Introduction
Arbuscular mycorrhizal fungi (AMF) establish mutualistic symbioses with the majority of terrestrial plants in nature (Smith and Read Reference Smith and Read2008). This symbiosis contributes to approximately 80% of the nitrogen (N)/phosphorous (P) needs of growing plants (van der Heijden et al. Reference van der Heijden, Martin, Selosse and Sanders2015). Furthermore, AMF act as a sensitive indicator of ecological soil quality in diverse ecosystems (Verbruggen et al. Reference Verbruggen, Van Der Heijden, Weedon, Kowalchuk and Roling2012). The unique linkage of AMF in soil–plant macronutrient cycles might, therefore, affect the growth and community structure of weeds in variety of ways (Daisog et al. Reference Daisog, Sbrana, Cristani, Moonen, Giovannetti and Barberi2012; Helgason et al. Reference Helgason, Feng, Sherlock, Young and Fitter2014; Jordan et al. Reference Jordan, Zhang and Huerd2000; Veiga et al. Reference Veiga, Jansa, Frossard and Van Der Heijden2011). For instance, AMF could balance the competitive relationship between native and exotic species in many weed invasion events (Hilbig and Allen Reference Hilbig and Allen2015; Klabi et al. Reference Klabi, Hamel, Schellenberg, Iwaasa, Raies and St-Arnaud2014; Madawala Reference Madawala2014; Weber et al. Reference Weber, King and Aho2015). However, whether the root mycorrhizal infection characteristics differ between native and exotic plants or whether the soil AMF community that supports the coexisting plant system changes during the spreading period of exotic species is still poorly understood (Bunn et al. Reference Bunn, Lekberg, Gallagher, Rosendahl and Ramsey2014; Busby et al. Reference Busby, Stromberger, Rodriguez, Gebhart and Paschke2013; Weber et al. Reference Weber, King and Aho2015).
A wealth of studies have revealed that AMF might adopt different strategies in infecting the roots of native and exotic species, thus inducing different invasive results (e.g., Barto et al. Reference Barto, Friese and Cipollini2010; Endresz et al. Reference Endresz, Somodi and Kalapos2013; Hilbig and Allen Reference Hilbig and Allen2015). For example, the AMF infection in invasive species was more intense than in native species, thus directly benefiting the exotic species and contributing to the invasion (Bunn et al. Reference Bunn, Lekberg, Gallagher, Rosendahl and Ramsey2014). Moreover, priority effects caused by the order of plant arrival were proven to have strong impacts on the belowground parts of plants, thereby influencing the plant community composition (Fukami Reference Fukami2015; Weidlich et al. Reference Weidlich, von Gillhaussen, Max, Delory, Jablonowski, Rascher and Temperton2018). This may have specific implications for the invasion of exotic species, especially when the first exotic plant is mycorrhizal dependent. A meta-analysis showed a positive correlation between the AMF colonization and the growth response in native plants, while this correlation was absent in invasive plants (Bunn et al. Reference Bunn, Ramsey, Lekberg and Van Der Heijden2015). Other studies found that fine-root AMF could cause more positive feedback in the native–exotic plant co-occurrence ecosystems, but the mycorrhizal benefits in the two competitive species were proven to be related to a plant root–based foraging strategy (Herrera et al. Reference Herrera, Poudel and Bokati2013; Hilbig and Allen Reference Hilbig and Allen2015). Nevertheless, AMF induced changes, not only in the relative abundance but also in the community composition of weeds (Jordan et al. Reference Jordan, Zhang and Huerd2000; Veiga et al. Reference Veiga, Jansa, Frossard and Van Der Heijden2011). Root mycorrhizal colonization is commonly associated with soil AM fungal spore density, while these two indicators are affected by the soil nutrient status (e.g., N and P) and other soil properties (e.g., moisture) (Bunn et al. Reference Bunn, Lekberg, Gallagher, Rosendahl and Ramsey2014; Daisog et al. Reference Daisog, Sbrana, Cristani, Moonen, Giovannetti and Barberi2012; Liu et al. Reference Liu, Zheng, He and Feng2009; Wang et al. Reference Wang, Qiu, Yang, Hu, Tam and Xin2010, Reference Wang, Li, Bao, Bjorn, Li and Olsson2016). Moreover, season and phenology are important factors that influence both mycorrhizal colonization and soil sporulation (Santos-Gonzalez et al. Reference Santos-Gonzalez, Finlay and Tehler2007; Wang et al. Reference Wang, Li, Li, Bjorn, Rosendahl, Olsson, Li and Fu2015a; Welsh et al. Reference Welsh, Burke, Hamerlynck and Hahn2010). It is still unknown how AMF balance the relationships of root infection, soil property, soil AMF community, and spore density in response to the changed invasion status during the invasive process of exotic weeds (Bunn et al. Reference Bunn, Lekberg, Gallagher, Rosendahl and Ramsey2014).
Weed invasion commonly occurs in modestly managed lawns. Moreover, except for lawn weeds, a certain number of turfgrass species could form mycorrhizal symbioses in undisturbed natural conditions (Koske et al. Reference Koske, Gemma and Jackson1997). However, our knowledge about the mechanisms of weed invasion and distribution and the role of AMF in infecting lawn weeds and native turfgrass plants is still limited (Tanaka et al. Reference Tanaka, Miura and Tominaga2010; Vogelsang et al. Reference Vogelsang, Reynolds and Bever2006; Wu et al., Reference Wu, Sun, Wang, Xin, Ye and Peng2011). Furthermore, several questions remain unresolved, especially regarding co-occurring communities: (1) Is the mycorrhizal infection more prevalent in exotic than in native species during the invasion process? (2) Does the relationship between the root mycorrhizal colonization and the soil properties show interspecific (native and invasive) differences? (3) How do the soil AMF community and the AM fungal spore density change in response to the increasing invasion pressure?
To answer these questions, a four-season investigation in a coexisting turfgrass–weed lawn was conducted in 2012. The lawn initially was established with Zoysia tenuifolia Willd. ex Thiele (Poaceae) in 2000 but gradually became occupied by a competitive exotic weed species, threeflower beggarweed [Desmodium triflorum (L.) DC.] (Fabaceae), after approximately 12 yr of modest management. Five coverage levels of D. triflorum, ranging from level 1 to level 5, with five plots of each as replications, were set based on Braun-Blanquet results (Wikum and Shanholtzer Reference Wikum and Shanholtzer1978). Plants of the two species and soils that supported the co-occurrence were collected simultaneously for the determination of (1) root AMF colonizations of the two species, (2) soil AM fungal spore densities, (3) soil properties, and (4) soil AMF communities under different coverage levels of D. triflorum and in different seasons. The overall goal of this study was to identify the potential mechanisms of AMF in helping D. triflorum to spread successfully in the Z. tenuifolia lawn from plant (root mycorrhizal colonization), soil (physiochemical property), and microbial (soil AMF community and AM fungal spore density) aspects.
Materials and Methods
Site Description
The studied lawn is located on the Zhuhai Campus of Sun Yat-sen University, Zhuhai City (21°48′N to 22°27′N, 113°03′E to 114°19′E), Guangdong Province, China. The climate of Zhuhai is characterized as subtropical oceanic, with an average annual temperature of 22.3 C and a minimum temperature of 2.5 C during the year. The rainfall is abundant but is unevenly distributed throughout the year, with an annual precipitation of 1,700 to 2,200 mm. The rainy season runs from April to September and accounts for 84% of the total annual precipitation. The lawn was established in 2000 as a Z. tenuifolia monoculture. The soil under the turf was an artificial mix of materials often prepared for turf areas. Moreover, the lawn was managed modestly after it was established, with occasional mowing and irrigation, infrequent dethatching, and no fertilization or liming. During approximately 12 yr of management, plants of many weed species (Supplementary Table S1) invaded and established in the original Z. tenuifolia lawn. In particular, D. triflorum became the most aggressive species among these exotic weeds. Due to minimal weeding activity and the height of the D. triflorum plants (usually shorter than Z. tenuifolia plants), the spread of D. triflorum increased year by year compared with previous observations of the lawn (Supplementary Figure S1). Desmodium triflorum is a perennial herb that is widely distributed in tropical and subtropical regions of China and commonly grows in patches in natural environments, with a natural growth height of 1.5 to 2.5 cm. Desmodium triflorum also has a well-developed root system, including a taproot (approximately 82 cm for the ripe taproot), a few subroots, and many fibers growing on the taproot (Ma and Yang Reference Ma and Yang2002; Ma et al. Reference Ma, Yang, Chang, Tang and Huang2003). The stem organs of the plant include the erect stems and the stolons. The stolons originate from the mother plant and grow radially, while the interwoven growth of the stolons increases the distribution of this plant (Ma and Yang Reference Ma and Yang2002; Ma et al. Reference Ma, Yang, Chang, Tang and Huang2003). For a better understanding the spread of D. triflorum in the Z. tenuifolia lawn, coverage was used to describe the quantitative characteristics of D. triflorum in the native plant–exotic weed community. Moreover, the Braun-Blanquet coverage classification, one of the most widely used methods for characterizing the coverage of a specific species in plant communities, was applied in this study. The modified Braun-Blanquet coverage classification includes five levels: level 1 (coverage < 5%), level 2 (5% < coverage < 25%), level 3 (25% < coverage < 50%), level 4 (50% < coverage < 75%), and level 5 (75% < coverage < 100%) (Wikum and Shanholtzer Reference Wikum and Shanholtzer1978). In the studied lawn, D. triflorum and Z. tenuifolia were growing together, while other weeds represented only very small coverage in the lawn. Therefore, D. triflorum coverage in different areas of the lawn could represent different degrees of D. triflorum spread. Based on the definition of the Braun-Blanquet coverage classification, the spreading status of D. triflorum plants in the lawn was artificially divided into five levels: level 1, level 2, level 3, level 4, and level 5, corresponding to a coverage of <5%, 5% to 25%, 25% to 50%, 50% to 75%, and 75% to 100% for D. triflorum, respectively. The change of the coverage from level 1 to level 5 indicated an increase in the plant density of D. triflorum but a decrease in the plant density of Z. tenuifolia and other weeds in the lawn (Table 1).
Table 1. Definition of the coverage levels of Desmodium triflorum based on the Braun-Blanquet coverage classification (modified method from Wikum and Shanholtzer Reference Wikum and Shanholtzer1978) and the corresponding coverage of Zoysia tenuifolia and other weed species in each level.

Sample Collection
Plant and soil samples were collected for each coverage level of D. triflorum in April, July, September, and December of 2012, corresponding to spring, summer, autumn, and winter, respectively. For each coverage level of D. triflorum in a certain season, five 1 m by 1 m replicated plots, with a randomly selected 20 cm by 20 cm smaller plot in each, were chosen as representatives of that level in the lawn. Moreover, the four seasonal samples were collected from different smaller plots of the same 1 m by 1 m plot, and the smaller plots were marked using ropes and tags after sample collection to avoid resampling an area in the following season. An intact sample, including all the growing plants (Z. tenuifolia, D. triflorum, and other weed species) and the soil below the plants (from 0- to 10-cm deep), was collected from each smaller plot using a shovel. In this way, the aboveground parts (e.g., stems, leaves, and flowers) and the belowground parts (roots) of all the plants were still connected biologically, and only the roots clearly attached to the target plants were collected and used as root samples. The holes in the smaller plots were back filled after sample collections using 0 to 10 cm of soils collected from a nearby site outside the 1 m by 1 m plots. After collection, the plants and soil for each intact sample were separated into a plant sample and a soil sample. Within each plant sample, Z. tenuifolia and D. triflorum (intact plants, including aboveground and belowground parts) were carefully separated for subsequent root dyeing and AMF colonization studies. The soil samples were air-dried and prepared for AM fungal spore density quantification, physiochemical property determination, and mycorrhizal fungal community analyses.
Mycorrhizal Colonization and Spore Density Determination
Fine roots of Z. tenuifolia and D. triflorum were thoroughly washed and cut into 1-cm-long segments. The root segments were stained using the 10% KOH clearing–Trypan blue dyeing method (Philips and Hayman Reference Philips and Hayman1970) and were prepared for microscopic observation (Nikon, Eclipse E400, Tokyo, Japan). The mycorrhizal structures, mainly entry points, hyphae, and vesicles were observed under an optical microscope at 400× magnification. The root mycorrhizal colonization of the two species was then determined and calculated using the grid counting method (Giovannetti and Mosse Reference Giovannetti and Mosse1980). The AM fungal spores from each air-dried soil sample were sieved using the wet sieving-sucrose centrifugation method (An et al. Reference An, Hendrix, Hershman and Henson1990) and then were observed under a dissecting microscope (Zeiss, Stemi DV4, Oberkochen, Germany). The occurrence of AM fungal spores was recorded during microscopic observation by using a counter. The spore density was then calculated and expressed in units per gram of dry soil.
Soil Physiochemical Property Determination
The soil electrical conductivity (EC) was determined in the centrifuged supernatant of a soil–water mixture (200 g L–1) by using a conductivity meter (DDS-307, Fangzhou, Chengdu, China). The soil pH was determined in a mixture of soil–water (400 g L–1) by using a portable pH detector (PHS-3C, Leici, Shanghai, China), while the total organic C (TOC) was determined by the H2SO4-K2Cr2O7 heating method. The soil available phosphorus (AP) was determined by using the 0.05 M HCl-1/2 H2SO4 method, while the total phosphorus (TP) was determined by using the HClO4-H2SO4 method. The soil available nitrogen (AN) and total nitrogen (TN) (digested with H2SO4) were determined by titration of the distillates after Kjeldahl sample preparation and analyses. All determinations were performed using standard methods (Bao Reference Bao2000; Lu Reference Lu2000).
Molecular AMF Identification
One gram of soil was taken from each air-dried soil sample and mixed into a composite sample with soils at the same coverage level of D. triflorum, that is, every composite sample was a mixture of soils from four seasons with the same D. triflorum coverage levels. Thus, five composite soil samples were prepared to represent corresponding D. triflorum coverage levels for subsequent AMF community analyses. The total DNA of the composite soil samples was extracted using the PowerSoil DNA Isolation Kit (MoBio, Anbiosci Tech, Carlsbad, CA) according to the manufacturer’s instructions. The extracted DNA samples were then checked for quantity and quality using an ultramicrospectrophotometer (NanoDrop 2000, NanoDrop Technologies, Wilmington, DE) and were also checked for integrity using 1% agar gel electrophoresis (5 V cm−1, 30 min). A PCR was then performed by using fungi universal primers (ITS1F-CTTGGTCATTTAGAGGAAGTAA/ITS2-2043R-GCTGCGTTCTTCATCGATGC) (De Beeck et al. Reference De Beeck, Lievens, Busschaert, Declerck, Vangronsveld and Colpaert2014). Reactions were performed with a 60-ng template (extracted DNA), 1 μl of forward and reverse primers (10 μM), and 25 μl of premix taq (EX Taq™ Version 2.0 plus dye, Takara, Tokyo, Japan) in a final reaction volume of 50 μl. The PCR program was 94 C for 5 min, followed by 30 cycles (94 C for 30 s, 52 C for 30 s, and 72 C for 30 s), and a final extension step of 72 C for 10 min. DNA sequences were analyzed with the Illumina Miseq sequencing platform (Magigen Biotech Company, Guangzhou, China) and were compared with the public database using the BLAST sequence similarity search tool (GenBank). All AMF sequences were grouped into operational taxonomic units (OTUs) with sequence similarities ≧97%, using the Mothur program (Schloss et al. Reference Schloss, Westcott, Ryabin, Hall, Hartmann, Hollister, Lesniewski, Oakley, Parks, Robinson, Sahl, Stres, Thallinger, Van Horn and Weber2009). The relative abundance of each AMF OTU was calculated after learning the relative abundance of the Glomeromycota within the whole soil fungal community.
Statistical Analyses
Two-way ANOVA followed by LSD tests were conducted to compare the soil properties, root mycorrhizal colonizations, and spore densities within D. triflorum coverage levels and seasons. Three-way ANOVA followed by mean separation using Fisher’s protected LSD was conducted to compare the root colonizations within different D. triflorum coverage levels and seasons and between species (Z. tenuifolia and D. triflorum). Simultaneously, Pearson correlation analyses were carried out to explore the relationships among the total colonization (TC), hyphal colonization (HC), and vesicular colonization (VC) of Z. tenuifolia/D. triflorum roots and the interactive relationship between the two species’ root mycorrhizal colonizations. Correlation analyses were also performed to test the relationships among the mycorrhizal colonizations, spore densities, and soil properties in different D. triflorum coverage levels. All of the statistical analyses were performed using SPSS v. 17.0 (IBM, Armonk, NY, USA). The statistically significant difference was analyzed at the P < 0.05 level, unless otherwise stated.
Results and Discussion
Dynamics of the Soil Properties within Seasons and Desmodium Triflorum Coverage Levels
Except for the AN concentration, concentrations of other soil property indicators, including the pH, EC, TOC, AP, TP, and TN, showed significant differences (P < 0.001) among the four seasons during the 1-yr investigation (Figure 1). The lawn soil underwent an acidification process, with the pH decreasing from 5.8 in spring to 4.7 in winter. A decrease from spring to summer followed by an increase from summer to winter was observed in the soil TOC, AP, and TP concentrations. Significant differences among the five coverage levels of D. triflorum were observed in the pH and TOC, TP, and TN concentrations (Figure 1A, C, E, and G). Moreover, the level 5 soil with the highest D. triflorum coverage in the lawn showed relatively higher pH, EC, and TOC, TP, and TN concentrations compared with soils of the other coverage levels (Figure 1B, C, E, and G). In contrast, the level 1 soil with the lowest D. triflorum coverage showed relatively lower TOC, TP, and TN concentrations in summer and autumn in comparison with soils of the other coverage levels (Figure 1C, E, and G).
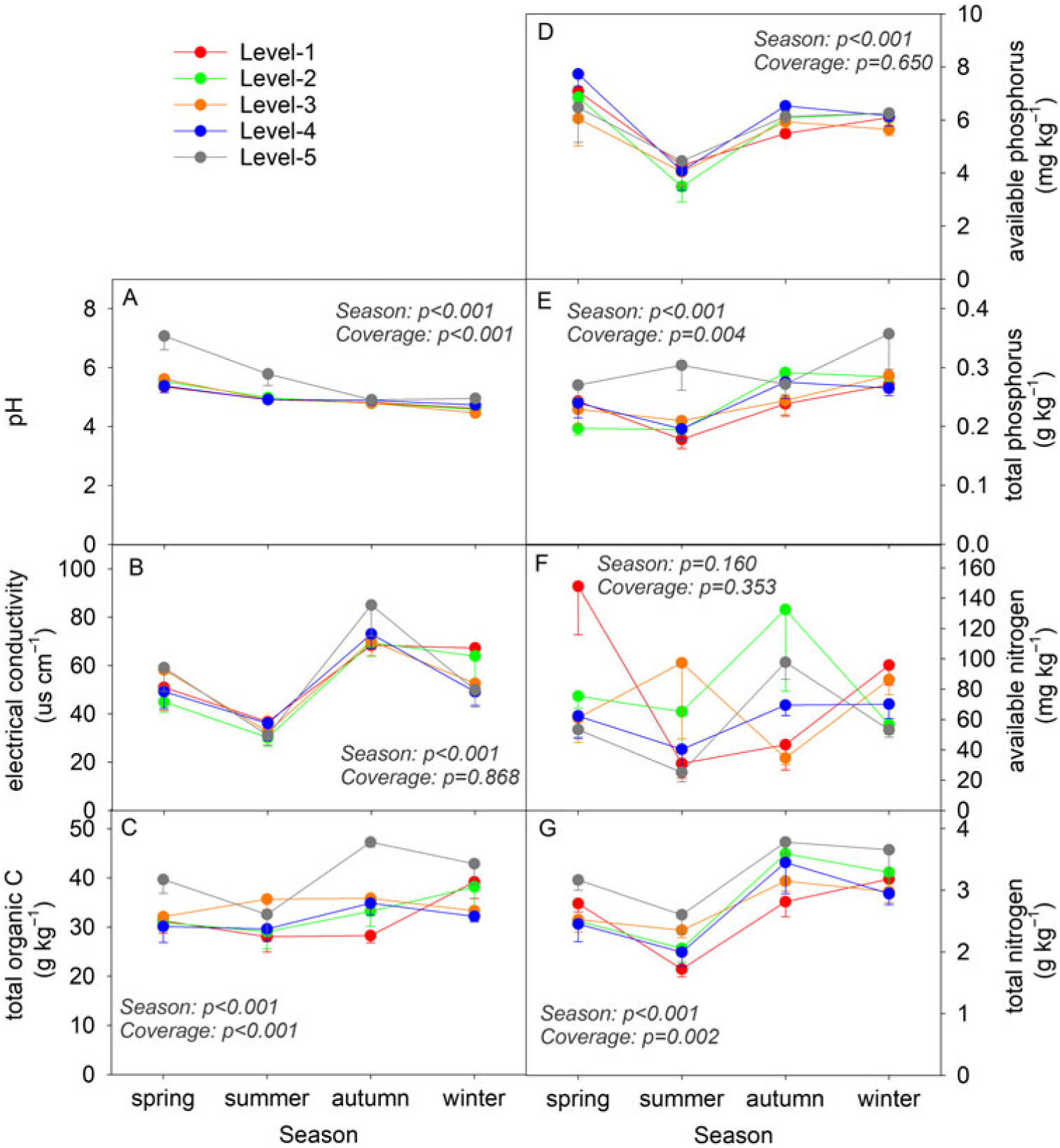
Figure 1. Dynamics of the soil physiochemical properties (average ± SE, n = 5) within different Desmodium triflorum coverage levels and seasons. “Season” and “Coverage” indicate ANOVA results of each indicator among seasons and D. triflorum coverage levels, respectively. Level 1, level 2, level 3, level 4, and level 5 indicate the coverage levels of D. triflorum in the Zoysia tenuifolia lawn, respectively, in this and all following figures.
Characteristics of Plant Root Mycorrhizal Colonizations within Seasons and Desmodium Triflorum Coverage Levels
Hyphae and vesicle structures were commonly present, while the arbuscular structure was not observed in the plant roots of both species (Supplementary Figure S2). The TC, HC, and VC of D. triflorum were significantly higher than those of Z. tenuifolia (P < 0.001) (Figure 2), implying that as the invasive species in the lawn, D. triflorum had a greater advantage in mycorrhizal infection than the native species, Z. tenuifolia. Moreover, the mycorrhizal colonizations of both Z. tenuifolia and D. triflorum showed consistently changing trends within seasons, that is, an increase from spring to autumn followed by a decrease to winter (Figure 2), coinciding with seasonal dynamics of the AM fungal infection in the two species. During the whole investigation period, the TC of D. triflorum was in the range of 10.0% to 48.8%, while that of Z. tenuifolia was in the range of 5.2% to 28.0% (Figure 2A and D). The TC of Z. tenuifolia showed a “decrease–increase–decrease” variation tendency with the coverage level of the D. triflorum increase in the lawn (from level 1 to level 5) in all four seasons (Figure 2A). The TC of D. triflorum in spring and winter showed similar change trends, with the coverage level of D. triflorum increasing in the lawn, while the TC of D. triflorum showed an opposite trend (increase–decrease–increase) in autumn but an obviously decreasing trend in summer (Figure 1D). The HC of Z. tenuifolia was in the range of 4.3% to 24.5%, which was also lower than that of D. triflorum (5.1% to 47.1%) (Figure 2B and E). With the coverage level of D. triflorum increasing in the lawn, the HC of Z. tenuifolia showed a change trend similar to that of the TC, while the HC of D. triflorum showed a decreasing trend in summer but an increasing trend in autumn (Figure 2B and E). The VC of Z. tenuifolia was in the range of 1.5% to 15.6%, while that of D. triflorum was in the range of 4.7% to 28.9%, respectively (Figure 2C and F). In addition to the significant seasonal variances of VC, a significant coverage by season interaction effect was also observed in the VC of the two plants (Figure 2C and F).

Figure 2. Dynamics of the total, hyphal, and vesicular colonizations of Zoysia tenuifolia and Desmodium triflorum among different D. triflorum coverage levels and seasons. “Season,” “Coverage,” and “Species” indicate ANOVA results of each indicator among seasons and D. triflorum coverage levels and between the two plants, respectively.
The seasonal dynamics of plant root mycorrhizal colonization are thought to be linked to soil properties and spore density (Verbruggen et al. Reference Verbruggen, Van Der Heijden, Rillig and Kiers2013) and vary among plant species, mycorrhizal structures, and AM fungal communities (Bencherif et al. Reference Bencherif, Boutekrabt, Dalpe and Sahraoui2016; Smith Reference Smith1980; Wang et al. Reference Wang, Li, Li, Qiu, Li and Xin2015b). For example, root colonization of saltmeadow cordgrass [Spartina patens (Aiton) Muhl.] was high in the growth period and decreased in the reproductive and aging periods, showing growth-period specificity (Welsh et al. Reference Welsh, Burke, Hamerlynck and Hahn2010). Therefore, the seasonal dynamics of the two plants’ root colonizations in the present study could be explained by the growth season aspects: the lower soil temperature, dormant AMF spores, and weakly growing plants in the early spring induced a low root colonization; the increased soil temperature from spring to summer benefited not only spore germination and mycorrhizal formation but also plant photosynthesis and root growth, which further promoted mycorrhizal infection in the roots of the two plants, while the root colonizations were highest in the late growth period (autumn); furthermore, the decreased soil temperature in autumn and winter depressed plant growth and mycorrhizal infection, therefore inducing a decline of root colonization (Figure 2). Moreover, the commonly observed hyphae and vesicle structures and the rarely seen arbuscular structure of the two plants might be related to the traits of the two host plants and the host specificity of soil AMF taxa (Opik and Moora Reference Opik and Moora2012; Verbruggen et al. Reference Verbruggen, Van Der Heijden, Rillig and Kiers2013).
The D. triflorum roots showed significantly higher mycorrhizal colonizations (total, hyphal and vesicular) compared with Z. tenuifolia roots in all five coverage levels of D. triflorum (Figure 3). This is contrary to the prevailing viewpoint that exotic plants with lower dependences on AMF symbiosis have greater chances to invade a new community compared with those with strong AMF associations (Endresz et al. Reference Endresz, Somodi and Kalapos2013; Pringle et al. Reference Pringle, Bever, Gardes, Parrent, Rillig and Klironomos2009). Nevertheless, the more intense AMF infections in the invasive species (D. triflorum) than in the native species (Z. tenuifolia) in the present study are supported by other studies of symbiotic communities (Bunn et al. Reference Bunn, Ramsey, Lekberg and Van Der Heijden2015; Greipsson and DiTommaso Reference Greipsson and DiTommaso2006). This might be partly due to the fact that D. triflorum is a legume. Previous studies have demonstrated that the mycorrhization in legume roots facilitates the nodule symbiotic efficiency and further promotes N2 fixation and plant growth (Bournaud et al. Reference Bournaud, James, de Faria, Lebrun, Melkonian, Duponnois, Tisseyre, Moulin and Prin2018; de Oliveira et al. Reference de Oliveira, Jesus, Lisboa, Berbara and de Faria2017). In a symbiotic community with a leguminous species and a non-leguminous species, the non-leguminous plant would receive multiple benefits owing to the nutrient transfer via extraradical hyphae of the neighboring leguminous plants (Temperton et al. Reference Temperton, Mwangi, Scherer-Lorenzen, Schmid and Buchmann2007). Considering that D. triflorum was much more dominant than the other weed species in the lawn, we speculated that D. triflorum might be the first species invading the lawn and then inhibited the development of the following species because of the priority effects aboveground and belowground (Weidlich et al. Reference Weidlich, von Gillhaussen, Delory, Blossfeld, Poorter and Temperton2017, Reference Weidlich, von Gillhaussen, Max, Delory, Jablonowski, Rascher and Temperton2018). Thus, the priority effects and the superior competitive ability of D. triflorum arguably contributed to a high root mycorrhizal colonization of its own, and that D. triflorum overcame Z. tenuifolia as well as other weed species in the lawn. Furthermore, the AM fungal colonization of native plants would decrease when grown with/after invasive plants (Bunn et al. Reference Bunn, Ramsey, Lekberg and Van Der Heijden2015; Stinson et al. Reference Stinson, Campbell, Powell, Wolfe, Callaway, Thelen, Hallett, Prati and Klironomos2006). But this is inconsistent with our results, because there was no signal demonstrating a significant decline in the root mycorrhizal colonizations of Z. tenuifolia with the coverage level of D. triflorum increase in the lawn, possibly because Z. tenuifolia is also AMF dependent and could form mycorrhizal structures of its own. On the other hand, the relatively higher mycorrhizal infections in D. triflorum imply that D. triflorum may exploit the AM symbiosis more efficiently than the native species Z. tenuifolia, absorbing soil nutrients such as phosphorus from a wider area (Walling and Zabinski Reference Walling and Zabinski2004). In addition in the symbiotic community dominated by Z. tenuifolia and D. triflorum, the long taproots of the D. triflorum plants (Ma and Yang Reference Ma and Yang2002) could reach much deeper in soil than those of Z. tenuifolia, facilitating additional nutrient and water uptake via extraradical hyphae. However, to confirm this, additional data, including plant/soil nutrient concentrations, extraradical hyphae of the two species, and the relationships of the root mycorrhizal colonizations and plant growth responses are needed. Therefore, D. triflorum’s more efficient symbiosis (reflected in the number of mycorrhizal infections) and greater nutrient/water absorption might contribute to D. triflorum outcompeting Z. tenuifolia, leading to its successful spread in the lawn.

Figure 3. Dynamics of the soil arbuscular mycorrhizal fungal spore density within Desmodium triflorum coverage levels and seasons.
Characteristics of the Soil AMF Spore Densities within Seasons and Desmodium Triflorum Coverage Levels
The soil AM fungal spore density varied significantly (P < 0.05) within not only the four seasons but among the five D. triflorum coverage levels (Figure 3). The soil spore density was low in spring, increased in summer, reached its highest level in autumn, and then decreased in winter. Similar change trends were observed in spring and summer as the coverage level of D. triflorum increased in the lawn; that is, the AM fungal spore density was lower in the level 1 soil and increased in the level 2 soil, but decreased in the soils of higher D. triflorum coverage levels (levels 3, 4, and 5) (Figure 3). Whereas the level 1 and level 2 soils in autumn and the level 2, level 3 and level 4 soils in winter showed relatively higher AM fungal spore densities than the soils of the other coverage levels (Figure 3). Previous studies suggested that the seasonal dynamics of the soil AM fungal spore density differed depending upon soil types and host plant species (Cuenca and Lovera Reference Cuenca and Lovera2010; Liu et al. Reference Liu, Li, Diao, Li and Lin2013; Verbruggen et al. Reference Verbruggen, Van Der Heijden, Rillig and Kiers2013; Wang et al. Reference Wang, Li, Li, Qiu, Li and Xin2015b; Xin et al. Reference Xin, Ye, Wu, Wang and Sugawara2012). In the Z. tenuifolia–D. triflorum co-occurring lawn soil, the seasonal change trends of the AM fungal spore density are consistent with the seasonal dynamics of the spore densities in the rhizosphere soils of three turfgrass plants (Koske et al. Reference Koske, Gemma and Jackson1997). These special dynamics might be partially due to the soil temperature dependency of AM fungal spore germination and growth (Xin et al. Reference Xin, Ye, Wu, Wang and Sugawara2012): after a cold winter, a lower number of active spores remained in the soil. The AM fungal spores began to germinate and promote infections when the climate became warmer and wetter in spring. Moreover, the root mycorrhizal infection contributed to sporulation, thus inducing an increase of the spore density from spring to summer. Therefore, the root mycorrhizal colonizations were highest in autumn, while the total number of soil spores reached a peak at the same time (Figures 2 and 3). Decreasing soil temperature in winter led to a decline in the germination rate of AM fungal spores that had been produced in summer and autumn, thus inducing large amounts of spore accumulation in soils. On the other hand, the weakened plant photosynthesis in winter might limit the nutrient transport between plants and symbiotic AM fungi (van der Heijden et al. Reference van der Heijden, Martin, Selosse and Sanders2015), thus inducing nutrient malnutrition in fungi, leading to greater sporulation and higher AM fungal spore density in the soil (Figure 3).
Correlations of Soil Properties, Root Mycorrhizal Colonizations, and Spore Densities in Different Desmodium Triflorum Coverage Levels
According to Pearson correlation analyses, the relationships among the soil properties, spore density, and root colonization of the two plants exhibited different trends within the five D. triflorum coverage levels. Pairwise significant positive correlations were observed among the TC, HC, and VC of Z. tenuifolia and among those of D. triflorum in all of the five D. triflorum coverage levels (Figure 4; Supplementary Table S2), which showed a mutual influence of the hyphae and the vesicle after mycorrhizal infection. However, the correlations between the mycorrhizal colonizations of D. triflorum and those of Z. tenuifolia were considerably different within the five D. triflorum coverage levels. Positive correlations were observed between the root colonizations (TC, HC, and VC) of Z. tenuifolia and the HC of D. triflorum in the soil of level 1, which had the lowest spreading status of D. triflorum (Figure 4). Both the HC and TC of Z. tenuifolia showed significant positive correlations with the root colonizations (TC, HC, and VC) of D. triflorum in the level 2 soil. Subsequently, both the HC and TC of Z. tenuifolia showed significant positive correlations with the HC and TC but not the VC of D. triflorum in the level 3 soil. Finally, pairwise significant positive correlations were observed among the six root colonization indicators (TC, HC, and VC of Z. tenuifolia, and TC, HC, and VC of D. triflorum) in the level 4 and level 5 soils (Figure 4), thus showing an interactive relationship between the mycorrhizal colonizations of the two plants. The positive correlations between the root colonizations of native species (Z. tenuifolia) and those of the invasive species (D. triflorum) (Figure 4) implied that a mutualistic relationship based on the mycorrhizal infections of the two species was established immediately after the spread of D. triflorum and the formation coexistence within the lawn. Moreover, the significantly positive correlations between the HC/VC of Z. tenuifolia and the HC of D. triflorum in the level 1 soil presumably imply that D. triflorum was colonized by the extraradical mycelia of Z. tenuifolia because of the close root-to-root contact of the two plants (Enkhtuya et al. Reference Enkhtuya, Poschl and Vosatka2005; Sykorova et al. Reference Sykorova, Rydlova and Vosatka2003); this pathway of mycorrhizal infection still needs more evidence (micrographic observations). Furthermore, the root colonizations of the two plants were more significantly correlated with each other, especially in the higher coverage level soils (Figure 4), which reflected the ability of AM fungal mycelia to establish a potentially large network interconnecting with different plants in the coexistent community (Giovannetti et al. Reference Giovannetti, Sbrana, Avio and Strani2004; Opik and Moora Reference Opik and Moora2012). Therefore, the exact role of extraradical mycelia in the coexistence system needs to be further investigated to understand the mycorrhizal function during the invasion process of D. triflorum.

Figure 4. Correlations among the root mycorrhizal colonizations, arbuscular mycorrhizal fungal spore densities (“AMF spore density”), and soil properties in different coverage levels of Desmodium triflorum. ZTC, ZHC, and ZVC in light-green circles indicate the total colonization (TC), hyphal colonization (HC), and vesicular colonization (VC) of Zoysia tenuifolia, respectively. DTC, DHC, and DVC in light-red circles indicate the TC, HC, and VC of D. triflorum, respectively. Green lines and green-colored numbers indicate significant correlations between the colonization indicators of Z. tenuifolia and corresponding correlation coefficients, respectively. Red lines and red-colored numbers indicate significant correlations between the colonization indicators of Z. tenuifolia and corresponding correlation coefficients, respectively. Dark-green double arrows and dark-green numbers indicate the correlations between the colonizations of Z. tenuifolia and those of D. triflorum and corresponding correlation coefficients, respectively. Light-blue double arrows and light-blue numbers indicate the correlations between the spore densities and soil properties/root colonizations and corresponding correlation coefficients, respectively. Dark-yellow double arrows and dark-yellow numbers indicate the correlations between the soil properties and root colonizations and corresponding correlation coefficients, respectively. Correlation is significant at: *P < 0.05; **P < 0.01; ***P < 0.001. The minus sign indicates a negative correlation. Insignificant correlations are not shown.
The correlations between the soil AM fungal spore densities and the root mycorrhizal colonizations also differed within the five D. triflorum coverage levels (Figure 4; Supplementary Table S2). The soil AMF spore density was positively correlated with the TC, HC, and VC of Z. tenuifolia in the level 1 soil, thus showing a possible dominance of the AMF-infected Z. tenuifolia roots for sporulation. However, the correlation between the spore density and the root colonization was not significant in the level 2 and level 3 soils. The AMF spore density was significantly correlated with the TC of D. triflorum in the level 4 soil and with all three colonizations of D. triflorum in the level 5 soil (Figure 4), possibly explaining the various relationships between the AM fungal spore density and the root mycorrhizal colonization at different coverage levels of the D. triflorum plants. Root AMF infection could benefit sporulation (Soteras et al. Reference Soteras, Becerra, Cofre, Bartoloni and Cabello2012), the dominant contributor of soil spores changed with the development of D. triflorum in the lawn. At the early stage (level 1) of the D. triflorum spreading process, the hyphae and vesicles in the root of Z. tenuifolia contributed to the formation of AM fungal spores. With the development of D. triflorum in the lawn, the hyphal and vesicular structures in the roots of D. triflorum gradually contributed to sporulation; therefore, soil AM fungal spores might be a result of sporulation from both species in level 2 and level 3 soils. When D. triflorum dominated in the lawn (level 4, level 5), the AM fungal spores were predominantly produced by the mycorrhizal structures of D. triflorum, thus inducing significant correlations between the spore densities and the root colonizations of D. triflorum (Figure 4). In the native–invasive relationships, the host preference of AMF might lead not only to different AMF communities in the roots of the co-occurring plants but also to a change in the AMF community composition in the soil of native species (Hawkes et al. Reference Hawkes, Belnap, D’Antonio and Firestone2006; Klironomos Reference Klironomos2003; Vandenkoornhuyse et al. Reference Vandenkoornhuyse, Ridgway, Watson, Fitter and Young2003; Zhang et al. Reference Zhang, Yang, Tang, Yang, Hu and Chen2010). The AM fungal spore composition, therefore, might also be affected by the altered soil, as well as the root mycorrhizal fungal groups, because of exotic species invasion (Zhang et al. Reference Zhang, Yang, Tang, Yang, Hu and Chen2010). Therefore, we argue that the spread of D. triflorum gradually changed the AM fungal community composition of the native soil to facilitate the invasion and its own host–fungus specificity. To verify this speculation, the AMF species in the roots of the two plants and the AM fungal spore groups in soils that support the coexistence system need to be identified in follow-up analyses.
The correlations between the soil properties and the spore densities, as well as the soil properties and root colonizations of the native (Z. tenuifolia) and invasive (D. triflorum) plants indicated a difference among the five coverage levels of D. triflorum (Figure 4; Supplementary Table S2). Except for the level 1 soil, all of the D. triflorum coverage level soils showed a significant negative correlation between pH and spore density (Figure 4), which indicated that the more acidic soil supported a higher number of spores in the soil. The soil AN was significantly correlated with the TC of Z. tenuifolia in the level 1 soil, the HC and VC of Z. tenuifolia in the level 3 soil, and not only the TC of Z. tenuifolia but also all the colonization indicators of D. triflorum in the level 4 soil (Figure 4). Both the EC and TN showed significant correlations with the TC and VC of D. triflorum in the level 2 soil (Figure 4). The relationship among the soil properties, root mycorrhizal colonizations, and spore densities was most significant in the level 4 soil, where growth of D. triflorum plants was stimulated more than Z. tenuifolia plants: the TP and EC showed significant correlations with the root colonizations of both Z. tenuifolia and D. triflorum, while the TN was significantly correlated with the colonizations of only Z. tenuifolia (Figure 4). At the highest coverage level of D. triflorum (level 5), the soil TOC and AN showed significant correlations with the root colonizations of both plants, while the pH was significantly correlated with all of the colonization indicators of only D. triflorum, thus showing different relationships among the soil properties and mycorrhizal colonizations during the development of D. triflorum in the Z. tenuifolia lawn. The positive correlations of the mycorrhizal colonizations and the soil N/P (AN, TN, TP) concentrations potentially indicate the nutritional needs of mycorrhizae after formation (Della Monica et al. Reference Della Monica, Saparrat, Godeas and Scervino2015; Deng et al. Reference Deng, Feng, Chen and Zou2017; Jiang et al. Reference Jiang, Liu, Luo, Qin, Johnson, Opik, Vasar, Chai, Zhou, Mao, Du, An and Feng2018). However, the nutrient (N, P) concentrations of the two plants’ tissues are further needed for a better understanding of the relationships between soil nutrients and host plants via the mediation of the root mycorrhizal structures. Moreover, the soil property–root colonization correlation was most significant in the level 4 soil (Figure 4), indicating a complex relationship between the soil and AMF in this stage. Except for the close linkage with the root mycorrhizal colonization, the AMF sporulation was suggested to be strongly influenced by other soil traits, such as soil depth and physiochemical properties (Cuenca and Lovera Reference Cuenca and Lovera2010; Liu et al. Reference Liu, Li, Diao, Li and Lin2013). However, unlike the previous finding of a positive soil pH–spore relationship (Verbruggen et al. Reference Verbruggen, Van Der Heijden, Rillig and Kiers2013), in our study, except in the level 1 soil, the spore density was negatively correlated with the pH (Figure 4). This inconsistency might be due to the differences between the studied plant species and the native soil properties. Thus, the lower soil pH would benefit AMF sporulation in the soil.
Dynamics of the Soil AMF Community within Different Desmodium Triflorum Coverage Levels
Except for the level 3 soil, the four other D. triflorum coverage level soils showed that Ascomycota was the dominant fungal phylum, with a relative abundance of more than 50% of the entire fungal community (Supplementary Table S3). Basidiomycota was the second most-abundant phylum in soil, with a relative abundance of 5.6% to 35.4%, whereas Glomeromycota, the fungal phylum to which AMF belongs, only occupied a relative abundance of 0.4% to 1.6% in the whole fungal community (Supplementary Table S3). Moreover, AMF OTUs were 18, 16, 28, 17, and 27 in the level 1, level 2, level 3, level 4 and level 5 soils, respectively (Figure 5B). Furthermore, a total of 41 AMF OTUs were observed in the lawn soil, including 6 OTUs in Archaeosporales, 5 OTUs in Diversisporales, 20 OTUs in Glomerales, 1 OTU in Paraglomerales, and 9 other OTUs from unknown orders (Figure 5B). For the AMF community in the five different D. triflorum coverage level soils, Glomerellaceae was dominant, with the highest relative abundance in the level 2 soil (75.9%) and the lowest relative abundance in the level 4 soil (20.0%) (Figure 5A). Opposite results were observed in Claroideoglomeraceae: the highest and the lowest relative abundances were 27.8% in the level 4 soil and 0% in the level 2 soil, respectively. The abundance of Ambisporaceae was the lowest (3.3%) in the level 2 soil but the highest (22.1%) in the level 5 soil. Acaulosporaceae occupied the lowest relative abundance (0.5% to 8.1%) in the five families defined, showing different contributions of AMF species in different families (Figure 5A).

Figure 5. The relative abundance and community composition at the family (A) and species levels (B) of arbuscular mycorrhizal fungi (AMF) in soils of different Desmodium triflorum coverage levels.
Our molecular analysis results showed markedly different lawn soil AMF community compositions among the five D. triflorum coverage levels (Figure 5), presumably due to the different host–fungal specificity of the invasive D. triflorum in comparison to that of Z. tenuifolia (Zhang et al. Reference Zhang, Yang, Tang, Yang, Hu and Chen2010). Except in the level 4 soil, Glomerellaceae was the most abundant in the lawn soil, which implied that both Z. tenuifolia and D. triflorum are easily colonized by the AMF species of this family. The relative abundances of both Ambisporaceae and Acaulosporaceae were the lowest, but that of Glomerellaceae was the highest, while a lack of Claroideoglomeraceae and the lowest number of AMF OTUs were observed in the level 2 soil in comparison to soils of other levels (Figure 5). This reflects that the level 2 soil might have the simplest AMF community composition. In contrast, the relative abundance of Claroideoglomeraceae was the highest, while that of Glomerellaceae was the lowest in the level 4 soil, indicating a most responsive AMF community in the stage when D. triflorum began to become dominant in the lawn. Moreover, the soil AM fungal spore density differed significantly in response to the increased D. triflorum coverage levels (Figure 3). However, this change trend is not in line with previous results that more highly invaded grass plots held more abundant AMF spores than those in the less-invaded plots (Madawala Reference Madawala2014). Geography, microclimate, and soil status might partly contribute to this difference, because AMF occupied two niches simultaneously in the soil–plant connections: the intraradical niche for carbon supply and the bulk soils for survival and development (Helgason et al. Reference Helgason, Feng, Sherlock, Young and Fitter2014).
Possible Mechanisms of AMF During the Spreading Process of Desmodium Triflorum
Based on the results of root mycorrhizal colonizations and correlation analyses, we analyzed the possible role of AMF in the spreading process of D. triflorum (Figure 6). (1) At the beginning of the lawn establishment with only the Z. tenuifolia plants growing but without D. triflorum spreading, the soil AM fungal spores that existed in the soil before the lawn was established, first infected the fine roots of Z. tenuifolia and completed the life cycle on their own. (2) At the first spreading stage (level 1) of D. triflorum, the roots of the two plants came into contact with each other, which induced the external hyphae that originally grew closely on the Z. tenuifolia roots to infect the roots of D. triflorum. The inherent competitive advantages of D. triflorum in not only mycorrhizal symbiosis but also nutrient absorption finally contributed to higher root mycorrhizal colonizations of D. triflorum compared with Z. tenuifolia. However, at this stage, the D. triflorum plants were still not as competitive as the Z. tenuifolia plants. Therefore, the soil AM fungal spores were still predominantly produced by the mycorrhizal structures of the infected Z. tenuifolia roots. (3) Desmodium triflorum continued spreading in the lawn (level 2, level 3). The aboveground and belowground contact of the two plants became more frequent and gradually induced a closer relationship between the AM infections of the two plants (Figure 4). Moreover, the increased numbers of the D. triflorum plants in the lawn and the advantages of D. triflorum in mycorrhizal infections facilitated the contribution of the mycorrhizal structures of the D. triflorum roots to sporulation. Therefore, the soil AM fungal spores were produced by the mycorrhizal structures of both plants, thereby inducing insignificant correlations between the spore densities and the root colonizations of either Z. tenuifolia or D. triflorum (Figure 4). (4) At the late spreading stage (level 4, level 5), the AM infection advantage of D. triflorum as well as the large numbers of the D. triflorum plants finally resulted in the mycorrhizal structures of D. triflorum predominantly contributing to sporulation in the soil, thereby inducing significant correlations between the spore densities and the root colonizations of D. triflorum (Figures 4 and 6). Notably, at the different spreading stages of D. triflorum in the lawn, the soil AM fungal communities varied as a result of the changed contributions of the AMF-infected host plants to sporulation (Figures 5 and 6).
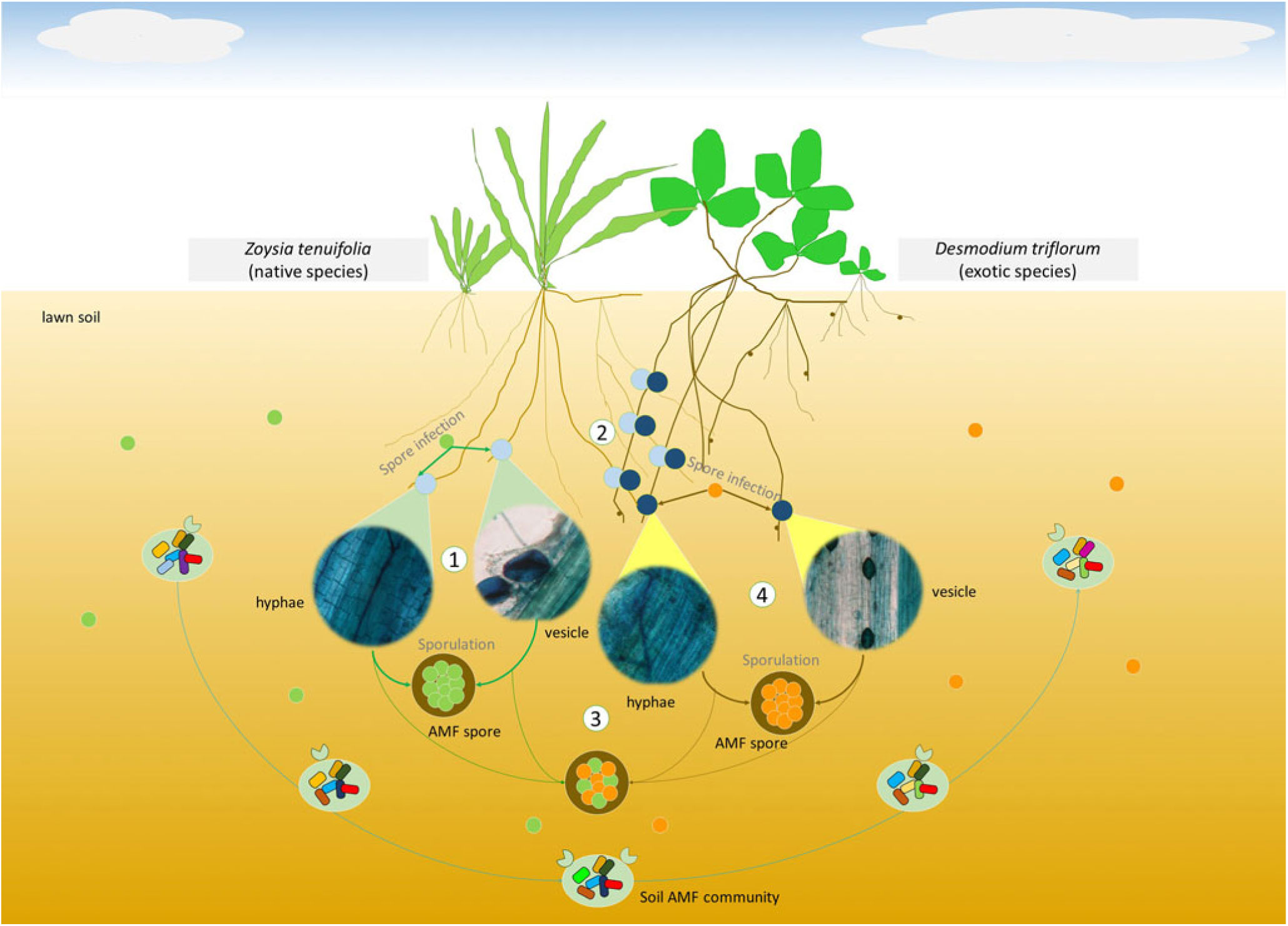
Figure 6. Conceptual framework demonstrating possible mechanisms of soil arbuscular mycorrhizal fungi (AMF) during the spreading process of Desmodium triflorum in the Zoysia tenuifolia lawn. Numbers 1, 2, 3, and 4 indicate different spreading stages of the invasive plant D. triflorum. Corresponding mycorrhizal structures were shown as the four microscopic views. Light-green and medium-yellow circles indicate AM fungal spores predominantly produced by the root mycorrhizal structures of Z. tenuifolia and D. triflorum, respectively. Medium-green and dark-yellow lines indicate the life cycle of spores in Z. tenuifolia plants and in D. triflorum plants, respectively. The AM fungi might influence the spread of D. triflorum by the following steps: (1) the early stage of the lawn’s development with only Z. tenuifolia growing but without D. triflorum present. This occurs at the very beginning of the lawn establishment, and the AM fungal spores that previously existed in the lawn soil first infected the fine roots of Z. tenuifolia and completed the life cycle on their own. (2) The early spreading stage of D. triflorum (level 1). The roots of the two plants come into contact with each other, inducing the external hyphae that originally grow closely on the Z. tenuifolia roots to infect the roots of D. triflorum. The difference between the mycorrhizal infections of the two host plants contributes to higher root mycorrhizal colonizations of D. triflorum compared with Z. tenuifolia. However, at this stage, D. triflorum is not as competitive as Z. tenuifolia in the lawn, although it has advantages in terms of mycorrhizal infections. Therefore, the soil AM fungal spores are still predominantly produced by the mycorrhizal structures of the AMF-infected Z. tenuifolia roots. (3) The intermediate spreading stage of D. triflorum (levels 2 and 3). Desmodium triflorum continues to spread in the lawn. The contact of the two plants becomes more frequent and further induces a much closer relationship between the AM infections of the two plants. The increased D. triflorum plants in the lawn and the advantage of D. triflorum in root mycorrhizal infections facilitate the contribution of the mycorrhizal structures of the D. triflorum roots to sporulation. Thus, in this stage, the soil AM fungal spores were produced by the mycorrhizal structures of both plants, thereby inducing insignificant correlations between the spore densities and the root colonizations of either Z. tenuifolia or D. triflorum. (4) The late spreading stage of D. triflorum (levels 4 and 5). Desmodium triflorum is dominant in the lawn. The large numbers of D. triflorum plants and the AM infection advantage of D. triflorum facilitate AMF sporulation in the soil, thereby inducing significant correlations between the spore densities and the root colonizations of D. triflorum. At the different spreading stages of D. triflorum, the soil AM fungal communities also change as a result of the changed contributions of the AMF-infected host plants to the sporulation.
Previous studies have presented a series of potential AMF functions that help exotic plants succeed in invasion, such as changing the root AM dependency, growth response, and fungal community difference between native and invasive species (Bunn et al. Reference Bunn, Lekberg, Gallagher, Rosendahl and Ramsey2014; Endresz et al. Reference Endresz, Somodi and Kalapos2013; Klironomos Reference Klironomos2002; Lekberg et al. Reference Lekberg, Gibbons, Rosendahl and Ramsey2013; Zhang et al. Reference Zhang, Yang, Tang, Yang, Hu and Chen2010). In the current study, the significantly higher root colonizations of D. triflorum compared with those of Z. tenuifolia might explain the successful invasion of D. triflorum plants to some extent. On the other hand, the investigated soil spores and AM fungal communities in the present study are only representatives of the current spreading status in the lawn, while the life-history strategies of AMF (López-García et al. Reference López-García, Palenzuela, Barea and Azcón-Aguilar2014) during the whole period still remain unclear. Moreover, the resident AMF species in the roots of the two different plant species still remain a mystery but are essential, especially to the initial root colonization in D. triflorum plants because of the priority effects and host specificity of AMF in infecting plant roots (Herrera et al. Reference Herrera, Poudel and Bokati2013; Opik et al. Reference Opik, Metsis, Daniell, Zobel and Moora2009; Santos-Gonzalez et al. Reference Santos-Gonzalez, Finlay and Tehler2007; Weber et al. Reference Weber, King and Aho2015; Werner and Kiers Reference Werner and Kiers2015). Therefore, the future work of this study will focus mainly on the identification of AMF species that colonized the Z. tenuifolia and D. triflorum plant roots and the identification of AMF spore species in the soils where the two plants were growing. It is worth mentioning that we explored the invasion mechanism of D. triflorum from only the mycorrhizal infection aspects. Whether AMF induced differences by influencing the plant growth or root morphology constructions of the two plants (Enkhtuya et al. Reference Enkhtuya, Poschl and Vosatka2005; Fan et al. Reference Fan, Dalpe, Fang, Dube and Khanizadeh2011; Wu et al. Reference Wu, Zou and He2010; Wu QS et al., Reference Wu, Zou, He and Luo2011) and the competitive relationships of the two plants (Danieli-Silva et al. Reference Danieli-Silva, Uhlmann, Vicente-Silva and Sturmer2010; Li et al. Reference Li, Ran, Zhang, Sun and Xu2009) needs future exploration. Moreover, as a leguminous plant, D. triflorum might have more advantages in nutrient usage/absorption, biomass accumulation, root secretion production, and other physiological processes than the native species, Z. tenuifolia. The relationship of non-leguminous (Z. tenuifolia) and leguminous (D. triflorum) species in AMF interactions (Klabi et al. Reference Klabi, Hamel, Schellenberg, Iwaasa, Raies and St-Arnaud2014) should be taken into consideration; therefore, a future laboratory incubation experiment simulating different D. triflorum/Z. tenuifolia stem densities in the lawn needs to be conducted to achieve a better understanding of the possible advantages of D. triflorum in plant growth, nutrient use, root morphology construction, and biomass responses under the infection of AMF.
In the present study, we compared the root mycorrhizal colonizations, soil AM fungal spore densities, and soil AMF communities under different invasion pressures of D. triflorum in a Z. tenuifolia lawn. Our results suggest that the development of D. triflorum in the lawn was likely to have been initiated by the root hyphal connection from Z. tenuifolia roots, while the preference of root mycorrhizal structures in contributing to soil sporulation tended to change from Z. tenuifolia to D. triflorum. Meanwhile, the different soil AMF community compositions and relative abundances of AMF OTUs among D. triflorum coverage levels might also reflect changes in the mycorrhizal colonizations, soil properties, and interspecific relationships of native Z. tenuifolia and invasive D. triflorum during this spreading period. Taken together, the successful spread of D. triflorum in the lawn is due not only to the advantage of root mycorrhizal infection, but also to the dependency of soil properties, spore properties, and root colonization of D. triflorum in comparison to Z. tenuifolia. Future evidence, such as the detailed root AMF/soil AMF spore species that initiated and supported the spreading process, as well as data on plant growth, nutrient utilization, root morphology construction, and interspecific competitive advantages of D. triflorum are needed to fully unveil the mechanisms of D. triflorum in successfully outcompeting Z. tenuifolia in the lawn.
Acknowledgments
This work was financially supported by grants from the National Natural Science Foundation of China (31071357,31371567), the Natural Science Foundation of Guangdong Province (9251027501000010), the YangFan Innovative and Entepreneurial Research Team Project (2015YT02H032), and the Hong-da Zhang Science Research Fund of Sun Yat-sen University. We are very grateful to the three anonymous reviewers who provided constructive comments for improving this paper. We are also grateful to Jianhua Guo, Hongbin He, Xue Peng, and Yingying Huang for their assistance in field investigation and laboratory analyses. The authors declare that the research was conducted in the absence of any commercial or financial relationships that could be construed as a potential conflict of interest.
Supplementary Materials
To view supplementary material for this article, please visit https://doi.org/10.1017/wsc.2019.50