INTRODUCTION
The therapeutic, prophylactic and metaphylactic use of antimicrobials is common practice in modern food-animal husbandry [Reference Berge, Atwill and Sischo1–3]. Concerns have grown that this widespread use of antimicrobial drugs may lead to an increase in antimicrobial resistance in numerous bacteria potentially affecting public health [4, Reference Jordan5]. Standardized and continuous surveillance programmes are necessary to monitor the occurrence and persistence of antimicrobial resistance in food animals [3, Reference Aarestrup, Butaye, Witte, Gilmore, Clewell, Courvalin, Dunny, Murray and Rice6, Reference Wallmann7]. Indicator bacteria are generally used to monitor antimicrobial resistance since they can be commonly found in healthy animals. In addition, these bacteria acquire antimicrobial resistance faster than other commonly found bacteria [Reference Aarestrup, Butaye, Witte, Gilmore, Clewell, Courvalin, Dunny, Murray and Rice6, 8, Reference Miranda9]. Commensal Escherichia coli and Enterococcus faecalis are internationally used as respective Gram-negative and Gram-positive indicator bacteria for monitoring antimicrobial resistance because of their common presence in the animal intestinal tract [Reference Wray and Gnanou10–Reference de Jong12]. Surveillance of antimicrobial resistance is performed in several countries [Reference Aarestrup2], yet these surveillance programmes have generally been focused on cattle, pig and broiler production. Programmes in laying hens are still scarce. Therefore, there is a need to monitor antimicrobial resistance development in laying hens [3].
According to the Council Directive 1999/74/EC, from January 2012 onwards conventional battery cages will be forbidden in the European Union (EU) [Reference Wall, Tauson and Elwinger13]. Only enriched cages and non-cage housing systems will be allowed. Non-cage housing systems consist of an indoor area that may or may not be combined with covered (‘wintergarden’) or uncovered (‘free-range’) outdoor facilities [14, 15]. The non-cage systems can be categorized into two groups: single level systems with a ground floor area which is fully or partially covered with litter and aviaries, consisting of a ground floor area plus one or more platforms [14, 15]. Free-range organic flocks have the same structure as a free-range system but there are some additional requirements concerning maximum flock size, beak trimming and the origin of the feed. Moreover, the application of antimicrobials in these organic flocks is strictly restricted to therapeutic usage. Presently it is not clear whether the different housing and management systems for laying hens will influence the occurrence of antimicrobial resistance. Recent reports indicate that both in poultry and other animal species the move from conventional indoor production towards free-range and organic production exerts a beneficial effect on the levels of antimicrobial resistance in zoonotic and indicator bacteria [Reference Ray16–Reference Young19]. On the other hand it has been shown that this move to non-cage housing systems resulted in an increased incidence of bacterial diseases [Reference Fossum20, Reference Kaufmann-Bart and Hoop21], which could potentially lead to increased antimicrobial usage. However, epidemiological data on the prevalence of antimicrobial resistance in the above-mentioned indicator bacteria in laying hens in different housing systems is still limited.
The aim of this paper was to investigate the prevalence of antimicrobial resistance in E. coli and E. faecalis isolates recovered from 283 laying flocks in four European countries and to evaluate the potential association between housing systems and observed multi-drug resistance.
MATERIAL AND METHODS
Farm selection
Flocks were selected from a registry list of laying-hen farms provided by the official identification and registration authorities of the participating countries (Belgium, Germany, Italy, Switzerland). The farm size (>1000 laying hens) and the housing type were the only two selection criteria. The distribution of sampled farms aimed for was 20% conventional battery cage systems and 80% non-cage (alternative) housing systems. Within the group of alternative housing systems an equal number of aviaries, raised-floor, free-range and organic farms was targeted. The farmers were contacted by telephone and the purpose of the study was explained. The planned date of depopulation was noted to make sure that the farm could be sampled in the month prior to depopulation.
Sampling of the laying-hen farms
Four hens in the sampled flock were evenly selected throughout the house and from each hen one cloacal swab was taken by inserting a sterile cotton-tipped swab about 5 cm into the cloaca. The swabs were directly placed in individual tubes containing Ames medium.
A questionnaire was completed during an on-farm interview at the same time that collection of samples took place. The questionnaire consisted of 92 questions, with 31 open questions and 61 closed questions. Prior to the study, the questionnaire was tested on two Belgian laying-hen farms, one with conventional battery cages and one with a free-range production system in order to check whether the questions were relevant to the aim of this study. The questions were related to general farm and flock characteristics such as flock size, breed, age of hens and biosecurity measures. Special attention was paid to the housing system of the sampled flock and the antimicrobial treatments the hens had received during the current production cycle. The same questionnaire was used in all participating countries; a translation from English to Dutch, German and Italian was performed during a meeting with all participating countries. For each of the participating countries one person was designated to collect the samples and conduct the interview with the farmer. Questionnaires can be obtained from corresponding author upon request.
Bacteriological examination of the samples
E. coli
For the isolation of E. coli the swabs were plated onto MacConkey plates (Oxoid®, France) and incubated aerobically for 24 h at 37°C. From each primary plate, one colony was selected and plated again onto a MacConkey agar plate. Suspected colonies were confirmed as E. coli by positive glucose/lactose fermentation, gas production and absence of H2S production on Kligler iron agar (Oxoid) and absence of aesculin hydrolysis (bile aesculin agar; Oxoid). In Switzerland, the following equivalent method was used: the cloacal swabs were plated onto MacConkey agar (Oxoid) and incubated for 24 h at 37°C under aerobic conditions. Strains which were lactose-positive were subcultured on Brolacin agar (Merck®, Germany) and incubated for 24 h at 37°C under aerobic conditions. Confirmation of the strains as E. coli was performed using RapiD 20 E (bioMérieux, France).
E. faecalis
For the isolation of Enterococcus the swab was plated on Slanetz & Bartley agar (Oxoid). After incubation for 48 h at 42±1°C, one suspected E. faecalis colony per sampled animal was purified and verified by using Rapid ID 32 STREP strips (bioMérieux). In Switzerland, E. faecalis was isolated from the cloacal swabs on Enterococcosel agar (Becton, Dickinson and Company, USA) and incubated for 48 h at 37°C under aerobic conditions. On Columbia 5% sheep blood agar (Oxoid) strains were subcultured and incubated for 24 h at 37°C under anaerobic conditions. Confirmation of the strains as E. faecalis was performed using Rapid ID 32 STREP strips (bioMérieux).
Antimicrobial susceptibility testing
For both indicator bacteria, susceptibility was tested by means of broth microdilution using custom made Sensititre® plates (Trek Diagnostics Systems Ltd, UK). The antimicrobials tested and their ranges are listed in Tables 1 and 2 for E. coli and E. faecalis, respectively. The results were read visually after 24 h incubation at 37°C and the minimum inhibitory concentration (MIC) was defined as the lowest concentration of the antimicrobial that completely inhibited visible growth. E. coli ATCC 25 922 and E. faecalis ATCC 29 212 were used for quality control. The European Committee on Antimicrobial Susceptibility Testing (EUCAST) epidemiological breakpoints for MIC determination were used. When these EUCAST breakpoints were not available (which was the case for apramycin, sulfamethoxazole and kanamycin), the breakpoints mentioned in the reports of the Danish Integrated Antimicrobial Resistance Monitoring and Research Programme were used [22].
Table 1. Minimum inhibitory concentration (MIC) distribution (%) for 1102 Escherichia coli isolates (vertical black line indicates cut-off value)

Table 2. Minimum inhibitory concentration (MIC) distribution (%) for 792 Enterococcus faecalis isolates (vertical black line indicates cut-off value)

Statistical data analysis
An isolate was defined as multiple drug-resistant (MDR) if it exhibited resistance to two or more antimicrobials. For both the E. coli and E. faecalis models, MDR was used as an outcome variable in statistical analysis. For E. coli, ceftiofur resistance was also used as an outcome variable. Three separate statistical models were developed to test these outcome variables. The questionnaire responses were transformed to binary or categorical variables. Pearson's χ2 test was used to determine potentially significant differences between categorical explorative variables (P<0·05), and those factors that were significant in this univariate analysis were further tested in the multivariate models. The predictive categorical factors, consisting of country, housing type, presence of other farm animals (e.g. pigs, cattle, sheep) on farm, the presence of hens in the flock originating from different rearing sites and antimicrobial treatment of the flock were tested for inclusion in the models. A stepwise forward selection process was used for the variable selection in a population average logistic regression model with a P value ⩽0·2 for entry, and with a P value ⩽0·10 for retention in the model. This model did not control for clustering of isolates within farms but was used for preliminary testing of significant predictive factors. The factors that were significant in this model were introduced into a model using generalized estimating equations (GEE) to control for clustering of samples within a farm using an independent correlation matrix. Interaction effects were tested for variables retained in the final GEE model. Odds ratios and 95% confidence intervals were calculated for the parameters that were retained in the GEE model and only factors with a P value ⩽0·05 were retained in the final GEE model. The statistical software package SAS (SAS for Windows, version 9.1, SAS Institute Inc., USA) was used for data analysis.
Antimicrobial resistance patterns were described using cluster analysis. Binary cluster analysis was performed using the Jaccard matching coefficient and the centroid method to obtain discrete clusters with no intra-cluster variability. Due to the large number of antimicrobial resistance clusters, the descriptive and stratified analysis was limited to the 15 most common resistance patterns describing more than 80% of the isolate dataset.
RESULTS
In total, 1102 E. coli isolates and 792 E. faecalis isolates were collected from 283 laying-hen flocks (69 Belgian, 85 German, 30 Italian and 99 Swiss flocks). The participation rate was more than 90% in Belgium, Italy and Switzerland and 70% in Germany. A detailed description of the number of isolates per housing type and per country is presented in Table 3. The median flock size of the sampled flocks was 7612 laying hens (range 1000–84 000 hens). The flock size in conventional battery cage houses was significantly higher than the flock size in non-cage systems. Twenty-four of the 283 sampled flocks were treated with antimicrobials during the current production cycle according to the declaration of the farmers. The number of treated flocks differed significantly between countries (P<0·05) but not between housing types. In Italy none of the sampled flocks were treated with antimicrobials, for Belgium this was 3/69 sampled flocks, for Switzerland 5/99 flocks and for Germany 16/85 sampled flocks. Colistin was the most frequent treatment (16 flocks), followed by amoxicillin (five flocks), neomycin (two flocks) and enrofloxacin (one flock). There was a significant difference between countries in the number of laying-hen farms where other production animals were also kept (P<0·05). In Italy, only 16·7% of the sampled laying-hen farms managed other animal production on the same site, whereas in Germany, Belgium and Switzerland this was 38·5%, 43·8% and 75·5%, respectively.
Table 3. Number of isolates per country and per housing type for Escherichia coli and Enterococcus faecalis

Success rate of isolation for E. coli=97·3%; for E. faecalis=70·0%.
The distribution of the MICs (in %) of each antimicrobial is described in Table 1 for E. coli and in Table 2 for E. faecalis.
MDR in E. coli
The majority of the isolates (55·0%) were susceptible to all 18 antimicrobials, 16·9% were resistant to one antimicrobial and the remaining 28·1% were multi-resistant. The 15 most common resistance phenotypes of antimicrobial resistance clusters are described in Table 4. The housing of hens in raised-floor systems, compared to conventional battery cages (P=0·02) and country (P=0·03) turned out to be risk factors for higher levels of MDR in the final GEE logistic regression model (Table 5). Other factors such as other animal production on the farm, the presence of hens originating from different rearing farms in the sampled flock and antimicrobial treatment of the flock were not retained in the final model. When looking at factors affecting ceftiofur resistance in E. coli, Belgium was more likely to have ceftiofur-resistant isolates than the other three countries. Ceftiofur resistance varied from 0·0% (Switzerland), >2·5% and 4·5% (Italy and Germany, respectively), to 12·1% in Belgium. No significant potential association between the other tested risk factors and ceftiofur resistance was observed.
Table 4. The 15 most common antimicrobial resistance clusters in Escherichia coli isolated from European laying hens

AMC, Amoxicillin/clavulanic acid; AMP, ampicillin; APR, apramycin; CEF, cefpodoxime; CEP, cefalothin; CHL, chloramphenicol; CIP, ciprofloxacin; COL, colistin; FLO, florfenicol; GEN, gentamicin; NAL, nalidixic acid; NEO, neomycin; SPT, spectinomycin; STR, streptomycin; SUL, sulfamethoxzole; TET, tetracycline; TRI, trimethoprim; XNL, ceftiofur.
* AR cluster=antimicrobial resistance cluster describing the pattern of resistance of the isolates.
† AR freq=antimicrobial resistance frequency: the number of antimicrobials to which the E. coli were classified as resistant (R).
‡ The number and percentage of isolates described in this table represent 81·8% of the 1102 total isolates included in this study.
Table 5. Results of the GEE logistic regression analysis for the identification of risk factors for the presence of multiple drug resistance in Escherichia coli from European laying hens

OR, Odds ratio; CI, confidence interval.
MDR in E. faecalis
The majority of E. faecalis isolates were multi-resistant (51·1%), 34·5% were resistant to one antimicrobial and only 14·4% of all isolates were pan-susceptible. The 15 most common resistance phenotypes of antimicrobial resistance clusters are described in Table 6. The results of the GEE logistic regression model, showing factors associated with MDR in E. faecalis are presented in Table 7. The isolates from laying hens housed in free-range systems were more likely to have lower levels of MDR (P=0·03) compared to conventional battery cage systems. Isolates from Belgian hens had lower levels of resistance than hens in Germany and Italy. Similar to the observations in E. coli, other factors such as other animal production on the farm, antimicrobial treatment of the flock and the presence of hens originating from different rearing plants in the flock did not significantly interact with the levels of MDR.
Table 6. The 15 most common antimicrobial resistance clusters in Enterococcus faecalis isolated from European laying hens
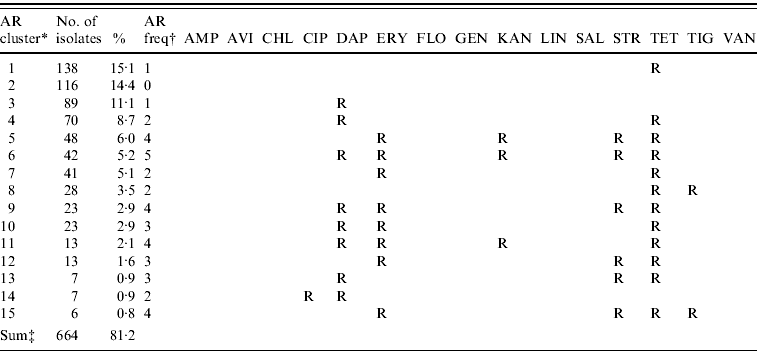
AMP, ampicillin; AVI, avilamycin; CHL, chloramphenicol; CIP, ciprofloxacin; DAP, daptomycin; ERY, erythromycin; FLO, florfenicol; GEN, gentamicin; KAN, kanamycin; LIN, linezolid; SAL, salinomycin; STR, streptomycin; TET, tetracycline; TIG, tigecyclin; VAN, vancomycin.
* AR cluster=antimicrobial resistance cluster describing the pattern of resistance of the isolates.
† AR freq=antimicrobial resistance frequency: the number of antimicrobials to which the E. faecalis were classified as resistant (R).
‡ The number and percentage of isolates described in this table represent 81·2% of the 803 total isolates included in this study.
Table 7. Results of the GEE logistic regression analysis for the identification of risk factors for the presence of multiple drug resistance in Enterococcus faecalis from European laying hens

OR, Odds ratio; CI, confidence interval.
DISCUSSION
The levels of MDR in E. faecalis were lower in free-range laying hens than in the conventional battery cage system, whereas increased levels of MDR were seen in E. coli in raised-floor hens. This is in contrast to the studies of Schwaiger et al. [Reference Schwaiger, Schmied and Bauer17, Reference Schwaiger, Schmied and Bauer18] who found significantly lower levels of antimicrobial resistance in E. coli and faecal enterococci in free-range organic laying hens compared to laying hens housed in conventional battery cages. There are several possible explanations for the ambiguous association between the non-cage housing types and the level of MDR. A first important factor is exposure to antimicrobials. In the current study, apart from the free-range organic flocks, the reported antimicrobial use in laying hens in the non-cage systems was not significantly lower than in conventional battery cages. The higher incidence of bacterial diseases in laying hens housed in non-cage systems as described in several studies [Reference Fossum20, Reference Kaufmann-Bart and Hoop21] may have resulted in a more frequent use of antimicrobials. If this were the case, it raises the question whether, besides the advantages at the animal-welfare level, there will be any adverse consequences for public health on the level of spread and persistence of antimicrobial resistance in laying hens in the future. Second, in non-cage systems the chance of oro-faecal transmission of bacteria is much higher than in conventional battery cages, both between hens and between the animals and the environment. This could also be of importance since, apart from antimicrobial usage, other factors such as localization and size of the microbial population [Reference van den Bogaard and Stobberingh23], and immunity and contact intensity of the host [Reference Walson24] play a role in antimicrobial resistance development. Finally, the fact that many of the sampled farms with non-cage production systems made the change to the new production systems only a few years before the onset of the study and that these new production systems are often located in the same house where previously the battery cages were present, might also explain the observed results. It has been described that the decrease in antimicrobial resistance following changes in the production system or in antimicrobial usage policy in food-producing animals is very slow and that resistance against certain antimicrobials can still be detected long after direct selection pressure by antimicrobial usage has ended [Reference Dunlop25, Reference De Gelder26].
The difference in effect of the housing system on MDR in E. coli and E. faecalis might be the result of different biological characteristics of both bacterial species. E. coli is typically an inhabitant of the intestinal tract and is to a considerable extent present in the hens' faeces. The animals have frequent oro-faecal contact in non-cage indoor production systems, resulting in an intense exchange of E. coli between hens. This high-contact intensity could cause the higher levels of MDR in laying hens in raised-floor systems. Since enterococci are widely distributed in the soil and the environment [Reference Aarestrup, Butaye, Witte, Gilmore, Clewell, Courvalin, Dunny, Murray and Rice6], access to a pasture may exert some kind of diluting effect on the intestinal enterococcal population, leading to lower levels of MDR in free-range laying hens. However, although these differences between the housing types are statistically significant, it is not yet clear whether they have some biological relevance and therefore further study is necessary to confirm and clarify these findings.
Although differences in methodology of sampling and analysis between different studies have to be taken into account, the results of our study illustrate that, in general, the levels of antimicrobial resistance in indicator bacteria in laying hens are relatively low compared to broilers, pigs and – to a lesser extent – cattle [Reference de Jong12, Reference Bywater27, Reference Persoons28]. This is in accord with previous studies in laying hens [Reference Schwaiger, Schmied and Bauer17, Reference Schwaiger, Schmied and Bauer18, Reference Kojima29]. The overall limited antimicrobial usage in egg-producing laying hens, as shown in the results, could play a role in this observation since it is generally accepted that the use of antimicrobials is one of the major risk factors for the development and spread of antimicrobial resistance [Reference Wegener30, Reference van den Bogaard31]. A reason for the lower levels of resistance seen in the layers compared to broilers may be that the layers in this study were sampled on average at age 74 weeks, compared to broilers that are usually aged 6 weeks at time of slaughter. It has been described for several animal species that antimicrobial resistance levels decrease with increasing age [Reference Langlois32, Reference Butaye33]. From this point of view, it would be very interesting to perform longitudinal studies to observe the interaction between antimicrobial resistance and the age of the hens and to monitor the use of antimicrobials during the rearing of the pullets.
The fact that the samples were analysed in different laboratories in different countries may have slightly influenced the results, despite the equivalent methodology that was used in all four participating countries. However, the observed differences in MDR levels between countries, probably also result from regional differences in animal husbandry and antimicrobial usage and the fact that the distribution of the housing system of the sampled farms was not the same in each country. A marked finding in this respect is the ceftiofur resistance in E. coli in Belgium. Whereas in Germany, Italy and Switzerland only very low levels of ceftiofur resistance were found, in Belgium this resistance was 12·1% in E. coli. Consequentially the odds to have resistance against ceftiofur were higher in Belgium compared to the other countries, although only the difference with Switzerland was significant. This study result coincides with recent findings of high levels of ceftiofur resistance in broilers in Belgium [Reference Persoons28]. It has recently been stated by several authors that one of the reasons for the increasing levels of ceftiofur resistance may be the worldwide and systematic use of ceftiofur in breeding eggs and 1-day-old chicks in the hatcheries [34, Reference Webster35]. In Belgium the use of ceftiofur in poultry has not been licensed for a decade [Reference Bertrand36] but might be continued off-label [Reference Persoons28].
Another hypothesis for the increased ceftiofur resistance might be the production on the same farm of other animal species, in which the use of ceftiofur is still permitted. This could result in horizontal transmission of resistance genes between bacterial populations of different animal species [Reference Kruse and Sorum37, Reference Lorenz and Wackernaegel38]. However, the number of mixed-production farms in Belgium did not significantly differ from the situation in Germany and was even less than in Switzerland. Possibly the high density of farms in the northern part of Belgium, where 90% of all animal production is situated, may enhance contact between the bacterial populations of different ecological systems, for example surface water, leading to an efficient horizontal spread of antimicrobial resistance. For humans, it has been demonstrated that a higher population density enhances the development and spread of antimicrobial resistance [Reference Garau39, Reference Bruinsma40]. Further eco-epidemiological studies are needed to elucidate this possibility.
CONCLUSION
The results of this study suggest that the levels of antimicrobial resistance in indicator bacteria such as E. coli and E. faecalis in laying-hen flocks are relatively low. The differences observed between both indicator bacteria with respect to the potential association between the housing system and MDR suggest that it is important to not focus on a sole bacterial species when attempting to assess risk factors for antimicrobial resistance. It is crucial to conscientiously monitor the prevalence and evolution in time of antimicrobial resistance in laying hens, both during the rearing period and the production cycle, in order to be able to detect early changes in antimicrobial resistance and to minimize the spread of resistant bacteria to humans. Therefore it is recommended that detailed epidemiological studies in the field and under experimental conditions on a broader spectrum of indicator bacteria are performed.
ACKNOWLEDGEMENTS
This research was funded by the EU FP6, under the contract 035547 (Safehouse project). The authors thank all farmers for permission to sample their farm and all laboratory technicians at the different institutions for their skilful help during the bacteriological analyses.
DECLARATION OF INTEREST
None.