- CYP3A4
cytochrome P450 A4
- MAOI
monoamine oxidase inhibitors
A drug–nutrient interaction is considered to be one which results from a physical, chemical, physiological or pathophysiological relationship between a drug and a nutrient present in a food (including an enteral or parenteral feed) or a supplement(Reference Roe1). Drugs and nutrients share several characteristics, including similar sites of absorption in the intestine, the ability to alter physiological processes and the capacity to cause toxicity in high doses(Reference Thomas2). It is not therefore surprising that drugs can interact with nutrients in several ways. Drugs can potentially influence the bioavailability of nutrients via effects on appetite, absorption, gastrointestinal motility, hepatic metabolism and urinary excretion, while drug absorption and metabolism can sometimes be influenced by nutrients and food supplements(Reference Boullata, Barber, Boullata and Armenti3).
Clinical importance
The potential for interactions may appear to be infinite, and it is unclear what proportion of the total has been identified. More importantly, it is also unclear how many of the identified drug–nutrient interactions are clinically relevant(Reference Santos and Boullata4). A drug–nutrient interaction is considered clinically significant if therapeutic response is altered (reduced or enhanced). Such interactions may result in partial or total failure of drug therapy, although the latter is quite rare(Reference Genser5). Interactions can also cause adverse drug events (e.g. monoamine oxidase inhibitors (MAOI) and certain types of cheese). Such effects can result in the patient discontinuing the drug therapy(Reference Roe1).
Many drug–nutrient interactions, however, are quite harmless, since most drugs are designed to produce blood levels well above those required for therapeutic efficacy. So if a nutrient or food supplement reduces the blood level of a drug, this may not prejudice its clinical effects. Drugs with a narrow therapeutic range (e.g. lithium, phenytoin and theophylline) and those drugs where dosage and blood levels require careful control (e.g. anticoagulants) are those in which drug–nutrient interactions are likely to be the most clinically significant(Reference Boullata, Barber, Boullata and Armenti3, Reference Leibovitch, Deamer and Sanderson6).
Patients at risk
The effect of interactions differs from one patient to another with some groups of patients at particular risk(Reference Thomas2). Infants and children are at particular risk because of the relative inefficiency of the gastrointestinal and hepatic drug metabolising enzymes and poorly developed renal function. Patients on multiple or long-term therapy, who are in an increasing number, are more at risk than patients on short single courses of drugs. Risk of interactions is also increased in patients who are already malnourished because of poor diet and in those with diseases that may lead to nutrient deficiencies (e.g. celiac disease and cystic fibrosis). The risk is also greater in those with increased nutritional requirements (e.g. those with cancer or severe burns).
Mechanistically, there are several types of drug–nutrient interactions. Pharmacokinetic interactions in which a drug can influence the blood concentration of a nutrient or vice versa, often through effects on gastrointestinal absorption, would include the two-way interaction between certain tetracyclines and Ca, and levothyroxine and Ca(Reference Mazokopakis, Giannakopoulos and Starakis7, Reference Singh, Singh and Hershman8). Pharmacodynamic interactions (e.g. vitamin B6 and levodopa, folic acid and phenytoin) are those where the interaction influences drug or nutrient action on body systems, often through an effect on enzymes and/or drug receptors(Reference Boullata, Barber, Boullata and Armenti3). Another type of interaction is where the drug and nutrient or supplement has similar mechanisms of action (e.g. fish oils and anticoagulants). Many interactions are disadvantageous to the patient, but some are not. Emerging evidence suggests, for example, that antibiotic-associated diarrhoea can be treated with some specific probiotics(Reference McFarland9).
Nutrient intake
Drugs may influence nutrient intake by causing gastrointestinal disturbances or by altering appetite and taste. An enormous number of drugs are associated with nausea and vomiting as a side effect, which can affect desire to eat(Reference Martin10).
Many drugs also lead to changes in appetite(Reference Martin10, Reference Thomas11). Drugs that reduce appetite, such as amantadine, digoxin, fluoxetine, levodopa, lithium, metformin and penicillamine may result in poor nutrition and weight loss, while drugs that increase appetite, such as cyproheptadine, MAOI, tricylic antidepressants and valproate, may lead to weight gain. When patients complain of poor appetite, a prescribed drug could be one of the causes.
Several drugs may lead to alteration in taste perception (e.g. angiotensin-converting enzyme inhibitors, allopurinol, amiodarone, baclofen, griseofulvin, lithium, metformin, metronidazole, penicillamine and terbinafine)(Reference Doty and Bromley12). Taste is mediated by chemosensory nerves that respond to stimulatory chemicals by direct receptor binding, opening ion channels or second messenger channels using nucleotides or phosphorylated inositol(Reference Roberts, Dvoryanchikov and Roper13, Reference Shimada, Ueda and Ishida14). Drugs disrupting these cellular processes can cause loss or distortion of taste. Change in taste perception can also be caused by dry mouth. Decreased saliva production alters ion concentrations between saliva and plasma, resulting in decreased taste sensation(Reference Matsuo15). Antimuscarinic (anticholinergic) drugs (e.g. antihistamines, tricyclic antidepressants, benzatropine, orphenadrine, oxybutinin, procyclidine, propantheline and trihexyphenidyl hydrochloride) and selegeline cause dry mouth as a side effect(Reference Martin10). Such medication-induced changes can lead to reduced oral intake and weight loss.
Nutrient absorption
Drugs may affect nutrient absorption via several mechanisms. Reduced gastric acid secretion, which can occur as a result of the administration of proton pump inhibitors (e.g. omeprazole) and histamine H2 antagonists (e.g. cimetidine and ranitidine), can influence the secretion of intrinsic factor and the absorption of vitamin B12(Reference Termanini, Gibril and Sutliff16). In relation to omeprazole, the presence of a polymorphic cytochrome P450 (CYP) enzyme, identified as CYP2C19, significantly affected serum vitamin B12 levels in people on long-term therapy with omeprazole, suggesting that in the future patient genotyping may be useful(Reference Sagar, Janczewska and Ljungdahl17, Reference Ruscin, Page and Valuck18). These drugs, and also antacids, affect gastrointestinal pH, and have been thought to lead to poor absorption of Fe(Reference O'Neil-Cutting and Crosby19). Fe is preferentially absorbed in the ferrous form, but if the pH of the intestine increases, the poorly soluble ferric form is precipitated. However, in a study of 109 patients with Zollinger–Ellison syndrome, continuous treatment with omeprazole for 6 years or continuous treatment with any gastric antisecretory drug for 10 years did not cause decreased body Fe stores or Fe deficiency(Reference Stewart, Termanini and Sutliff20). Moreover, an open-label study in 22 patients with Fe deficiency anaemia found that aluminium hydroxide had no significant influence on Fe uptake by the eythrocyte(Reference Potgieter, Potgieter and Venter21).
Gastric emptying and gastrointestinal motility may be altered by drugs, which in turn can influence nutrient absorption. Gastrointestinal motility can be altered by drugs such as laxatives, metoclopramide, opioids and antimuscarinics. Antimuscarinics and opioids reduce motility, whereas laxatives and metoclopramide increase it(Reference Chan, Shils, Shike, Ross, Caballero and Cousins22). A reduction in motility is unlikely to influence nutrient absorption, but an increase in motility may reduce absorption of nutrients(Reference Roe1). Chronic use of stimulant laxatives (e.g. bisacodyl and senna) can lead to depletion of minerals and liquid paraffin causes malabsorption of fat-soluble vitamins(Reference Roe1). Chronic laxative use in older people has also been associated with reduced vitamin B12 status, while use of oat bran as an alternative to laxatives improves vitamin B12 bioavailability(Reference Sturtzel, Dietrich and Wagner23).
Nutrient absorption may also be influenced by drugs that modulate gastrointestinal mucosal enzymes and transporters. Phenytoin, for example, is hypothesised to interfere with intestinal conjugases that split dietary folates, which are in the polyglutamate form, to the absorbable monoglutamate(Reference Hoffbrand and Necheles24). However, findings in relation to this hypothesis have been inconsistent. Studies have shown that phenytoin can reduce the absorption of conjugated folate, whereas there was no decrease in folic acid absorption when phenytoin was administered with the unconjugated form found in multivitamins and fortified foods(Reference Hoffbrand and Necheles24, Reference Rosenberg, Godwin and Streiff25). However, other studies have not confirmed this(Reference Houlihan, Scott and Boyle26, Reference Baugh and Krumdieck27). Phenytoin also acts as a cofactor in the metabolism of folic acid, a less controversial mechanism for the finding that phenytoin reduces folate status(Reference Berg, Boullata and Armenti28).
Gastrointestinal cytochrome P450 A4 (CYP3A4), present in the epithelial intestinal tissues, plays a role in regulating the oral bioavailability of a large number of drugs and nutrients(Reference Chan, Shils, Shike, Ross, Caballero and Cousins22). Functional alteration of CYP3A4, either through induction or through inhibition, can have a profound effect on the amount of nutrients or drug absorbed (i.e. pre-systemic clearance or first-pass metabolism)(Reference Fujita29).
Grapefruit juice is a classic example of a selective intestinal CYP3A4 inhibitor(Reference Girennavar, Jayaprakasha and Patil30). It destroys and deactivates intestinal CYP3A4 enzymes and can increase the bioavailability of some drugs 5-fold(Reference Dahan and Altman31–Reference Bressler33). Examples of interactions involving grapefruit juice are shown in Table 1. The onset of interaction is immediate with the first glass of grapefruit juice(Reference Lundahl, Regardh and Edgar34). The magnitude of enzyme inhibition increases with each glass. Because the interaction involves the destruction of the enzyme, it cannot be corrected by spacing out the doses. On stopping grapefruit juice, increased absorption of the drug is expected to continue for 3–7 d(Reference Lilja, Kivisto and Neuvonen35). There is also evidence that consuming whole grapefruit(Reference Bailey, Dresser and Kreeft36), lime juice(Reference Bailey, Dresser and Bend37) and Seville orange juice(Reference Malhotra, Bailey and Paine38) results in inhibition of the CYP3A4 enzymes and with an impact on the bioavailability of felodipine. In a comparator study with grapefruit juice, citrus-containing soft drinks had no significant impact on ciclosprorin metabolism(Reference Schwarz, Johnston and Bailey39).
Table 1. Grapefruit juice interactions
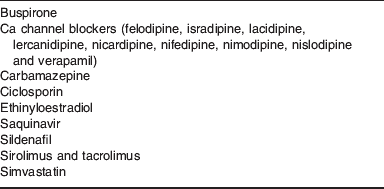
Minerals such as Fe and Zn form insoluble complexes with drugs such as tetracyclines(Reference Andersson, Bratt and Dencker40) and 4-quinolones(Reference Lehto, Kivisto and Neuvonen41, Reference Polk, Healy and Sahai42). This leads not only to poor absorption of the mineral but also to poor absorption of the drug. Penicillamine, a drug used to chelate excess Cu in the treatment of Wilson's disease, also chelates Fe. Fe has been shown to reduce penicillamine absorption by about two-thirds(Reference Osman, Patel and Schuna43, Reference Lyle44). For optimal absorption of penicillamine, Fe should be given at least 2 h after the penicillamine. This should reduce their admixture in the gut(Reference Lyle44). Absorption of fat-soluble vitamins and folic acid can be reduced by the lipid-lowering drugs, colestyramine and colestipol, which bind bile acids(Reference Leonard, Desager and Beckers45). Bleeding tendency associated with vitamin K malabsorption may increase with these drugs and supplements of fat-soluble vitamins may be prescribed if patients are on long-term treatment(Reference Knodel and Talbert46).
Nutrient metabolism
Some drugs have so-called antivitamin effects. These include isoniazid, MAOI, methotrexate, phenytoin and trimethoprim. Isoniazid affects pyridoxine metabolism and may cause peripheral neuropathy, particularly where there are pre-existing risk factors such as diabetes, alcohol dependence, chronic renal failure, malnutrition and HIV infection(Reference Martin10). In these circumstances, pyridoxine (10 mg/d) should be given from the start of treatment(Reference Martin10).
The long-term use of phenytoin and other anticonvulsants can interfere with vitamin D and Ca metabolism and may result in osteomalacia(Reference Kulak, Borba and Bilezikian47). A small number of reports suggest that people taking phenytoin respond poorly to vitamin D replacement(Reference Asherov, Weinberger and Pinkhas48, Reference Rubinger, Korn-Lubetzki and Feldman49) and the dose of vitamin D required to achieve normal plasma 25-hydroxyvitamin D levels in people taking anticonvulsants appears to vary widely(Reference Collins, Maher and Cole50). Observational studies also suggest an association between use of anticonvulsant medication, particularly the older drugs such as phenytoin, phenobarbitone, carbamazepine and valproate, and increased metabolism of vitamin D, osteoporosis and fracture(Reference Lee, Lyles and Colon-Emeric51, Reference Nakken and Tauboll52). All patients taking such medications should have their bone mineral density screened and osteoprotective behaviour such as weight bearing exercise, sunlight exposure, and adequate intakes of Ca and vitamin D should be recommended.
The two-way interaction between phenytoin and folic acid is well known. Phenytoin is a folic acid antagonist, whereas folic acid supplementation can reduce serum phenytoin levels(Reference Berg, Boullata and Armenti28). Lowering of serum folate by phenytoin has ranged from 27 to 91% and has occurred 6–24 months after starting on phenytoin. Doses of folic acid associated with phenytoin lowering have been in the range of 1–30 mg, rather than the 400 μg dose often taken(Reference Berg, Boullata and Armenti28). However, women taking phenytoin during pregnancy or when planning a pregnancy are often prescribed a supplement of 5 mg folic acid daily. To date there is limited information on the influence of the newer anti-epileptic medications on folic acid metabolism.
Oral contraceptives have been reported to influence the metabolism of several vitamins, including vitamin A, vitamin C, vitamin B6 and folic acid(Reference Prasad, Oberleas and Moghissi53). However, most of these studies are now very old and with the advent of lower dose contraceptives, this interaction may not be significant and there is little justification for women on oral contraceptives taking multivitamins.
Interactions between drugs and dietary supplements
The popularity of over-the-counter food supplements has increased during recent decades, but some supplements may interact with certain drugs. Healthcare professionals, including pharmacists, dietitians, nurses and doctors, should always check whether patients are taking medication and supplements at the same time.
Vitamin B6 can reduce or abolish the effects of levodopa(Reference Hsu, Bianchine and Preziosi54–Reference Duvoisin, Yahr and Cote56). Dietary intake need not be adjusted as pyridoxine is required for the transformation of levodopa to dopamine, but increased availability of pyridoxine results in excessive transformation of levodopa outside of the brain and the drug fails to reach its target site of action. This interaction is of limited relevance now as levodopa is mostly prescribed in its combination form (e.g. co-beneldopa or co-careldopa). The inclusion of the dopa decarboxylase inhibitor reduces the wasteful peripheral metabolism of levodopa and much larger amounts are available for entry into the central nervous system. So even in the presence of large amounts of pyridoxine, the peripheral metabolism remains unaffected and the serum levels of levodopa are virtually unaffected(Reference Mars55).
Supplements containing minerals bind several drugs in the gastrointestinal tract with a consequent reduction in the absorption of both the drug and the mineral. Chelation of levodopa by Fe(Reference Greene, Hall and Hider57) can potentially lead to reduced control of Parkinson's disease. Both Fe and Zn form insoluble complexes with several antibiotics, including the tetracyclines(Reference Andersson, Bratt and Dencker40) and some of the 4-quinolones (e.g. ciprofloxacin, norfloxacin and ofloxacin)(Reference Lehto, Kivisto and Neuvonen41, Reference Polk, Healy and Sahai42), and with penicillamine(Reference Osman, Patel and Schuna43, Reference Lyle44). Because drug absorption is often reduced by more than one mineral, it is wise to separate doses of the drug and mineral preparation by at least 2 h. K supplements should be avoided by patients taking angiotensin-converting enzyme inhibitors (e.g. captopril and enalapril), K-sparing diuretics (e.g. amiloride) and ciclosporin because of a risk of severe hyperkalaemia that can be life threatening(Reference Martin10).
Fish oils contain the long-chain n-3 fatty acids, DHA and EPA, which as a result of eicosanoid cascade these particular fatty acids initiate may reduce the coagulability of the blood. This is a potential benefit of fish oil for people at risk of CHD, but many of these patients may also be taking anticoagulants and bleeding tendency may be increased. A case study from Tehran reported that a patient taking warfarin and n-3 fatty acids developed a high international normalised ratio, which returned to normal 2 d after the medications were discontinued. The warfarin was then restarted without the n-3 fatty acids and the international normalised ratio remained within the normal range(Reference Jalili and Dehpour58). A similar finding came from a US case study(Reference Buckley, Goff and Knapp59), but was not replicated in a patient in an earlier study(Reference Bender, Kraynak and Chiquette60). Patients taking warfarin and n-3 preparations should be carefully monitored.
Some non-nutrient supplements may also interact with drugs. Although a herbal supplement, St John's wort is worthy of particular mention because it is widely used. St John's wort is a potent inducer of CYP450 enzymes, including CYP3A4 that can reduce the bioavailability of various drugs(Reference Izzo and Ernst61, Reference Kober, Pohl and Efferth62). Because the CYP3A4 gene is up-regulated, activity can remain high for weeks after stopping St John's wort. Key drugs with which St John's wort can interact are shown in Table 2. St John's wort is also a potent inducer of P-glycoprotein, an intestinal transporter protein(Reference Izzo and Ernst61). Transporter proteins regulate the rate at which substrates (e.g. drugs or nutrients) are presented to the intestinal metabolising enzymes for regulating the absorption of drugs and nutrients. Some transporter proteins regulate efflux of molecules already absorbed back into the intestinal lumen thereby decreasing bioavailability of some substances. Together, P-glycoprotein and CYP3A4 are the most common regulators affecting oral bioavailability of drugs. For example, ciclosporin absorption is limited by P-glycoprotein efflux and pre-hepatic CYP3A4 enzymes. P-glycoprotein not only regulates how much and how fast ciclosporin is presented to CYP3A4 but also transports some of the absorbed ciclosporin back into the intestinal lumen(Reference Staatz, Goodman and Tett63). This provides CYP3A4 with repeated opportunities for metabolism. If this coupled transport metabolism is disrupted (e.g. by grapefruit juice or St John's wort) the absorption of ciclosporin is affected.
Table 2. St John's wort interactions

SSRI, selective serotonin re-uptake inhibitor; ↑, increase; ↓, decrease.
Studies evaluating use of supplements and prescribed medicines
Several studies have reported the concomitant use of supplements and prescribed medicines. A US study in 1539 adults found that 44% were taking prescribed medicines and of these 20% were using herbal or high-dose vitamins(Reference Eisenberg, Davis and Ettner64). A UK study including 164 herbal medicine users found that 59% had taken conventional medicines(Reference Gulian, Barnes and Francis65), while a Canadian study in 195 older patients found that 97% were on prescription medicines and 17% were using natural health products(Reference Dergal, Gold and Laxer66). Studies in cancer patients(Reference Patterson, Neuhouser and Hedderson67) and HIV patients(Reference Risa, Nepon and Justis68) have found that 50–65% use food supplements and/or other complementary medicines. In a US study involving 979 pre-operative patients undergoing anaesthesia, 17·4% reported current use of herbal or dietary supplements(Reference Meyer, Baisden and Roberson69).
Studies evaluating the potential for interactions
Further studies have evaluated the potential for interactions among people taking both supplements and medication. A survey among 458 US patients taking prescription medicines found that 197 (43%) were taking supplements, including vitamins, minerals, ginkgo biloba, garlic, saw palmetto and ginseng. Among these patients, 89 (45%) had potential for one or more interactions, of which 6% were potentially serious(Reference Peng, Glassman and Trilli70).
In a further study among cancer patients, supplements were used by 61% (121 patients) with 65 patients (54%) reportedly taking more than one supplement. Risk for interaction was identified in 12% of patients. However, the patient's medical record documented supplement use in only 28% of patients(Reference Lee, Ingraham and Kopp71).
Supplement use is widespread among cancer patients and longer-term survivors of cancer. In studies combining different cancer sites, 64–81% of survivors reported using any vitamin or mineral supplements and 26–77% reported using any multivitamins. In contrast, approximately 50% of US adults use dietary supplements and 33% use multivitamin/multimineral supplements. Between 14 and 32% of survivors initiate supplement use after diagnosis, and use differs by cancer site. Breast cancer survivors reported the highest use, whereas prostate cancer survivors reported the least. Higher level of education and female sex emerged as factors most consistently associated with supplement use. Up to 68% of physicians are unaware of supplement use among their cancer patients(Reference Velicer and Ulrich72).
Another study evaluated 1795 patients in six specialty clinics. Among these patients, 39·6% (710) reported use of supplements. In total, 107 interactions with potential clinical significance were identified. Five supplements (garlic, valerian, kava, gingko and St John's wort) accounted for 68% of the potentially clinically significant interactions. The four most common classes of prescription medications with a potential for interaction (antithrombotic medications, sedatives, antidepressant agents and antidiabetic agents) accounted for 94% of the potential clinically significant interactions. No patient was harmed seriously from any interaction(Reference Sood, Sood and Brinker73).
In a recent study among 130 older people on the US–Mexico border region, almost half of the older adult participants were at risk for a potential drug–drug interaction, with approximately one-third having a potential interaction between their medications, herbs or nutritional supplements(Reference Loya, Gonzalez-Stuart and Rivera74).
Conclusions
Drugs and nutrients share several pharmacokinetic and pharmacodynamic characteristics and can interact according to a variety of mechanisms. Drugs can affect the bioavailability of nutrients, whereas nutrients and supplements can influence the bioavailability of drugs. The theoretic potential for such interactions is almost infinite, but it is unclear how many are clinically relevant. However, the complexity of many drug regimens is sufficient to suggest that patients on such regimens, including older people and those with long-term chronic conditions, may be at greater risk of drug–nutrient interactions than those on single short courses of therapy. Several recent studies have evaluated the numbers of people taking both supplements and medication and found that high proportions, particularly those with conditions such as cancer, take both types of preparation. The risk of serious interactions has been found in 6–12% of patients in these studies. However, clinically relevant data on all potential drug–nutrient interactions have not so far been explored and further research is sorely needed.
Acknowledgements
The author provides consultancy services to the Health Supplements Information Service and received no sources of funding to produce this paper.