Introduction
Breast-conserving surgery in conjunction with radiotherapy is the standard of care for treating early-stage breast cancer patients.Reference Fisher, Anderson and Bryant1–Reference Van Dongen, Voogd and Fentiman3 Traditionally, conventional breast irradiation consisted of a beam arrangement using two opposing tangential beams, allowing adequate coverage of the breast tissue while minimising the dose to critical structures (e.g., ipsilateral lung, contralateral breast and heart). Physical or dynamic wedges, field-in-field (FiF) or direct fields are frequently added to modify these tangential beams to develop the most homogenous plan to compensate for the significant contour changes.Reference Al-Rahbi, Al Mandhari and Ravichandran4 However, this requires a manual trial and error method and may be very time consuming.Reference Fisher, Anderson and Bryant1, Reference Trombetta, Julian and Valakh5
Intensity-modulated radiation therapy (IMRT) has emerged as a leading technique in the treatment of many cancer sites.Reference Zelefsky, Fuks and Happersett6, Reference Longobardi, De Martin and Fiorino7 Recent randomised trialsReference Pignol, Olivotto and Rakovitch8, Reference Donovan, Bleakley and Denholm9 and other studiesReference Harsolia, Kestin and Grillis10, Reference Freedman, Anderson and Li11 have reported that IMRT for breast cancer can improve the dose homogeneity and decrease acute skin toxicity and dose to the contralateral breast when compared with the traditional three-dimensional (3D) tangential method. Unfortunately, IMRT can increase the volume of low dose received to the organs at risk (OARs) and healthy tissue.Reference Hall and Wuu12–Reference Abeyaratne15
A more recent alternative to 3D conventional or IMRT treatment is a hybrid approach, combining the two techniques with the aim to create a more optimised dosimetric outcome. This technique involves a certain percentage of the prescription dose (e.g., 70%) delivered via tangential beams with no beam modifiers. The remaining dose (e.g., 30% of prescription dose) is delivered through a number of IMRT fields that may be tangential with or without the addition of beams directed to the surgical cavity. The IMRT plan is optimised in order to achieve increased homogeneity and superior target volume coverage than is achievable with tangent fields alone. Several studies have investigated the effectiveness of this technique compared with the 3D conformal method. Ahunbay et al.Reference Ahunbay, Chen and Thatcher16 retrospectively analysed 15 patients comparing the hybrid technique with the 3D conformal and IMRT techniques and found the hybrid technique to be equal to, if not better than the other approaches with a higher uniformity index across the target area, a lower lung dose, reduced monitor units compared with IMRT and reduced planning time by ~75% compared with 3D conformal. Descovich et al.Reference Descovich, Fowble, Bevan, Schechter, Park and Xia17 performed a similar study and discovered that the hybrid technique reduced the volume of the breast receiving hot spots of >105% total dose, provided more homogenous dose coverage of the tumour cavity, was less dependent on planner experience and required less planning time than the other two techniques. Many other studies have been performed investigating other variations of the hybrid technique and proved that it is comparable, if not superior, with 3D conformal or IMRT planning alone.Reference Mayo, Urie and Fitzgerald18–Reference Peulen, Hanbeukers and Boersma20 There is, however, limited literature on the use of the optimal ratio of static tangent field dose compared with IMRT field dose for hybrid techniques.
This study aims to compare the conventional simultaneous integrated boost (SIB) planning technique with a hybrid SIB IMRT technique with varying open tangent to IMRT field dose ratios. The quality of the plans will be assessed by quantifying the target volume coverage, dose homogeneity and conformity and dose to predetermined OARs. Furthermore, we wish to investigate which of the dose ratios proves the most favourable as a class solution across a sample.
Methods
Study design
This study was conducted retrospectively with ten patients with left-sided breast cancer and five patients with right-sided breast cancer requiring treatment of the whole breast and deeply located tumour cavity with no nodal involvement. All patients had completed treatment with conventional SIB treatment in the supine position, conforming to the standard department protocol. More left-sided patients were included in order to increase the statistical power for the cardiac structure indices. Three hybrid plans were created retrospectively on the same dataset using three varying dose ratios (open field:IMRT). For each plan, all patients received 50·4 Gy to the whole breast with an additional 14 Gy to the tumour cavity over 28 fractions using 6 MV only. This study only evaluated this one fractionation schedule that is used for selected high-risk patients.Reference Hurkmans, Meijer and van Vliet-Vroegindeweij21 The plans were compared and assessed using dose delivered to OARs and dose conformity.
Volume delineation
Dose structures/OARs
For each of the datasets, the ipsilateral lung, both lungs as a single structure, heart and left anterior descending artery (LAD) (left-sided cases) had been contoured by a radiation oncologist (RO) for planning using the conventional tangent technique. For the purposes of this study, the contralateral breast was also contoured. A structure was created consisting of the non-irradiated volume and labelled as ‘Dose Ring’, that is, volume of the body not including the planning target volume (PTV). Interobserver variation was limited by having one planner checking and approving all structures.
Target volumes
The clinical target volume boost (CTVboost) was volumed by the RO, using the usual clinical parameters such as surgical clips, ultrasound, mammograms and/or and histopathological reports. The PTV boost (PTVboost) was created by expanding the CTVboost by 0·5 mm in all directions but clipped to ensure it was 0·5 mm from the skin anteriorly and abutted the pectoralis major muscle posteriorly.
A breast PTV was created by the RO, using clinical and computed tomography (CT) imaging parameters. This volume was determined using a combination of placing radioopaque wire around the palpable breast tissue and CT contouring.Reference Offersen, Boersma and Kirkove22 A reduced PTV structure, PTV evaluation (PTVeval), was created from the PTV for the purpose of clinical evaluation and IMRT optimisation. This PTVeval volume was created by reducing the PTV by 2 cm superiorly and inferiorly and 0·8 cm anteriorly and posteriorly, with the further deletion of all lung, heart, bowel, liver, ribs and intercostal muscle from the volume (Figure 1). This better represented a volume of breast tissue void of OARs, whereas the reduction anteriorly avoided a region of high dose at the skin edge. The PTVeval also excluded the PTVboost by use of Boolean operators to allow IMRT optimisation and appropriate dose–volume histogram (DVH) evaluation.
Figure 1 Representation in transverse (a) and sagittal (b) of planning target volume boost (PTVboost) and PTV evaluation (PTVeval) as a reduction of the PTV to represent a target volume void of organs at risk.
Treatment planning
Each plan was created and assessed using Eclipse software (version 11, Varian Medical Systems).
Conventional SIB breast treatment
Before this study, conventional tangential plans were the departmental standard treatment for early breast cancer, with opposing tangential fields to cover the PTV. A SIB photon boost technique was used for deeply positioned boost volumes where a sequential electron boost was not suitable. For this technique, individual plans were developed employing physical wedges (15, 30, 45 and 60°) and varied beam weighting to achieve a homogeneous plan. The planning protocol requires fields to have multileaf collimation (MLCs) on the posterior aspect to limit lung dose and 2 cm overshoot anteriorly to account for respiratory motion. FiF were used as required, to further ensure dose homogeneity and target coverage. Finally, two photon beams with a 0·7 cm MLC margin around the PTVboost (positioned at the planner’s discretion using visual and statistical dose assessment) were used to achieve total dose (TD) to the volume. Additional dynamic wedging was also used to ensure target coverage when required.
Both plans shared the same isocentre, allowing for simultaneous treatment of all volumes. Two normalisation points were also required, with one at the central slice of the PTV and another in the centre of the PTVboost.
Hybrid technique
The hybrid technique consisted of a two-step process resulting in a six-field plan in all instances. The first plan used two tangential fields with MLCs conforming to the PTV along the posterior edge and including 2 cm of overshoot anteriorly, reflecting a conventional tangent plan. A portion of the total breast dose, that is, 60, 70 or 80%, was calculated to a reference point as per ICRU 50 guidelines, and weightings were used to provide an even distribution of dose across the whole breast. No additional fields or beam modifiers were used in this plan.
A second IMRT plan was created at the same isocentre as the conventional plan and comprised of four fields; two tangential fields at the same gantry angle as the original two tangents and two fields angled to best access the boost cavity. The two non-tangential fields were dedicated to treat the boost cavity by locking the jaws around the PTVboost during the optimisation process. The IMRT plan was optimised using a standard optimisation template employing sliding window dynamic IMRT with Eclipse optimisation software to achieve dose constraints and utilise avoidance volumes to reduce undesirable excess dose. A basic level of planning experience was simulated by limiting optimisation calculations and use of avoidance structures to two. The plan was dosed with the remaining, that is, 40, 30 or 20%, of the total breast dose and 14 Gy additionally for the boost cavity.
The sum of the two plans was assessed against departmental protocol unto which all plans were compared, using visual assessment, maximum and minimum doses and DVH for target volumes and critical structures. This process was repeated using a total of three different dose ratios between the two plans (Table 1) in order to identify possible clinical benefits.
Table 1 Dose ratios for each hybrid arm
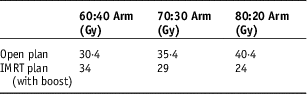
Abbreviation: IMRT, intensity-modulated radiation therapy.
Plan analysis
Each plan was evaluated with plans considered acceptable when a minimum of 90%, of 50·4 Gy, covered the PTVeval and 95%, of 64·4 Gy, covered the PTVboost. The maximum point dose was kept below 110% of the prescribed dose to each of the PTVs. Although all plans were deemed clinically acceptable by the RO, some conventional plans did not achieve 90% coverage of the PTVeval. Therefore, in order to accurately compare the dose with OARs and target coverage all plans were normalised to achieve a minimum 90% coverage to the PTVeval.
The dosimetric data used for plan comparison were the mean, maximum and V20 dose for the ipsilateral lung and total lungs. The heart mean, maximum, V30, V25 and V5 dose were also measured for left-sided patients as well as the LAD mean, maximum and V30 dose. In addition to this, the mean, maximum, V1, V2 and V5 doses were collected for the contralateral breast.
The conformity index (CI) for the PTVboost was calculated using absolute volumes quantified by the treatment planning system. In this study, the CI is defined as the ratio of the intersection and the union of the PTVboost and the 95% dose structure, where an outcome of 1 represents identical structures and 0 represents no overlap at allReference Jameson, Holloway, Vial, Vinod and Metcalfe23 (Figure 2). As the CI cannot give any information regarding plan heterogeneityReference Feuvret, Noel, Mazeron and Bey24 other evaluation tools were also used, namely assessment of the dose to OARs and the heterogeneity index (HI).
Figure 2 Equation for the conformity index (CI) used in the study. Contour 1 represents the planning target volume boost and Contour 2 represents the 95% isodose structure (61·18 Gy).
The HI indicates the ratio between the maximum and minimum dose within the volume, calculated using the formula (Dmax−Dmin)/Dmean.Reference Kataria, Sharma, Subramani, Karrthick and Bisht25 In this scenario, a lower value indicates more homogeneity and a higher represents undesirable inhomogeneity. In this study, the HI is applied only to the PTVboost volume due to the higher dose received at the interface of target volumes.
Average difference in dose–volume parameters between the cohorts were tested with a Student’s t-test. Due to multiple pairwise comparisons, a Holm-Bonferroni correction was applied with the level statistical significance set at p<0·02.
Results
All plans created, both conventional and in the hybrid arm were deemed qualitatively acceptable according to department criteria/protocol and clinical assessment.
Target
The dose to the PTVeval was considerably lower across all three hybrid arms compared with the conventional plan. The point maximum decreased by an average of at least 2 Gy using the hybrid technique, whereas the mean dose was brought down from 54·0 GY to, 51·8 Gy, 51·9 Gy and 51·6 Gy in the 60:40, 70:30 and the 80:20 hybrid plan, respectively. These plans were cooler than the conventional arm while delivering a mean dose more aligned with the ICRU 83 guidelines prescribed dose reporting, that is, 50·4 Gy.26 The volume of PTVeval receiving 110% of prescribed dose (i.e., 55·44 Gy) reduced considerably in the hybrid arms from 31·3 to 9·3, 10 and 9·9% for the 60:40, 70:30 and 80:20 arms, respectively.
Dose to the PTVboost volumes remained similar between techniques (Table 2). It was notable that the mean doses for the PTVboost were ideal for all hybrid plans at 64·3 Gy (60:40), 64·5 Gy (70:30) and 64·3 Gy (80:20) for a prescribed dose of 64·4 Gy when compared with 63·9 Gy for the conventional technique. Although the minimum dose to the PTVboost increased across all hybrid arms and average maximums remained alike these results did not show statistical significance compared with the conventional arm.
Table 2 The comparable target dosimetric parameters for conventional treatment and hybrid arms
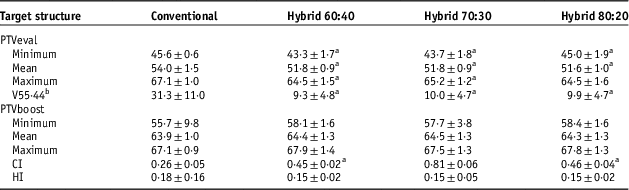
Notes: Values are mean±1 SD.
a Student’s t-tests (p<0·02), conventional versus hybrid.
b V55·44: 110% of prescribed dose to the PTVeval.
Abbreviations: PTVeval, planning target volume evaluation; PTVboost, planning target volume boost; CI, conformity index; HI, heterogeneity index.
Conformity, to the PTVboost, for all hybrid arms was considered statistically significant with the 70:30 group proving most conformal with an average 0·81 CI as opposed to 0·26 for the conventional technique (Table 2). The homogeneity index was lower in all hybrid arms at 0·15 compared with 0·18 in the conventional arm. However, this result was not statistically significant. Conformity and homogeneity index were not evaluable for PTVeval due to shape irregularity caused by the removal of the PTVboost structure and therefore did not conform to an isodose line. Coverage of the PTVeval was instead analysed using the DVH and the plans were qualitatively assessed for conformity. Qualitative assessment of all patients also favoured the hybrid arms in terms of conformity to the PTVeval (Figure 3).
Figure 3 (a) Series represents dose contours from a conventional plan; (b) series represent dose from a hybrid plan with ratio 70:30.
OARs
Dose to both lungs was reduced in all hybrid plans compared with the conventional plan in all measures (Table 3). The hybrid 60:40 and 70:30 significantly improved the V20 lung dose measure. The dose to the ipsilateral lung was reduced in all measures with the use of the hybrid plans except for an increase of 0·3% for the hybrid 60:40 V5.
Table 3 The comparable organ at risk (OAR) dosimetric parameters for conventional treatment and hybrid arms
Notes: Values are mean±1SD.
a Student’s t-tests (p<0·02), conventional versus hybrid.
Cardiac doses displayed in the table represent the ten left-sided patients only to obtain more significant and categorical data. The LAD received lower mean and maximum doses for all hybrid techniques, which was statistically significant compared with the conventional plans. The hybrid 60:40 showed the largest reduction in the maximum LAD dose with an average of 9·3 Gy. The V30 for the LAD was also reduced across all hybrid arms though not statistically significant (Table 3).
Although the hybrid plans showed a significant reduction in dose for the V30 and V25 heart indices, there was a marked increase in the V5 and mean heart doses, though these were not statistically significant. The hybrid plans reduced the maximum dose to the heart although in the 80:20 hybrid arm this was not statistically significant.
For the contralateral breast, the mean dose, V5 and V1 were reduced in all the hybrid arms but did not show statistical significance. The average increase in the maximum dose of 0·79 Gy observed in the hybrid 70:30 was statistically significant and there was an increase in the V2 measure in all hybrid arms (Table 3).
The non-irradiated volume (Dose Ring) received a significantly lower dose with a reduction of at least 3 Gy in maximum dose for all hybrid plans.
Discussion
Our aim was to directly compare a conventional SIB breast treatment technique to a developed hybrid technique based on dose to OARs, target coverage and dose homogeneity. Results have successfully shown that all the hybrid arms are clinically and statistically comparable as a class solution to the conventional arm in this sample of 15 patients.
The hybrid arm results concerning ipsilateral lung dose parameters were on average comparable, if not lower, than the conventional arm with doses for all plans well within recommended QUANTEC standards.Reference Marks, Bentzen and Deasy27 Much of the available hybrid literature examines dose to the lungs based on treating the whole breast to a single dose with no boost volume.Reference Descovich, Fowble, Bevan, Schechter, Park and Xia17–Reference Farace, Zucca and Solla19, Reference Xie, Ouyang and Wang28, Reference Shiau, Hsieh and Tien29 Due to the beam angles chosen for this hybrid technique and escalated PTVboost dose, it is difficult to compare these results with other studies. Ipsilateral lung dose in the conventional plans is also affected by the choice of boost-targeting beam angles, which may increase dose to the lung to achieve TD to a deeply located PTVboost. This is likely due to the conventional plan lacking the ability to limit dose to structures that is provided by the IMRT portion of the hybrid plan. Dramatic reduction of high doses to the lung evident in the hybrid plans can be attributed to the inclusion of IMRT, though conversely the IMRT also creates additional scatter thereby increasing low-dose regions as reported by other studies.Reference Al-Rahbi, Al Mandhari and Ravichandran4, Reference Zhang and Zheng13, Reference Mansouri, Naim, Glaria and Marsiglia14 Furthermore, beam angles chosen in the hybrid plans to treat the boost contribute to a higher V5. Further investigation into this hybrid technique to reduce V5 doses could involve selection of angles that reduce exit to the ipsilateral lung. Within the hybrid arm, all ratios reduced dose to all lung parameters compared with the conventional plans, apart from the V5 ipsilateral measure of which the 60:40 plan showed the only increase in dose.
The recommended dose limitations to cardiac organs are debatable as the dose–response relationship is difficult to assess.Reference Gagliardi, Constine and Moiseenko30 Nevertheless, several studies have produced evidence that the risk of major coronary event increases by 7·4%/every additional increase of 1 GyReference Darby, Ewertz and McGale31 to the mean dose to the heartReference Henson, McGale, Taylor and Darby32–Reference Darby, McGale, Taylor and Peto34 and LAD.Reference Lind, Pagnanelli and Marks35, Reference Evans, Prosnitz and Yu36
Heart and LAD doses showed significant decrease in maximum and mean measures across all hybrid arms compared with the 3D conformal radiotherapy (3DCRT) arm. However, conversely all hybrid arms showed an increase in V5 measures of the heart compared with 3DCRT. The ability of IMRT to use the heart as a dose-limiting structure coincides with reduced maximum and mean doses. The increase in lower dose regions compared with 3DCRT technique is consistent with other IMRTReference Al-Rahbi, Al Mandhari and Ravichandran4, Reference Zhang and Zheng13, Reference Abeyaratne15, Reference Guerrero, Li, Earl, Safraraz and Kiggundu37 and hybrid breast studies.Reference Farace, Zucca and Solla19, Reference Xie, Ouyang and Wang28 The increase in V5 may again be partially contributed to by the selection of boost field angles exiting through the heart. Other than optimisation of beams and shielding, the utilisation of breath-hold techniques is another technique to reduce the dose to the heart in the tangential beam path.Reference Korreman, Pedersen, Aarup, Nottrup, Specht and Nystrom38–Reference Swanson, Grills and Ye40
The use of physical wedges used on the lateral tangent in conventional planning, is associated with a three-fold increase in scattered dose compared with virtual wedges or hybrid IMRT.Reference Williams, Moran and Hsu41, Reference Woo, Pignol and Rakovitch42 This is observed in the contralateral breast parameters with the mean, V5 and V1 doses showing a decrease in the hybrid arms. The increase in V2 in the hybrid arms in the contralateral breast can be attributed to the absence of IMRT objectives to control for these lower doses. Furthermore, the lateral beam angle selected to treat the PTVboost in the hybrid arms could be optimised to avoid exit dose through the contralateral breast. If these considerations were applied, the dose to the contralateral breast could be further reduced.
The PTVeval minimum coverage was considered acceptable with lower doses recorded for all parameters in hybrid arms compared with conventional, though was still within acceptable limits. All PTVeval mean (D50) doses were reported higher than the prescribed dose in the hybrid arms by 1·4 Gy, 1·4 Gy and 1·2 Gy (60:40, 70:30 and 80:20, respectively) compared with 3·6 Gy higher for the 3DCRT technique. Higher doses are considered acceptable due to the proximity of the boost region to the PTVeval as the dose gradient increases rapidly at the interface of the two volumes. Volumes of D110% and higher across target volumes are undesirable, having been shown to increase skin toxicityReference Freedman, Anderson and Li11, Reference Fernando, Ford and Powles43–Reference Pignol, Olivotto and Rakovitvh46 and cause poor cosmetic outcomes.Reference Johansson, Svensson and Denekamp45, Reference Back, Guerrieri, Wratten and Steigler47 The hybrid arms showed a reduction (21·4%) in volume over the conventional arm (Table 2), whereas there were no significant changes in the volume of D110% of prescription dose between the hybrid arms.
Maximum PTVboost doses were similar across both techniques but results showed far greater dose conformity to the volume in the hybrid arms over the conventional. Among the hybrid arms, the 70:30 arm showed a statistically significant CI of 0·81 compared with 0·45, 0·46 and 0·26 for the 60:40, 70:30 and 80:20 and conventional arms, respectively. As evident in other comparative studies,Reference Zhang and Zheng13, Reference Ahunbay, Chen and Thatcher16, Reference Peulen, Hanbeukers and Boersma20, Reference Mihai, Rakovitch and Sixel48–Reference Singla, King, Albuquerque, Creech and Dogan50 IMRT shows greater dose conformity to target structures with our results further reflected in comparative hybrid studies.Reference Descovich, Fowble, Bevan, Schechter, Park and Xia17, Reference Mayo, Urie and Fitzgerald18, Reference Xie, Ouyang and Wang28, Reference Small, Kelly, Beldham-Collins and Gebski51 When considering the dose homogeneity across the PTVboost, the HI indicates that the hybrid technique creates a more homogenous dose at 0·15 for all arms compared with 0·18 with the conventional plan. Although decreasing dose to OARs is important for reducing toxicity, increasing dose conformity and ensuring homogeneity across target volumes is fundamental in achieving the prescribed dose and increases the probability of reducing local recurrence.Reference Bartelink, Maingon and Poortmans52–Reference Freedman, Anderson and Bleicher54 All the hybrid arms exhibit superiority over the conventional results when considering CI and HI, though the 70:30 ratio proves the optimal ratio, as a class solution, between the hybrid results.
Limitations
There is a consensus among the investigators that it was significantly quicker to achieve acceptable plans using the hybrid technique and is supported by other studies.Reference Mayo, Urie and Fitzgerald18, Reference Farace, Zucca and Solla19 It is assumed that treatment times would also be reduced, as staff would not be required to enter the room to add or change accessories.Reference Small, Kelly, Beldham-Collins and Gebski51 However, duration of individual plan development and treatment was not timed for conventional or hybrid plans. Finally, no further contouring or input would be required by the RO although additional quality assurance is required by medical physics initially when introducing any new technique.
We chose to use two SIB boost fields in this study for both conventional and hybrid plans. We noticed that in some cases these boost fields caused an increase in low dose to the heart, and lung. Future analysis of hybrid plans should include a comparison between one and two SIB boost fields with statistical analysis of dose conformity and V2 lung dose. Lung V2 was not considered for this study, however, this would create a greater depth of information. Furthermore, the use of one boost field rather than two, could allow comparative treatment for patients with superficial cavity sites, requiring electron treatment. The use of hybrid SIB treatment for these patients would reduce the total number of treatments from 33 to 28 and allow increased patient throughput for the department for this high-risk patient group. In our department, the majority of patients are now treated with 70:30 ratio using only one boost field that gives excellent conformity and reduces scatter to OARs. To date, there have been no instances where the 70:30 hybrid ratio has not satisfied tolerances and targets and required use of a different ratio, however, superficially located tumours may still be treated with subsequent electron boosts. In this study, a class solution was identified regardless of patient factors such as separation, PTVboost location, size of PTVboost, etc.
Increasing patient numbers would facilitate an increase in the statistical power of this study, however, 15 patients with four plans per patient, was considered statistically acceptable providing significant results for a number of the dose parameters considered.
Conclusion
There is limited literature on the use of hybrid techniques to treat SIB, especially in comparison with hybrid or IMRT whole breast treatment (no boost). There are fewer publications still on the optimal ratio of static tangent field dose compared with IMRT field dose. This study shows significant improvement in most OAR parameters and increased dose conformity in all three hybrid arms. It is noted that the V5 for the heart and ipsilateral lung should be further investigated with the hybrid technique to further improve doses and the practitioner’s clinical judgement should be used in all cases to prioritise risks of low-dose regions to OARs verses high-dose regions within the PTV. Overall, all hybrid plans exhibited greater benefits to the patient than the conventional plan. From this research, the optimal hybrid ratio as a class solution is the 70:30 due to increased conformity and homogeneity as other comparable parameters are not clinically significant between the three hybrid arms.
Acknowledgements
The authors would like to acknowledge May Whitaker and the Chris O’Brien Lifehouse.
Financial support
This research received no specific grant from any funding agency, commercial or not-for-profit sectors.
Conflicts of Interest
None.
Ethical Standards
This study is a retrospective analysis on radiotherapy plans which were not treated on any human or animal. This study meets all requirements as outlined in the National Statement on Ethical Conduct in Human Research. This compliance has been approved by the Human Research Ethics Review Committee (RPAH Zone), who have been accredited by the NSW Department of Health as a lead Human Research Ethics Committee under the model for single ethical and scientific review and is constituted and operates in accordance with the National Health and Medical Research Council’s National Statement on Ethical Conduct in Human Research and the CPMP/ICH Note for Guidance on Good Clinical Practice. The reference protocol for this study is: ‘Protocol No X14-0203 & LNR/14/RPAH/266—‘Planning comparison of hybrid intensity-modulated radiation therapy (IMRT) simultaneous integrated boost (SIB) technique to 3D conformal radiotherapy tangents with SIB for breast radiotherapy at Lifehouse’.