1. Introduction
A remarkable rise in the reports of noise-associated health perturbations, especially in metropolitan environments with high level of industrialization have been documented in several recent studies (GBD 2019 Hearing Loss Collaborators, 2021). Excessive noise exposure has been shown to disrupt physiological homeostasis, consequently eliciting a cascade of pathological responses prominent among which is hearing loss, diabetes mellitus, aging related stroke, and cardiovascular disease (Floud et al., Reference Floud, Blangiardo, Clark, de Hoogh, Babisch, Houthuijs, Swat, Pershagen, Katsouyanni, Velonakis, Vigna-Taglianti, Cadum and Hansell2013; Liu et al., Reference Liu, Huang, Fang, Zhang, Yang, Xuan, Wang, Lu, Cao, Wang, Li, Sha, Zha, Guo and Wang2018). In addition, continuous subjection to loud noise has been shown to impair growth and significantly decrease the weight of some vital organs in the exposed animals (Jalali et al., Reference Jalali, Saki, Sarkaki, Karami and Nasri2012). Moslehi et al. (Reference Moslehi, Nabavizadeh, Keshavarz, Rouhbakhsh, Sotudeh and Salimi2010) reported that noise may interfere with animal appetite by decreasing the secretion of saliva and gastric fluids, consequently impeding intestinal motility and rate of digestion. Furthermore, noise exposure has been shown to trigger alteration in serum biochemical parameters, DNA damage, and loss of neuronal function (Akefe et al., Reference Akefe, Ayo and Sinkalu2020; Ji et al., Reference Ji, Lee, Wan, Wang, Zhang, Sajjakulnukit, Schacht, Lyssiotis and Corfas2019).
Antioxidants are beneficial in ameliorating the adverse effects induced by noise stress via scavenging free radicals and reducing them to less harmful molecules (Marreiro et al., Reference Marreiro, Cruz, Morais, Beserra, Severo and De Oliveira2017). The flavonoid, kaempferol belongs to the family Zingiberaceae (Bigoniya et al., Reference Bigoniya, Singh and Shrivastava2013) and can be sourced from horseradish, green cabbage, green beans, spinach, olive oil, amaranth, aloe-vera, tea, apple, lettuce, grapes, strawberries, onions, cowpea, broccoli, and leek (Langeswaran et al., Reference Langeswaran, Selvaraj, Ponnulakshmi, Mathaiyan and Vijayaprakash2018). Zinc is a divalent cation, involved in many biochemical and physiological body functions in mammals (Prasad, Reference Prasad2014). Zinc plays a vital modulatory role in the nervous system and notably prevents inhibition mediated by gamma-aminobutyric acid (GABA) by impeding the GABA-A receptor (Andreini & Bertini, Reference Andreini and Bertini2012). Although antioxidant assortment has been suggested to yield synergistic effects in treating different conditions (Yasuno et al., Reference Yasuno, Tanimukai, Sasaki, Ikejima, Yamashita, Kodama, Mizukami and Asada2012), no study is yet to evaluate the effects of combining both metal and flavonoid antioxidants in ameliorating noise-induced deficits in body weight gain and biochemical imbalances in Wistar rats.
2. Objectives
Our objective was to determine the changes in body weight gain and biochemical parameters in Wistar rats administered with kaempferol and zinc gluconate and exposed to ambient noise of approximately 100 dB. We hypothesized that supplementation of kaempferol and gluconate either alone or in combination would be beneficial to ameliorate the negative effects of noise stress in Wistar rats. To examine this hypothesis, we assessed serum biochemical parameters and percentage body weight gain in separate groups of Wistar rats given these antioxidants alone and in combination.
3. Methods
3.1. Ethics statement
The Ahmadu Bello University Committee on Animal Use and Care reviewed and approved all animal procedures (ABUCAUC No.: 02/17/22) in conformity with the National Institutes of Health guide for the care and use of laboratory animals.
3.2. Animals and experimental protocol
Thirty-five 7-week-old male Wistar rats (120 and 125 g) were utilized in this experiment. They were maintained in plastic cages under acceptable laboratory conditions. The rats were examined for health and pathogen-free status and subjected to minimum stress or discomfort throughout the experimental period by ensuring minimal handling. The rats were fed with standard commercially prepared rat pellet and water ad libitum. The animals were randomly grouped into five cohorts of seven rats each as follows: Groups I and II were administered with deionized water; Group III, kaempferol; Group IV, zinc gluconate; Group V, kaempferol + zinc gluconate for 34 days. Groups II, III, IV, and V were subjected to noise stress of 100 dB dose daily for 4 hr per day and for 15 days consecutively, from days 33 to 48 of the experimental period. At the end of the experiment, the animals were anaesthetized by injection of ketamine + xylazine 65/4 mg/kg, i.p. and then sacrificed by severing the jugular. Blood was collected from all the rats and thereafter, serum was retrieved and maintained at 4°C for further analysis.
3.3. Drug and dosage
Kaempferol and zinc gluconate (Aldrich Chemical Co. Ltd., Gillingham Dorset, England, Cat. No. 25,556-4) were reconstituted in deionized water and administered orally at a dose of 5 mg/kg body weight (Bigoniya et al., Reference Bigoniya, Singh and Shrivastava2013; Isaac, Joseph, et al., Reference Isaac, Joseph, Victor, Lamidi and Andrew2017) and 50 mg zinc/kg body weight (Saki et al., Reference Saki, Jalali, Sarkaki, Karami and Ahangarpoor2016) respectively, while the controls were given deionized water (2 ml/kg body weight).
3.4. Noise stress induction
Briefly, noise was produced by a loudspeaker (15 W), mounted 30 cm over the cages, and propelled by a white noise generator (DT-8850; Shenzhen Technology Co. Ltd., Shenzhen, Guangdong, China). The sound intensity was uniformly maintained at 100 dB using a precision sound level meter (Voltcraft SL-200, Hirschau, Germany). During the experiment, the noise level peaked at 100 dB/4 hr/day for 15 days (Akefe et al., Reference Akefe, Ayo and Sinkalu2020; Wankhar et al., Reference Wankhar, Sheela Devi and Ashok2014). Throughout the noise exposure, the control group was kept in a different room with the uniform baseline noise level, and once the noise was switched off, they were returned to the same room with the other groups.
3.5. Measurements
Body weights of all the rats were recorded on days 0, 8, and 15 (following the beginning of noise exposure) using a weighing scale (Scaltec SPO-62; Scaltec Instruments; sensitivity: 0:1 g) and expressed in percentage. The concentrations of total protein, albumin, globulin, cholesterol, triglyceride, low density lipoproteins, high density lipoproteins, and electrolytes (K+, Na+, Cl−, HCO3−) were determined using an automated haematologic analyser (Selectra Junior Spinlab 100, Vital Scientific, Dieren, Netherlands; Spinreact, Girona, Spain) according to the manufacturers’ instructions. Standard controls were run prior each determination, and the values obtained for the different biochemical parameters were always within the normal ranges. To determine the concentration of malondialdehyde, we used the method described by Draper and Hadley (Reference Draper and Hadley1990). The spectrophotometric evaluation of color change during the reaction between thiobarbituric acid (TBA) and malondialdehyde (MDA) was performed. The level of MDA was assessed via the absorbance coefficient of MDA–TBA complex 1.56 × 105/cm, calculated in nmol/mg protein.
3.6. Statistical analysis
Data derived from this study were expressed as mean ± standard error of mean (±SEM). Values were subjected to one-way analysis of variance (ANOVA), followed by Tukey’s post hoc test to evaluate significance of the difference between the group means. GraphPad Prism was used to analyze all the data and values of p < .05 were considered significant.
4. Results
4.1. Effect of treatments on body weight changes
Noise exposure significantly (p < .05) decreased the percentage body weight gain. Conversely, administration of kaempferol and zinc gluconate singly, and in combination significantly (p < .05) improved percentage body weight gain (Figure 1).
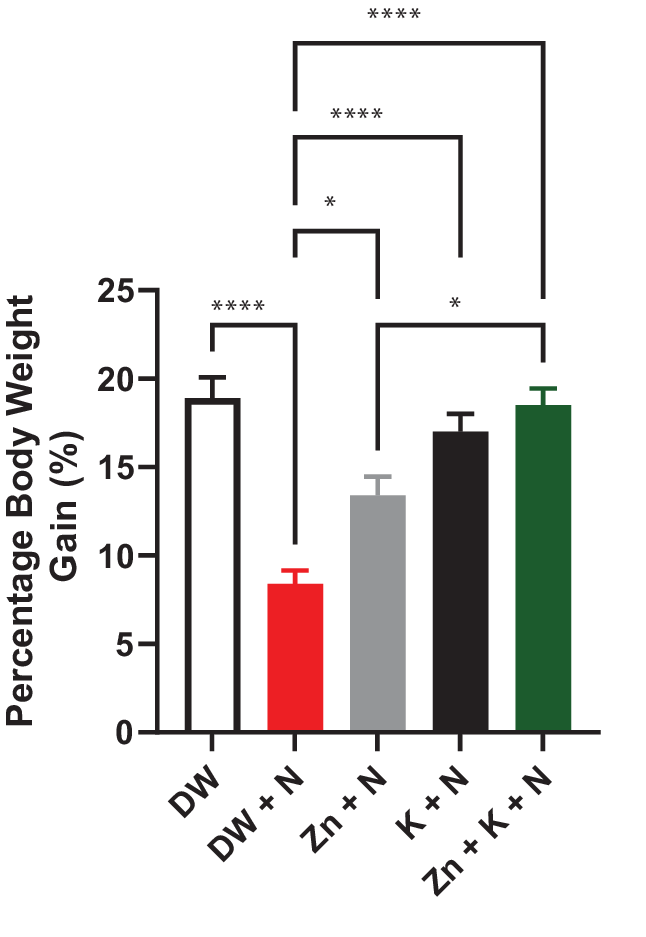
Figure 1. Effects of kaempferol and zinc gluconate on percentage body weight gain in male Wistar rats exposed to noise stress (mean ± SEM, n = 7). *p < .05 and ****p < .0001 are significantly different compared to control group (DW + N). DW, deionized water; K, kaempferol; N, noise; ZnG, zinc gluconate.
4.2. Effects of treatments on serum electrolytes concentration
The individual serum electrolyte concentrations in the DW + N group decreased marginally when compared to the other treatment groups, however, this change was not significant (p > .05) between the groups (Figure 2).
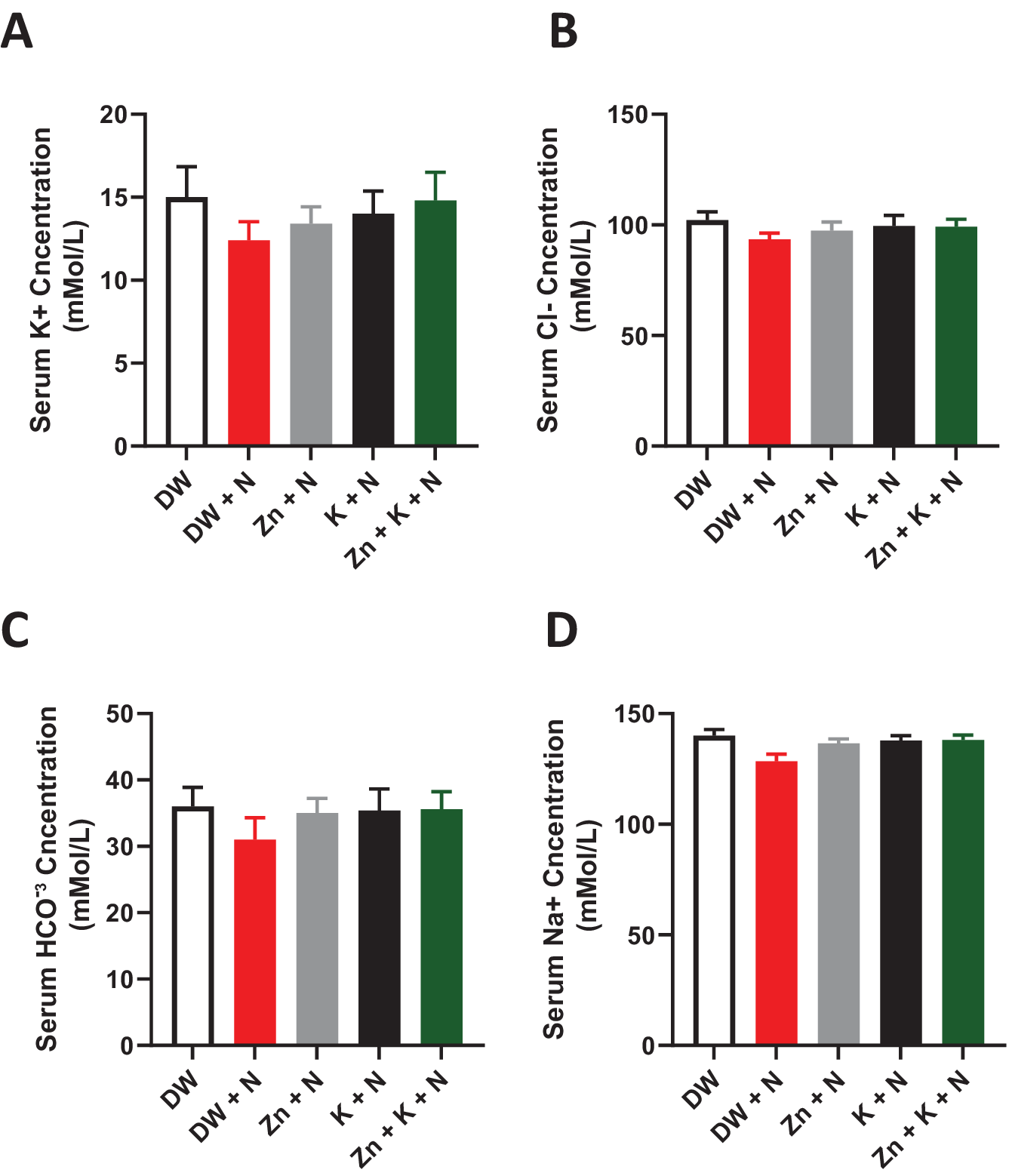
Figure 2. Effects of kaempferol and zinc gluconate on serum electrolyte concentration (mMol/L−) in Wistar rats exposed to noise stress. (a) Potassium (K+), (b) chloride (Cl−), (c) bicarbonate (HCO−3), and (d) sodium (Na+). DW, deionized water; K, kaempferol; N, noise; Zn, zinc gluconate (mean ± SEM, n = 7).
4.3. Effect of treatments on glucose concentration
There was a significant (p < .05) decrease in serum glucose concentration in the groups treated with DW, K + N, and K + Zn + N, compared to the DW + N, group (Figure 3). The lowest concentration of glucose was obtained in the group treated with deionized water only (75.21 ± 2.4 mg/dl), whereas the group treated with DW + N had the highest concentration (104.31 ± 31 mg/dl).
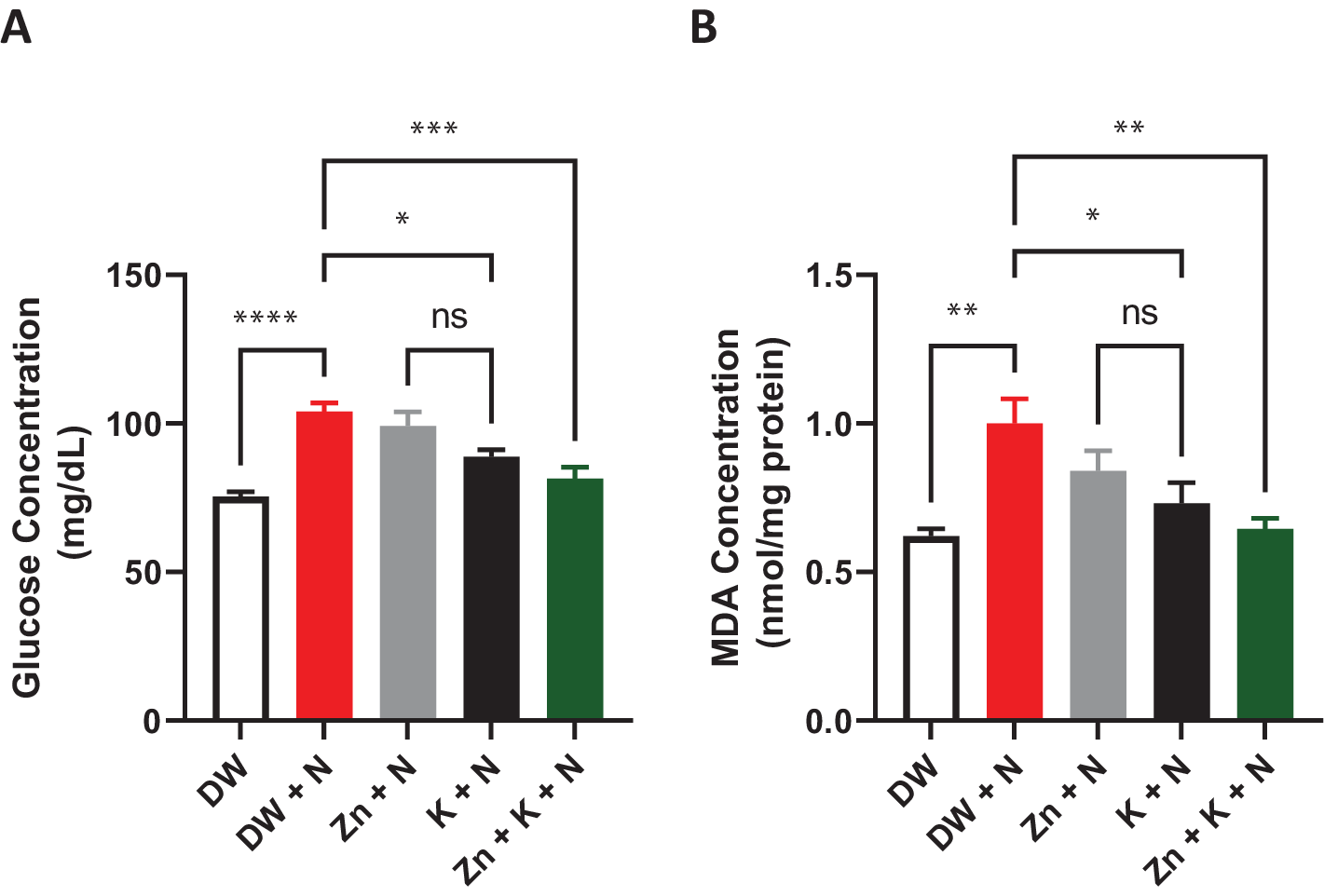
Figure 3. Effects of kaempferol and zinc gluconate on serum (a) glucose concentration (mg/dl) and (b) malondialdehyde concentration (MDA) (nmol/mg) in Wistar rats exposed to noise stress (mean ± SEM, n = 7). *p < .05, **p < .01, ***p < .001, and ****p < .0001 are significantly different compared to control group (DW + N). DW, deionized water; K, kaempferol; N, noise; Zn, zinc gluconate.
4.4. Effects of treatments on concentration of serum protein fractions
The serum total protein was decreased significantly (p < .05) in the group treated with DW + N, compared to the Zn + K + N group. There was no significant (p > .05) difference in serum total protein concentration in the DW + N group, compared with Zn + N and K + N groups (Table 1). Serum albumin concentration was significantly higher (p < .05) in the K + N (3.89 ± 0.21; 7.3%) and Zn + K + N (3.93 ± 0. 15; 10.6%) groups, compared to the group treated with DW + N, which had the least serum albumin concentration (1.73 ± 0.13). There was no significant (p > .05) difference in serum globulin concentration between the groups (Table 1). There was no statistically significant difference between Zn + K + N group and the DW group for all the assayed protein fractions.
Table 1. Ameliorative effect of kaempferol and zinc gluconate on concentration of serum protein fractions (mg/dl) of Wistar rats exposed to noise stress (mean ± SEM, n = 6)
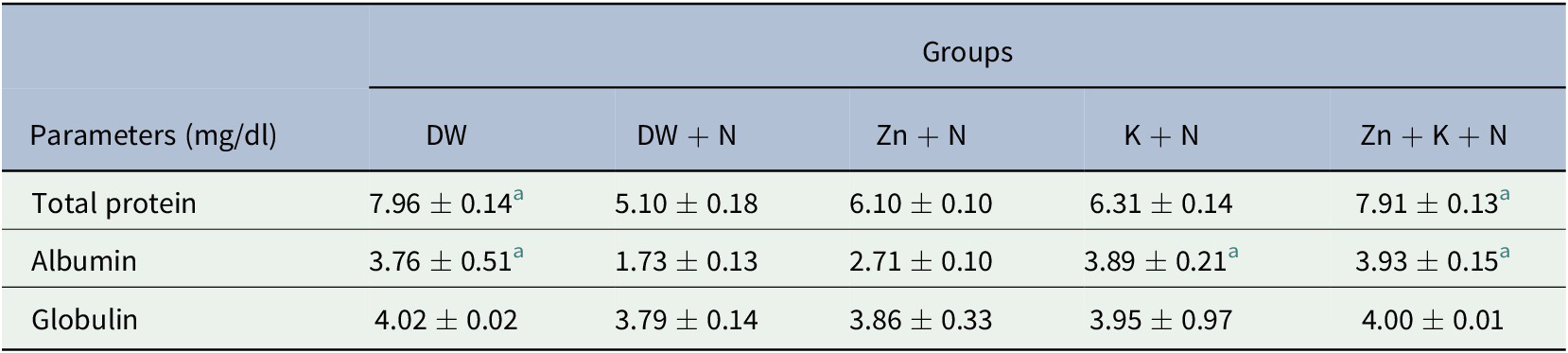
Abbreviations: DW, deionized water; K, kaempferol; N, noise; Zn, zinc gluconate.
a Values that are significantly (p < .05) different compared to the DW + N group.
4.5. Effects of treatments on concentration of serum lipid fractions
Cholesterol and triglyceride levels in serum was significantly lower (p < .05) in the other groups except for Zn + N group when compared to the DW + N group. The lowest concentrations of cholesterol and triglyceride was detected in the DW group (96.18 ± 4.18 mg/dl and 119.04 ± 3.57 mg/dl, respectively) while the highest concentration was observed in the DW + N group (168.54 ± 9.26 mg/dl and 189.16 ± 8.43 mg/dl, respectively). The lowest high-density lipoprotein (HDL) concentration was detected in the DW + N group (17.42 ± 1.14 mg/dl) while Zn + K + N group had the highest concentration (34.17 ± 1.04 mg/dl). The difference in the levels of HDL between these two groups was statistically significant (p < .05). The highest level of low-density lipoprotein (LDL) was seen in the DW + N group (110.67 ± 4.23 mg/dl), and this was significantly higher (p < .05) compared to the other groups. The lowest LDL level was obtained in the DW group (32.43 ± 1.27 mg/dl). There was no statistically significant difference between Zn + K + N group and the DW group for all the assayed lipid fractions (Table 2).
Table 2. Ameliorative effect of kaempferol and zinc gluconate on concentration of serum lipid fractions (mg/dl) in Wistar rats exposed to noise stress (mean ± SEM, n = 6)
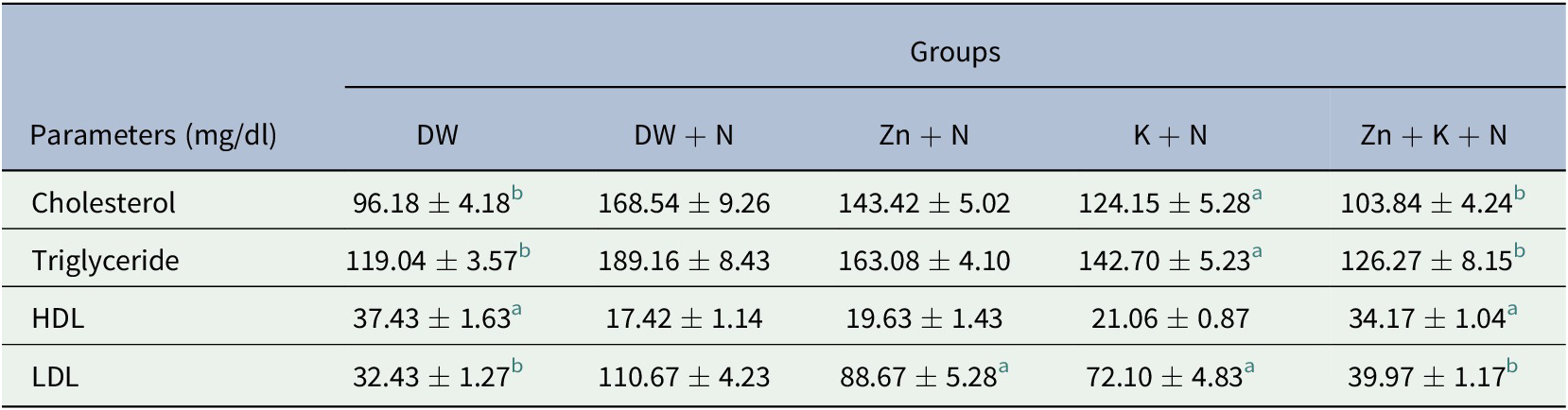
Abbreviations: DW, deionized water; HDL, high-density lipoprotein; K, kaempferol; LDL, low-density lipoprotein; N, noise; Zn, zinc gluconate.
a,b Values with different superscript letters are significantly (p < .05) different compared to the DW + N group.
4.6. Effects of treatment on MDA Concentration
The MDA concentration decreased significantly (p < .05) in rat groups treated with kaempferol + zinc gluconate + noise (0.64 ± 0.20 nmol/mg), compared to the concentrations recorded in deionized water + noise group (1.0 ± 0.20 nmol/mg), which was significantly (p < .05) higher than in both the deionized water-treated group (0.62 ± 0.10 nmol/mg) and kaempferol + noise group (0.64 ± 0.20 nmol/mg). The difference in MDA concentrations between the group administered with deionized water and that treated with kaempferol + zinc gluconate + noise was insignificant.
4.7. Relationship between MDA concentration and body weight gain
A strong correlation was observed between the concentration of MDA and body weight gain in the different treatment groups. Pearson’s correlation coefficient of r = 0.7570 and p = .0001 was observed (Figure 4).
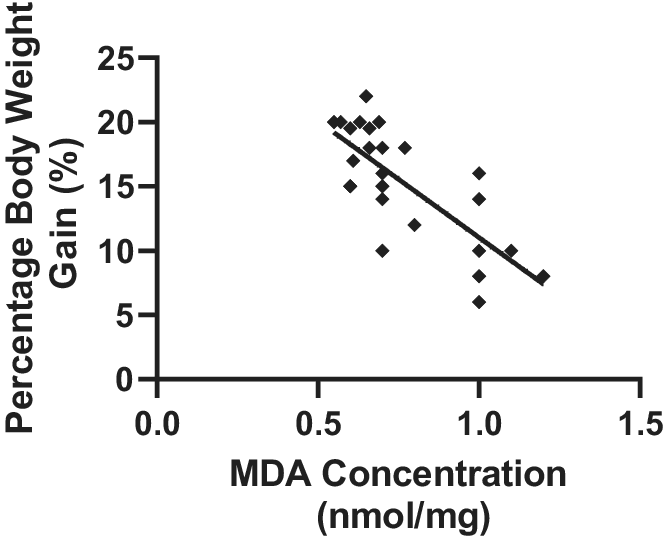
Figure 4. Relationship between MDA concentration and percentage body weight gain in Wistar rats exposed to noise stress (mean ± SEM, n = 7).
5. Discussion
Variation in body weight gain alongside biochemical parameters is considered vital for the assessment of stress and degree of oxidative impairment. Results from this study showed that the body weight gain of male rats was significantly decreased in the group exposed to noise without antioxidant treatment, indicating that noise exerts a negative effect on the growth and development of rats. This corroborates previously reported studies (Jalali et al., Reference Jalali, Saki, Sarkaki, Karami and Nasri2012; Taban et al., Reference Taban, Mortazavi, Vosoughi, Khavanin and Asilian Mahabadi2017) which affirm the inhibitory effect of noise on body weight gain in exposed subjects. Our results demonstrate that co-administration of kaempferol and zinc gluconate significantly improved noise-induced deficit in body weight gain observed in the exposed rats. To the best of our knowledge, this is the first time this has been reported. The mechanism by which this antioxidant assortment exerts its effect may be by improving basal metabolism, appetite, and gastrointestinal motility in the treated rats (Isaac, Ibrahim, et al., Reference Isaac, Ibrahim, Andrew, Ibrahim, Edward and Solomon2017; Zhang et al., Reference Zhang, Gong, Zhang, Song, Xu, Cai, Tang, Zhang, Liu, Jia and Zhang2015).
The dyshomeostasis observed in some of the biochemical parameters evaluated in the present study indicates that aside from noise-induced hearing loss, noise induces other biochemical perturbations in the exposed animals. The observation of no significant change in serum electrolyte concentrations recorded in this study corroborates the report of Zare et al. (Reference Zare, Nassiri, Monazzam, Pourbakht, Azam and Golmohammadi2016), who reported that occupational noise exposure had no significant effect on serum potassium electrolyte. However, the fact that there were marginal alterations in levels of electrolytes should not be totally ignored as this may indicate that noise exposure triggers some level of metabolic changes which is reflective of endogenous mechanisms of oto-protection in the exposed animals (Ji et al., Reference Ji, Lee, Wan, Wang, Zhang, Sajjakulnukit, Schacht, Lyssiotis and Corfas2019). Our study also revealed an increase in blood glucose levels in rats exposed to noise without any antioxidant treatment. This result suggests that noise affects glucose metabolism, which stems from the loss of insulin-sensitivity associated with noise exposure as seen in previous studies that highlighted the role of chronic noise exposure in diabetogenesis, and noise-associated increase in blood lipid levels (also observed in our study; Dzhambov, Reference Dzhambov2015; Liu et al., Reference Liu, Huang, Fang, Zhang, Yang, Xuan, Wang, Lu, Cao, Wang, Li, Sha, Zha, Guo and Wang2018). The increased glucose level was reversed to normal in the group administered a combination of both antioxidants, and this gives credence to previous studies which suggested that kaempferol (Al-Numair et al., Reference Al-Numair, Chandramohan, Veeramani and Alsaif2015) and zinc (Ranasinghe et al., Reference Ranasinghe, Pigera, Galappatthy, Katulanda and Constantine2015) possess antidiabetic properties, consequently, their combination yielded an improved effect.
Importantly, this study also demonstrated that chronic exposure to noise initiated considerable decrease in the concentration of serum total protein, partly owing to the detrimental effects of noise on the associated body tissues and hypoalbuminaemia because of a decrease in the synthesis of albumin by the liver following impaired hepatic function. Ji et al. (Reference Ji, Lee, Wan, Wang, Zhang, Sajjakulnukit, Schacht, Lyssiotis and Corfas2019) submitted that impaired metabolism may signify oxidative damage and perturbed metabolism of protein, induced by noise. Co-administration of kaempferol and zinc gluconate improved the decreased levels of serum total protein and albumin, arising from noise exposure.
Previously, Melamed et al. (Reference Melamed, Froom, Kristal-Boneh, Gofer and Ribak1997) reported a significantly higher levels of cholesterol, triglyceride, LDL, and HDL, mainly in younger men exposed to high industrial noise. Similarly, our study showed a significant increase in cholesterol, triglyceride, LDL, and HDL in rats exposed to noise. This alteration in serum lipid concentrations may be attributed to impairment of the metabolism of lipids alongside increased peroxidation of membrane lipids, associated with noise exposures (Akefe et al., Reference Akefe, Yusuf and Adegoke2019; Karafakioğlu, Reference Karafakioğlu2019). Co-administration of kaempferol and zinc gluconate in noise-exposed rats, ameliorated the increase in serum lipids observed in this study, thus suggesting their potential in maintaining metabolic homeostasis.
The increase in MDA concentration demonstrated that noise stress induced an increase in ROS-induced cell damage and death (Henderson et al., Reference Henderson, Borba, Daley, Boxill, Nguyen, Culhane, Louie, Cather, Evins, Freudenreich, Taber and Goff2006; Lamidi et al., Reference Lamidi, Hudu, Akefe, Adamu and Salihu2020; Zhang et al., Reference Zhang, Gong, Zhang, Song, Xu, Cai, Tang, Zhang, Liu, Jia and Zhang2015). The significant decrease in MDA observed in the group treated with kaempferol + zinc gluconate, compared to the groups separately treated with kaempferol and zinc gluconate, indicated that combination of kaempferol and zinc yielded an improved outcome in ameliorating the adverse effects of noise stress, induced via increased lipid peroxidation (Tu et al., Reference Tu, Lian, Yen, Chen and Wu2007). The result of the present study also agrees with those of Khalaf et al. (Reference Khalaf, Ibrahim, Tohamy, Allah and Zaki2017) and Nunes et al. (Reference Nunes, Pasko, Tyszka-Czochara, Szewczyk, Szlosarczyk and Carvalho2017), who reported that zinc supplementation considerably increases activities of oxidative stress-related enzymes and stabilizes the concentration of MDA, thus signifying its antiperoxidative potential. Overall, the result of the present study demonstrates that consumption of foods, containing the antioxidants, kaempferol, and zinc, may be beneficial to high noise-risk populations.
6. Conclusion
Exposure of Wistar rats to noise significantly decreased body weight gain and altered the concentrations of serum lipid and protein fractions. Treatment with kaempferol and/or zinc ameliorated noise-induced alteration in body weight gain, serum glucose, lipid and protein, and MDA levels. This suggests that biochemical dyshomeostasis and lipid peroxidation may be involved in the molecular mechanism underlying noise stress. Single administration of kaempferol was more effective than zinc gluconate, while their assortment produced an improved outcome in mitigating the adverse effects of noise stress in Wistar rats.
Acknowledgments
The authors would like to acknowledge the Faculty of Veterinary Medicine, Ahmadu Bello University, Zaria, for providing the facilities used for this research.
Funding Statement
This research received no specific grant from any funding agency, commercial or not-for-profit sectors.
Conflict of Interest
The authors declare no conflict of interest.
Authorship Contributions
J.O.A. and I.O.A. conceived and designed the study. V.O.S., I.O.A., and J.O.A. conducted data gathering, V.O.S. performed statistical analyses, V.O.S., J.O.A., and I.O.A. wrote the article.
Data Availability Statement
The authors confirm that the data supporting the findings of this study are available within the article.
Comments
Comments to the Author: As kaempferol is insoluble in water, why was deionized water used as its vehicle and administered to control group as vehicle control? Improper solubility of kaempferol in this vehicle also questions the homogeneity of dosage between different rats.
Important parameters of ambient stress like cortisol level, T-4/ T-3 level, heat shock proteins should be determined.
Results of feed intake is missing.
Levels of leptin should be assayed.
What about the weight of different organs?
How did authors access the hearing loss in the rats as is claimed in the manuscript?
How do authors justify oxidative imbalance just by assaying LPO and excluding several other parameters of oxidative stress?
Page 5 line 125-126 is not clear. It seems contradictory to the preceding sentence.
Different superscripts should be provided on the result of different groups presented in figures/ tables. Every group should be statistically compared with other groups.
Overall the study is too superficial.