Implications
The fact that supplementation with summer turnip and forage rape had no effect on milk production and composition is an advantage for dairy farmers, as it may increase farm profitability. The replacement of grass silage and commercial concentrates with brassicas reduces feeding costs and increases profitability. It also contributes to diversification of the forage base for dairy production in temperate grazing systems.
Introduction
Summer brassicas are used to supply feed in times of seasonal forage/feed shortage resulting from low rainfall and high temperatures, which reduce pasture quality and growth. The main summer brassica crops are summer turnips (STs) and forage rape (FR; Barry, Reference Barry2013). They can produce high yields of DM (8 to 15 tons DM/ha) in a relatively short period of time (60 to 120 days), are highly digestible (85 to 90% DM digestibility), have a variable CP content (120 to 200 g/kg DM) and are characterized by NDF contents below 280 g/kg DM (Westwood and Mulcock, Reference Westwood and Mulcock2012). It has been observed that brassicas can improve animal performance in terms of milk production, with a response of 0.8 l of milk per kg of supplemented brassica, and liveweight gain (Moate et al., Reference Moate, Dalley, Roche, Grainger, Hannah and Martin1999) and reduce the environmental impact due to lower methane production (2.7 to 7.4 g/day; Sun et al., Reference Sun, Waghorn, Hoskin, Harrison, Muetzel and Pacheco2012) compared to ruminants grazing grass-based pasture diets.
Generally, ST has greater metabolizable energy (ME) contents than FR (3.0 and 2.8 Mcal/kg DM, respectively), while CP content is greater in FR (160 to 200 g/kg DM) than in ST (110 to 140 g/kg DM, respectively) (Westwood and Mulcock, Reference Westwood and Mulcock2012; Barry, Reference Barry2013). Recently, Keim et al. (Reference Keim, Cabanilla, Balocchi, Pulido and Bertrand2019) concluded that most of the differences that were observed in terms of chemical composition and degradation kinetics between ST and FR did not affect in vitro fermentation products. However, extrapolations of in vitro results to in vivo situations must be done carefully (Yanez-Ruiz et al., Reference Yanez-Ruiz, Bannink, Dijkstra, Kebreab, Morgavi, O’Kiely, Reynolds, Schwarm, Shingfield, Yu and Hristov2016).
Barry (Reference Barry2013) suggested that although brassicas are highly digestible, there are inconsistencies regarding their effects on animal performance. This might be due to detrimental effects of secondary compounds (e.g. S-methyl-cysteine sulphoxide (SMCO), glucosinolates and nitrates) that are present in higher concentrations in brassicas than in other forages. Other factors such as physical limitation of dry matter intake (DMI) due to their high forage water content, inadequate fibre intake for ruminal function and altered fermentation in the rumen may also contribute to the effects on animal performance when these crops are fed (Lambert et al., Reference Lambert, Abrams, Harpster and Jung1987).
The secondary compounds mentioned above can produce nutritional disorders (Keogh et al., Reference Keogh, French, McGrath, Storey and Mulligan2009a), might affect rumen fermentation and may produce subclinical alterations that are implicated in reduced voluntary feed intake and the under-performance of brassica-fed livestock (Barry, Reference Barry2013).
Few experiments have evaluated milk production responses to brassica supplementation (Moate et al., Reference Moate, Dalley, Martin and Grainger1998 and Reference Moate, Dalley, Roche, Grainger, Hannah and Martin1999) and there is limited published literature reporting the effect of brassica forages on rumen physiology in the dairy cow (Barry, Reference Barry2013; Keogh et al., Reference Keogh, French, McGrath, Storey and Mulligan2009a). Most of the experiments have studied ST, which have shown in some cases similar responses to barley supplementation for dairy cows in mid-lactation (Moate et al., Reference Moate, Dalley, Martin and Grainger1998), whereas Moate et al. (Reference Moate, Dalley, Roche, Grainger, Hannah and Martin1999) observed a lower milk production response in cows fed ST compared to concentrate supplementation. Williams et al. (Reference Williams, Moate, Deighton, Hannah, Wales and Jacobs2016) reported increases in milk production from cows supplemented with FR, and a reduction in methane intensity and in acetate : propionate ratio (Ac : Pr) in the rumen. Nevertheless, there is a lack of studies that compare productive responses and rumen metabolism of dairy cows fed different summer brassicas (Barry, Reference Barry2013). Thus, we hypothesize that using summer brassicas (ST and FR) to partially replace grass silage and commercial concentrate maintains milk production and composition of mid-lactation dairy cows, without harming metabolic parameters. The aim of this study was to determine DMI, rumen fermentation and milk production responses of mid-lactation dairy cows supplemented with summer (ST or FR) brassicas.
Material and methods
Animals and experimental design
The study was conducted at the Experimental Research Station of Universidad Austral de Chile, Valdivia, Chile, between January and March 2017.
Twelve pregnant multiparous lactating Holstein Friesian dairy cows (mean ± Standard error: 22.7 ± 0.67 kg milk/day, 534 ± 7.5 kg liveweight and 155 ± 6.6 days in milk) were randomly allocated to three dietary treatments in a replicated 3 × 3 Latin square design. The experiment lasted 63 days and was divided into three 21-day periods each; the first 14 days of each period consisted in adaptation of the animals to the diets and the last 7 days were used for data collection. Seven days before the first experimental period, the cows were housed in tie-stalls with rubber bedding for adaptation to experimental conditions and received a grass silage and concentrate diet.
Brassica crop production and animal feeding
Turnip cv. Barkant and FR cv. Spitfire were sown in October and November 2016 in two adjacent 0.5 ha area at a density of 3 and 4 kg seed/ha, respectively. The crops were sown on two dates with a 20-day interval, in order to offer plant material with a similar stage of maturity throughout the experiment. A fresh allocation of ST was harvested manually daily and soil attached to the roots removed, whereas FR was harvested with a cutter bar mower (Bertolini 140 L, Reggio Emilia, Italy) at 10 cm above ground level daily.
Cows were divided into three groups according to the dietary treatments (control, ST and FR). For the control diet, 16.2 kg DM of grass silage, 2.25 kg DM of commercial concentrate and 2.25 kg DM soybean meal were offered on a daily basis. For the other two dietary treatments, 25% of the DM from silage and concentrates were replaced with FR (leaf : stem ratio: 65 : 35) or ST (leaf : root ratio: 46 : 54). The amount of soybean meal remained constant to keep the three diets isoenergetic and isonitrogenous, which met the ME and protein requirements based on AFRC (1995). Chemical composition of feed ingredients and diets is reported in Table 1. Prior to feeding, all feeds were weighed and offered individually for each cow according to the dietary treatments. Grass silage and soybean meal were offered twice a day at 0800 and 1700 h. Once cows consumed the soybean meal, grass silage was offered. For ST and FR, orts of grass silage were removed at 1100 h and the total allowed amounts of ST or FR were offered at once for a 5 h period. Concentrates were offered twice daily during milkings.
Table 1 Chemical composition of feed ingredients, diets (control, rape and turnip) and proportion of ingredients offered to mid-lactation dairy cows
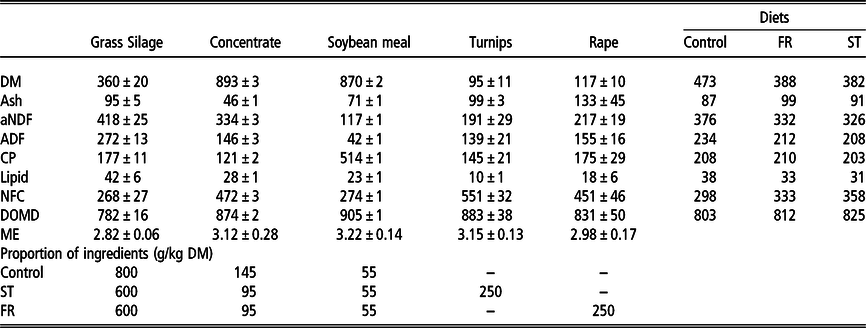
FR = treatment with a 25% of forage rape inclusion in the diet; ST = treatment with a 25% of summer turnip inclusion in the diet; DM = dry matter (g/kg); aNDF = neutral detergent fibre with a heat stable amylase (g/kg DM); ADF = acid detergent fibre (g/kg DM); CP = crude protein (g/kg DM); NFC = non-fibrous carbohydrates (NFC = 1000 – (ash + lipid + CP + NDF) (g/kg DM); DOMD = digestible organic matter on DM basis (g/kg DM); ME = metabolizable energy (Mcal/kg DM).
Feeds and nutrients intake, milk production and composition
Feed offered and feed refusals were recorded daily. The content of DM in the feeds was determined on days 1, 3 and 5 of week 3 of each period. Sub-samples of forages (silage, ST and FR) collected once per experimental period, were freeze-dried and ground through a 1 mm screen (Wiley Mill, 158; Arthur H. Thomas, Philadelphia, PA, USA) prior to chemical analyses. Leaves and roots of ST and leaves and stems of FR were analysed separately. Leaf : root and leaf : stem ratios were determined for ST and FR, respectively. Dry matter content was determined by weighing before and after drying in a forced-air oven at 105°C for 12 h. For each sample, ash and lipids were analysed according to AOAC (1996; method 942.05 and 920.39 for ash and lipids, respectively); nitrogen (N) content was determined by combustion (Leco Model FP-428 Nitrogen Determinator; Leco Corporation, St Joseph, MI, USA) and was used to calculate CP content (N × 6.25). Neutral detergent fibre was determined as aNDF (Van Soest et al., Reference Van Soest, Robertson and Lewis1991) using heat stable amylase (Ankom Technology Corp., Macedon, NY, USA) and ADF according to AOAC (1996; method 973.18). Digestible organic matter on DM basis (DOMD) was measured according to Tilley and Terry (Reference Tilley and Terry1963) and was used to estimate ME by regression (ME = 0.279 +0.0325 * DOMD(%)) (Poff et al., Reference Poff, Balocchi and Lopez2011). Non-fibrous carbohydrates (NFCs; g/kg DM) were estimated as follows:

Cows were milked at 0700 and 1600 h and milk yield was recorded daily with a flow sensor (MPC580 DeLaval, Tumba, Sweden) during the experimental periods. The average for the final week of each period is reported. Representative milk samples were collected at morning and afternoon milkings for 3 days in the last week of the experimental period for fat, protein, lactose and milk urea analyses by mid-IR spectrophotometry (Foss 4300 Milko-scan; Foss Electric, Hillerod, Denmark).
Rumen fermentation
Rumen fluid was harvested by stomach tubing (Flora Rumen Scoop; Prof-Products, Guelph, ON, Canada) before (1000 h) and 6 h (1600 h) after brassica supplementation on day 6 of week three in each experimental period.
Samples were strained through four layers of cheesecloth. A 10-ml sample was drawn off, mixed with 0.2 ml of 50% (wt/vol) sulphuric acid and stored at −20°C pending determination of short-chain fatty acid (SCFA) and ammonia (NH3) concentrations. Rumen fluid was allowed to thaw for 16 h at 4°C and then centrifuged at 10 000×g for 10 min at 4°C. Six microlitres of supernatant was drawn off and then centrifuged at 10 000×g for 10 min at 4°C. Thawed supernatant of rumen fluid samples was analysed for SCFAs by gas chromatography as described by Tavendale et al. (Reference Tavendale, Meagher, Pacheco, Walker, Attwood and Subathira2005) and for NH3 by the phenol-hypochlorite reaction method (Weatherburn, Reference Weatherburn1967). Total SCFA (tSCFA) were considered as the sum of acetate, butyrate, proportionate, isobutyrate, valerate, isovalerate and caproate. Minor SCFAs are the sum of isobutyrate, isovalerate, valerate and caproate.
For the whole experiment, rumen pH was monitored by wireless telemetric pH bolus (eCow, Exeter, UK). Before the experiment started, an internal validation of the pH bolus was conducted with three cannulated cows. The ruminal samples were collected during 2 days from three sites within the rumen (cranial, ventral and caudal) every 2.5 h. Immediately after collection of rumen fluid, pH was measured by glass electrode (Model HI98127; Hanna Instruments, Woonsocket, RI, USA). The pH boluses were calibrated before use, programmed to measure rumen pH at 15-min intervals and inserted directly into the ventral sac of the rumen of each cow. The data were transmitted wirelessly to a transceiver connected to a cell phone and then transferred to a laptop computer. The pH data were summarized as mean pH, pH per hour, time spent with pH < 6.2 and >6.0, and below pH 6.0.
Urine collection and purine derivative measurements
Spot urine samples (20 ml) were collected by vulva stimulation (Cosgrove et al., Reference Cosgrove, Jonker, Lowe, Taylor and Pacheco2017) every 3 h once a day during day 5 of week 3 in each experimental period, to estimate rumen microbial N flow based on purine derivatives (PDs, allantoin + uric acid) by HPLC. Samples were acidified with 2 ml sulphuric acid (10% v/v) to maintain pH below 3 and stored at −20°C. Urine samples were thawed, a composited sample per cow was made for each period and analysed for allantoin, uric acid and creatinine by HPLC. Urine volume was estimated using creatinine concentration as a marker (Lindberg, Reference Lindberg1989). The amount of microbial purines absorbed (PA, mmol/day) and the PD excreted was estimated from the predictive model proposed by Singh et al. (Reference Singh, Sharma, Dutta, Singh, Verma and Mehra2007) and the microbial nitrogen flow (MN, g/day) was estimated according to Makkar (Reference Makkar, Makkar and Chen2004). Full equations are presented in Supplementary Material S1.
Haematological measures
Blood samples were collected on day 4 of week 3 in each period, after morning milking. Blood was collected from a coccygeal vessel using two evacuated blood collection tubes (BD Vacutainer; Becton, Dickinson and Company, New Jersey, USA): one containing Ethylenediaminetetraacetic acid (4 ml) and one with no anticoagulant (9 ml). Samples were transported on ice to the haematology laboratory of the Universidad Austral de Chile Teaching Hospital for analysis, where they were centrifuged at 3000 × g for 10 min at 25°C. Whole blood was used for determination of complete blood count; red blood cell (RBC); white blood cell (WBC); haemoglobin concentration; packed red cell volume (PCV); mean corpuscular volume and mean corpuscular haemoglobin concentration. For evaluation of these haematologic parameters, an automated haematology analyzer (KX-21 N; Sysmex, Kobe, Japan) was used. The blood smears were stained with rapid staining (Hemacolor; Merck, Darmstadt, Germany) for a differential WBC count. Heinz-Ehrlich bodies were counted in blood smears prepared on average 5 h after sampling, using crystal violet staining, and expressed as per cent erythrocytes containing one or more inclusion bodies. Serum samples were stored at −20°C until analysed for urea and gamma-glutamyl transferase (GGT) using a Wiener Metrolab 2300 auto-analyzer (Wiener Lab., Rosario, Argentina) at 37°C, and triiodothyronine (T3) using a validated Elisa Kit (MyBioSource, San Diego, CA, USA).
Statistical Analyses
The data were analysed using the mixed model procedure of SAS (2006; version 9.4; SAS Institute Inc., Cary, NC, USA) to account for carryover effect according to the following model:

where yijklm is an observation for each dependent variables; μ is the general mean; Si is the fixed effect of the ith treatment sequence; A(i)j is the random effect of the jth cow in the ith sequence; P(i)k is the fixed effect of the kth period; Tl is the fixed effect of the lth treatment; Cm is the fixed carryover effect from the previous period (C = 0, if period = 1) and e(ijk)l is the random error. If carryover effects were not detected, a simplified model for a replicated Latin square was used:

where yijklm is the observation for dependent variables; μ is the general mean; Si is the random effect of the ith square; A(i)j is the random effect of the jth cow in the ith square; P(i)k is the fixed effect of the kth period; Tl is the fixed effect of the lth treatment and e(ijk)l is the random error. The interaction of treatment and period was tested and was determined to be not significant (P > 0.05). As a result, this interaction was removed from the model. Data for DMI, milk yield, milk composition, microbial N and haematological measures were summarized by day. For haematological measures, three tubes were not reported by the laboratory due to coagulation of samples, and therefore different standard errors are reported for each treatment. Data for SCFA, NH3 and pH were analysed with the same model but including sampling time or hour as a repeated measurement and the interaction of treatment and repeated measurement, with cow as a subject. The estimation method was REML and the df method was Kenward–Roger. The variance–covariance structure that yielded the lowest corrected Akaike information criterion was compound symmetry and selected for the final model. Values reported are least squares means and associated standard errors of the mean. Statistical significance was declared at P ≤ 0.05 and trends at 0.05 < P ≤ 0.10. The PDIFF command, incorporating the Tukey–Kramer adjustment for multiple pairwise comparisons of treatment means, was used (Supplementary Material S2).
Results
Feed and nutrient intake, milk production and composition
Cows supplemented with either ST or FR had a lower DMI (P < 0.001) compared to those fed the control diet, basically due to the lower silage (−3.56 kg/day) and supplement (−0.73 kg/day) intake that was not completely compensated by the ingestion of brassica (3.18 kg/day) (Table 2). Among brassica-supplemented cows, ST intake tended (P = 0.09) to be greater (+0.18 kg/day) compared to FR.
Table 2 Feeds and nutrient intake, and milk yield composition of cows supplemented with turnip or rape
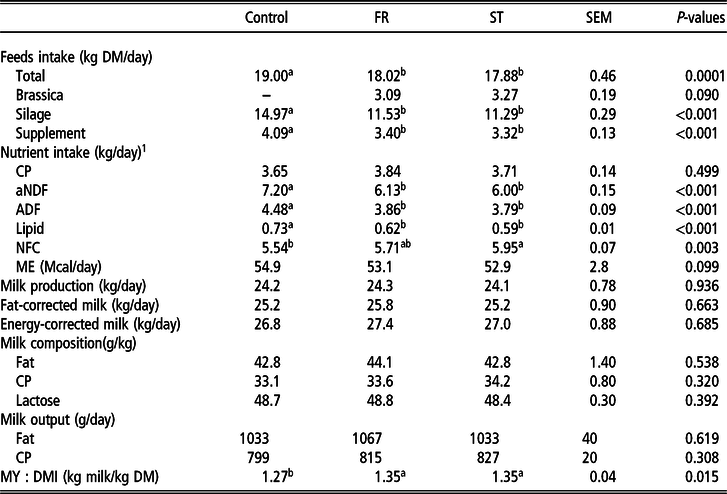
FR = treatment with a 25% of forage rape inclusion in the diet; ST = treatment with a 25% of summer turnip inclusion in the diet; aNDF = neutral detergent fibre with a heat stable amylase; NFC = non-fibrous carbohydrates; ME = metabolizable energy (ME = 0.279 + 0.0325 * DOMD (%))2; fat-corrected milk = 0.4 × kg milk + 15 × kg fat; energy-corrected milk = (12.82 × kg fat) + (7.13 × kg protein) + (0.323 × kg milk); MY : DMI = feed conversion efficiency (milk yield per unit of dry matter intake); SEM = standard error of the mean; means within a row with different superscripts differ (P < 0.05); DOMD = digestible organic matter on DM basis.
1 Unless stated.
No difference in CP intake was observed (P = 0.499), whereas cows supplemented with either ST or FR had lower lipid, aNDF and ADF intakes (P < 0.001). Cows supplemented with ST increased their NFC intake by 0.41 kg/day compared to the control treatment (P = 0.003).
Milk production and composition were not affected by brassica supplementation (P > 0.05). This resulted in a greater feed conversion efficiency for cows supplemented with ST or FR compared to the control diet (+0.08 kg milk/kg DM; P = 0.015).
Rumen pH and fermentation
Significant interactions among diet and time of sampling were observed for tSCFA, the relative molar proportion of each SCFA in tSCFA and NH3 concentrations (P < 0.05; Table 3). Total SCFA concentration and butyrate molar proportion were similar among diets prior to brassica supplementation. However, 6 h after supplementation, the rumen fluid of cows supplemented with ST had greater concentrations of tSCFA and butyrate compared to that from cows fed the control diet. Acetate molar proportion was similar among diets before brassica supplementation, whereas after 6 h it was greater in the rumen of cows supplemented with FR. Meanwhile, propionate molar proportion was lower in the rumen of cows fed ST prior to brassica feeding but lowest in cows fed FR after feeding of the crop. The molar proportions of minor SCFA and NH3 concentration were similar among diets prior to brassica supplementation, but 6 h after supplementation a significant reduction on minor SCFA and NH3 was observed with either FR or ST supplementation. Finally, Ac : Pr was only increased with FR supplementation.
Table 3 Effect of diet and sampling time on total short-chain fatty acids (tSCFA) and ammonia (NH3) concentrations, and in the molar proportions of individual SCFA in the rumen fluid of cows supplemented or not with turnip or rape
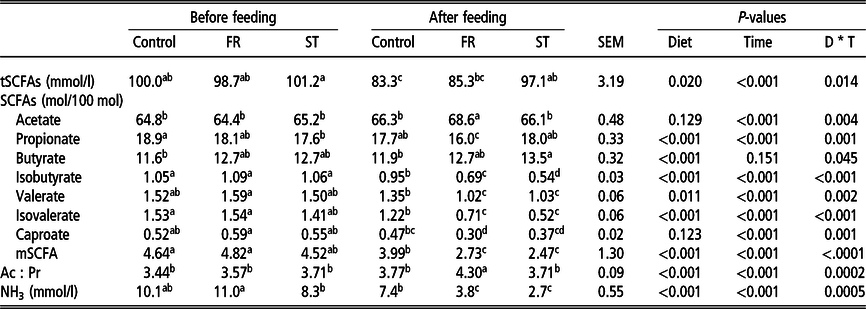
FR = treatment with a 25% of forage rape inclusion in the diet; ST = treatment with a 25% of summer turnip inclusion in the diet; tSCFA, total short-chain fatty acids (acetate + butyrate + propionate + isobutyrate + isovalerate + valerate + caproate; mmol/l); mSCFA, minor short-chain fatty acids (isobutyrate + isovalerate + valerate + caproate) (mol/100 mol); Ac : Pr = acetate : propionate ratio; NH3 = ammonia (mmol/l); SEM = standard error of the mean for ‘diet*time of the day’ interaction; D * T = interaction between diet and time of the day; means within a row with different superscripts differ (P < 0.05).
The daily mean of rumen pH was lower (6.23) for cows supplemented with ST compared to the control and FR diets (6.32 and 6.30, respectively; Table 4) and remained below 6.2 for 143 and 110 more min compared to control and FR diets, respectively. A significant interaction was observed for diet and time of the day (Figure 1). Rumen pH followed a similar pattern for all treatments, except after p.m. (1600 h) milking: for the control diet, the rumen pH dropped from 6.38 to 6.14 and then remained relatively constant until 2000 h, when it started to increase. For FR and ST diets, the rumen pH dropped to 5.96 after p.m. milking and then increased.
Table 4 Effect of rape and turnip supplementation on rumen pH, urinary purine derivative (PD) excretion and blood and milk urea concentrations of mid-lactation dairy cows.
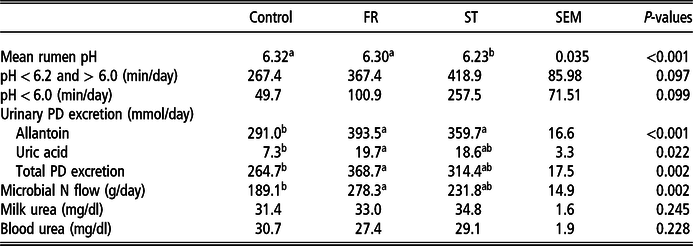
FR = treatment with a 25% of forage rape inclusion in the diet; ST = treatment with a 25% of summer turnip inclusion in the diet; SEM = standard error of the mean; means within a row with different superscripts differ (P < 0.05).
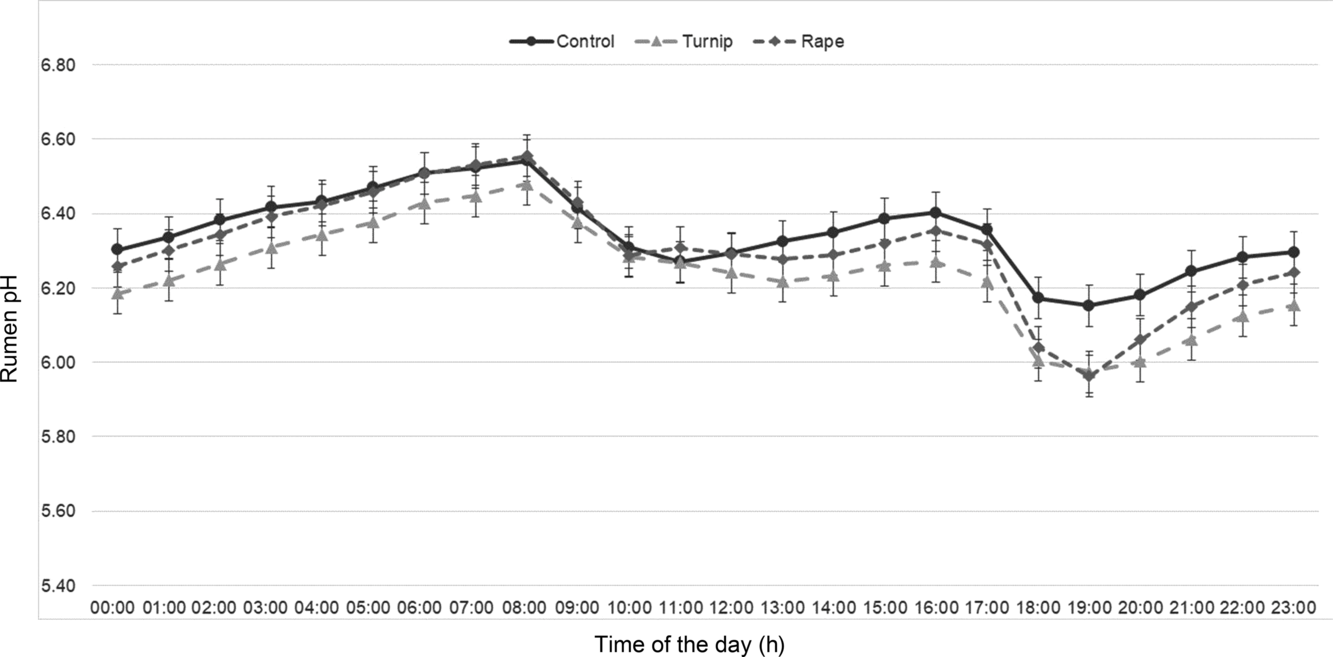
Figure 1 Effect of supplementation with turnips or rape on diurnal variation of rumen pH of mid-lactation dairy cows.
Urinary purine derivatives, milk and blood urea and haematological measures
Daily urinary excretion of allantoin was increased with ST and FR supplementation (P < 0.001), whereas uric acid and PD excretion were greater for cows fed FR than for cows fed the control diet, which resulted in a greater estimated MN (+89 g/day; P = 0.002). Blood and milk urea were not affected by brassica supplementation (P > 0.05) (Table 4).
No differences for any of the haematological values were observed (P > 0.05). No Heinz-Ehrlich bodies were detected for any of the dietary treatments. Also, GGT and T3 were not affected by either FR or summer ST supplementation (Table 5).
Table 5 Effect of rape and turnip supplementation in the diet of lactating cows on blood health parameters (least square mean values ± standard error of the mean)
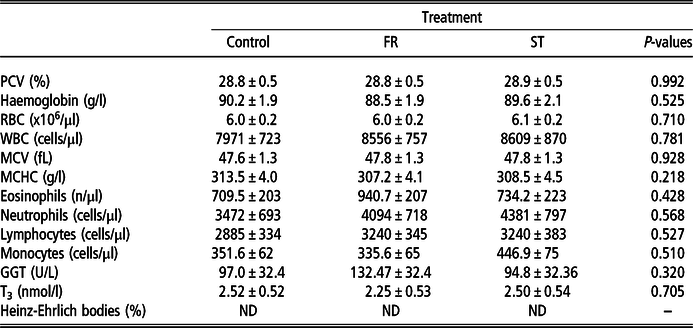
FR = treatment with a 25% of forage rape inclusion in the diet; ST = treatment with a 25% of summer turnip inclusion in the diet; PCV = packed red cell volume; RBC = red blood cell; WBC = white blood cell; MCV = mean corpuscular volume; MCHC = mean corpuscular haemoglobin concentration; GGT = gamma-glutamyl transpeptidase; T3 = triiodothyronine; ND = not detected.
Discussion
Intake, performance and milk composition
Total DM intake was reduced in cows receiving either ST or FR, due to a lower DMI of 3.1 and 3.3 kg DM (for FR and ST, respectively) out of the 5 kg DM of brassica that were offered to replace the silage and concentrate from the control diet. This finding is similar to that from Moate et al. (Reference Moate, Dalley, Martin and Grainger1998), who reported a reduction in DMI when lactating dairy cows were supplemented with ST. The reduction in voluntary DMI may be attributed to physical limitations for ingestion due to the high water content and bulkiness of brassicas that increase the fresh weight that cows have to ingest, and therefore affects DMI (Stefanski et al., Reference Stefanski, Garcia, Farina, Tan and Tanner2010). For example, compared to the control diet, the fresh weight intake amounts increased by 16.1 and 23.3 kg for cows supplemented with FR and ST, respectively. Cows supplemented with ST spent 46 and 98 more min eating (in 11 h of observation; data not shown) compared to cows offered the FR and control diets, respectively. In contrast, cows ruminating time was 42 and 61 min longer for cows fed the control diet compared to those in the FR and ST groups, respectively.
Estimated ME (54.9, 53.1 and 52.9 Mcal/day for control, FR and ST, respectively) and CP intakes (3.65, 3.84 and 3.71 kg/day for control, FR and ST, respectively) were similar across diets. Milk production levels observed in this study were around 24.1 and 24.3 kg/day. According to AFRC (1995), ME and protein allowable milk production for a 550 kg liveweight Holstein Friesian cow were 29 and 34 kg of milk/day, respectively (AFRC, 1995). Therefore, ME and CP intakes were not limiting milk production of these mid-lactation dairy cows.
The brassicas used in this study were representative of the characteristics previously described by others, namely high readily fermentable carbohydrates with low NDF (<280 g/kg DM) concentrations (Barry, Reference Barry2013). These characteristics resulted in a greater NFC and lower NDF intake compared to the control diet, due to the partial replacement of grass silage with either ST or FR.
Moate et al. (Reference Moate, Dalley, Martin and Grainger1998) reported that replacing 3 kg of barley with 3 kg of ST did not affect milk production. Including 4.4 kg of ST instead of barley reduced milk production, whereas the combination of 2.8 kg of ST with a protein source (cottonseed meal or lupins), increased milk production (Moate et al., Reference Moate, Dalley, Roche, Grainger, Hannah and Martin1999). No changes in milk composition with ST supplementation have been reported in other studies (Moate et al., Reference Moate, Dalley, Martin and Grainger1998 and Reference Moate, Dalley, Roche, Grainger, Hannah and Martin1999; Thomson et al., Reference Thomson, Clark, Waugh, van der Poel and MacGibbon2000). Williams et al. (Reference Williams, Moate, Deighton, Hannah, Wales and Jacobs2016) replaced 8.0 kg DM of lucerne cubes with FR and observed an increase in milk production (+3.6 kg/day), without changes in milk composition.
Rumen function
Summer turnips and FR diets contained a greater concentration of NFC compared to the control diet. According to Keim et al. (Reference Keim, Cabanilla, Balocchi, Pulido and Bertrand2019), Barkant ST contains 82 g/kg of starch compared to 59 g/kg of starch in Spitfire FR (the cultivars used in this study) and more water soluble carbohydrates (144 v. 131 g/kg DM; specifically sucrose, glucose and fructose). These differences in type and quantity of NFC are expected to modulate rumen fermentation (Oba, Reference Oba2011) and might be responsible for the greater tSCFA and butyrate in the rumen of cows (6 h after supplementation) supplemented with ST. Cows supplemented with FR had greater acetate and lower propionate proportions in the rumen fluid, compared to the other two diets, resulting in greater Ac : Pr. The changes in SCFA molar proportions may be explained by lower starch concentrations in FR that did not compensate, as much as ST did, for the starch reduction associated with the reduction in grain-based concentrate in the diet of the cows in this study, since it is well known that fermentable starch increases propionate concentration and reduces pH in the rumen (Mohammed et al., Reference Mohammed, Kennelly, Kramer, Beauchemin, Stanton and Murphy2010). Although mean ruminal pH was lower for cows supplemented with ST compared to the other dietary treatments, ruminal pH values were below 6.0 for 258 min per day but did not reach values below 5.8 at any time of the day, and therefore the risk of subclinical acidosis was considered minimal based on the accepted guidelines (Krause and Oetzel, Reference Krause and Oetzel2006). This is in agreement with Keogh et al. (Reference Keogh, French, Murphy, Mee, McGrath, Storey, Grant and Mulligan2009b) who found that when feeding 100% kale to pregnant dry dairy cows found that ruminal pH was not depressed below pH 6.1. The authors attributed this to the large bulk density of forage kale resulting in high levels of saliva production, thus buffering rumen pH which is similar to what was observed in this study
In contrast to our results, Sun et al. (Reference Sun, Waghorn, Hoskin, Harrison, Muetzel and Pacheco2012) reported no differences in tSCFA and molar proportions of individual SCFAs in the rumen of sheep fed ST or FR. The contrast between the report of Sun et al. (Reference Sun, Waghorn, Hoskin, Harrison, Muetzel and Pacheco2012) and our study might be explained by differences in fermentation pathways across animal species. For example, Muetzel et al. (Reference Muetzel, Hunt and Tavendale2014) observed greater acetate and lower propionate concentrations in the rumen fluid from cattle compared to that of sheep and also reported donor species by feedstuff interactions on the molar proportions of acetate in in vitro batch-culture studies. Supplementation with both summer ST and FR reduced minor SCFA and NH3 concentrations in the rumen, which is consistent as these metabolites are produced in the rumen from deamination and decarboxylation processes from feed or microbial protein, and differences in branched chain SCFA concentrations probably reflect differences in one or both of these components (Liu et al., Reference Liu, Wang, Guo, Huo, Zhang, Pei, Zhang and Wang2018). The difference in minor SCFA is in agreement with the greater MN observed with FR supplementation compared to the control diet. As rumen bacteria use NH3 nitrogen and branched chain SCFAs for their growth, therefore lowering NH3 N and branched chain SCFAs concentrations which is comparable to our study with greater MN (Roman-Garcia et al., Reference Roman-Garcia, White and Firkins2016). The greater MN when supplementing FR may be due to better energy supply in the rumen because of its high DM degradation rate (Keim et al., Reference Keim, Cabanilla, Balocchi, Pulido and Bertrand2019) or due to changes in microbial communities. For example, Sun et al. (Reference Sun, Henderson, Cox, Molano, Harrison, Luo, Janssen and Pacheco2015) reported that feeding FR to lambs modified bacterial communities in the rumen compared to ryegrass-fed animals. They observed greater protozoal cell numbers in the rumen of lambs fed FR. This increase might be in agreement with the greater MN observed in our study, as protozoa can represent from 10% to 30% (rarely exceeding 20%) of MN to the small intestine (Sok et al., Reference Sok, Ouellet, Firkins, Pellerin and Lapierre2017) and the technique we used to estimate MN (PDs; Supplementary Material S3) also considers protozoa. The greater MN in cows supplemented with FR did not result in greater milk protein concentration or protein yield. This might be due to a surplus in the supply of amino acids to the mammary gland relative to the animal’s demands, as it is required that cows have the genetic merit to produce more milk protein (Chagunda et al., Reference Chagunda, Romer and Roberts2009). The postulated excess in amino acids relative to the requirements may also provide an explanation for the similar blood and milk urea concentrations observed in the three dietary treatments, despite the lower ruminal NH3 concentrations measured in this study. Lower NH3 concentrations in the rumen would be expected to have resulted in lower plasma urea concentrations (Lapierre et al., Reference Lapierre, Berthiaume, Raggio, Thivierge, Doepel, Pacheco, Dubreuil and Lobley2005), and these be accompanied by lower milk urea concentrations (Pacheco and Waghorn, Reference Pacheco and Waghorn2008). However, amino acids that are surplus to requirements will be catabolized and contribute to the urea pool. Also, the efficiency of transfer of absorbed amino acids into milk protein decreases with increasing supply of protein and is linked directly with increased hepatic removal of amino acids (Lapierre et al., Reference Lapierre, Berthiaume, Raggio, Thivierge, Doepel, Pacheco, Dubreuil and Lobley2005 and Reference Lapierre, Pacheco, Berthiaume, Ouellet, Schwab, Dubreuil, Holtrop and Lobley2006).
Haematological measures
Secondary compounds in brassicas, such as glucosinolates and SMCO, can produce nutritional and health disorders (Keogh et al., Reference Keogh, French, Murphy, Mee, McGrath, Storey, Grant and Mulligan2009b). For example, Coxganser et al. (Reference Coxganser, Jung, Pushkin and Reid1994) observed increased Heinz-Ehrlich body counts on RBCs (which are associated with anaemia and depressed DMI), lower PCV and altered thyroid function in lambs grazing brassicas. Furthermore, Collett et al. (Reference Collett, Stegelmeier and Tapper2014) stated that some nitrile derivatives of glucosinolates from ST and FR can be hepatotoxic. However, in our study no Heinz-Ehrlich bodies were observed, and there were no differences for any of the blood parameters suggesting that at the level of inclusion we used, supplementation with summer ST and FR does not cause anaemia, liver damage or altered thyroid function.
The fact that supplementation with summer ST and FR did not decrease milk production and milk composition is an advantage for dairy farmers. These crops may increase farm profitability and allow increases in the feeding budget (due to their greater DM yields than grass) and promote diversity of the feed base used. The replacement of grass silage and commercial concentrates with brassicas reduces feeding costs. Garcia et al. (Reference Garcia, Fulkerson and Brookes2008) reported that growing crops on-farm helps to reduce the need for purchased supplements and, therefore, reduces production costs and increases profitability.
These results indicate that mid-lactation dairy cows fed with brassicas are able to maintain production despite the reduced intake, probably due to improved rumen fermentation and nutrient utilization.
Acknowledgements
This research was funded by the Chilean National Fund for Science and Technology (FONDECYT), Project code 11150538.
O. Balocchi 0000-0003-1664-783X
R. Pulido 0000-0002-7107-8054
P. Sepúlveda-Varas 0000-0003-3349-0977
D. Pacheco 0000-0002-9307-9197
J. P. Keim 0000-0003-4277-6491
Declaration of interest
All authors declare no conflicts of interest.
Ethics statement
All experimental procedures were approved by the Universidad Austral Institutional Animal Care and Use Committee (Approval Number: 237/2015).
Software and data repository resources
If requested, data can be provided via e-mail by the corresponding author.
Supplementary material
To view supplementary material for this article, please visit https://doi.org/10.1017/S175173112000021X