder. This powder contained the transgenically expressed Bacillus thuringiensis crystal protein Cry2Ab, at a concentration of 6 mg g−1 powder (Mahon et al., Reference Mahon, Olsen, Garsia and Young2007). Toxin in the leaf was calibrated using an enzyme-linked immune-sorbent assay (ELISA) method on aliquots of leaf material after freeze-drying and homogenization. ELISA methods and protein extraction are detailed in Holt et al. (Reference Holt, Mares and Akhurst2002). All subsequent generations were selected at this dose. Moths used to establish a susceptible H. armigera colony were collected from the field from a range of crops such as chickpea, pigeon pea, cotton, etc., and bulk mated to form a colony. All colonies were maintained at the Australian Cotton Research Institute, Narrabri, New South Wales.
Pupae of Bt-resistant and -susceptible larvae from Narrabri were maintained at an average temperature of 250 ± 10°C and 80% RH ± 1%, and a photoperiod of 12:12 h (L:D) at UQ. After emergence, male and female moths were placed together in a container (20 cm width × 20 cm length × 30 cm height) and supplied with 10% sucrose solution. They were allowed to mate and females were able to oviposit on fabric covering the top of the container. The fabric with eggs was placed in a sealed plastic bag with a wet-cotton wick to prevent the eggs from desiccating. Newly-hatch neonates (≤1 h) were used in each of the experiments. For general rearing and experiments, a standard soyflour-based artificial diet was used which is described by Teakle and Jensen (Reference Teakle, Jensen, Singh and Moore1985) and later modified in Perkins et al. (Reference Perkins, Cribb, Hanan and Zalucki2010).
Experiments on the drop-off behaviour of H. armigera neonates resistant and susceptible to Bt were conducted on artificial diet with and without Bt toxin and on Bt and non-Bt cotton plants (Bt and non-Bt) in the laboratory.
Methods
Experiment 1: drop-off behaviour of Bt-resistant and -susceptible H. armigera neonates on artificial diet with and without Bt toxin
Experiments examined how larvae with different physiologies (Bt-resistant and Bt-susceptible strains) behaved in terms of drop-off behaviour when placed on artificial diet with and without Bt toxin. The apparatus was designed as a complex of two petri dishes: a small petri dish (9 mm in diameter) sitting atop a 10 mm-high column above a large petri dish (15 mm in diameter). Each apparatus had one of two treatments: (1) the small petri dishes containing artificial diet (2 mm thick) with 1–2 mg cm−2 Cry2Ab applied to the surface, and (2) an experimental control with artificial diet and water as a surface treatment. Treatments were spread evenly over the surface and allowed to dry. All large dishes contained artificial diet that was not treated. On each small dish, 15–20 neonates were introduced (fig. 2). The number and position (on small dishes or large dishes) of larvae were recorded at 30, 60, 90, 120, 180 min and 12 h. There were 40 replicates for Bt-resistant larvae (20 exposed to Bt-treated diet and 20 controls) and 54 replicates for Bt-susceptible larvae (29 exposed to Bt-treated diet and 25 controls).
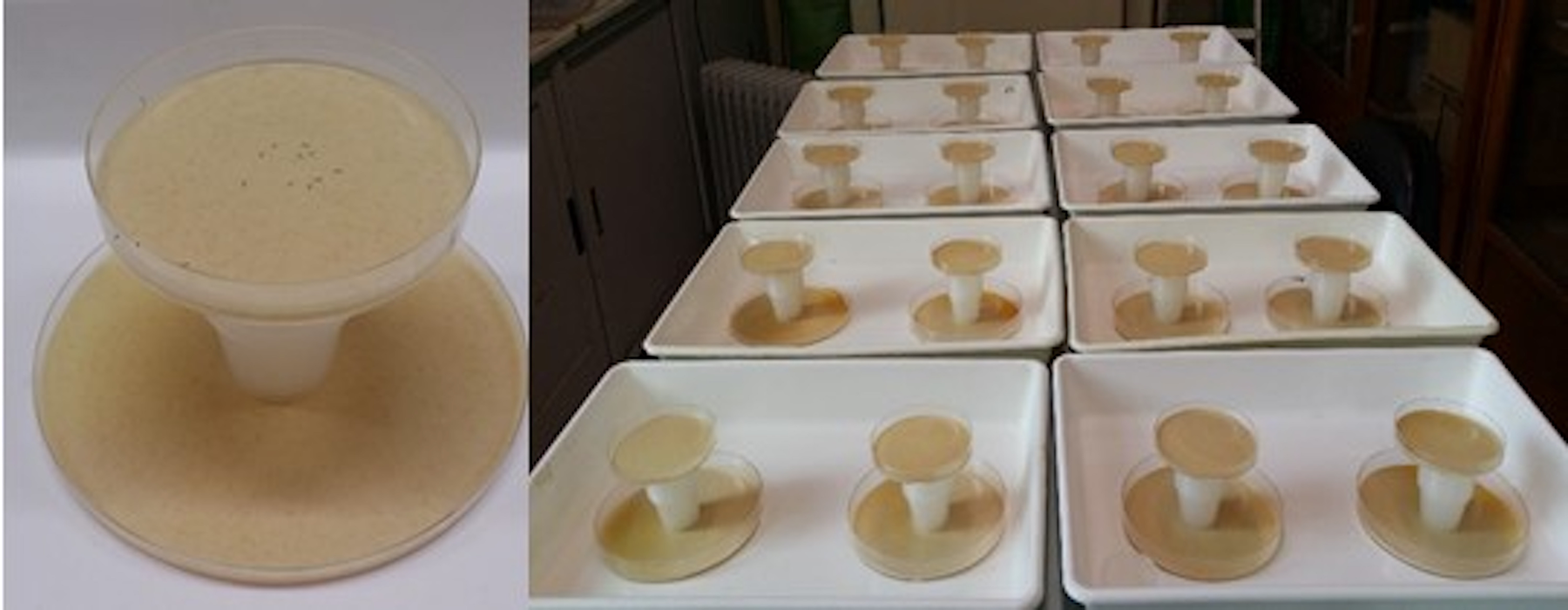
Figure 2. The design of the drop-off experiment on artificial diet.
After 12 h, the survival of Bt-resistant larvae was similar in all treatments with and without Bt toxin (Luong et al., Reference Luong, Zalucki, Cribb, Perkins and Downes2018). Thus, only surviving Bt-susceptible larvae that had moved or stayed were transferred after 12 h to artificial diet and reared to examine the difference in their survival with respect to drop-off behaviour. The survival of larvae that dropped off or remained on Bt and non-Bt diet was recorded at 3 days.
Experiment 2: drop-off behaviour of Bt-resistant and -susceptible H. armigera neonates on Bt cotton and non-Bt cotton plants
This experiment examined if drop-off behaviour of Bt-resistant and -susceptible H. armigera neonates differed between Bt and non-Bt cotton plants. Individual Bt and non-Bt cotton plants were transferred from the glasshouse to the laboratory for the experiment. Before doing the experiment, plants were checked to ensure that they were clean of mites and other insects (whiteflies, thrips, etc.). All plants used in the experiment were at flowering, and/or squaring stage. Larvae used for the experiment were neonates within 1 h of hatching.
An experiment was performed for each strain of larvae (Bt-resistant and -susceptible) as follows. Ten or 12 trays were arranged in two rows with five or six trays in each row; one row of trays held Bt cotton plants and the other held non-Bt cotton plants. Each tray contained one cotton plant. Within each row, plants were placed randomly. Trays were filled with water. On each plant, 20 newly-hatched neonates were divided into four groups of five and placed using a fine brush on one of four different positions: (1) a young leaf, (2) a terminal, (3) a mature leaf and (4) a second mature leaf (fig. 3). The number of neonates (Bt-resistant and Bt-susceptible) remaining on cotton plants (Bt and non-Bt cotton) was recorded at 1, 2, 3 and 6 h after being released. The potted cotton plants were placed on a table in the laboratory without exposure to wind, at 28 ± 3°C and 50–80% RH.
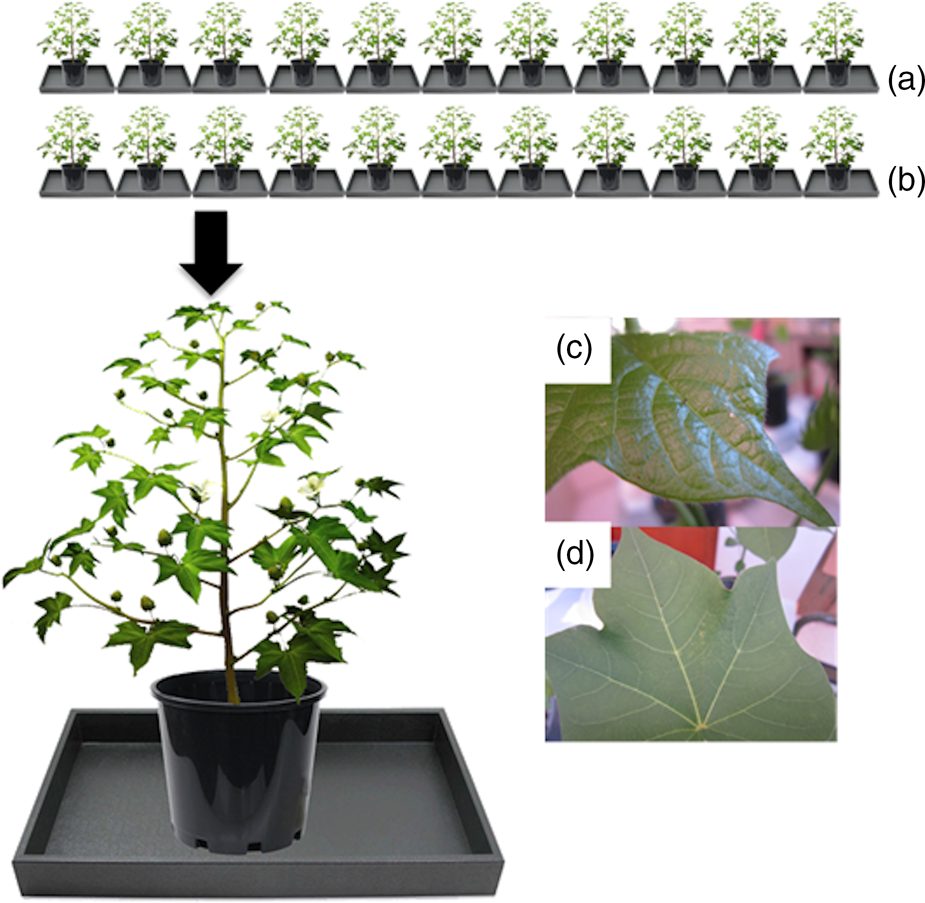
Figure 3. A diagram of the design of the drop-off experiment with plants of (a) Bt cotton and (b) non-Bt cotton arranged in two rows on a bench in the laboratory. Newly-hatched neonates were placed on: (c) terminals, (d) young leaves or (e) mature leaves.
Each plant constituted a replicate. The experiment was repeated four times giving a total of 44 replicates for the Bt-resistant strain and 44 replicates for the Bt-susceptible strain for both cotton species (i.e. 22 replicates for each cotton and strain combination). A total of 880 Bt-resistant and 880 Bt-susceptible neonates were used.
Data analysis
All of the data from experiments was converted to percentages and arcsin√x transformed to normalize it. All statistical analyses were performed in MINITAB v.19.0 (Minitab, Sydney, NSW 2000, Australia).
Three-way repeated-measures ANOVA was used to determine whether there were effects of strain of larvae (Bt-resistant and -susceptible neonates), substrate (artificial diet or cotton plants with and without Bt toxin), and time exposed to substrate (artificial diet or cotton plants) on H. armigera neonate drop-off behaviour as measured by the percentage of larvae that dropped-off their starting substrate. In the first experiment, there are two levels of strain of larvae (Bt-resistant and -susceptible neonates), two levels of artificial diet (Bt diet and non-Bt diet) and six levels of time (30, 60, 90, 120, 180 min and 12 h). In the second experiment, there are two levels of strain of larvae (Bt-resistant and -susceptible neonates), two levels of plant genotypes (Bt and non-Bt cotton) and four levels of time (1, 2, 3 and 6 h). Tukey Pairwise Comparisons were used to separate means at the 95% confidence level. Two-way ANOVAs were performed on the data of larval drop-off at each exposure time period separately. One-way ANOVAs were used for data analysis from each strain separately, and each diet separately.
Results
Experiment 1: drop-off behaviour of Bt-resistant and Bt-susceptible H. armigera larvae on artificial diet with and without Bt toxin
Three-way repeated-measures ANOVA analysis showed that the three-way interaction among strain of larvae, diet treatment and time interval was significant (F (5,563) = 2.67, P = 0.021) (table 1). There was also significant two-way interactions between strain of larvae and time intervals (F (5,563) = 2.47, P = 0.032) and between larval strain and diet treatment (F (1,563) = 41.43, P < 0.01) suggesting that the different H. armigera larval strains (Bt-resistant and Bt-susceptible) reacted differently to the presence or absence of Bt toxin on artificial diet at different time of exposure. There was no significant interaction between larval behaviour on the different artificial diet treatments and the time exposed to diet (F (5,563) = 0.74, P = 0.594). All three factors showed significant main effects: Bt-resistant and -susceptible strains differed in the percentages of larvae that dropped off (F 1,563 = 8.37, P = 0.004), artificial diet treatment (covered with Bt toxin or water) affected drop-off behaviour of larvae (F 1,563 = 8.37, P = 0.011), and time exposed to the diet treatment also affected drop-off of larvae (F 5,563 = 35.35, P < 0.001).
Table 1. Mean percentage of Bt-resistant (SP15) and Bt-susceptible (GR) Helicoverpa armigera larvae that dropped off Bt and non-Bt diet after each 30 min until 180 min and at 12 h of observation time
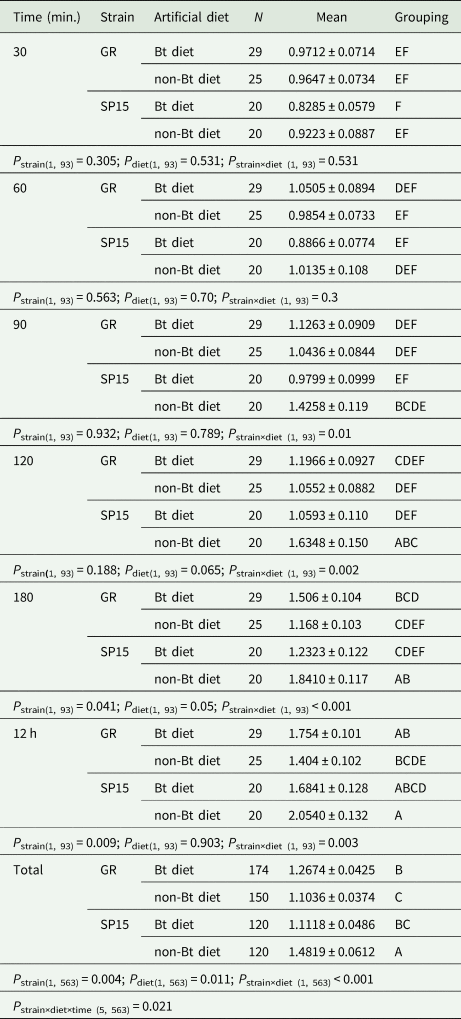
P strains identifies the difference between Bt-resistance vs. susceptible larvae in the percentages of larvae that dropped off a diet. P diet identifies the differences between Bt- and non-Bt diet in the percentage of each larval strain (Bt-resistant or Bt-susceptible larvae) dropped off. P strain×diet identifies the interaction between strains of larvae and diets. P strain×diet×time identifies the interaction among strains of larvae vs. diets and time intervals. Means that do not share a letter are significantly different.
Two-way ANOVA analyses performed on the data from each time period of exposure of larvae to diet treatment showed significant interactions between strain of larvae and diet treatment at the longer time periods of 90, 120, 180 min and 12 h, but not at the shorter exposure times of 30 and 60 min (table 1). The strain of larvae had a significant main effect at exposure times of 180 min and 12 h; however, diet treatment did not have a significant main effect at any period of exposure. Although there were no significant differences across time among Bt-resistant and -susceptible H. armigera larvae in the percentage of larvae leaving dishes, Bt-resistant larvae did consistently drop off Bt-treated diet less often than their susceptible counterparts (fig. 4, left panel). Bt-resistant larvae also reacted differently on non-Bt diet (fig. 4, right panel); after 90 min, they were more likely to leave dishes. Bt-susceptible larvae tended to move less than Bt-resistant larvae with a significantly lower number of Bt-susceptible larvae (14%) dropping off non-Bt diet in comparison to Bt-resistant larvae (23%).
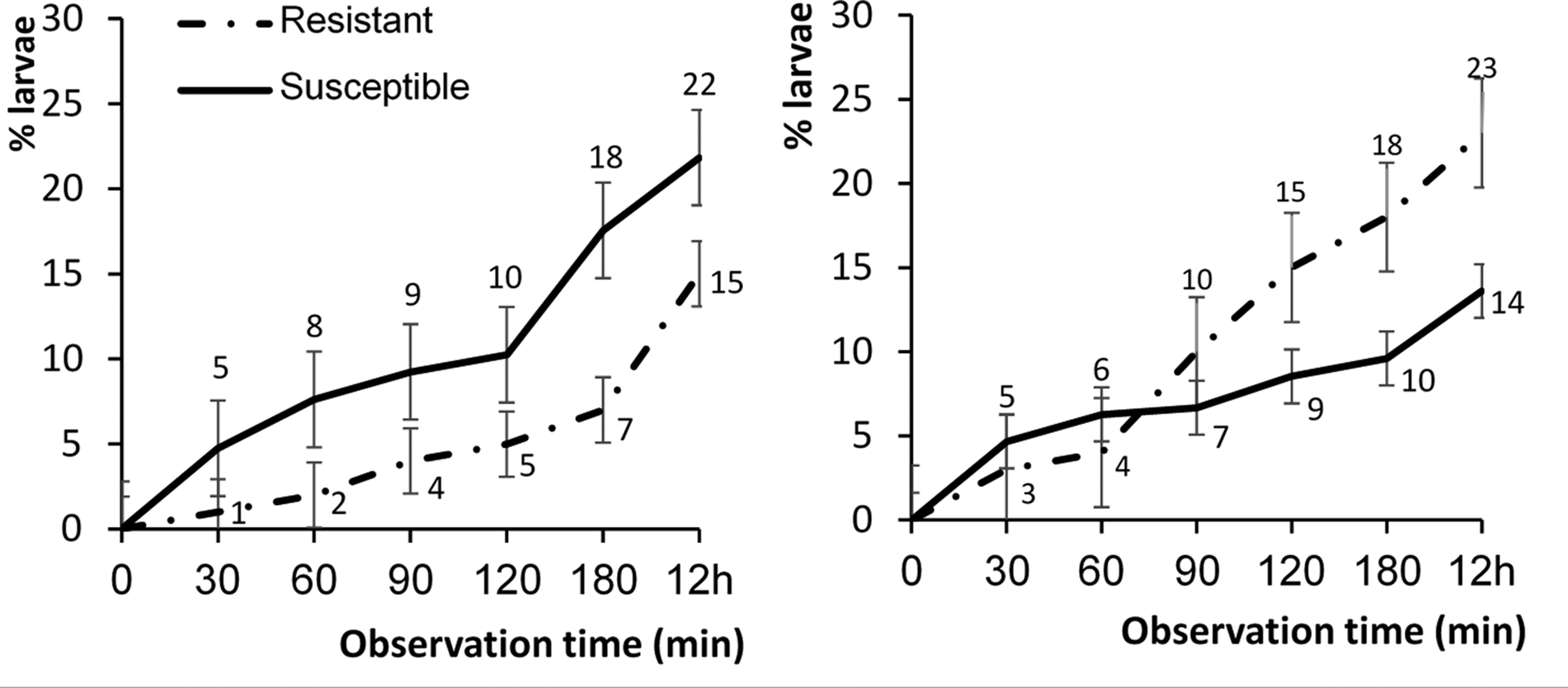
Figure 4. Mean (±SE) percentage of Bt-resistant (n = 40) (dash dot line) and Bt-susceptible (n = 54) (solid line) Helicoverpa armigera neonates that had dropped off Bt-treated artificial diet (left panel) and non-Bt-treated diet (right panel) over a 12 h period. Asterisk indicates a significant difference between percentages within a time interval strains of larvae (comparison based on mean percentages (ANOVA): P < 0.01).
For Bt-resistant larvae in the first 90 min and at 12 h after release, there was no significant difference between Bt and non-Bt diet in the percentage of that dropped off (table 1), but at 120 and 180 min, Bt diet had a significant lower percentage of resistant H. armigera larvae that had left than on non-Bt diet. In contrast, the number of Bt-susceptible larvae that stayed on Bt diet was always lower than that on non-Bt diet after 30 min but not significantly so (table 1).
The survival of Bt-susceptible H. armigera larvae to the next rearing stage
Survival of Bt-susceptible H. armigera larvae to the next stage was high regardless of whether they dropped off (100%) or stayed on non-Bt diet (94%). This contrasts the survival of Bt-susceptible larvae when placed on Bt diets; when they started on Bt diet (small dishes) and stayed survival was 3% which was substantially lower than when they dropped off to the non-Bt diet and survived at 27% (fig. 5).
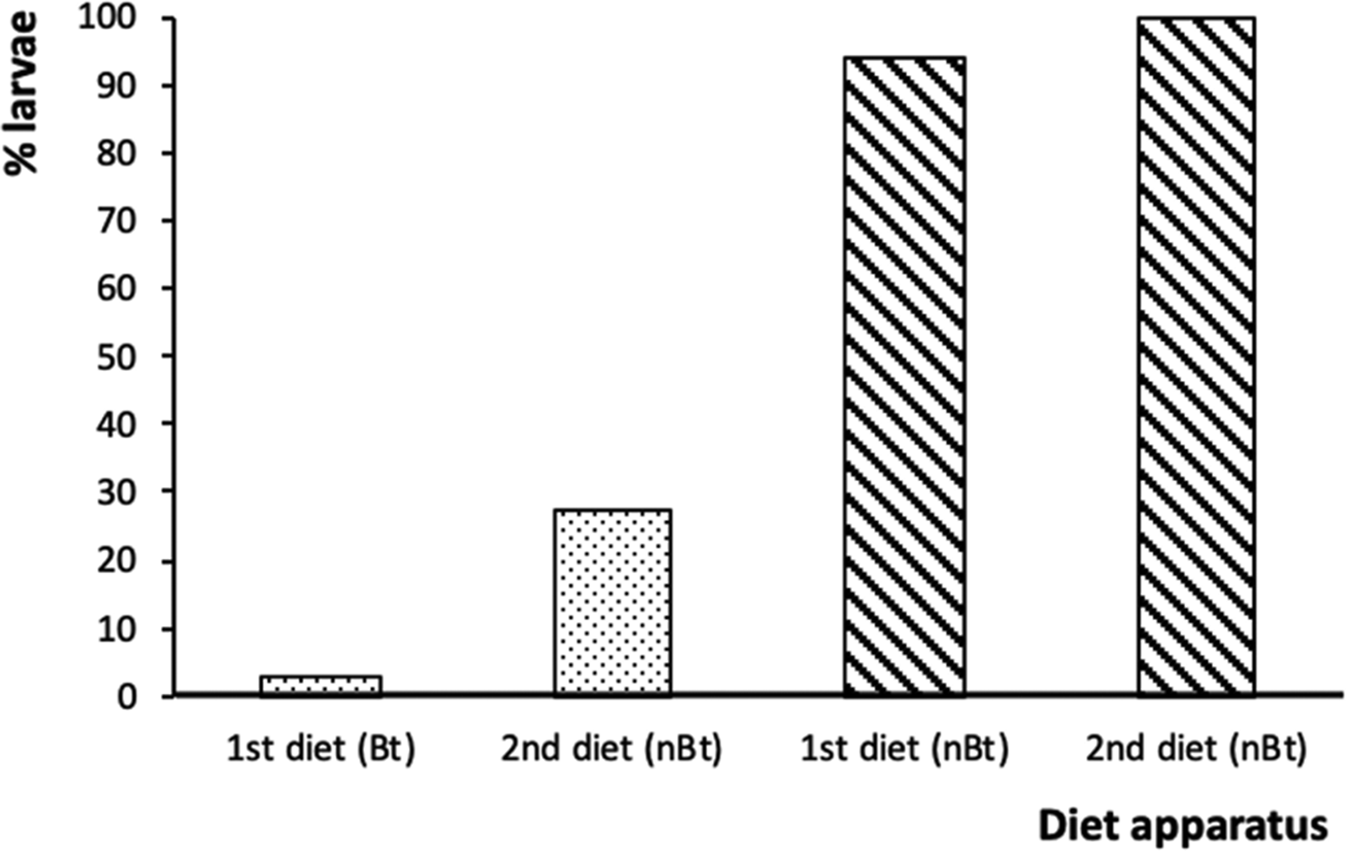
Figure 5. The percentages of surviving Bt-susceptible Helicoverpa armigera neonates from the of Bt diet apparatus (dotted bars) and non-Bt diet apparatus (diagonal stripe bars) that remained (first diet) or dropped off (second diet) at 12 h when reared on non-Bt diet for 3 days.
Experiment 2: drop-off behaviour of Bt-resistant and -susceptible H. armigera neonates on Bt and non-Bt cotton plants
Similar to the behaviour on artificial diet, three-way repeated-measures ANOVA analysis showed that the three-way interaction among strain of larvae, cotton type and time interval was significant (F (3,351) = 2.62, P = 0.05; table 2). There was also significant two-way interactions between larval strain and the cotton type that H. armigera larvae were exposed to (F (1,351) = 22.01, P < 0.01). There was no significant interaction between larval behaviour on the different cotton type and the time exposed to diet (F (3,351) = 1.07, P = 0.363). All three factors showed significant main effects: Bt-resistant and -susceptible strains differed in the percentages of larvae that dropped off (F 1,351 = 6.69, P = 0.01), cotton type affected drop-off behaviour of larvae (F 1,351 = 14.51, P < 0.001), and time exposed to the cotton plant also affected drop-off of larvae (F 3,351 = 102.56, P < 0.001).
Table 2. Mean percentage of Bt-resistant and Bt-susceptible Helicoverpa armigera larvae that dropped off Bt and non-Bt cotton plants at 1–6 h intervals
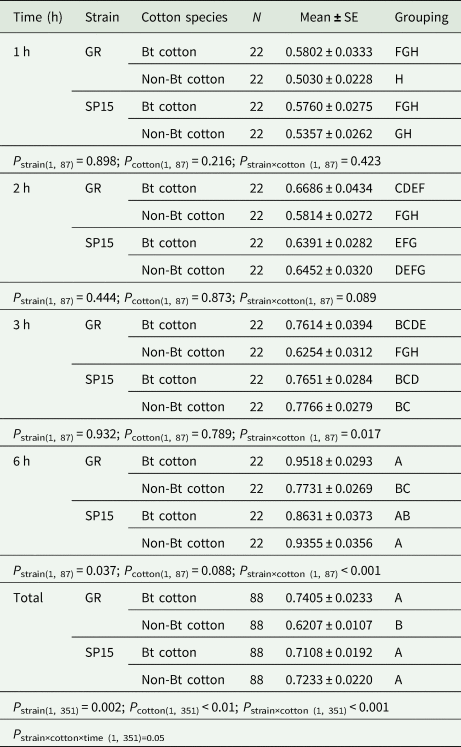
P strains identifies the difference between Bt-resistance vs. susceptible larvae in the percentages of larvae that dropped off a cotton plant. P diet identifies the differences between Bt- and non-Bt cotton plants in the percentage of each larval strain (Bt-resistant or Bt-susceptible larvae) dropped off. P strain×cotton identifies the interaction between strains of larvae and cotton species. P strain×diet×time identifies the interaction among strains of larvae vs. cotton species and time intervals. Means that do not share a letter are significantly different.
Two-way ANOVA analyses performed on the data from each time period of exposure of larvae to cotton plant showed significant interactions between strain of larvae and cotton type at 3 h (F (1,87) = 5.95, P = 0.017; table 2) and 6 h (F (1,87) = 17.94, P = 0.000; table 2), but not at the shorter exposure times of 1 h (F (1,87) = 0.65, P = 0.423; table 2) and 2 h (F (1,87) = 2.96, P = 0.089; table 2). The strain of larvae had a significant main effect at exposure times of 6 h (F (1,87) = 4.48, P = 0.004); however, cotton type did not have a significant main effect at any period of exposure (table 2).
Bt-resistant and -susceptible larvae behaved differently on Bt and conventional cotton plants (F 1,351 = 9.52, P < 0.01). For the Bt-resistant strain, there were no significant differences in the percentages of larvae that dropped off Bt vs. conventional cotton plants during experiment (table 2). At 6 h, nearly the same numbers of Bt-resistant larvae had dropped off Bt and non-Bt cotton plants (51 and 57%, respectively) (fig. 6). However, the number of Bt-susceptible larvae that dropped off Bt cotton plants (46 and 66%) was significantly higher than that on conventional cotton plants (36 and 52%) at 3 and 6 h, respectively (fig. 6).
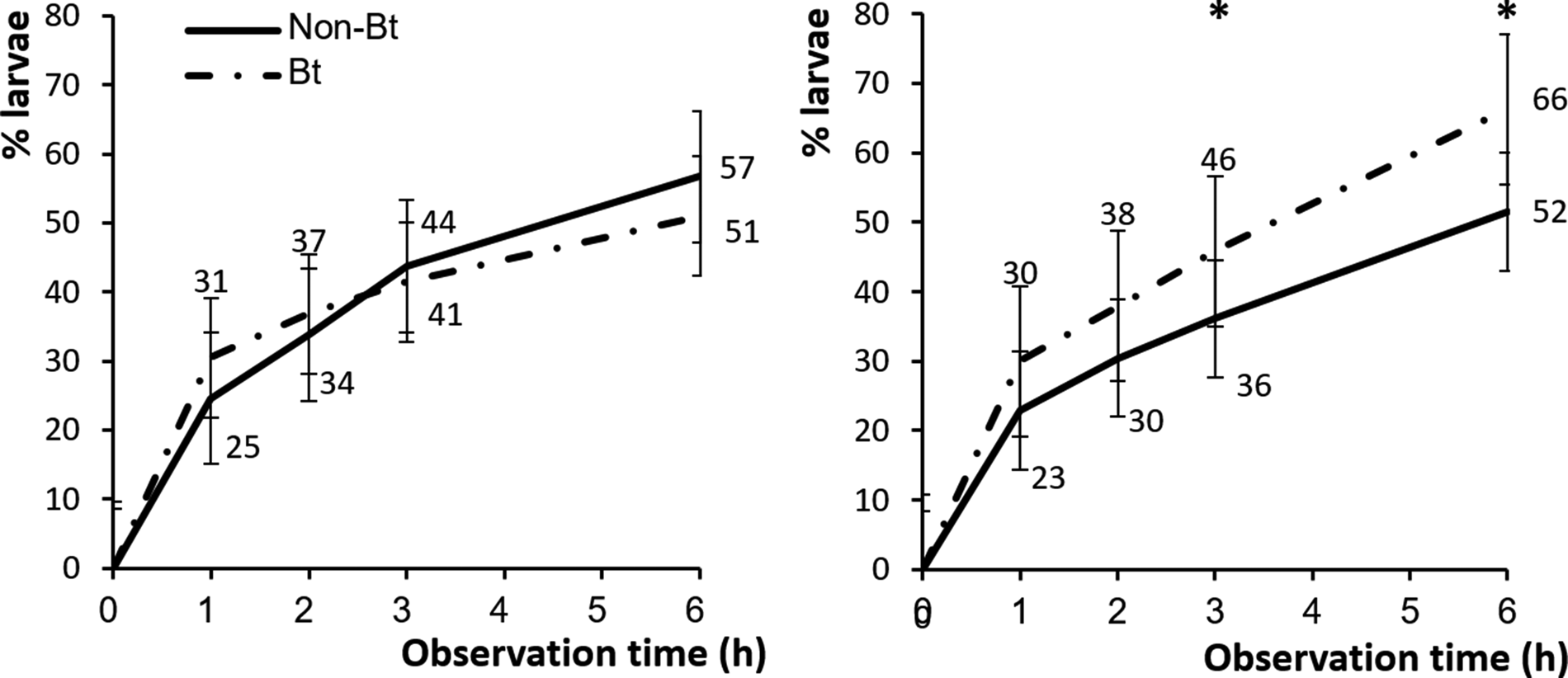
Figure 6. Mean (±SE) percentage of Bt-resistant (n = 22) (left) and Bt-susceptible (n = 22) (right) H. armigera larvae that had dropped off Bt cotton (dash dot line) and non-Bt cotton plants (solid line) after 1, 2, 3 and 6 h. Asterisk indicates a significant difference between mean percentages within a time interval at P < 0.01 (ANOVA).
Discussion
Behaviour is often invoked as a mechanism of insecticide resistance but is difficult to demonstrate (Zalucki and Furlong, Reference Zalucki and Furlong2017). Drop-off behaviour may help H. armigera neonates leave a toxic area enabling them to survive on a diet of Bt and could form the basis of behavioural resistance. In this study, Bt-susceptible larvae showed a significant difference in drop-off tendencies between Bt and non-Bt diet 12 h post release. Most Bt-susceptible larvae had likely fed on and then left Bt diet. Wang et al. (Reference Wang, Furlong, Walsh and Zalucki2019) presented evidence that diet had a clear influence on H. armigera caterpillar movement. Luong et al. (Reference Luong, Zalucki, Cribb, Perkins and Downes2018) found that H. armigera larvae were more likely to leave Bt-containing diets although they could not initially discriminate between diets with and without Bt-toxin in a choice test. Similarly, in the current experiment, a high percentage of larvae left the Bt diet and this increased over time suggesting a post-ingestion effect. The survival of Bt-susceptible larvae that dropped off Bt diet was much higher in comparison to the survival of larvae that stayed on the Bt diet. Not surprisingly, Bt-susceptible larvae survive better if they move to a non-toxic environment after leaving Bt substrates (Luong et al., Reference Luong, Zalucki, Cribb, Perkins and Downes2018). On artificial substrates, Bt-resistant larvae were more likely to stay on Bt diet than on non-Bt diet. The percentages of Bt-resistant larvae that dropped off non-Bt diet were significantly higher than those on Bt diets which was contra to our expectation that their behaviour would be similar. It is possible that the Bt-resistant larvae have a fitness advantage in Bt-toxin environments and therefore are less likely to move away.
Not surprisingly, larvae were much more likely to leave cotton (e.g. after 3 h overall 40–50% movement from plants compared to 5–15% on diet); however, the two strains of larvae reacted differently in terms of drop-off behaviour between artificial diet and cotton plants. While in both experimental set ups, Bt-susceptible larvae were more likely to leave Bt-containing substrates, for Bt-resistance larvae the reluctance to move from Bt diet substrates did not translate to a significant difference in the percentages that dropped off Bt and non-Bt cotton plants. It is unlikely that Bt-resistant strains did not discriminate the presence of Bt-toxins within Bt cotton and non-Bt cotton plants (see below). An important difference between the artificial diet experiments and the plants is that Cry1Ac is likely to also have been expressed to low levels in the latter. The Bt-resistant larvae can survive on Cry2Ab toxin but are susceptible to Cry1Ac. It is therefore possible that the Bt-resistant larvae were more likely to move from the Bt toxins within plants than on artificial diet as the cost to them staying on plants was greater.
Several studies indicate that the presence of Bt toxin in the cotton can significantly influence the intra- and inter-plant movement of heliothine larvae. Our own studies show that significantly greater numbers of Bt-susceptible larvae drop off Bt cotton than non-Bt cotton plants. These results for Bt-susceptible H. armigera neonates were similar to those for other Lepidoptera observed in previous studies. For example, Bt proteins in plants elicited avoidance behaviour by larvae of the light brown apple moth, Epiphyas postvittana (Walker) (Lepidoptera: Tortricidae) (Harris et al., Reference Harris, Mafileo and Dhana1997) and gypsy moth, Lymantria dispar (Linnaeus) (Lepidoptera: Erebidae) (Yendol et al., Reference Yendol, Hamlen and Rosario1975). Heliothis spp. larvae exhibited different dispersal patterns on Bollgard® cotton than on non-Bollgard cotton (Benedict et al., Reference Benedict, Altman, Umbeck and Ring1992, Reference Benedict, Sachs, Altman, Ring, Stone and Sims1993; Parker and Luttrell, Reference Parker and Luttrell1999). In those studies, higher numbers of larvae left Bollgard® cotton than conventional cotton. In a laboratory bioassay, Gould and Anderson (Reference Gould and Anderson1991), Jyoti et al. (Reference Jyoti, Young, Johnson and McNew1996) and Greenplate et al. (Reference Greenplate, Head, Penn and Kabuye1998) found that larvae had an ability to detect and avoid Bt toxin. Gore et al. (Reference Gore, Leonard, Church and Cook2002) found that H. zea larvae began moving away from Bollgard® cotton terminals within 1 h of exposure; within 6 h, <10% of larvae remained in Bollgard® terminals. This finding was similar to the present study in that the percentages of Bt-susceptible larvae that left Bt cotton plants was 39% at 1 h and 66% of larvae at 6 h. Overall, the percentage of larvae that dropped off from whole plants was much higher than from artificial diet. The difference might be due to the more nutritionally balanced composition of artificial diet. Artificial diets are usually complete foods designed for high insect performance and usually considered to be better than natural diets (Katsikis et al., Reference Katsikis, Wang and Zalucki2020). Additionally, whole plants have stimuli that could cause neonate larvae to leave them (Zalucki et al., Reference Zalucki, Clarke and Malcolm2002; Perkins et al., Reference Perkins, Cribb, Brewer, Hanan, Grant, de Torres and Zalucki2013).
The presence of the toxin in Bt cotton and its probable detection by H. armigera neonates post-ingestion is likely to increase the probability of dispersal from the plant after the larvae first feeds. Luong et al. (Reference Luong, Zalucki, Cribb, Perkins and Downes2019) indicated that both strains of larvae showed consistent trends in drop-off behaviour. Fewer larvae remained on young leaves and mature leaves, while more larvae stayed on flowers and squares. Specifically, there was a significant difference between Bt-resistant and -susceptible larvae in the numbers of larvae that stayed on cotton flowers at 1 h of exposure, and significantly more Bt-susceptible larvae were found on squares (both Bt and non-Bt cotton) at 3 h of exposure compared to Bt-resistant larvae (Luong et al., Reference Luong, Zalucki, Cribb, Perkins and Downes2019). These findings were similar to those of Yang et al. (Reference Yang, Johnson and Zalucki2008) and Lu et al. (Reference Lu, Downes, Wilson, Gregg, Knight, Kauter and McCorkell2011) who found significantly more Bt-susceptible larvae on flowers and squares of cotton plants than other structures (leaves, bolls) at 24 and 48 h. This result can be explained by that likelihood that certain parts of flower might express lower Bt toxin levels than leaves (Shahid et al., Reference Shahid, Muhammad, Muhammad, Misbah, Zia, Saghir and Abid2021). As a consequence, larval survival might be higher. Furthermore, the higher nutritional value of flowers could be another possible explanation for the higher survival of larvae on flowers (Eisikowitch and Loper, Reference Eisikowitch and Loper1984) and could allow larvae to overcome the effect of Bt toxin.
Perović et al. (Reference Perović, Johnson, Scholz and Zalucki2008) demonstrated that H. armigera neonates that dropped to the ground within 5 cm from the plant could re-establish on a plant, which in row crops would be common. Larvae were able to survive off the plant for at least 1 h, travel up to 80 cm from where they dropped to the ground, and they navigated through deep cracks in the soil (Terry et al., Reference Terry, Bradley and Duyn1989). Luong et al. (Reference Luong, Zalucki, Cribb, Perkins and Downes2018) showed that larvae could recover well on artificial diet in a laboratory environment after starving for 48 h. In addition, the higher survival of H. armigera Bt-susceptible larvae on Bt cotton flowers than other structures (Luong et al., Reference Luong, Zalucki, Cribb, Perkins and Downes2016) suggested these structures could support first instars to grow to latter stages. Komarlingam (Reference Komarlingam2020) reported that larvae which ingest Bt toxins feed less but move significantly more on Bt-cotton plants and avoid further feeding, and many migrate down the plant to soil after exhibiting drop-off behaviour. Latter instars have a higher tolerance for Bt and could survive better on other structures with higher Bt toxin levels.
Conclusions
The results of our bioassays showed that Bt-susceptible H. armigera neonates were more likely to drop off Bt cotton than non-Bt cotton, while Bt-resistant H. armigera neonates showed similar trends in drop-off on both Bt and non-Bt cotton plants. The difference in the percentages of larvae that dropped off between Bt and non-Bt cotton plants could partly explain how Bt-susceptible larvae survived on Bt cotton plants. However, the differences in methodology, plant age, plant variety or growing conditions, etc., could result in different expression of Bt toxin among plant parts (Lu et al., Reference Lu, Downes, Wilson, Gregg, Knight, Kauter and McCorkell2011). Further experiments in the field should examine the ability of larvae to recover after dropping off Bt cotton plants. These findings may help to understand how Bt-susceptible larvae can establish on Bt cotton.
Acknowledgements
Support for this project was provided by MOET (Vietnamese Government Scholarship), The University of Queensland, CSIRO, and The Cotton Research and Development Corporation.
Conflict of interest
None.