1. Introduction
Chronic Kidney Disease (CKD) affects 11–13% of the world population (Hill et al., Reference Hill, Fatoba, Oke, Hirst, O’Callaghan, Lasserson and Hobbs2016) and Renal Replacement Therapies (RRTs) stand as a life support for most of these patients. The RRTs generally associated to the Artificial Kidney (AK) are membrane-based treatments which assure the separation functions of the failing kidney in extracorporeal blood circulation. In the 1990s, the progress from conventional hemodialysis (HD) controlled by diffusion and known as low-flux hemodialysis (LFHD) to new RRTs like hemofiltration(HF), hemodiafiltration (HDF) and high-flux HD (HFHD) (Cheung et al., Reference Cheung, Levin, Greene, Agodoa, Bailey, Beck, Clark, Levey, Leypoldt, Ornt, Rocco, Schulman, Schwab, Teehan and Eknoyan2003) was due to the introduction of ultrafiltration (UF) membranes characterized by high convective permeation fluxes. Despite the advancement on the efficient removal of small water-soluble toxins and slight improvement on the removal of middle size molecules and protein bound uremic toxins (Vanholder et al., Reference Vanholder, De Smet, Glorieux, Argilés, Baurmeister, Brunet, Clark, Cohen, De Deyn, Deppisch, Descamps-Latscha, Henle, Jörres, Lemke, Massy, Passlick-Deetjen, Rodriguez, Stegmayr and Stenvinkel2003), fluid volume removal and hemodynamic management remains as a matter of concern due to its association to cardiovascular stress and potential organ damage (Canaud et al., Reference Canaud, Chazot, Koomans and Collins2019; Poorkhalil et al., Reference Poorkhalil, Mouzakis, Kashefi and Mottaghy2019).
2. Objective
Despite the understanding of hemodynamics as being directly dependent on fluid mechanics fundamentals, little attention has been given to the main focus of the present work, which is the influence of the fluid removal rates typical of HFHD on the flow field, particularly on the increase of the potentially blood-traumatizing shear stresses developed adjacently to the membrane. In fully developed flow, as the slit flow case herein, the pressure drop mirrors the magnitude of the forces responsible for the shear stresses near the wall and its quantification is performed in a surrogate system of an AK/hemodialyzer mimicking tangential fluid velocities and membrane removal rates with circulating water.
3. Methods
Two flat-sheet laboratory-made ultrafiltration (UF) hybrid membranes of cellulose acetate(CA)/silica(SiO2) are tested. The synthesis and characterization (SEM, ATR/FTIR, NMR) of CA30/5%SiO$ {}_2 $ and CA22/5%SiO
$ {}_2 $ was previously reported by Mendes et al. (Reference Mendes, Faria, Carvalho, Gonçalves and de Pinho2018) (membrane A5) and Beira et al. (Reference Beira, Silva, Condesso, Cosme, Almeida, Corvo, Sebastião, Figueirinhas and de Pinho2019), respectively. Figure 1 (a) shows the experimental setup, comprising the two-compartment AK surrogate where the semi-permeable membrane (surface area of 0.0021 m
$ {}^2 $) separates the top slit (height, h = 600
$ \mu $m
$ \ll $ width, W = 3 cm – Figure 1 (b)) for feed circulation from the bottom chamber receiving the membrane permeating fluid. The feed/water (T = 37
$ {}^{\circ } $C) pumped through the slit at volumetric flowrates 40 ml/min
$ \le $ Q
$ \le $ 160mL/min generates pressure drops,
$ \Delta P $ = PT1−PT2, along length L = 25 cm, registered for permeable and impermeable walls. The
$ \Delta P $ uncertainty,
$ {\sigma}_{\Delta P}=\pm \hskip1.5pt 20\% $, depends on the pressure sensors precision (high accuracy:
$ \pm \hskip1.5pt 1.33\times {10}^2 $ Pa) and, mainly, on both the slit height and local pressure losses uncertainties (estimated through literature empirical correlations). Pressure sensors are computer connected through the data acquisition program LabVIEW. Transmembrane pressure ranges from
$ 3\times {10}^3 $ Pa to
$ 1.5\times {10}^4 $ Pa. A fully-developed laminar flow of a Newtonian-fluid (dynamic viscosity
$ \mu $) inside a slit, with hydrodynamic smooth-surfaces, experiences the pressure reduction
$ \Delta {P}_{\mathrm{th}}=12\frac{\mu LQ}{Wh^3\left[1-0.63\frac{h}{W}\right]} $ (Bruus, Reference Bruus2008), with uncertainty
$ {\sigma}_{\Delta P}= \pm 18 $% depending on the intervening variables uncertainties (Miller & Miller, Reference Miller and Miller1993). These theoretical values are compared with the experimental ones.
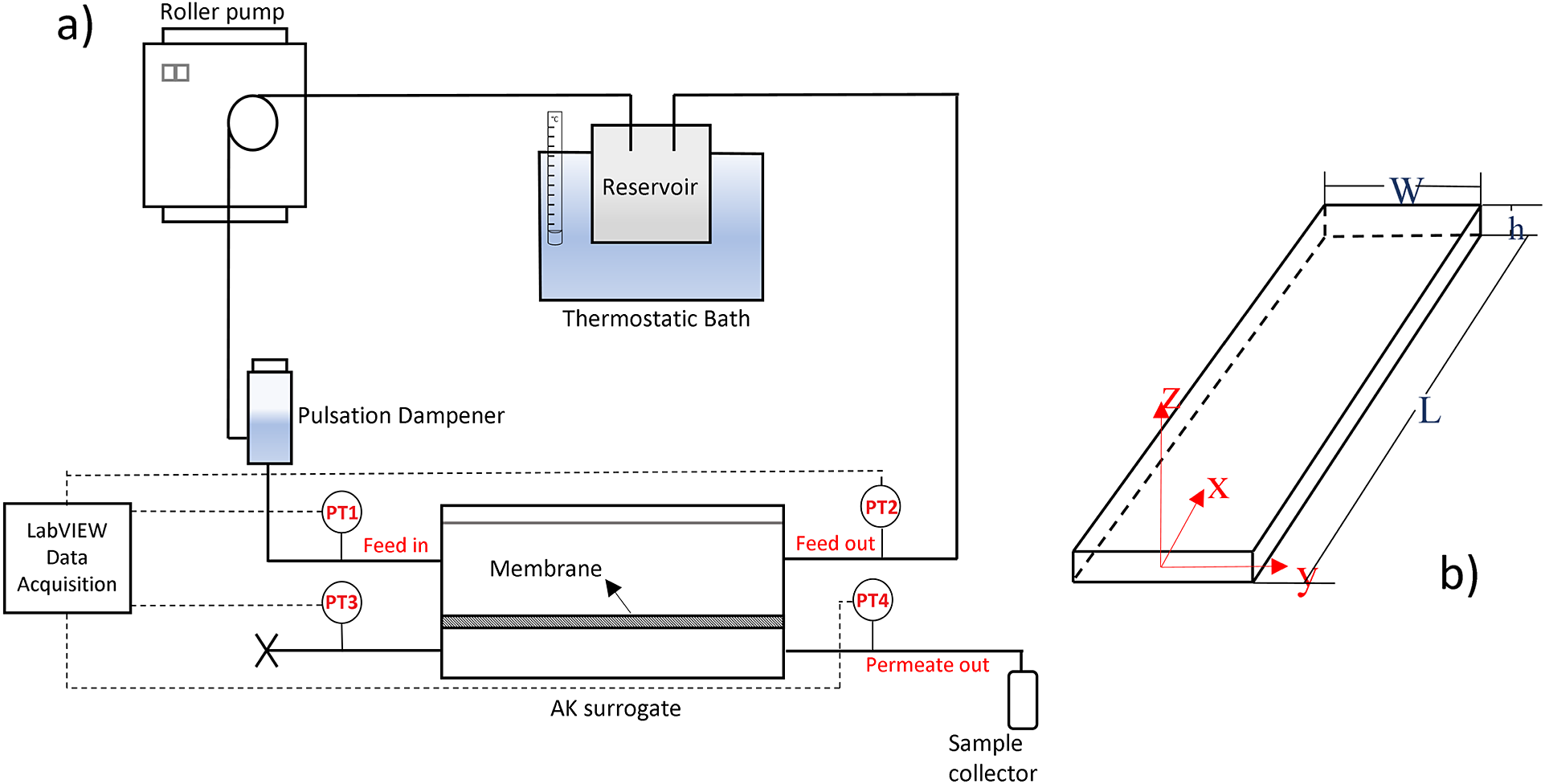
Figure 1. (a) Experimental setup. (b) Top slit geometry and coordinate axis.
4. Results and discussion
The hydraulic permeability of the membranes is 18.09 kg/h/m$ {}^2 $/bar for CA22/5%SiO2 and 198.65 kg/h/m
$ {}^2 $/bar for CA30/5%SiO2.
All figures ahead display pressure drop ($ \Delta P $) results as a function of the volumetric flowrate (
$ Q $) in the surrogate AK.
Figure 2 compares theoretical and experimental results without permeation. Linear dependence of $ \Delta P $ on
$ Q $, with a slope increase with the channel height reduction (compare red and black lines), confirms the laminar regime of a fully developed Poiseuille-type flow (Silva et al., Reference Silva, Leal and Semiao2008). The much larger experimental
$ \Delta P $ values (relatively to the theoretical ones: compare red and blue lines) emerge from the walls roughness. This can be modelled by an apparent viscosity (Magueijo et al., Reference Magueijo, Semiao and de Pinho2006).
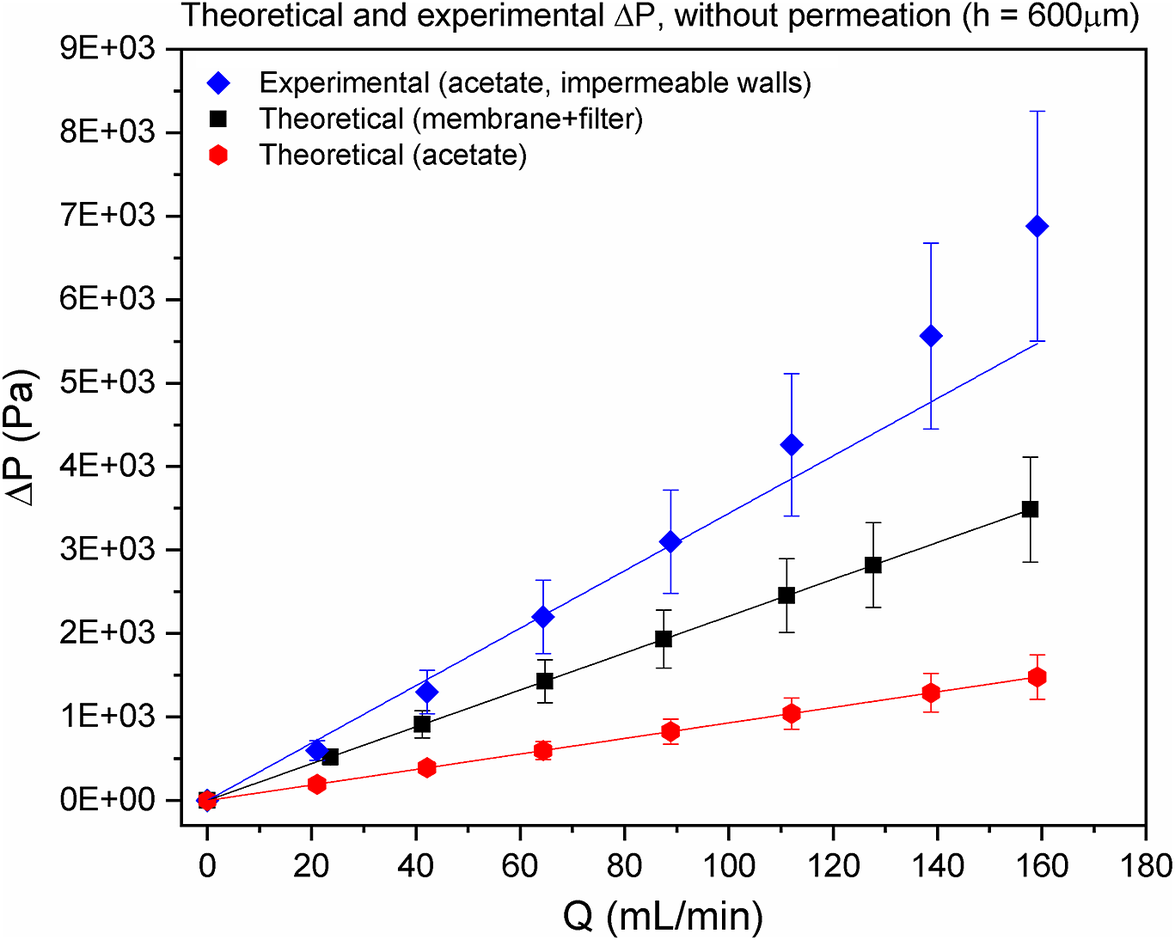
Figure 2. Theoretical and experimental pressure drop, $ \Delta P $, in an impermeable surrogate system of a hemodialyzer as a function of the volumetric flow rate,
$ Q $. Red line refers to theoretical pressure drop in the slit with an acetate at the bottom wall (without permeation). Black line refers to theoretical pressure drop in the slit with a membrane over a filter paper at the bottom wall (without permeation). Blue line refers to the experimental pressure drop in a slit with an acetate at the impermeable bottom wall.
Figure 3 illustrates in HFHD the effect of membrane removal rates on the slit flow field. Both experimental $ \Delta P $ lines with permeation exhibit consistently much larger slopes than the theoretical one (compare green and red lines against black line), evidencing the increase of potentially blood-traumatizing shear stresses at the membrane surface. Such enormous increase (ca. 512% for CA22/5%SiO2 and 576% for CA30/5%SiO2 membrane) is probably due to the high removal rates, which deform the velocity profile shifting its maximum towards the lower-half slit and, hence, increasing the velocity gradient at the membrane surface: the fully developed flow condition may be at stake. Moreover,
$ \Delta P $ line for membrane CA22/5%SiO2 exhibits a slope smaller than that of CA30/5%SiO2 (compare green line with red line). This is in accordance with the fact that the CA22/5%SiO2 is a smoother membrane.
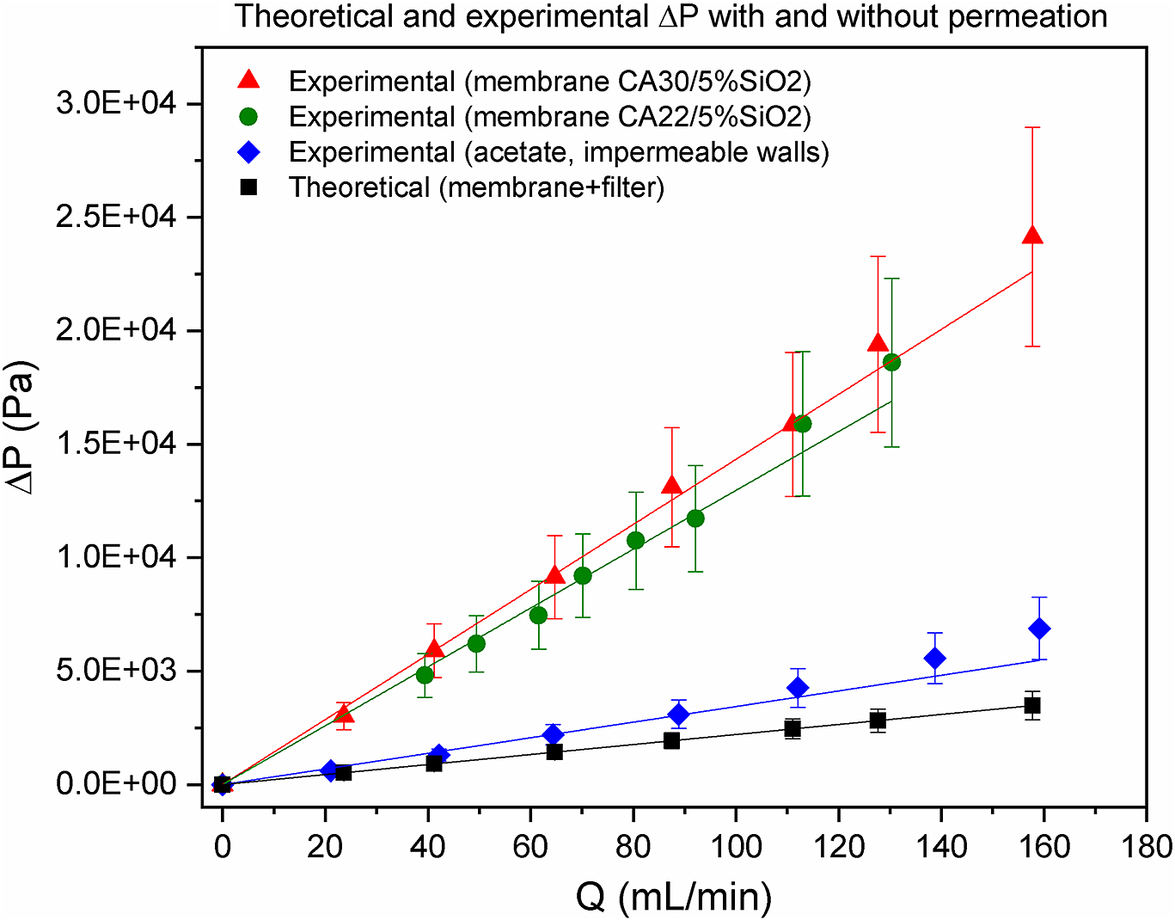
Figure 3. Theoretical and experimental pressure drop, $ \Delta P $, in a surrogate system of a hemodialyzer as a function of the volumetric flow rate,
$ Q $. Black line refers to the theoretical pressure drop in a slit with a membrane over a filter paper at the bottom wall (without permeation). Blue line refers to the experimental pressure drop in a slit with an acetate at the bottom wall (without permeation). Green and red lines refer to the experimental pressure drop in a slit with permeation (bottom wall with CA30/5%SiO2 membrane for the red line and CA22/5%SiO2 membrane for the green line).
Taking into consideration that the CA30/5%SiO2 membrane complies with the kidney metabolic functions of preferential permeation of urea and retention of albumin (Faria et al., Reference Faria, Moreira, Eusébio, Brogueira and de Pinho2020), Figure 4 shows the results of $ \Delta P $vs.
$ Q $ for the solutions of these two solutes and other typical toxins at different concentrations/different viscosities. Within the range of the experimental error, there is no significant effect of the solutions viscosity on
$ \Delta P $ (all experimental points are around the black line for water).
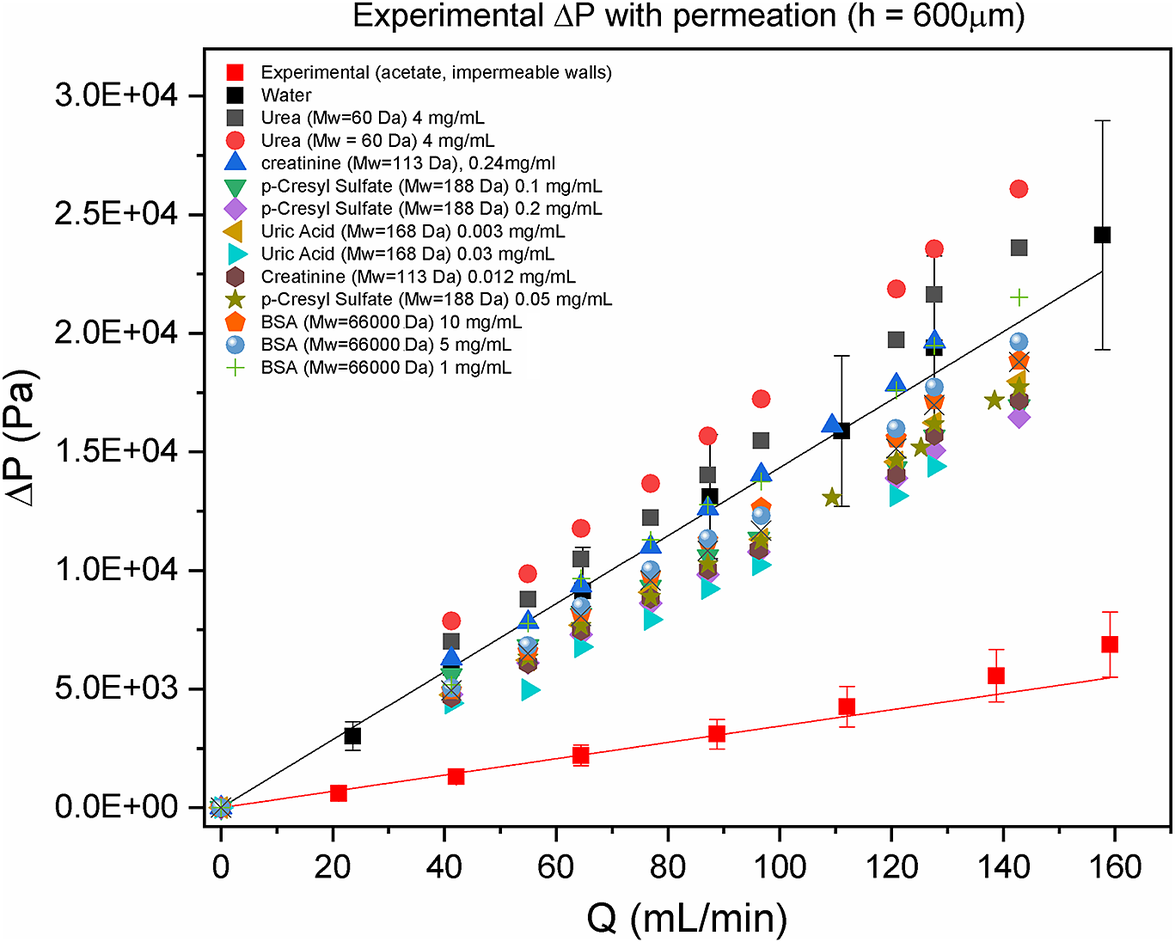
Figure 4. Experimental pressure drop, $ \Delta P $, with permeation of pure water and solutions of toxins & bovine serum albumin (BSA) in a surrogate system of a hemodialyzer as a function of the volumetric flow rate,
$ Q $, with membrane CA30/5%SiO2. Black line refers to the experimental pressure drop in a slit with pure water permeation. Red line is the reference for the experimental pressure drop without permeation. The solutions of the toxins (urea, creatinine, uric acid & p-cresyl sulfate) and of the BSA have different concentrations.
5. Conclusion
UF permeation experiments in HFHD typical conditions, performed in a surrogate AK/hemodialyzer, elucidated how fluid mechanics fundamentals rule the effects of high removal rates on the blood flow field. The paramount increase on pressure drop, compared to that of impermeable theoretical flow conditions, ca. 512% for membrane CA22/5%SiO2 and 576% for CA30/5%SiO2, reflects the increase on magnitude of the potentially blood-traumatizing shear stresses at the membrane surface. This comes from the distortion of the circulating fluid velocity profile, with its maximum shifted into the membrane surface direction, increasing, therefore, the velocity gradient there.
Funding Information
This work was supported by FCT, Fundação para a Ciência e Tecnologia, Portugal (through CeFEMA, Centre of Physics and Engineering Advanced Materials, UID/CTM/04540/2013 and project PTDC/CTM-BIO/6178/2014), (through IDMEC, under LAETA, project UIDB/50022/2020, IDMEC, Instituto Superior Técnico, Universidade de Lisboa, Lisboa, Portugal); and Italian Ministry of Education University and Research (Department of Civil, Chemical, Environmental and Materials Engineering, University of Bologna, Italy).
Conflict of Interest
The authors have no conflicts of interest to declare.
Data Availability Statement
Readers can contact the authors if they want access to such materials.
Comments
Comments to the Author: The authors focused the manuscript on the effect of ultrafiltration transmembrane permation on the flow field by using a surrogate system of a hemodialyzer. They tested two flat-sheet laboratory-made hybrid membranes of cellulose acetate(CA)/silica(SiO2): CA30/5%SiO2 and CA22/5%SiO2.
The experimental results are interesting and I suggest to revise the manuscript in order to clarify some minor issues.
1) The Authors should include in the manuscript information about the membranes used in this study (e.g., pore size, porosity, thickness, physico-chemical, etc.) in order to provide to readers a comprehensive view of the membrane characteristics and to appreciate the differences with other suitable commercial membranes.
2) A more detailed description and discussion for Figure 4 would be more appropriate.