Introduction
Giardia infection, epidemiology and assemblages
Giardia duodenalis (G. duodenalis) is of increasing public health importance and is the only one of six morphologically distinct species of Giardia to infect humans. This protozoan parasite results in approximately 4000 laboratory-confirmed giardiasis cases each year in the UK (Gov.uk, 2016; HPS Scotland, 2016) and 2.8 × 108 cases worldwide (Esch and Petersen, Reference Esch and Petersen2013). In 2006, the parasite was added to the Neglected Diseases Initiative to prompt a more comprehensive understanding of the parasite and its epidemiology, as well as the global disease burden (Savioli et al., Reference Savioli, Smith and Thompson2006).
Infection occurs when cysts shed by a host contaminate the environment, leading to ingestion by a new host via a fecal/oral cycle. Following ingestion, the parasite reproduces quickly within the gut causing exponential multiplication. New cysts are then formed which are shed in the feces of the host, contaminating the environment (Olson et al., Reference Olson, Goh, Phillips and McAllister1999; Adam, Reference Adam2001). A relatively low infective dose of between 10 and 100 cysts is required to cause human infection (Roxstrom-Lindquist et al., Reference Roxstrom-Lindquist, Palm, Reiner, Ringqvist and Svard2006). This, combined with vast numbers of cysts excreted per gram of stool gives the parasite a high potential for infection in host populations as infectious doses are low.
Infections can range from asymptomatic to severe. Symptomatic individuals often experience foul smelling mucoidal diarrhoea, nausea, headaches, fevers, stomach cramps and foul smelling flatulence and belching (Einarsson et al., Reference Einarsson, Ma'ayeh and Svard2016). Asymptomatic individuals with the disease show no signs of infection however will still shed the parasite in their feces. Differences in the symptomology are now suspected to be due to assemblage variance, with assemblage B of the parasite thought to result more commonly in symptomatic infection, compared with assemblage A, which appears to do this less so (Hussein et al., Reference Hussein, Ismail, Mokhtar, Mohamed and Saad2017; Puebla et al., Reference Puebla, Nunez, Santos, Rivero, Silva, Valdes, Millan and Muller2017). Host factors such as age, immune status and gut fauna are thought to be involved in the development of the infection. Young children and immune-compromised individuals are often thought to be more at risk; however, other more complex factors such as bacteria types located within the gut are suspected to be involved in infection vulnerability (as found in mice by Benyacoub et al., Reference Benyacoub, Perez, Rochat, Saudan, Reuteler, Antille, Humen, De Antoni, Cavadini, Blum and Schiffrin2005) (Robertson et al., Reference Robertson, Hanevik, Escobedo, Kristine and Langeland2010; Solaymani-Mohammadi and Singer, Reference Solaymani-Mohammadi and Singer2010; Halliez and Buret, Reference Halliez and Buret2013; Bartelt and Sartor, Reference Bartelt and Sartor2015). Infected hosts rely on both innate and adaptive immune responses in attempts to prevent/control infection. Innate responses such as mucus interfering with parasite adherence to epithelial cells, synthesis of cytotoxic compounds (Eckmann et al., Reference Eckmann, Laurent, Langford, Hetsko, Smith, Kagnoff and Gillin2000) such as nitric oxide, along with the production of cytokines, particularly IL-6 (Bienz et al., Reference Bienz, Dai, Welle, Gottstein and Muller2003; Zhou et al., Reference Zhou, Li, Zhu, Robertson, Nash and Singer2003; Kamda et al., Reference Kamda, Nash and Singer2012), limit proliferation of the parasite in the protective immune response. If innate responses fail, adaptive immune responses, triggered by increased antigen levels due to uncontrolled proliferation of the parasite, consist of both increasing antibody levels and immune T-cell production. IgA and IgG antibodies specific to Giardia have been previously detected in infected hosts (El-Gebaly et al., Reference El-Gebaly, Halawa, Moussa, Rabia and Abu-Zekry2012) and similarly so have CD4+ and CD8+ T cells (Singer and Nash, Reference Singer and Nash2000). Giardiasis is however a treatable disease and drugs including, but not limited to, metronidazole, tinidazole, ornidazole or albendazole are all prescribed when diagnosis is confirmed (Gardner and Hill, Reference Gardner and Hill2001).
Additionally, research shows that infection is associated with longer term health problems, such as irritable bowel syndrome and chronic fatigue (D'Anchino et al., Reference D'Anchino, Orlando and De Feudis2002; Stark et al., Reference Stark, van Hal, Marriott, Ellis and Harkness2007; Robertson et al., Reference Robertson, Hanevik, Escobedo, Kristine and Langeland2010; Halliez and Buret, Reference Halliez and Buret2013).
In combination with host immune factors, it is suspected that the various subtypes of G. duodenalis known as ‘Assemblages’ may result in differing severity of disease. Assemblages are termed ‘A–H’ with each having a different host range, supporting the hypothesis that these assemblages represent potentially different species (Ryan and Cacciò, Reference Ryan and Cacciò2013). Assemblages A and B have a much wider host range than the other assemblages and include humans, which has been an argument for the zoonotic status of this parasite (Ryan and Cacciò, Reference Ryan and Cacciò2013). There is warranted speculation that the parasite is zoonotic and this will be explored in this review (Stuart et al., Reference Stuart, Orr, Warburton, Jeyakanth, Pugh, Morris, Sarangi and Nichols2003; Thompson, Reference Thompson2004; Sprong et al., Reference Sprong, Cacciò and van der Giessen2009; Feng and Xiao, Reference Feng and Xiao2011; Esch and Petersen, Reference Esch and Petersen2013). To add complexity, within these assemblages there are defined sub-assemblages. In cases where the sub-assemblage has been described, results are contradictory. For assemblage A, of the sub-assemblages (AI, AII, AIII and AIV), only AII is thought to be the most serious threat of infection in humans (Xiao and Fayer, Reference Xiao and Fayer2008). Understanding the epidemiology of these sub-assemblages is paramount in the battle against human giardiasis.
The full extent of the impact that the parasite has on public health is unclear due to variations in reporting/surveillance systems, differences in testing criteria and laboratory testing methods. Outbreaks are likely to be under-reported and, of those which have been, they are often linked to contaminated food and water sources, public swimming pools or children day care centres (Robertson, Reference Robertson1996; Nygard et al., Reference Nygard, Schimmer, Sobstad, Walde, Tveit, Langeland, Hausken and Aavitsland2006; Smith et al., Reference Smith, Reacher, Smerdon, Adak, Nichols and Chalmers2006; Karanis et al., Reference Karanis, Kourenti and Smith2007; Daly et al., Reference Daly, Roy, Blaney, Manning, Hill, Xiao and Stull2010; Rimhanen-Finne et al., Reference Rimhanen-Finne, Hanninen, Vuento, Laine, Jokiranta, Snellman, Pitkanen, Miettinen and Kuusi2010). Compared with the similar waterborne protozoan parasite, Cryptosporidium, less information is available on the costs of outbreaks and incidences of disease; direct hospitalization costs are estimated at US$34 million annually, within the USA (Collier et al., Reference Collier, Stockman, Hicks, Garrison, Zhou and Beach2012) but no further information has been found on other economic costs for human health elsewhere (Siwila, Reference Siwila2017). Livestock disease is associated with economic losses for farmers due to the adverse impact on production, feed efficiency, weight gain and sometimes death (Feng and Xiao, Reference Feng and Xiao2011; Painter et al., Reference Painter, Gargano, Collier and Yoder2015).
The threat of G. duodenalis is amplified by a lack of understanding of possible transmission routes and the misconception, certainly from within the UK, is that infection remains largely thought to be associated with foreign travel. Recent data in the UK, however, highlight that over 50% of cases within Scotland may be locally acquired (Alexander et al., Reference Alexander, Currie, Pollock, Smith-Palmer and Jones2017).
This review summarizes potential infection/transmission routes covering water, food person-to-person infection and zoonotic transmission from livestock and companion animals. Furthermore, the review investigates the evidence and role of different assemblages in transmission, as well as considering detection methods and prevention measures, highlighting gaps in knowledge and making recommendations for future research.
Public health risks
Contaminated water
A review of waterborne disease outbreaks, between the years of 2004 and 2010, stated that G. duodenalis accounted for 70/199 (~35%) reported outbreaks of human disease due to waterborne protozoa within high-income countries (Baldursson and Karanis, Reference Baldursson and Karanis2011). This is supported by recent data from 2011 to 2016, where 37% of worldwide waterborne protozoa outbreaks were due to G. duodenalis (Efstratiou et al., Reference Efstratiou, Ongertha and Karanis2017b). It was however noted that the majority of the data in these studies was taken from higher income countries, as low-income countries often lack the necessary systems allowing surveillance capabilities. The real-world burden of waterborne disease outbreaks with G. duodenalis will remain unknown until global surveillance is implemented; however, where possible, collation of available data from within these regions indicates the size of this burden (Torgerson et al., Reference Torgerson, Devleesschauwer, Praet, Speybroeck, Willingham, Kasuga, Rokni, Zhou, Fevre, Sripa, Gargouri, Furst, Budke, Carabin, Kirk, Angulo, Havelaar and de Silva2015). Young children are often at the forefront of the effects of diarrhoeal-causing disease organisms, such as G. duodenalis, which can have a large impact on development. Regions of the world where giardiasis thrives tend to be in those with poor living conditions, often the population lives within systems of malnourishment, squalor and unsanitary conditions which promote spread of the disease. Children are particularly at risk of acquiring the disease within these locations and are often impacted heavily due to commonly being already unhealthy and potentially infected with other pathogenic organisms such as bacteria or other protozoan species (Sylvia and Ryan, Reference Sylvia and Ryan2017; Daniels et al., Reference Daniels, Smith and Jenkins2018).
A study of US outbreaks found that drinking water was the source in 75% of cases, with recreational and environmental waters, such as swimming baths and lakes, respectively, resulting in accidental ingestion of contaminated waters (Porter et al., Reference Porter, Ragazzoni, Buchanon, Waskin, Juranek and Parkin1988; Slifko et al., Reference Slifko, Smith and Rose2000; Katz et al., Reference Katz, Heisey-Grove, Beach, Dicker and Matyas2006; Adam et al., Reference Adam, Yoder, Gould, Hlavsa and Gargano2016)
Effective filtration and identification of the parasite from water samples is becoming significantly important to minimize the risk of water-borne outbreaks. Water industries throughout the UK maintain high standards with regular sampling occurring to ensure that their water is safe for human consumption. However, a number of private water supplies exist that are not under scrutiny from local water authorities and may be at higher risk of contamination (Risebro et al., Reference Risebro, Breton, Aird, Hooper and Hunter2012). In the USA, G. duodenalis is monitored via the EPA Safe Drinking Water Act (SDWA) and regulated using US EPA Method 1623 (U.S. Environmental Protection Agency, 2012), which has had a positive impact on reducing cases of giardiasis within the country (Painter et al., Reference Painter, Gargano, Collier and Yoder2015). Detection methods are still currently at a level whereby 100% recovery of parasite numbers within samples is not achievable, with the majority of the losses occurring in the filtration steps (Wohlsen et al., Reference Wohlsen, Bates, Gray and Katouli2004; Efstratiou et al., Reference Efstratiou, Ongerth and Karanis2017a; Horton et al., Reference Horton, Katzer, Desmulliez and Bridle2018). Recoveries ranging between 3 and 97% are to be expected, depending on which of the UK DWI-approved detection methodologies is employed (UK Environment Agency, 2010). Commonly utilized, DWI-approved, filtration methods in the UK include the Genera FiltaMax and FiltaMax xpress systems, PAL Envirochek filters and those using polycarbonate membranes or chemical flocculation methods. In the UK, the DWI highlight that the FiltaMax system has the highest recoveries for Giardia cysts (UK Environment Agency, 2010).
Adapting/improving existing systems, which are known to work well, is desirable as it reduces the time required to implement the new method as well as limits the costs involved. The IDEXX FiltaMax xpress system already moves processing towards automation, limiting time taken by analysts when processing samples. Full automation of sample processing into existing systems (Kerrouche et al., Reference Kerrouche, Desmulliez and Bridle2015; Horton, et al., Reference Horton, Katzer, Desmulliez and Bridle2018) is also now being investigated, which is an area that should be explored further in order to standardize processes more efficiently. Automated systems will allow quicker and less laborious achievement of results, which could point towards potential breaches in public health protection more effectively. Earlier warning means authorities can act quicker, thus reducing incidences of public infection through consumption of unsafe waters. In addition, being able to detect both Cryptosporidium (which is subjected to strict quality regulations in the UK) and Giardia species in a single test would be of great advantage (DWI REPORT 70-2-155, 2003). Detection methods for standard water monitoring typically utilize microscopy, though molecular-based studies would be needed, to identify the presence of different G. duodenalis assemblages in water to determine public health risk posed.
Contaminated food
It is well known that G. duodenalis infection within humans can occur due to the consumption of contaminated food items, with historical data of outbreaks highlighting this (Osterholm et al., Reference Osterholm, Forfang, Ristinen, Dean, Washburn, Godes, Rude and McCullough1981; Petersen et al., Reference Petersen, Cartter and Hadler1988; Porter et al., Reference Porter, Gaffney, Heymann and Parkin1990; Mintz et al., Reference Mintz, Wragg, Mshar, Cartter and Hadler1993; Smith et al., Reference Smith, Cacciò, Cook, Nichols and Tait2007). Food-related outbreaks of giardiasis are however less commonly reported than those originating from water supplies, as shown in the USA between 1971 and 2011, whereby 74.8 and 15.7% of outbreaks were reported as waterborne and foodborne, respectively (Adam et al., Reference Adam, Yoder, Gould, Hlavsa and Gargano2016).
The difference however may also be due to the difficulty in the reporting/detection of foodborne outbreaks in the population, compared with water source outbreaks, which tend to have a more identifiable point of origin. Waterborne outbreaks tend to be confined regionally with increased cases detected within a single health authority, whilst foodborne outbreak cases tend to be far greater dispersed and thus increasingly difficult to notice. Commercially prepared contaminated food, e.g. such as vegetables or salad items, can often travel a great distance before being consumed by the individual who will potentially become infected, masking point of contamination of food. This has been observed in a previous outbreak of foodborne Cryptosporidium parvum within the UK highlighted by surveillance work (McKerr et al., Reference McKerr, Adak, Nichols, Gorton, Chalmers, Kafatos, Cosford, Charlett, Reacher, Pollock, Alexander and Morton2015).
Salad items and vegetables have been often highlighted as a foodborne risk for giardiasis. Salad and vegetable items which are (a) improperly washed to remove cysts or (b) washed, but in contaminated water, and (c) poor food handler hygiene can lead to human giardiasis. The need for clean water when preparing and washing vegetables and salad for human consumption has been highlighted by Smith et al. (Reference Smith, Cacciò, Cook, Nichols and Tait2007) whereby it was referred that a 250 g bag of prepared salad maintains around 2% of the wash water within it which is difficult to remove in preparing. In the event that water used to clean the salad is contaminated, this could potentially result in occasional bags becoming contaminated with enough oocysts to infect the consumer, leading to an outbreak. This could potentially occur in a location far afield from the source of infection and thus be difficult to trace. Additional methods of foodborne giardiasis include infection via cold drinks (contaminated water frozen to make ice) (de Lalla et al., Reference De Lalla, Rinaldi, Santoro, Nicolin and Tramarin1992), shellfish which are contaminated with cysts (filter feeding shellfish can accumulate cysts within them) (Giangaspero et al., Reference Giangaspero, Papini, Marangi, Koehler and Gasser2014), mechanical transmission to food items via insects such as filth flies (Graczyk et al., Reference Graczyk, Knight and Tamang2005).
No food monitoring for Giardia is routinely undertaken, as far as the authors are aware, and thus little is known about the prevalence of foodborne Giardia in Scotland and the UK. This appears to be true worldwide. In addition, whilst the final detection methods for the cysts are similar to those utilized in water, different extraction methodologies are necessary to remove Giardia for food matrices. Some attempts to develop useful and efficient extraction methods for specific foodstuffs have been carried out, with varying results for Giardia (Robertson and Gjerde, Reference Robertson and Gjerde2001; Cook et al., Reference Cook, Nichols, Wilkinson, Paton, Barker and Smith2007; Schets et al., Reference Schets, van den Berg and de Roda Husman2013). Future studies are required within this area to develop optimized enrichment methodologies of cysts from food sources, which would allow further investigation.
Infected persons
G iardia duodenalis prevalence within the global human population varies between high- and low-income countries throughout the world, with infection estimates of between ~5 and ~20%, respectively (Roxstrom-Lindquist et al., Reference Roxstrom-Lindquist, Palm, Reiner, Ringqvist and Svard2006). Within the high-income countries, strict control and filtration of drinking water, as well as high-quality sanitation systems, prevent the disease being as prevalent as it is in poorer areas of the world.
Due to the cysts being infectious when excreted in feces, humans have the potential to pass on the pathogen to other humans either from direct contact or via transmission from contaminated sources, e.g. foods, most likely due to poor hygiene. Cases may be mild, short-lived or even asymptomatic and therefore individuals may be highly infectious without knowing, and will therefore not seek medical attention. In addition, long time delays between infection and consultation of a general practitioner (GP) can lead to delayed diagnosis which increases the chances of spreading the infectious cysts (Cacciò and Sprong, Reference Cacciò, Sprong, Lujan and Svard2011a). Infected individuals may not consult a GP for short-term health issues (diarrhoea, etc.) despite these patients still shedding the parasite in their feces (Espelage et al., Reference Espelage, Heiden an der, Stark and Alpers2010; Minetti et al., Reference Minetti, Chalmers, Beeching, Probert and Lamden2016; Waldram et al., Reference Waldram, Vivancos, Hartley and Lamden2017).
In the UK, Public Health England and Wales reported 3624 laboratory-confirmed cases of giardiasis in the population (Gov.uk, 2016) in 2013. In Scotland, there were only 167 reported cases of giardiasis in the population in the same year (HPS Scotland, 2016). Assuming similar testing criteria is applied throughout the UK, the differences may partly be due to regional differences in G. duodenalis distribution. Increased infection numbers within England and Wales would be expected due to a much larger population than that of Scotland [England has almost 10 times that of the population found in Scotland alone, as of 2014 (Ons.gov.uk, 2016)]. Scottish per capita rates of infection range from 0 to 19.2 per 100 000 (personal communication from Health Protection Scotland, 2017), whilst England and Wales capita rates range from 4.1 to 18.1 (Gov.uk, 2016).
A study in London by Breathnach et al. (Reference Breathnach, McHugh and Butcher2010) found that human infection prevalence with G. duodenalis assemblage B agreed with other similar study results in different countries worldwide (Cacciò and Ryan, Reference Cacciò and Ryan2008), being more common than assemblage A. In Scotland however, the situation could be somewhat different as Alexander et al. (Reference Alexander, Jones, Inverarity and Pollock2014) reported that the occurrence of assemblages A and B in infections in Scotland was actually the reverse of London, and indeed worldwide, with assemblage A being more commonly diagnosed in infections.
To improve detection and obtain more accurate information regarding the extent of Giardia infection in humans, there are significant improvements required to laboratory testing algorithms and procedures to minimize delays and under-reporting of this pathogen. In the UK, Giardia testing is often only requested by GPs in individuals who have recently travelled to endemic areas. This means that locally acquired infections go undetected, and therefore untreated, which increases the risk of disease spread (Alexander et al., Reference Alexander, Currie, Pollock, Smith-Palmer and Jones2017). Diagnostic microbiology laboratories mostly use microscopy for the detection of cysts (and trophozoites) (Alexander et al., Reference Alexander, Currie, Pollock, Smith-Palmer and Jones2017). As G. duodenalis is known to have sporadic shedding cycles within hosts (Goka et al., Reference Goka, Rolson, Mathan and Farthing1990; as reviewed by Johnson et al., Reference Johnston, Ballard, Beach, Causer and Wilkins2003), the examination of at least three samples is preferred (but often not achieved) to confirm a diagnosis of Giardia in over 90% of suspected cases. It is not uncommon for laboratories to only receive a single stool for testing from an individual which greatly reduces the chances of finding cysts. Duodenal fluid can also be examined in order to identify trophozoites in cases where there is an absence of cysts in stools; in Scotland, this roughly occurs 10 times a year (personal communication with Consultant Clinical Scientist, 2017). The implementation of alternative methods into diagnostic microbiology laboratories with improved sensitivity has been validated which include antigen detection using enzyme-linked immunosorbent assays (Johnston et al., Reference Johnston, Ballard, Beach, Causer and Wilkins2003; Jahan et al., Reference Jahan, Khatoon and Ahmad2014), and detection of DNA using molecular assays (Minetti et al., Reference Minetti, Lamden, Durband, Cheesbrough, Fox and Wastling2015). Whilst being more sensitive, these permit automation of much larger numbers of samples to permit the testing of all stools, rather than a small subset and remove the need for specialist microscopy expertise. Advancement in genotyping methods however, as well as increased specificity, is also paramount. Currently G. duodenalis genotyping often focuses on small subunit rRNA-based approaches, or triose-phosphate isomerase (tpi), glutamate dehydrogenase (GDH) and/or β-giardin genes to characterize human G. duodenalis isolates. Often in these gene targets, when attempting genotyping, intra-assemblage variance is seen, which affects the quality of genotyping efforts; however, the extent that this could be caused by differences in methodology used (non-standardized primers and heterogeneous gene fragment sizes), or affected by degrees of intra-assemblage/sub-assemblage polymorphisms existing within the G. duodenalis genus, is unknown (Bonhomme et al., Reference Bonhomme, Le Goff, Lemee, Gargala, Ballet and Favennec2011). Studies have shown that this occurs more commonly when using tpi for genotyping G. duodenalis isolate sequences (as opposed to β-giardin or GDH), and also with increased frequency when attempting to determine assemblage B isolates, particularly when attempting multilocus genotyping (Lecova et al., Reference Lecova, Weisz, Tumova, Tolarova and Nohynkova2018). The lack of complete homologies existing to date further highlights common doubts which appear when attempting to sub-genotype Giardia assemblages using these methods (Bonhomme et al., Reference Bonhomme, Le Goff, Lemee, Gargala, Ballet and Favennec2011). Past and current approaches using intergenic spacer regions have proved successful recently, allowing both assemblage and sub-assemblage typing (Hussein et al., Reference Hussein, Ismail, Mokhtar, Mohamed and Saad2017). The identification of novel target genes suitable for genotyping would be advantageous, along with more reliable methodologies when using tpi, β-giardin or gdh genotyping. This is true for both assemblage and sub-assemblage identification, and is especially true for sub-assemblages of Giardia which pose increased risk to public health (zoonotic assemblages).
As the human-infective forms, assemblages A and B, have the ability to infect both humans and animals, they have been deemed to be zoonotic (Feng and Xiao, Reference Feng and Xiao2011). There is now a wide range of evidence to support this zoonotic potential; however, debate now tends to focus on how commonly zoonotic transfer occurs between various stages of the infection cycle. As the cysts are infectious on excretion, and the same assemblages that infect humans also infect a range of animal hosts, animal-to-animal, animal-to-human transmission and human-to-animal transmissions are all possible; however, how commonly these occur, and in what ratios, is unknown. It is thought that assemblage A is the most likely to be commonly transferred zoonotically into humans due to a wider range of hosts than B, including livestock (Ryan and Cacciò, Reference Ryan and Cacciò2013). However, assemblage B has also been observed in a range of animals as well as humans, which still suggests potential for zoonotic crossovers into humans to a lesser extent than assemblage A.
Furthermore, human-infective assemblages appear to have independent cycles due to their numerous host species, allowing lifecycles within specific reservoirs to carry on for generations before entering human hosts possibly through contaminated water or food for human consumption (Hunter and Thompson, Reference Hunter and Thompson2005; Monis et al., Reference Monis, Cacciò and Thompson2009). It is however largely unknown how these independent lifecycles interact with each other and this must be understood in detail before conclusions can be drawn. Waters et al. (Reference Waters, Hamilton, Sidhu, Sidhu and Dunbar2016) describe mathematic models to highlight that even if the infection cycles do not cross, there is still a high potential for human infection via infected animals.
Livestock
Farm animals and their environments are often suggested as potential reservoirs for human infections (O'Handley et al., Reference O'Handley, Cockwill, McAllister, Jelinski, Morck and Olson1999, Reference O'Handley, Olson, Fraser, Adams and Thompson2000; Appelbee et al., Reference Appelbee, Frederick, Heitman and Olson2003; Ralston et al., Reference Ralston, McCallister and Olson2003; Trout et al., Reference Trout, Santin and Fayer2007; Hoar et al., Reference Hoar, Paul, Siembieda, das Gracas, Pereira and Atwill2009; Santin et al., Reference Santin, Trout and Fayer2009; Sprong et al., Reference Sprong, Cacciò and van der Giessen2009; Miguella et al., Reference Miguella, Wade, Chang, Schaaf and Mohammed2012; Ryan and Cacciò, Reference Ryan and Cacciò2013). This is due to the large numbers of livestock animals present globally in combination with the potential of these animals to create widespread contamination due to infected waste (Ryan and Cacciò, Reference Ryan and Cacciò2013). Their potential to contaminate the environment is however thought to be very dependent on the practices of the farm and region of the world.
It has been previously highlighted that livestock pastures are significant sources of water contamination worldwide (as reviewed by Plutzer et al., Reference Plutzer, Ongerth and Karanis2010). Farm practices that allow cattle direct access to rivers or steams permit defecation directly into water sources, potentially spreading infective cysts downstream to other environments (Budu-Amoako et al., Reference Budu-Amoako, Greenwood, Dixon, Barkema, Hurnik, Estey and Trenton Mcclure2011). A direction to reduce land contamination could be to improve the education of farmers on the subject, however is difficult to enforce in practice. Scotland and indeed other regions of the UK have a rich history of farming practices and large areas of uninhabited countryside, notably the Scottish highlands are often used for grazing livestock. Due to this factor, an increased level of G. duodenalis within these countryside areas, when compared with other more urbanized parts of the UK, would be anticipated.
Studies described to date suggest many livestock animals worldwide are infected with G. duodenalis assemblage E (as reviewed by Feng and Xiao, Reference Feng and Xiao2011; Ryan and Cacciò, Reference Ryan and Cacciò2013), which would be expected due to the assemblage most commonly infecting livestock or hoofed grazing animals as hosts. The potentially zoonotic assemblage A has also been found to be present in some of these livestock animals, such as cattle, and it has been found that in certain cases, co-infections of assemblages A and E are also found (Geurden et al., Reference Geurden, Vanderstichel, Pohle, Ehsan, von Samson-Himmelstjerna, Morgan, Camuset, Capelli, Vercruysse and Claerebout2012).
Several longitudinal studies, as reviewed by Feng and Xiao (Reference Feng and Xiao2011), have found that overall the infection rates within cattle range between 73 and 100% within studied timeframes. Sprong et al. (Reference Sprong, Cacciò and van der Giessen2009) found that in the cattle isolates investigated (total 562), 75 and 23% were assemblages E and A, respectively, within Europe. In contrast, a recent study by Geurden et al. (Reference Geurden, Vanderstichel, Pohle, Ehsan, von Samson-Himmelstjerna, Morgan, Camuset, Capelli, Vercruysse and Claerebout2012) found that 43% of 2072 cattle samples from areas of Europe were infected with assemblage A. This has also been suggested previously by Feng and Xiao (Reference Feng and Xiao2011). Geographical variations in the assemblage types are likely, with Geurden et al. (Reference Geurden, Vanderstichel, Pohle, Ehsan, von Samson-Himmelstjerna, Morgan, Camuset, Capelli, Vercruysse and Claerebout2012) describing assemblage A prevalence to be 61% in France and 41% for Germany, but only 29% within the UK and 28% in Italy (based on β-giardin gene molecular characterization). Similar levels were found using tpi gene molecular characterization on the same samples.
Assemblage B has been identified within some cattle populations in China and also in Canada (Uehlinger et al., Reference Uehlinger, Barkema, Dixon, Coklin and O'Handley2006; Coklin et al., Reference Coklin, Farber, Parrington and Dixon2007; Dixon et al., Reference Dixon, Parrington, Cook, Pintar, Pollari, Kelton and Farber2011; Liu et al., Reference Liu, Zhang, Zhang, Wang, Li, Shu, Zhang, Shen, Zhang and Ling2012). Work in Ontario, Canada, by Dixon et al. (Reference Dixon, Parrington, Cook, Pintar, Pollari, Kelton and Farber2011) however came to the conclusion that within dairy cattle, more so than beef, there was a potential zoonotic risk of Giardia infection for humans. They found that the beef cattle tested within the study were only infected with assemblage E which is of no threat to humans. However, some assemblage B isolates, which are known to infect humans, were found within the dairy livestock.
Most assemblage A-positive cattle tend to be infected with AI (at least within Europe) followed by AII (Sprong et al., Reference Sprong, Cacciò and van der Giessen2009); however, a large number of studies did not confirm the specific sub-assemblage of assemblage A infecting an animal, leaving the zoonotic threat levels somewhat unconfirmed for assemblage A. Sub-assemblage AIII tends to be more restricted to wild animals but is occasionally found in livestock (Cacciò and Sprong, Reference Cacciò, Sprong, Lujan and Svard2011b). A case–control study, by Khan et al. (Reference Khan, Debnath, Pramanik, Xiao, Nozaki and Ganguly2011), found that dairy farm workers in India were infected with a sub-assemblage AI, which suggested that the disease was contracted from cattle contact due to its frequency in the farm cattle. This is inconsistent with a study in Bangladesh, by Ehsan et al. (Reference Ehsan, Geurden, Casaert, Parvin, Islam, Ahmed, Levecke, Vercruysse and Claerebout2015), in which there was no link between cattle and the human population rearing them in the area. In the Bangladesh study, the majority of cattle were infected with assemblage E, whilst the human samples contained human-specific sub-assemblages of assemblages A and B. This would suggest that the two infection cycles within cattle and humans in this area were functioning independently.
The situation is much the same in other livestock/farmyard animals, such as sheep, goats and pigs. These animals are most commonly infected with G. duodenalis assemblage E and infrequently with A (Robertson, Reference Robertson2009). Work by Sprong et al. (Reference Sprong, Cacciò and van der Giessen2009) found a similar situation within sheep and goats as in cattle, where the majority was infected with assemblage A. Most sheep and goats within the study that were positive for assemblage A were shedding sub-assemblage AI (78% of the isolates typed) and the remaining samples (22%) typed as AII. A review of the zoonotic giardiasis threat from sheep by Robertson (Reference Robertson2009) detailed that the majority of sheep were infected with assemblage E. However, by extrapolating data from numerous studies, the author estimated that around 30% of isolates from sheep have the potential to be zoonotic. Pigs have also been found to contain a similar range of assemblages to cattle, sheep and goats, with assemblage E being dominant. Pigs also have been shown to harbour sub-assemblage AI, again similar to other farmyard animals (Feng and Xiao, Reference Feng and Xiao2011). Less common farmyard animals have also been found to be infected, such as alpaca. However, the data for these animals vary with authors suggesting increased or decreased assemblage A and E isolates in different studies (Cebra et al., Reference Cebra, Mattson, Baker, Sonn and Dearing2003; Trout et al., Reference Trout, Santín and Fayer2008; Gomez-Couso et al., Reference Gomez-Couso, Ortega-Mora, Aguado-Martinez, Rosadio-Alcantara, Maturrano-Hernandez, Luna-Espinoza, Zanabria-Huisa and Pedraza-Diaz2012).
A suggestion by Feng and Xiao (Reference Feng and Xiao2011) into the lack of human-infective G. duodenalis isolates being detected in livestock compared with species-specific assemblages is due to competition within hosts. Hosts, which are susceptible to both human-infective assemblages (A) as well as host-specific assemblages (E), may prevent the human-infective assemblage becoming the dominant parasite. This would lead to a decrease of human-infective assemblages in species in which co-infection with a host-specific assemblage of G. duodenalis occurs. This is often seen to be the case, with assemblage E being much more common compared with assemblage A in cattle (Feng and Xiao, Reference Feng and Xiao2011 and Olson et al., Reference Olson, Ryan, Ralston, McAllister and Thompson2004). The hypothesis is not unrealistic as competition between isolates is proven to occur in in vitro cultures (Thompson et al., Reference Thompson, Lymbery, Pearce, Finn, Reynoldson and Meloni1996) and similar situations have been reported for similar protozoan parasites (Mideo, Reference Mideo2009).
Olson et al. (Reference Olson, Ryan, Ralston, McAllister and Thompson2004) suggested that outbreaks could be transmitted by humans to the cattle rather than vice versa. Undoubtedly, the cattle could have contaminated the surrounding area, particularly surface water; however, these animals may have been initially infected due to human practices resulting in land contamination, such as agricultural procedures (e.g. spreading of unsuitably treated manure or slurry to fields, which could contain viable parasite cysts). Olson et al. (Reference Olson, Ryan, Ralston, McAllister and Thompson2004) also mentioned that the infection is difficult to remove once in the cattle population, despite using widespread disinfection and treatment of herds, causing a cycle of re-infection for adult and young cattle alike once introduced by human activity.
Interestingly, Abdel-Moein and Saeed (Reference Abdel-Moein and Saeed2016) reported that in rural areas, a high proportion (62.5%) of the human population with illness were also found to be infected with ‘assemblage E’. This highlights again the lack of understanding of the parasite epidemiology. It also further raises concerns of zoonotic potential, as well as identifying the potential threat livestock pose as a reservoir for human-infective Giardia species.
Detection methods in livestock studies are typically molecular techniques utilizing samples extracted from feces. Infected animals are known to often shed very large numbers of cysts and as such it would be thought that sample concentration/enrichment protocols would not always require to be optimized, as is the case with monitoring of food and water samples. This however is not always the case, as animals are known to have varied shedding patterns, often identified in longitudinal studies, and time periods with very low cyst shedding. Such time periods are suspected to be (Horton, Reference Horton2017) possibly overlooked by a molecular technique utilizing a detection threshold above levels contained within the sample. Due to this, the development of increasingly dependable sample concentration methods are highly desirable, allowing insight into previously undetectable low-level shedding cycles which may exist following, and/or in-between, initial observable infection. Methodologies of cyst extraction and concentration, such as those developed by Wells et al. (Reference Wells, Thomson, Ensor, Innes and Katzer2016) for Cryptosporidium, would be a welcome addition to pre-molecular technique steps for Giardia sample processing. Wells et al. (Reference Wells, Thomson, Ensor, Innes and Katzer2016) observed an increased detection sensitivity from 100 oocysts g−1 of feces, down to 5 oocysts g−1, using this method. Such adaptations, if transferrable to Giardia, could allow the potential identification of positive samples which may be very low in cyst content, giving a better indication of disease progression and infection length.
Little is known about the extent of the livestock disease within the UK. A recent small-scale study in Scotland, using DNA extracted from calf feces, found that 64% of the animals within the sample set were shedding G. duodenalis at some point throughout the time period (Horton, Reference Horton2017). The work, using a pan-assemblage β-giardin G. duodenalis qPCR, found many animals also to have sporadic shedding with positive and negative sample detections on adjacent days. This sporadic shedding pattern, along with a time point of around 4 weeks noted to have increased cyst detection, has also been reported by other authors (Goka et al., Reference Goka, Rolson, Mathan and Farthing1990; Ralston et al., Reference Ralston, McCallister and Olson2003; as reviewed by Johnson et al., Reference Johnston, Ballard, Beach, Causer and Wilkins2003). Whether these animals were truly negative for cyst shedding is debatable, as it is thought likely that the levels of cysts being shed on these ‘negative’ days were simply below the detection threshold of the technique. This again highlights the requirements for employment of efficient concentration steps utilized prior to molecular techniques. Interestingly, two animals in this study were noted to be infected very early in their life (as early as 5 days after birth). This would suggest immediate exposure to cysts within the environment from birth, something additional authors have noted, which highlights the extent of the environmental contamination which can occur with the parasite (Mark-Carew et al., Reference Mark-Carew, Khan, Wade, Schaaf and Mohammed2010).
Companion animals
Companion animals have been thought to be potential sources of infection for human-infective assemblages of G. duodenalis, notably cats and dogs in which there are 77 and 93 million in the USA alone (Esch and Petersen, Reference Esch and Petersen2013). However, studies into giardiasis in dogs and cats are far and few between and often differ in results, similar to other animals.
Both Palmer et al. (Reference Palmer, Traub, Robertson, Devlin, Rees and Thompson2008) and Feng and Xiao (Reference Feng and Xiao2011) undertook comprehensive reviews of the status of companion animals with regards to zoonotic infection with G. duodenalis. In Australia, Palmer et al. (Reference Palmer, Traub, Robertson, Devlin, Rees and Thompson2008) found that when infection was found in a companion animal, it was suspected that it could reflect the practices of human activity in the area. Feng and Xiao (Reference Feng and Xiao2011) found, based on a large number of studies, that when human contact is involved (i.e. dogs owned by humans, etc.) there is an increased likelihood of G. duodenalis assemblage A being present in the animals. Thompson (Reference Thompson2000) found that in urban areas, dogs were just as likely to be infected with assemblage A as they were with the dog-specific assemblage C, and it was speculated that this was due to low infection pressure. They suggested that, similar to cattle with assemblage E, competition may occur with the dog-specific assemblages C and D, resulting in suppressed assemblage A infection and resulting in the situation that whatever assemblage the dog was exposed to, the dog would become infected with. Thomson (Reference Thompson2000) thus considered that the zoonotic potential for G. duodenalis was low in this case, which was supported by Ballweber et al. (Reference Ballweber, Xiao, Bowman, Kahn and Cama2010) who found that much of the published evidence suggested that there is no zoonotic potential.
However, the opposite was found in a small community in India (as reviewed by Palmer et al., Reference Palmer, Traub, Robertson, Devlin, Rees and Thompson2008) where dogs and owners were both found to be infected with assemblage A. This was suggested to be due to the practices of the humans in the area and the availability of human feces for dogs to consume in the community. It is more than likely in this population that the parasite was being passed from human to animal due to poor hygiene. Other papers did show evidence of zoonotic transmission being possible in some communities (Ballweber et al., Reference Ballweber, Xiao, Bowman, Kahn and Cama2010), and Feng and Xiao (Reference Feng and Xiao2011) claimed the presence of assemblage A in dogs will increase the levels of human infection in the population as a consequence. In addition to potential animal–human transmission, Feng and Xiao (Reference Feng and Xiao2011) also noted that there is another infection cycle from dog to dog, passing assemblages C and D to each other, resulting in two potential separate cycles within the canine population.
Cats in various locations worldwide have been found to be most often infected with either the cat-specific assemblage F or A of varying sub-assemblages. Some cats in Europe have also been reported to be infected with assemblages B, C, D and E isolates, albeit much less commonly than assemblages A or F (Palmer, Reference Palmer, Traub, Robertson, Devlin, Rees and Thompson2008; Ballweber et al., Reference Ballweber, Xiao, Bowman, Kahn and Cama2010). Dogs generally come into contact much more frequently with humans due to behavioural habits, such as licking humans after cleaning themselves, which could enable transmission of the parasite from dog to human to be much more frequent (however rare access for dogs to human feces will limit the infection being passed back to the dogs, especially in high-income countries). A large-scale analysis by Bouzid et al. (Reference Bouzid, Halai, Jeffreys and Hunter2015), investigating work which used canine and feline stool samples, found that prevalence rates varied, with at 15.2 and 12% for dogs and cats, respectively. This study highlighted that there was a difference in methods used which impacted on results, suggesting standardization within the community is desirable to better identify the real parasite epidemiology within the animals. The study also observed that in general, dogs kept as pets tended to be less commonly infected, which was not true for cats. Cats are often however allowed to roam outdoor environments by their owners, whereas dogs are usually not, which could explain this difference in exposure. In addition to a need for standardized detection methods, Ballweber et al. (Reference Ballweber, Xiao, Bowman, Kahn and Cama2010) highlighted the lack of data for Giardia in dogs and cats within industrialized nations, which is in need of addressing. Previously, small studies have been carried out within London on the prevalence of Giardia within dogs housed in rescue shelters; however, large-scale country wide data are still lacking. These studies found that the dog-specific assemblages largely predominated this population; however, the presence of a potentially zoonotic assemblage was noted suggesting the possibility of a zoonotic transmission cycle (Sykes and Fox, Reference Sykes and Fox1989; Upjohn et al., Reference Upjohn, Cobb, Monger, Geurden, Claerebout and Fox2010).
Wildlife
Both G. duodenalis assemblages A and B infect wild animals as well as humans and livestock. Feng and Xiao (Reference Feng and Xiao2011) noted that beavers are a source of water contamination. Beavers were also the reasoning behind the World Health Organisation (WHO) classifying giardiasis as a potentially zoonotic disease (Thompson, Reference Thompson2004). The disease is commonly called ‘Beaver fever’ due to the past association with the rodents and disease presence in watersheds. It is not clear whether the beavers introduce the parasite to the watershed, or simply become infected by Giardia already present in the supply (Feng and Xiao, Reference Feng and Xiao2011). Beavers have previously been seen to be infected with both assemblages A and B of the parasite (Sulaiman et al., Reference Sulaiman, Fayer, Bern, Gilman, Trout, Schantz, Das, Lal and Xiao2003; Fayer et al., Reference Fayer, Santin, Trout, DeStefano, Koenen and Kaur2006; Feng and Xiao, Reference Feng and Xiao2011). Recently re-introduction of European beavers into Scotland was formally risk assessed, which included potential effects on public health risk via Giardia and Cryptosporidium within the animals. It was determined that the risk of these animals having a significant impact on drinking water infection with the two protozoan parasites was low (Boden and Auty, Reference Boden and Auty2015).
Non-human primates are known to harbour assemblages A and B and therefore could be involved in zoonotic contamination leading to human disease (Graczyk et al., Reference Graczyk, Bosco-Nizeyi, Ssebide, Thompson, Read and Cranfield2002; Levecke et al., Reference Levecke, Dorny, Geurden, Vercammen and Vercruysse2007; Volotao et al., Reference Volotao, Souza Júnior, Grassini, Peralta and Fernandes2008; Sprong et al., Reference Sprong, Cacciò and van der Giessen2009; Ye et al., Reference Ye, Xiao, Ma, Guo, Liu and Feng2012). Reviews by Ryan and Cacciò (Reference Ryan and Cacciò2013) and Feng and Xiao (Reference Feng and Xiao2011) reported that non-human primates harbour isolates belonging mostly to assemblage B.
Wild ungulates tend to predominantly shed assemblage E whilst wild carnivores appear to be infected with a mix of assemblage isolates, including A, B, C and D. The assemblage infecting them appears to vary with location [surprisingly, African hunting dogs were found mostly not to shed assemblages C and D, but instead shed assemblages A and B (Ash et al., Reference Ash, Lymbery, Lemon, Vitali and Thompson2010)]. Marsupials form a large amount of the native wildlife in Australia and these animals were found to contain mainly isolates of assemblages A and B, much like other reported wildlife worldwide. Shorebirds were also found to be infected with similar assemblage mixes, consisting of A, B or H. Marine mammals such as seals were found to be infected with assemblages including A, B, D and H, dependant on host species (most dolphins and porpoises contained assemblage A isolates, whereas seals were more mixed between assemblages A, B, D and H). When infected with assemblage A, marine species were found to contain either assemblage AI or AII. Work by Ghoneim et al. (Reference Ghoneim, Abdel-Moein and Saeed2012) found evidence that fish, farmed and wild fish, could also be a potential reservoir for the parasite, with potentially human-infective ‘assemblage A’ forms.
Overview of G. duodenalis assemblage, host species and sub-assemblages
Table 1 highlights the different assemblages of G. duodenalis and host species in which they have been documented to infect. If determined by the reference paper, the sub-assemblage has been included, as well as the genotyping gene used and the geographical location of the identification.
Table 1. Overview of G. duodenalis assemblage, host species and sub-assemblages
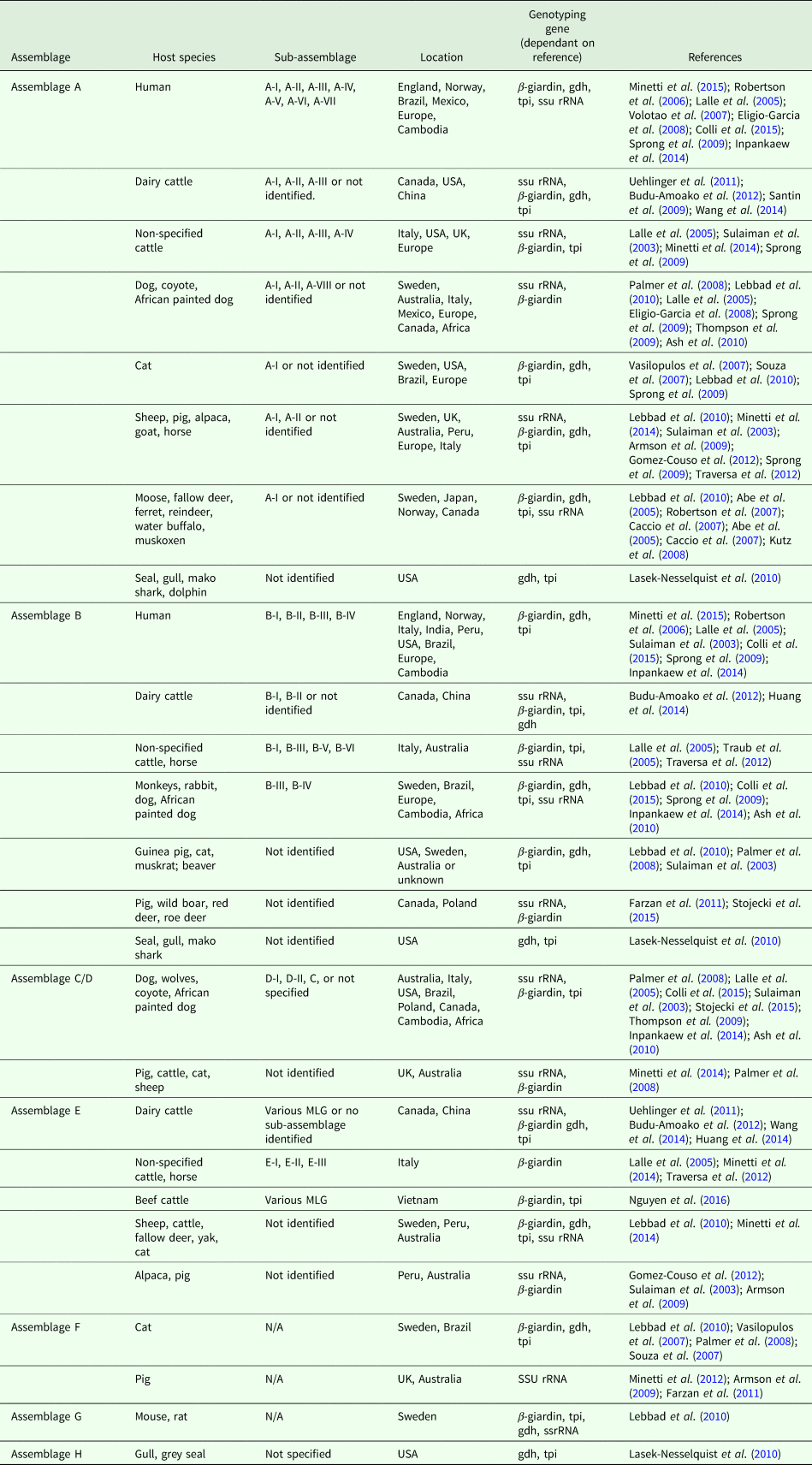
Recommendations
With the increasing evidence to highlight the significant underestimation of giardiasis cases and the emerging threat to public health, improved efforts are urgently required to explore potential sources, identify risk factors and understand transmission routes. Therefore, the following recommendations are highlighted;
(1) All human stool samples submitted for diagnostic testing, irrespective of travel history, should be screened for Giardia cysts. This will permit the accurate reporting of locally acquired cases, in which there is an increasing evidence of within the UK, in addition to travel-associated cases. Improved testing will result in improved patient care through the administration of appropriate drug therapy and will minimize the spread of disease. The majority of stools submitted to diagnostic laboratories are either semi-solid or diarrhoeal; however, even solid stools submitted from cases presenting with bouts of loose/diarrhoeic stools, followed by constipation, have been found to harbour Giardia cysts. Thus, stool consistency cannot be used as a factor to determine Giardia burden, and therefore testing of all submitted stool samples should be encouraged.
(2) To permit further developments, the optimization and standardization of detection methods for isolating and enriching cysts is required using a range of sample types, in particular, those from environmental, drinking water and food sources.
(3) Due to the lack of nationally funded laboratory typing schemes and standardized detection approaches, little is known about the assemblages and sub-assemblages in humans, and those within the environment and the animal kingdom. To fully understand the genetic diversity, the outbreak potential and the geographical, temporal and seasonal influences of variants, molecular typing studies are required to provide much more in-depth information. With the advent of sequencing tools with higher discriminatory power, such as next generation sequencing, in-depth work will be possible which is essential to significantly improve our understanding of this neglected pathogen.
(4) In addition to human studies, detailed molecular analysis of Giardia found in companion animals, livestock, wild animals, birds and fish is essential to permit a fuller understanding of zoonotic transmission routes. Supportive data from these sources will permit a greater understanding of the risks to public health to formulate improved strategies to monitor disease and potential outbreaks.
Financial support
This work received no specific grant from any funding agency, commercial or not-for-profit sectors.
Conflict of interest
None.
Ethical standards
Not applicable.