Introduction
The increase in herbicide-resistant weed species is a major threat to food production globally (Oerke Reference Oerke2006). Long-term use of herbicides as the primary form of weed control has selected for individuals capable of evading herbicide application (Gillespie Reference Gillespie2010; Gressel Reference Gressel2009). Currently, 486 monocot and dicot weed species have evolved resistance globally to various herbicide sites of action (Heap Reference Heap2017). Continued reliance on herbicides for weed control has resulted in new resistance cases reported in Australia and globally, including the evolution of metabolic resistance, which is of great concern, as it can confer unpredictable resistance to various sites of action, including herbicides never used (Beckie et al. Reference Beckie, Lozinski, Shirriff and Brenzil2013; Busi et al. Reference Busi, Vila-Aiub, Beckie, Gaines, Goggin, Kaundun, Lacoste, Neve, Nissen, Norsworthy, Renton, Shaner, Tranel, Wright, Yu and Powles2013; Yu and Powles Reference Yu and Powles2014).
Multiple weed species currently challenge Australian grain production systems (Llewellyn et al. Reference Llewellyn, Ronning DaC, Mayfield, Walker and Ouzman2016). The most problematic of these is rigid ryegrass (Lolium rigidum Gaudin), which is a genetically diverse, obligate-outcrossing weed species with widespread adaptations across all major cropping regions of southern Australia; it has evolved resistance to multiple herbicides with different sites of action (Busi et al. Reference Busi, Girotto and Powles2016; Han et al. Reference Han, Yu, Owen, Cawthray and Powles2016; Preston and Powles Reference Preston and Powles2002; Yu and Powles Reference Yu and Powles2014). The evolution of resistance in L. rigidum to selective POST acetyl-CoA carboxylase (ACCase)-inhibiting (diclofop-methyl) (Heap and Knight Reference Heap and Knight1982) and acetolactate synthase (ALS)-inhibiting (chlorsulfuron) (Powles et al. Reference Powles, Holtum, Matthews and Liljegren1990; Preston and Powles Reference Preston and Powles2002) herbicides, including populations with multiple resistance (Preston et al. Reference Preston, Tardif and Powles1996), has shifted reliance to PRE herbicides from the thiocarbamate family. Increased reliance on thiocarbamate herbicides has ensued with the evolution of resistance to the PRE dinitroaniline herbicide trifluralin (3(K1): inhibitors of microtubule assembly). Trifluralin resistance is widely documented in southern Australia (Boutsalis et al. Reference Boutsalis, Gill and Preston2012; Broster and Pratley Reference Broster and Pratley2006). Release of the PRE herbicides prosulfocarb (8(N): inhibitor of fat synthesis) and pyroxasulfone (15(K3): inhibitors of very-long-chain fatty-acid synthesis) provided effective options for the control of L. rigidum in cereal and pulse crops (Boutsalis et al. Reference Boutsalis, Gill and Preston2014; Tanetani et al. Reference Tanetani, Ikeda, Kaku, Shimizu and Matsumoto2013; Walsh et al. Reference Walsh, Fowler, Crowe, Ambe and Powles2011). Thiocarbamate herbicides currently provide one of the two effective options for the control of L. rigidum in cereals. Resistance evolution to thiocarbamate herbicides threatens the sustainability of Australian grain production. Resistance to the thiocarbamates will reduce the effective control strategies in cereals, thereby restricting the control of L. rigidum to one herbicide (pyroxasulfone) (Walsh et al. Reference Walsh, Fowler, Crowe, Ambe and Powles2011).
Only a few examples of weeds with resistance to thiocarbamate herbicides exist, one being triallate-resistant wild oat (Avena fatua L.) in Canada (Beckie et al. Reference Beckie, Hall, Meers, Laslo and Stevenson2004, Reference Beckie, Warwick and Sauder2012; O’Donovan et al. Reference O’Donovan, Sharma, Harker, Maurice, Baig and Blackshaw1994) with confirmed cross-resistance to pyroxasulfone and sulfentrazone (Mangin et al. Reference Mangin, Hall and Beckie2016). Resistance to prosulfocarb has also been documented in blackgrass (Alopecurus myosuroides Huds.) populations across Denmark (Keshtkar et al. Reference Keshtkar, Mathiassen, Moss and Kudsk2015). In addition, evolution of cross-resistance to prosulfocarb and triallate in L. rigidum was achieved by Busi and Powles (Reference Busi and Powles2013) in a glasshouse low-dose recurrently selected population MR4 displaying 58% and 35% survival, respectively. Busi and Powles (Reference Busi and Powles2016) also demonstrated cross-resistance to prosulfocarb + S-metolachlor and pyroxasulfone in L. rigidum recurrently selected with either herbicide.
Understanding the levels of resistance to thiocarbamate herbicides is necessary for the development of management strategies to slow the rate of resistance evolution and manage resistant weeds. The evolution of resistance to thiocarbamate herbicides has not yet been fully studied. The objective of this study was to determine the level of resistance and patterns of cross-resistance and multiple resistance in an L. rigidum population with suspected field-evolved resistance collected from Cockaleechie, Eyre Peninsula, South Australia, and to test its response to other PRE herbicides.
Materials and Methods
Plant Material
The seed of L. rigidum population EP162 (resistant [R]) used in this study was collected from a field in 2014 at Cockaleechie, Eyre Peninsula, South Australia (34.21°S, 135.84°E) with a long history of crop production. Preliminary testing revealed resistance to multiple herbicide sites of action, including ACCase, ALS, microtubule assembly, fat synthesis, and 5-enolpyruvylshikimate-3-phosphate inhibitors. Two well-characterized herbicide-susceptible L. rigidum populations, SLR4 and VLR1 (S), were also used in this study (Boutsalis et al. Reference Boutsalis, Gill and Preston2012).
Seedling Growth and Herbicide Application
Seeds from EP162, SLR4, and VLR1 were weighed (0.2 g=50 to 60 seeds) and spread onto the surface of 9.5 cm by 8.5 cm by 9.5 cm punnet pots (Masrac Plastics, SA, Australia) containing cocoa peat potting mix as described by Boutsalis et al. (Reference Boutsalis, Gill and Preston2012). Herbicides were applied immediately using a laboratory spray cabinet equipped with flat-fan nozzles (Hardi ISO F-110-01, Hardi, Adelaide, SA, Australia) delivering 118 L ha−1 water at a pressure of 4 kPa. Control pots were not treated with any herbicide. The experiment was conducted and repeated outdoors under natural growing conditions in winter during the normal growing season (July to August 2017). Pots were watered as needed to maintain potting mix near field capacity. There were three replicates for each herbicide dose, and pots were arranged in a randomized complete block design.
Dose–Response Experiment
Seven PRE herbicides were applied (Table 1) following the method described by Boutsalis et al. (Reference Boutsalis, Gill and Preston2012). The PRE herbicide triallate was applied to S biotypes at 25, 50, 100, 200, 400, 800, and 1,600 g ha−1 and to the R biotype at 400, 800, 1,600, 3,200, 6,400, and 12,800 g ha−1; in Australia, the recommended label rate of triallate for L. rigidum control is 1,500 g ha−1. Prosulfocarb was applied to S biotypes at 37.5, 75, 150, 300, 600, 1,200, and 2,400 g ha−1 and to the R biotype at 600, 1,200, 2,400, 4,800, 9,600, and 19,600 g ha−1, with the recommended label rate for L. rigidum control being 2,400 g ha−1. EPTC was applied to S biotypes at 67.5, 135, 270, 540, 1,080, 2,160, and 4,320 g ha−1 and to the R biotype at 1,080, 2,160, 4,320, 8,640, 17,280, and 34,560 g ha−1, with the recommended label rate for L. rigidum control being 4,320 g ha−1. Thiobencarb is not a registered herbicide for the control of L. rigidum in Australia but was included as another thiocarbamate herbicide and applied to S biotypes at 56.25, 112.5, 225, 450, 900, 1,800, and 3,600 g ha−1 and to the R biotype at 900, 1,800, 3,600, 7,200, 14,400, and 28,800 g ha−1.
Table 1 Active ingredients, formulations, and manufacturers of herbicides used in dose–response experiments.

Additional PRE herbicides with different sites of action were included, as these provide an alternative option for the control of L. rigidum in Australia. Trifluralin was applied to S biotypes at 7.81, 15.62, 31.25, 62.5, 125, 250, and 500 g ha−1 and to the R biotype at 125, 250, 500, 1,000, 2,000, and 4,000 g ha−1, with the recommended label rate for L. rigidum control being 500 g ha−1. Propyzamide was applied to S biotypes at 7.81, 15.62, 31.25, 62.5, 125, 250, and 500 g ha−1 and to the R biotype at 125, 250, 500, 1,000, 2,000, and 4,000 g ha−1, with the recommended label rate for L. rigidum control being 500 g ha−1. Pyroxasulfone was applied to S biotypes at 1.6, 3.2, 6.4, 12.8, 25.5, 51, and 102 g ha−1 and to the R biotype at 25.5, 51, 102, 204, 408, and 816 g ha−1, with the recommended label rate for L. rigidum control being 100 g ha−1. Pots were assessed at 28 d after herbicide treatment, and plants that had emerged and grown to the 2-leaf stage were counted. Emergence was assessed as the number of plants germinating in the treated pots compared with the average number germinating in control pots and calculated as a percentage.
Statistical Analysis
Following an ANOVA, both experiment runs were pooled (no statistical difference between experimental runs) and analyzed using a log-logistic equation (GraphPad Prism v. 7.0, GraphPad Software, San Diego, CA) fit to the survival data. LD50 (dose required to reduce emergence by 50% of individuals in the population) parameter estimates from the log-logistic analysis were used to calculate the resistance index (RI), which is the resistant/susceptible ratio of the LD50. The model fit was

where y represents plant survival (%) or biomass reduction (%), x is the log-dose of the herbicide used, IC50 is the herbicide dose required to cause 50% reduction in plant emergence (LD50), and b denotes the slope of the curve.
Results and Discussion
Plants of the S populations SLR4 and VLR1 were completely controlled at 1,500 g ha−1 triallate, the recommended field rate, while the resistant population EP162 showed no mortality at this rate (Figure 1A). The triallate rates required for 50% mortality (LD50) for the SLR4 and VLR1 populations were 248 g ha−1 and 181 g ha−1, respectively (Table 2). The LD50 for the resistant population EP162 was 3,188 g ha−1, giving an RI 14.9-fold higher than the mean of both S populations. Prosulfocarb at the recommended rate of 2,400 g ha−1 controlled both S populations, while EP162 had 48% mortality at this rate (Figure 1B). The LD50 rates for SLR4 and VLR1 were 311 g ha−1 and 246 g ha−1, respectively, and 2,608 g ha−1 for EP162 (9.4-hold higher than the S populations). EPTC completely controlled both S populations at the recommended rate of 4,320 g ha−1, while the EP162 population showed 68% mortality at this rate (Figure 1C). The LD50 rates for the SLR4 and VLR1 populations were 305 g ha−1 and 288 g ha−1, while the LD50 for the EP162 population was 2,867 g ha−1 (9.7-fold higher than the S populations). Even though thiobencarb is not registered for L. rigidum control in Australia, it completely controlled both S populations at 3,600 g ha−1 (Figure 1D). The LD50 rates for thiobencarb for the SLR4 and VLR1 populations were 370 g ha−1 and 265 g ha−1, respectively, and 4,332 g ha−1 for the EP162 population (13.6-fold higher than the S populations).
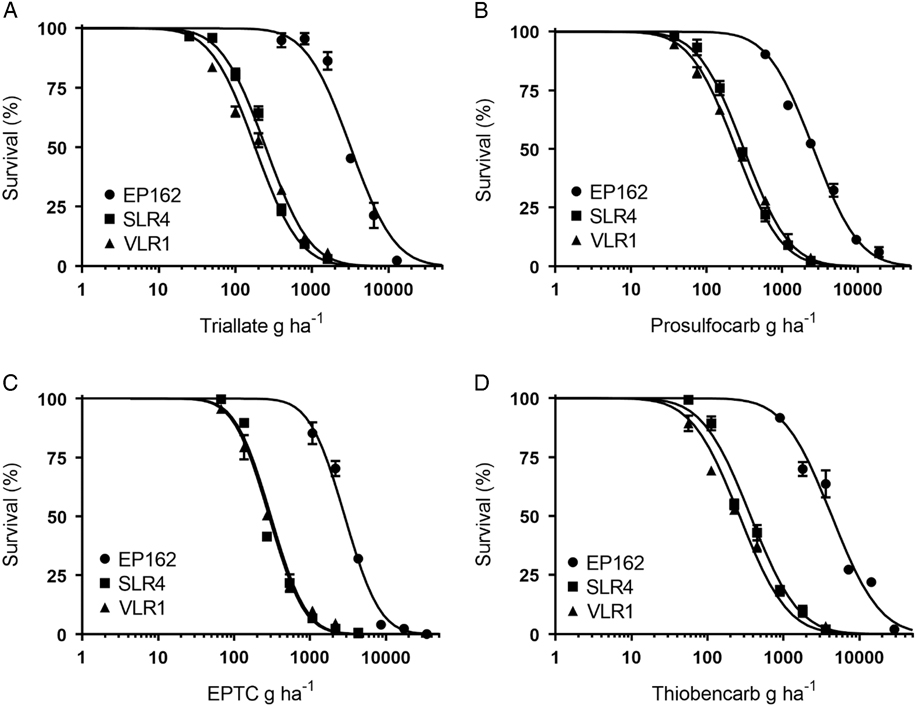
Figure 1 Response of EP162, SLR4, and VLR1 populations of Lolium rigidum treated with varying rates of triallate (A), prosulfocarb (B), EPTC (C), and thiobencarb (D). Each data point is the mean of six replicates, and bars represent standard error of the mean.
Table 2 Pooled dose–response data of triallate, prosulfocarb, EPTC, thiobencarb, trifluralin, propyzamide, and pyroxasulfone required for 50% mortality (LD50) of resistant and susceptible Lolium rigidum populations with 95% confidence intervals (in parentheses) and resistance index (RI).

a RI as compared with the standard susceptible populations (SLR4 and VLR1).
b Probability value indicates significant differences in LD50 values between L. rigidum populations treated with a particular herbicide.
*P<0.01.
Trifluralin completely controlled the SLR4 and VLR1 populations at the recommended rate of 500 g ha−1, with the EP162 population recording 45% mortality at this rate (Figure 2A). The LD50 rates for SLR4 and VLR1 were 39 g ha−1 and 27 g ha−1, respectively, and 455 g ha−1 for EP162 (13.8-fold higher than the S populations) (Table 2). Propyzamide completely controlled both the S and R populations at the recommended rate of 500 g ha−1 (Figure 2B). The LD50 rates for the SLR4 and VLR1 populations were 30 g ha−1 and 23 g ha−1, respectively, and 74 g ha−1 for the EP162 population (2.7-fold greater than the S populations). Pyroxasulfone completely controlled both S populations at the recommended rate of 100 g ha−1. The LD50 rates for the SLR4 and VLR1 populations were 9.5 g ha−1 and 6.2 g ha−1, respectively. The EP162 population displayed 28% survival to pyroxasulfone at the recommended rate (Figure 2C) and an LD50 of 64 g ha−1 (8.1-fold greater than the S populations).

Figure 2 Response of EP162, SLR4, and VLR1 populations of Lolium rigidum treated with varying rates of trifluralin (A), propyzamide (B), and pyroxasulfone (C). Each data point is the mean of six replicates, and bars represent standard error of the mean.
The EP162 population of L. rigidum showed cross-resistance and a similar RI to the thiocarbamate herbicides triallate, prosulfocarb, EPTC, and thiobencarb (Table 2). This suggests a similar resistance mechanism may be providing resistance to the thiocarbamate herbicides in this population. Resistance to triallate in A. fatua has previously been reported by Kern et al. (Reference Kern, Peterson, Miller, Colliver and Dyer1996) to occur through reduced herbicide activation (sulfoxidation), and such a mechanism may provide cross-resistance to other thiocarbamate herbicides. However, reduced activation does not explain resistance in this population to other PRE herbicides, such as trifluralin, that do not require sulfoxidation for activation (Kern et al. Reference Kern, Peterson, Miller, Colliver and Dyer1996). The EP162 population shows a response profile more similar to an A. myosuroides population from Denmark as reported by Keshtkar et al. (Reference Keshtkar, Mathiassen, Moss and Kudsk2015), which has a non–target site resistance mechanism conferring strong resistance to the herbicides prosulfocarb and pendimethalin.
The level of resistance to prosulfocarb observed in this field-selected population was similar to a recurrently selected L. rigidum population of Busi and Powles (Reference Busi and Powles2013). The RIs for triallate and thiobencarb were slightly higher than for prosulfocarb and EPTC, which could be related to the differences in water solubility and binding capacity among the thiocarbamate herbicides tested (Mangin et al. Reference Mangin, Hall, Schoenau and Beckie2017; Westra et al. Reference Westra, Shaner, Westra and Chapman*2014). The EP162 population displayed a level of trifluralin resistance similar to a recent report of 17-fold resistance conferred through a target-site point mutation in an L. rigidum population SLR74 (Fleet et al. Reference Fleet, Malone, Preston and Gill2017) and consistent with reported resistance in field-collected populations from across South Australia (Boutsalis et al. Reference Boutsalis, Gill and Preston2012). This observed level of resistance to other herbicide sites of action may be the result of enhanced herbicide metabolism via cytochrome P450 enzymes (Busi et al. Reference Busi, Gaines and Powles2017a) or by glutathione S -transferase conjugation with pyroxasulfone as reported by Busi et al. (Reference Busi, Porri, Gaines and Powles2017b) in L. rigidum.
Dose–response experiments also identified resistance to pyroxasulfone. Despite this, pyroxasulfone still provided a considerable level of control (72%) at the recommended field rate of 100 g ha−1 (Figure 2C). In contrast, the resistance observed to propyzamide (3(K1): inhibitors of microtubule assembly) was much lower than pyroxasulfone. This herbicide still provided effective control of the population at the recommended rate. Even though propyzamide has the same site of action as trifluralin, propyzamide inhibits polymerization of tubulin into microtubules via a different mechanism compared with trifluralin as reported by Hoffman and Vaughn (Reference Hoffman and Vaughn1994). These differences in resistance can be explained by the repeated use of trifluralin leading to higher levels of resistance compared which propyzamide, which has been used primarily in canola (Brassica napus L.) after being registered in Australia in 2014. Variations in resistance within a site of action are known; different target-site mutations can result in different patterns of resistance to herbicides within a site of action (Preston Reference Preston2017). In addition, herbicides belonging to the same site of action but to different chemical families will bind differently. One herbicide may be unable to bind, leading to resistance, while the binding of others may not be affected, leading to greater susceptibility (Oettmeier et al. Reference Oettmeier, Hilp, Draber, Fedtke and Schmidt1991).
This study is the first documented case of field-evolved resistance to thiocarbamate herbicides in a population of L. rigidum. In response to widespread trifluralin resistance, use of the herbicide prosulfocarb has allowed growers to control L. rigidum in wheat (Triticum aestivum L.) and barley (Hordeum vulgare L.) in Australia. However, resistance evolution to thiocarbamate herbicides will result in increased reliance by growers on alternative herbicides such as pyroxasulfone and propyzamide. Potential resistance evolution to pyroxasulfone is of great concern, as this herbicide currently provides the most effective way of managing multiply resistant L. rigidum populations in wheat. The resistance to pyroxasulfone detected in the EP162 population suggests resistance to this herbicide could occur due to selection with trifluralin and/or prosulfocarb as reported by Busi and Powles (Reference Busi and Powles2016). Control of L. rigidum in canola is currently achieved with propyzamide (3(K1)); however, overreliance on propyzamide also increases the risk of resistance evolution to this herbicide. Evolution of cross-resistance and multiple resistance to PRE herbicides has made L. rigidum management increasingly difficult in Australian cropping systems. Australian growers will need to consider alternative strategies in addition to using propyzamide and pyroxasulfone to effectively manage L. rigidum in the future. These strategies will need to include mixtures of PRE herbicides, increasing crop competition with weeds, and the adoption of strategies to reduce weed seeds returning to fields (Beckie Reference Beckie2006; Walsh and Powles Reference Walsh and Powles2007).
Acknowledgments
The authors acknowledge the Grains Research and Development Corporation for funding this research and Alicia Merriam, Ruwan Lenorage, and GeethaVelappan for technical assistance with herbicide application and assessment. No conflicts of interest have been declared.