Introduction
Landscape water restrictions and regulations are now commonplace as a result of the increase in population and water use in urbanized areas (Boyer et al. Reference Boyer, Dukes, Duerr and Bliznyuk2018). A promising strategy to reduce water use in turfgrass landscapes is the use of drought-tolerant turfgrass species (Boeri et al. Reference Boeri, Unruh, Kenworthy, Rios and Trenholm2021; Gomez-Armayones et al. Reference Gomez-Armayones, Kvalbein, Aamlid and Knox2018; Zhang et al. Reference Zhang, Unruh and Kenworthy2013, Reference Zhang, Unruh and Kenworthy2015). Bahiagrass is one species that possesses this attribute. This native of South America is adapted to sandy soils, has low fertility requirements, and does not require high inputs of water (Boeri et al. Reference Boeri, Unruh, Kenworthy, Rios and Trenholm2021; Burton Reference Burton1943; Parodi Reference Parodi1937; Trenholm et al. Reference Trenholm, Cisar and Unruh2003).
‘Pensacola’ and ‘Argentine’ are two bahiagrass cultivars available on the market that are widely utilized in subtropical areas. Pensacola bahiagrass was found near Pensacola, FL, and released in 1944 by the Georgia Soil Conservation Service and the Florida Agricultural Experiment Station (Burton Reference Burton1946; Houck Reference Houck2009). Compared to Argentine, Pensacola produces more seedheads, which make it less desirable for use in lawns but suitable for low-maintenance areas like roadsides (Trenholm et al. Reference Trenholm, Cisar and Unruh2003). Argentine, a cultivar native to Argentina, has broad light-green leaves and produces shorter and fewer seedheads than Pensacola, making it acceptable for use as a lawn grass (Busey Reference Busey1989; Rios et al. Reference Rios, Blount, Erickson, Quesenberry, Altpeter, Cellon and Kenworthy2013; Trenholm et al. Reference Trenholm, Cisar and Unruh2003). A lesser-known cultivar, ‘Wilmington’, which exhibits more cold tolerance, was found in coastal North Carolina and was released in 1971 by the Mississippi Agriculture Experiment Station and Natural Resource Conservation Service (Houck Reference Houck2009). Although it is difficult to find seeds on the market, Wilmington has improved turf characteristics, including narrow leaves that are darker green than other cultivars (Newman et al. Reference Newman, Vendramini and Blount2014; Rios et al. Reference Rios, Blount, Erickson, Quesenberry, Altpeter, Cellon and Kenworthy2013). Although Argentine and Wilmington have better turf characteristics than do other cultivars on the market, their unsightly seedheads and open growth habit limit consumer acceptance (Rios et al. Reference Rios, Kenworthy, Blount, Quesenberry, Unruh, Erickson, Altpeter and Moñuz2017).
Argentine and Wilmington are tetraploid cultivars that reproduce primarily by apomixis (Acuña et al. Reference Acuña, Blount, Quesenberry, Hanna and Kenworthy2007). Apomixis is the development of seeds without fertilization, which produces progeny that are genetically identical to each other and their maternal plant (Hanna and Bashaw Reference Hanna and Bashaw1987; Kannan et al. Reference Kannan, Davila-Olivas, Lomba and Altpeter2015). An apomictic mode of reproduction is a barrier to conventional breeding (Rios et al. Reference Rios, Kenworthy, Blount, Quesenberry, Unruh, Erickson, Altpeter and Moñuz2017). However, mutagenesis (i.e., using x-rays, gamma-rays, and ethyl methanesulfonate) is a method to create genetic variants in bahiagrass (Kannan et al. Reference Kannan, Davila-Olivas, Lomba and Altpeter2015; Pereira et al. Reference Pereira, Rios, Kenworthy, Quesenberry, Blount, Erickson, Altpeter and Munoz2017). Using mutagenesis as a breeding technique, researchers at the University of Florida developed nine new lines with improved density, darker green color, and fewer seedheads compared to their parental lines (Pereira et al. Reference Pereira, Rios, Kenworthy, Quesenberry, Blount, Erickson, Altpeter and Munoz2017; Rios et al. Reference Rios, Blount, Erickson, Quesenberry, Altpeter, Cellon and Kenworthy2013). However, their tolerance to commonly used herbicides remains unknown.
Turfgrass tolerance to herbicides with different mechanisms of action is essential for effective weed management and reduction of herbicide resistance (Norsworthy et al. Reference Norsworthy, Ward, Shaw, Llewellyn, Nichols, Webster, Bradley, Frisvold, Powles, Burgos, Witt and Barrett2012). Pensacola bahiagrass treated with triclopyr, fluroxypyr, dicamba, triclopyr + 2,4-D, and triclopyr + dicamba maintained acceptable turf quality (>6.5, using a 1 to 9 scale, based on the National Turfgrass Evaluation Program) and were not different from the nontreated control (McCarty et al. Reference McCarty, Colvin and Higgins1996). Freitas et al. (Reference Freitas, Ferreira, Silva, Barbosa, Miranda and Machado2003) showed that triclopyr (0.25 to 1.2 kg ha–1) and 2,4-D + picloram (480 + 128 g ha–1) did not damage bahiagrass. Bahiagrass treated with halosulfuron and metsulfuron had 38% and 29% damage, respectively, 7 d after treatment (DAT) (Costa et al. Reference Costa, Martins, Rodriguez and Cardoso2010). However, the grass initiated recovery by 14 DAT and had completely recovered by 26 DAT. Bentazon was safe (<20% damage, using a 0 to 100% scale, 0 as no damage and 100% as plant death) on bahiagrass (Costa et al. Reference Costa, Martins, Rodriguez and Cardoso2010). Da Silva et al. (Reference Da Silva, Cesarin, Martins, Ferrarezi, Da Costa, Alves and Pivetta2016) showed the selectivity of sulfentrazone + diuron, triclopyr, 2,4-D, and halosulfuron in bahiagrass. Sulfentrazone + diuron injured bahiagrass, whereas halosulfuron, triclopyr, and 2,4-D were safe (Da Silva et al. Reference Da Silva, Cesarin, Martins, Ferrarezi, Da Costa, Alves and Pivetta2016). Metsulfuron is a broadleaf herbicide that is known to control bahiagrass (McElroy and Martins Reference McElroy and Martins2013). However, differential response to metsulfuron has been reported among bahiagrass cultivars (Bunnell et al. Reference Bunnell, Baker, McCarty, Hall and Colvin2003; Henry Reference Henry2013).
Evaluating the response of bahiagrass experimental genotypes to different herbicides is a necessary step leading to cultivar release and commercialization. Therefore, the objective of this study was to evaluate the tolerance of nine experimental bahiagrass genotypes and Argentine to 12 herbicides with different modes of action.
Materials and Methods
Experimental Design and Trial Management
Two experimental runs were conducted at the University of Florida, West Florida Research and Education Center, Jay, FL (30.776567°N, 87.141228°W), under greenhouse conditions. The first run was conducted from August 2 to October 5 in 2017. The experiment was repeated from September 29 to December 2 in 2017. The experiment was set up as a randomized complete block design with four replicates. Blocks were oriented from North to South to account for temperature variability in the greenhouse produced by the location of the cooling pad system. Treatment design was a three-way factorial arrangement with genotypes, herbicides, and rates as factors. Nine experimental bahiagrass lines were tested for their herbicide tolerance: ‘ArgM27’, ‘ArgM1’, and ‘ArgM16’ (Argentine mutants); ‘WilM7’, ‘WilM10’ and ‘WilM13’ (Wilmington mutants); WT4 (wild coarse-leaf type plant); WT6 (dwarf germplasm acquired from Argentina); WT16 (wild type, assumed Wilmington); and Argentine (industry standard used as the control) (Table 1). Three sprigs of each grass were planted into 10-cm2 pots filled with potting soil (Sungro. Fafard® 3B Mix Metro-Mix® 830, Agawam, MA) and medium sand mixture (1:1 vol/vol). The bottom of each pot was covered with a 7-cm × 7-cm mesh screen to retain the media. Grasses were established for 21 d and fertilized using soluble fertilizer 20-20-20 (Southern AG. Rubonia, FL) at 12 kg N ha–1 every week during this period. During establishment, plants were trimmed weekly at 9 cm in height. Trimming was performed every 10 d thereafter to mimic standard turfgrass maintenance practices. All plants were established and had a uniform cover at the time of application. Greenhouse temperature was regulated at 26 C, and a microjet irrigation system was programmed to maintain the plants under optimum moisture conditions.
Table 1. Common name, label herbicide tolerance classification on bahiagrass, herbicide mechanism of action according to the Weed Science Society of America (WSSA), and rates of the selected herbicides. Mechanism of action and g ai ha–1 are given in order, respectively to common names.

a Abbreviations: D, damaging; I, intermediate; NR, not registered; S, safe.
b University of Florida’s Pest Control Guide for Turfgrass Managers (Unruh Reference Unruh2012).
c Summary of Herbicide Mechanism of Action According to the Weed Science Society of America (WSSA). https://wssa.net/wp-content/uploads/WSSA-Mechanism-of-Action.pdf
d SpeedZone Southern. PBI-Gordon Corp., 1271 West 12th Street, Kansas City, MO 64101.
e Basagran T&O. BASF Corp., 26 Davis Drive, Research Triangle Park, NC 27709.
f Buctril 4EC. Bayer CropScience LP, P.O. Box 12014, 2 T.W. Alexander Drive, Research Triangle Park, NC 27709.
g QuickSilver T&O. FMC Corp., Agricultural Products Group, 1735 Market Street, Philadelphia, PA 19103.
h Acclaim Extra. Bayer CropScience LP.
i Spotlight. Dow AgroSciences LLC. Indianapolis, IN 46268.
j Sedge Hammer. Gowan Company, P.O. Box 5569, Yuma, AZ 85366-5569.
k MSM Turf. Makhteshim Agan of North America, Inc., 4515 Falls of Neuse Road, Suite 300, Raleigh, NC 27609.
l Dismiss South. FMC Corp. Agricultural Products Group.
m Certainty. Monsanto Company, 800 N Lindbergh Boulevard, St. Louis, MO 63167.
n Celsius WG. Bayer CropScience LP.
o Confront. Dow AgroSciences LLC.
Twelve postemergence herbicides belonging to five different modes of action classes were included in this study (Table 1). Nine of the herbicides are commonly used on bahiagrass and were included to compare the herbicide tolerance of experimental lines compared to Argentine. Limited products are labeled for control of grasses and sedges in bahiagrass. Therefore, three additional nonregistered products (sulfosulfuron, halosulfuron, and thiencarbazone + iodosulfuron + dicamba) were included. Except for metsulfuron, the herbicides were applied at two rates (Table 1). The maximum label rate (1×) and a twofold label rate (2×) were selected to test possible over-rate application performed by pesticide applicators. As metsulfuron controls bahiagrass at the label rate, a 2× rate was deemed unnecessary (McElroy and Martins Reference McElroy and Martins2013). Treatments were applied using a DeVries Research Track Sprayer (86956 State Highway 251, Hollandale, MN) calibrated to deliver 98 L ha–1 of water (operation pressure, 138 KPa) carrier through a single even flat-fan nozzle (Even Flat Spray Tip, 8004 EVS; TeeJet® Technologies, Wheaton, IL). The selected active ingredients were applied alone or in combination, depending on the commercial product registered for turfgrass following the label recommendations.
Data Collection
Turfgrass leaf injury was evaluated visually on a 0 (no injury) to 100% (complete necrosis) scale. Within this range, values ≥ 20% were considered unacceptable. Injury ratings were taken at 7, 14, and 28 DAT. The aboveground biomass was collected 30 DAT by trimming the leaves to 9 cm. Clippings were dried at 50 C until a constant weight was achieved. To control for variability in dry-mass potential across genotypes, dry-weight values were converted to a percentage growth relative to the nontreated control in each block and genotype using Equation 1:

where ΔG is the percentage growth obtained from the dry clippings yield, DWt is the recorded dry weight of a treated genotype in a given block, and DWc is the recorded dry weight of that nontreated genotype. Later, a 50-DAT visual rating was collected to evaluate recovery.
Data Analysis
Data were subjected to ANOVA with the mixed-effect model procedure using the packages “lm4”, “lmerTest” and “emmeans” in R (Bates et al. Reference Bates, Machler, Bolker and Walker2015; Kuznetsova et al. Reference Kuznetsova, Brockhoff and Christensen2017; Lenth Reference Lenth2019; R_Core_Team 2020). Genotype, herbicide, and rate were treated as fixed factors (Table 2). Block and experiment replicate were treated as random effects. When necessary, a square-root transformation was performed to meet the ANOVA assumptions of linear models. Data were back-transformed to present means. As metsulfuron was only applied at a 1× rate, this treatment was analyzed separately, and the variables herbicide and rate were not included in the statistical model. Mean comparisons were assessed by the LSD test at P = 0.05 probability level. Two principal component analyses (PCAs) were performed to identify genotype clustering using the singular-value decomposition method (prcomp function R) (R_Core_Team 2020). The response variables used in the PCAs were the injury 28 DAT and growth 30 DAT (means). For other herbicides, 1× and 2× rates were combined, and only 1× for metsulfuron. The visualization was performed using the package “factoextra” (Kassambara and Mundt Reference Kassambara and Mundt2017).
Table 2. ANOVA results for herbicide, bahiagrass genotype, herbicide rate, and their interactions, on four dates for bahiagrass injury and growth in greenhouse experiments.

a Abbreviation: DAT, days after treatment; G, genotype; H, herbicide; ns, not significant at P ≤ 0.05; R, rate.
b Refer to Table 1 for herbicide rates and product information.
c Asterisks indicate probability levels: *Significant at P = 0.05; **significant at P = 0.01; ***significant at P = 0.001..
Results and Discussion
Herbicide Responses
ANOVA revealed a significant herbicide-by-rate interaction on all four rating dates for injury (Table 2). The herbicide-by-rate interaction was also significant for growth (Table 2). Therefore, herbicide main effects were presented by rate. In general, the treatments either increased injury or decreased growth throughout the duration of the experiments. None of the herbicides tested, applied at 1× or 2×, produced >20% injury 7 DAT (data not shown). At 14 DAT, fenoxaprop-2× was the most damaging herbicide, causing 18% injury (Table 3). At 28 DAT, the most injurious herbicide applied at 2× rate was fenoxaprop (23%) followed by thiencarbazone + iodosulfuron + dicamba (17%), and sulfentrazone + imazethapyr (13%) (Table 3). Fenoxaprop (14%) and thiencarbazone + iodosulfuron + dicamba (12%) were the most injurious at the 1× rate. At 50 DAT, the injury from thiencarbazone + iodosulfuron + dicamba-2× (28%), fenoxaprop-2× (27%), sulfentrazone + imazethapyr-2× (24%), fenoxaprop-1× (15%), thiencarbazone + iodosulfuron + dicamba-1× (14%), and sulfosulfuron-2× injury (16%) increased relative to 28 DAT (Table 3). Bentazon, bromoxynil, carfentrazone + 2,4-D + MCPP + dicamba, carfentrazone, fluroxypyr, halosulfuron, and triclopyr + clopyralid injury were ≤5% (Table 3). These herbicides did not exceed the 20% threshold even at the 2× rate.
Table 3. Bahiagrass injury and plant growth (i.e., percent biomass relative to the nontreated) affected by herbicide treatments. Herbicides applied at two rates.a,b
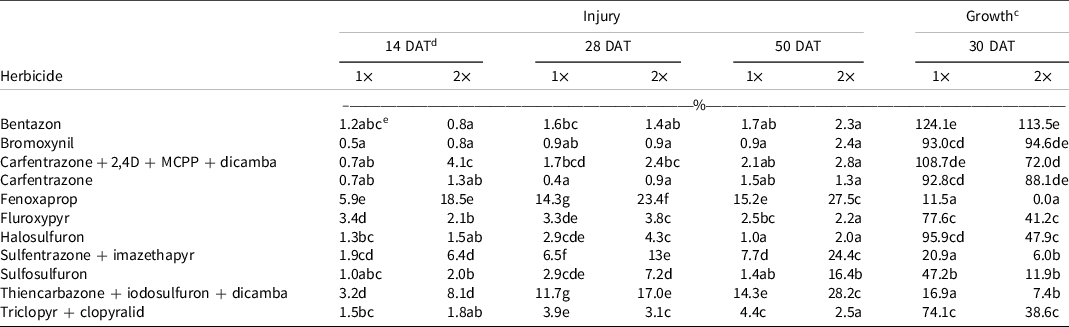
a Refer to Table 1 for herbicide rates and product information.
b Means were averaged across all genotypes (ArgM27, ArgM1, ArgM16, WilM7, WilM10, WilM13, WT4, WT6, WT16, and Argentine) and two experimental runs.
c Dry-weight values were converted to a percentage growth relative to nontreated control using Equation 1, described in Materials and Methods.
d Abbreviation: DAT, days after treatment.
e Values in the same column followed by the same letter are not significantly different (P = 0.05) according to LSD test.
In general, the same herbicides that produced injury also reduced growth. Fenoxaprop-2× completely suppressed growth (Table 3). Thiencarbazone + iodosulfuron + dicamba, sulfentrazone + imazethapyr, and sulfosulfuron-2× reduced growth to 10% (Table 3). Sulfosulfuron-1×, triclopyr + clopyralid-2×, fluroxypyr-2×, and halosulfuron-2× reduced growth by 50% to 60% (Table 3). Bentazon, bromoxynil, carfentrazone + 2,4-D + MCPP + dicamba, and carfentrazone did not affect growth (Table 3).
Genotype Responses
ANOVA revealed a significant genotype-by-rate interaction for injury 7 and 14 DAT (Table 2). However, this effect was not evident 28 and 50 DAT (Table 2). A significant herbicide-by-genotype interaction was not observed. Therefore, two methods were used to help elucidate and explain herbicide-by-genotype differences. As the injury of the safe herbicides was similarly low for all the genotypes and few differences between genotypes were observed, the safe herbicides (<20% injury) were removed from the analysis, and fenoxaprop, thiencarbazone + iodosulfuron + dicamba, and sulfentrazone + imazethapyr were analyzed separately (i.e., similar to metsulfuron). Furthermore, two PCAs were performed using injury 28 DAT (Figure 1) and growth data (Figure 2) from the damaging herbicides (i.e., fenoxaprop, thiencarbazone + iodosulfuron + dicamba, sulfentrazone + imazethapyr, and metsulfuron) to identify genotype clustering, related to their origin (i.e., coarse-texture genotypes and dwarf-type genotypes). The first and second principal components were extracted based on the percent of explained variances, and a two-dimensional biplot between the first and second principal components was generated.

Figure 1. Principal component analysis (PCA) of metsulfuron, fenoxaprop, sulfentrazone + imazethapyr, and thiencarbazone + iodosulfuron + dicamba, performed to identify genotype clustering using the singular-value decomposition method. Two-dimensional plot (Dim1 vs. Dim2). The response variable used in the PCA was injury 28 d after treatment (means averaged across 1× and 2× rates). Genotypes are shown as loadings and represented with dots. Herbicides are shown as vectors and represented with blue arrows.

Figure 2. Principal component analysis (PCA) of metsulfuron, fenoxaprop, sulfentrazone + imazethapyr, and thiencarbazone + iodosulfuron + dicamba, performed to identify genotype clustering using the singular-value decomposition method. Two-dimensional plot (Dim1 vs. Dim2). The response variable used in the PCA was % plant growth 30 d after treatment (means averaged across 1× and 2× rates). Genotypes are shown as loadings and represented with dots. Herbicides are shown as vectors and represented with blue arrows.
In general, genotype WT4 was the most susceptible to herbicide injury, whereas genotype WT6 was consistently the least injured (Tables 4–7). Genotype WT6 showed <20% injury from fenoxaprop, sulfentrazone + imazethapyr, and thiencarbazone + iodosulfuron + dicamba when applied at 1× (Tables 4–6). However, these herbicides suppressed growth almost completely (i.e., 9% to 25% of the nontreated) (Tables 4–6). The lesser injury of WT6, compared to the other genotypes, might be explained by the presence of abundant hairs or pubescence on its leaves, which could have reduced the herbicide contact (Su et al. Reference Su, Ozturk, Cakmak and Budak2009). In general, the coarse-texture genotypes showed more injury than the dwarf-type genotypes. The exception was Argentine, showing similar injury to genotype WT6 when treated with metsulfuron (Table 7).
Table 4. Bahiagrass genotype injury 14, 28, and 50 d after treatment (DAT), and plant regrowth (% relative to the nontreated) 30 d after fenoxaprop applied at two rates. a
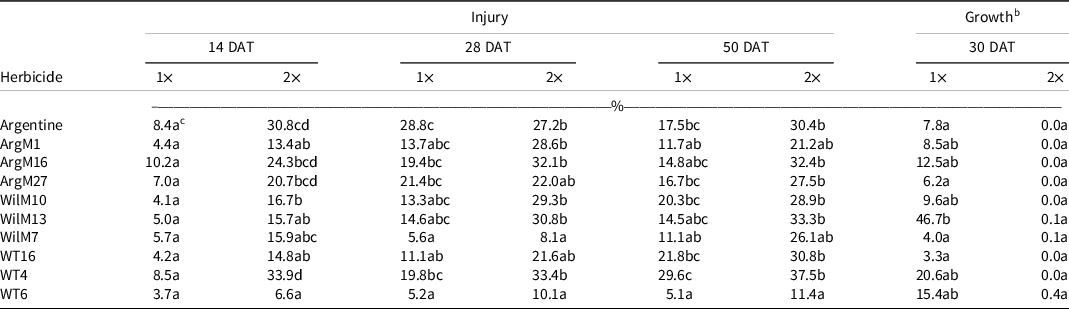
a Refer to Table 1 for herbicide rates and product information.
b Dry-weight values were converted to a percentage growth relative to nontreated control using Equation 1, described in Materials and Methods.
c Values in the same column followed by the same letter are not significantly different (P = 0.05) according to LSD test.
Table 5. Bahiagrass genotype injury 14, 28, and 50 d after treatment (DAT), and plant regrowth (% relative to the nontreated) 30 d after sulfentrazone + imazethapyr applied at two rates. a

a Refer to Table 1 for herbicide rates and product information.
b Dry-weight values were converted to a percentage growth relative to nontreated control using Equation 1, described in Materials and Methods.
c Values in the same column followed by the same letter are not significantly different (P = 0.05) according to LSD test.
Table 6. Bahiagrass genotype injury 14, 28, and 50 d after treatment (DAT), and plant regrowth (% relative to the nontreated) 30 d after thiencarbazone + iodosulfuron + dicamba applied at two rates. a

a Refer to Table 1 for herbicide rates and product information.
b Dry-weight values were converted to a percentage growth relative to nontreated control using Equation 1, described in Materials and Methods.
c Values in the same column followed by the same letter are not significantly different (P = 0.05) according to LSD test.
Table 7. Bahiagrass genotype injury 14, 28, and 50 d after treatment (DAT), and plant regrowth (% relative to the nontreated) 30 d after metsulfuron application. a

a Refer to Table 1 for herbicide rate and product information.
b Dry-weight values were converted to a percentage growth relative to nontreated control using Equation 1, described in Materials and Methods.
c Values in the same column followed by the same letter are not significantly different (P = 0.05) according to LSD test.
In the injury analysis, the first two principal components (PC1 and PC2) accounted for 83% of the total variability, where PC1 explained 63% of the total variability (eigenvalue = 2.54) and PC2 explained 20% (eigenvalue = 0.82) (Figure 1). Most of the herbicides were negatively correlated with PC1, meaning that the genotypes located on the left side of the graph had greater injury from the tested herbicides than those on the right. Metsulfuron also showed a positive association with PC2 (Figure 1). The PCA biplot generated a clear genotype clustering locating the Wilmington genotypes (WilM13, WilM10, and WilM7) in the upper right quadrant of the graph and the Argentine types (ArgM27, ArgM16, ArgM1, and Argentine) in the lower left quadrant (Figure 1). The association of these groups with the PC1 and PC2 suggests that the Wilmington types were less damaged by most of the herbicides but had greater damage by metsulfuron (Figure 1), whereas the Argentine types were less damaged by metsulfuron. Genotype WT4 was located in the upper left quadrant of the PCA, being the most susceptible genotype to most of the herbicides (Figure 1). WT6 occupied the opposite side of the graph showing the least herbicide damage (Figure 1).
The dwarf-type genotypes (WT6 and the Wilmington lines) were generally located on the right side of the PCA, showing less injury than the coarse-texture genotypes (WT4 and Argentine lines), suggesting a relationship between phenotype and herbicide tolerance. These relationships were also observed on the damaging herbicides (Tables 4–7). This result could suggest that genotypes with wider leaves had more surface area for herbicide contact, thus potentially increasing the amount of active ingredient in the plants and therefore greater herbicide injury. However, the current study did not measure herbicide plant concentration to sustain this assumption. The exception for this trend was metsulfuron, where the Argentine types showed less injury.
In the growth analysis, the PC1 explained 60% of variability (eigenvalue = 2.39), whereas the PC2 explained 29% (eigenvalue = 1.15) (Figure 2). Most of the herbicides were positively correlated with PC1, meaning that the growth of the genotypes located on the left side of the graph was less affected by the tested herbicides than those on the right. Sulfentrazone + imazethapyr was correlated to PC2. Therefore, genotypes located at the upper part of the graph were less affected by this herbicide combination. Genotype WilM13 was least affected by metsulfuron, fenoxaprop, and thiencarbazone + iodosulfuron + dicamba (Figure 2). In contrast, ArgM16 and WT6, located in the upper side of the graph, were less affected by sulfentrazone + imazethapyr. Even though ArgM16, WT6, and WilM13 were the least affected genotypes, to the tested herbicides, growth was still <50% (Tables 4–7). Finally, ArgM1 occupied the lower right side of the graph and was the most affected genotype (i.e., showing the least growth) to the four tested herbicides (Figure 2 and Tables 4–7).
The registered herbicides tended to follow the labeled tolerance specifications, whereas the unregistered products varied in their response. Regardless of the rate, fenoxaprop, sulfentrazone + imazethapyr, and thiencarbazone + iodosulfuron + dicamba reduced growth and caused turfgrass injury above an acceptable threshold. Triclopyr + clopyralid and fluroxypyr, herbicides that share the same mode of action, performed similarly in this study. Regardless of the rate, turfgrass injury was acceptable for these herbicides. Although triclopyr + clopyralid and fluroxypyr treatments reduced growth at the 2× rate, growth was 75% of the nontreated at the 1× rate. These results are in agreement with previous studies (Costa et al. Reference Costa, Martins, Rodriguez and Cardoso2010; Da Silva et al. Reference Da Silva, Cesarin, Martins, Ferrarezi, Da Costa, Alves and Pivetta2016). Although sulfosulfuron injury was below the acceptable range at both rates, biomass reduction was significant. Moreover, sulfosulfuron injury at the 2× rate was close to the 20% acceptability threshold. In the current study, halosulfuron injury was <5%. However, growth was reduced to 50% at the 2× rate. In other studies, halosulfuron has been classified as safe for bahiagrass (Da Silva et al. Reference Da Silva, Cesarin, Martins, Ferrarezi, Da Costa, Alves and Pivetta2016). Whereas Costa et al. (Reference Costa, Martins, Rodriguez and Cardoso2010) reported 38% damage to bahiagrass by halosulfuron, plants completely recovered by 26 DAT (Costa et al. Reference Costa, Martins, Rodriguez and Cardoso2010). This initial damage was not observed in our study, suggesting a differential cultivar sensitivity to this acetolactate synthase herbicide.
Previous studies suggested that herbicides can stimulate plant growth and produce biomass reallocation (Cedergreen Reference Cedergreen2008; Cedergreen et al. Reference Cedergreen, Streibig, Kudsk, Mathiassen and Duke2007; Cobb Reference Cobb1992). For example, synthetic auxins are known to increase cell elongation in roots and shoots at different concentrations (Cobb Reference Cobb1992). An increase in leaf elongation could explain why more clippings were harvested from some herbicide treatments, compared to the nontreated control.
Although there was variability in the experimental genotypes, Argentine showed similar responses to the Argentine mutants, whereas the Wilmington mutants showed the least injury. The exception to this occurred with the metsulfuron treatment. Metsulfuron produced >20% injury at 28 and 50 DAT in all the genotypes and inhibited growth. Argentine and WT6 were the least injured by metsulfuron. In previous studies, Argentine, ‘Paraguayan’, and common bahiagrass showed better tolerance to metsulfuron than Pensacola (Baker Reference Baker1996; Bunnell et al. Reference Bunnell, Baker, McCarty, Hall and Colvin2003). The authors concluded that these differences were not due to morphological traits but rather a possible site-of-action mutation or lack of esterase activity (Baker Reference Baker1996). Metsulfuron is used to control bahiagrass (McElroy and Martins Reference McElroy and Martins2013). Therefore, it is possible that the common cultivar Argentine might have some level of tolerance to the herbicide, and the mutants might have acquired that trait. Results from this research indicate that, in general, auxin mimics, protoporphyrinogen oxidase inhibitors, and their combinations are safe to use on both Argentine bahiagrass and the experimental lines. Conversely, acetolactate synthase inhibitors tend to be intermediate to damaging herbicides (especially when combined with other active ingredients). Although more research is needed (e.g., field trials), our finding suggests that halosulfuron could be safely used on bahiagrass at low rates. These results are in agreement with Da Silva et al. (Reference Da Silva, Cesarin, Martins, Ferrarezi, Da Costa, Alves and Pivetta2016). Finally, results from this research indicate that the bahiagrass experimental genotypes exhibited similar or less injury than Argentine bahiagrass to the tested herbicides.
Acknowledgments
The authors would like to acknowledge the West Florida Research and Education Center and UF Turfgrass Team for their contributions to the study. No conflicts of interest have been declared.