Introduction
The increasing burden of public health crises resulting from epidemics and humanitarian emergencies continue to pose challenges, particularly in countries with weak health systems [Reference Warsame, Blanchet and Checchi1, 2]. Of particular concern are infectious disease outbreaks, which continue to threaten regional and international public health security [Reference Lee3, Reference McCloskey4]. These outbreaks are driven by mass population movements, extensive transport networks, climate change and other ecological and environmental factors that facilitate the emergence and global spread of infectious pathogens [Reference Morganstein, Ursano, Fullerton, Weisaeth and Raphael5, Reference McMichael6]. In the African region, where more than 100 infectious disease outbreaks are detected and responded to annually [7], the risk is even higher due to the limited capacities of most countries to detect and respond to these events [Reference Talisuna8]. Limited efficacy and availability of proven public health preventive interventions as a result of the challenges faced by disease control programmes particularly in sub-Saharan Africa add to this risk [Reference Songane9]. Outbreaks of severe acute respiratory syndrome (SARS), Ebola virus disease (EVD) in West Africa and the Democratic Republic of Congo and the ongoing coronavirus disease 2019 (COVID-19) pandemic have shown that these events not only cost lives but also disrupt trade and economic activities, costing millions or billions of dollars [Reference Joo10, Reference Elmahdawy11]. This underscores the urgent need for tools and processes that are used to facilitate early detection and response to these public health events to mitigate their impact.
In addition to indicator-based surveillance, the International Health Regulations (IHR) 2005, emphasise the need for event-based surveillance to be used as part of holistic epidemic intelligence activities [Reference Merianos and Peiris12, Reference Fischer, Kornblet and Katz13]. Epidemic intelligence refers to the collection, processing and analysis of information on potential health-related hazards with the goal of early detection of outbreaks and health-related emergencies [Reference Paquet14]. The two main components of epidemic intelligence are indicator-based surveillance, which relies on information from structured or official sources such as health facility reports or other pre-identified reporting sites, and event-based surveillance, which includes information obtained from unstructured sources such as the media [15, 16]. The latter is responsible for most initial reports on epidemics [Reference Heymann and Rodier17].
In furtherance of compliance with IHR 2005, several guidance documents, frameworks and tools have informed the implementation and scale-up of epidemic intelligence activities in the African region. These include the WHO Emergency Response Framework (ERF) [18], Integrated Disease Surveillance and Response (IDSR) strategy [19], the Global Health Security Agenda (GHSA) [Reference Wolicki20] and the Early Warning and Response Network (EWARN) [Reference Cordes21]. Additionally, the internet or social media has rapidly enhanced epidemic intelligence monitoring of public health events, providing an exceptional medium for early detection of outbreaks [Reference Yan, Chughtai and Macintyre22]. Three internet-based platforms that have been used for epidemic intelligence in the African region include Early Alerting and Reporting (EAR), the Hazard Detection and Risk Assessment System (HDRAS) and more recently the Epidemic Intelligence from Open Sources (EIOS). The EAR detects and monitors information about chemical, biological, radiological and nuclear threats (CBRN) as well as pandemic influenza [Reference Barboza23], and the HDRAS is a platform for detection and monitoring of acute public health events [Reference Bernard24]. EAR has been found to be a feasible model for detecting intentional release of biological agents while HYDRAS has been deployed to detect outbreak in mass gathering events [Reference Riccardo25][, Reference Enderlein and Regmi26]. The EIOS encapsulates the experiences of EAR and HDRAS and serves as a medium to ‘identify, verify, assess, analyze, interpret and communicate relevant information on public health events for appropriate, timely and effective action’ [27].
The aim of this study is to review the epidemic intelligence processes adopted by WHO Regional Office for Africa (AFRO) to support the 47 Member States in the region, its ability to detect, report and provide evidence-based assessments for early outbreak response, as well as lessons learned. We specifically analysed the ability of the epidemic intelligence activities to detect true outbreaks, the frequency of outbreak detection and the results of evidence generated from outbreak risk assessments. We emphasise the need for timely and reliable event detection activities and describe how lessons learned can inform and guide future use and scale-up of support to member states in the African region to strengthen preparedness for, early detection of and rapid response to outbreaks that may constitute a public health event of international concern (PHEIC).
Methods
We performed a retrospective analysis of epidemic intelligence activities undertaken at AFRO over a four-year period – 2017−2020 – focusing on the processes, key outputs and contribution to the early detection and response to infectious disease outbreaks across the WHO African region. The WHO African region is composed of 47 member states, with all but Algeria located in sub-Saharan Africa [28].
Epidemic intelligence at AFRO
Epidemic intelligence activities were implemented in the context of the IDSR strategy widely adopted in the African region for early detection of outbreaks [Reference Kasolo29], consisting of indicator-based surveillance (IBS), event-based surveillance (EBS) and rapid risk assessments (RRA). AFRO relied on formal notification of outbreaks by member states using indicator-based surveillance while the organisation implemented event-based surveillance through monitoring of the media from internet-based sources and rumours (Fig. 1). A standard operating procedure (SOP) on detection, verification and risk assessment of acute public health events in the African region was adopted to guide a dedicated team of epidemiologists in the daily implementation of epidemic intelligence activities [30].

Fig. 1. Steps in the epidemic intelligence process at the WHO Regional Office for Africa adapted from WHO AFRO manual on detection, verification, and risk assessment of acute public health event in the WHO African region.
The IDSR strategy identifies a list of priority diseases with defined thresholds for determining alert and epidemic levels to trigger public health response. The implementation of indicator-based surveillance relied on analysis and interpretation of routine surveillance data obtained from formal reporting sites (mostly health facilities) in the respective member states. These data were shared weekly with AFRO for collation, analysis and monitoring of epidemic thresholds of priority diseases across the region. The outbreaks detected through indicator-based surveillance were officially notified to WHO by member states using the IHR (2005) Annex 2 decision instrument [31].
Event-based surveillance was performed mainly through media monitoring using internet-based epidemic intelligence platforms. The two platforms used during the studied period were HDRAS and EIOS, with the latter succeeding the former since June 2018. These platforms pooled epidemic intelligence information from several expert systems, including Medisys [32], Global Public Health Intelligence Network (GPHIN) [33], HealthMap [34] and ProMED [35], among others. They used automated methods for scraping, filtering and processing news from media institutions and other relevant online sources, which were categorised based on key terms such as outbreaks, epidemics, disease name, cluster of symptoms or deaths, disaster, etc. On the platforms, customised boards with sources from 42 languages filtered articles only of concern to member states in the African region.
A designated member of the epidemic intelligence team triaged media articles populating these platforms daily to detect any signal concerning an acute public health event. A signal was considered as any information or rumour relating to an event not yet officially reported to WHO that had caused or posed a potential risk to human health. The triage process involved filtering, screening and selecting signals for monitoring or further verification. During filtering, the designated epidemiologist identified and screened out any duplicate information analysed that was not automatically de-duplicated by the epidemic intelligence platforms, and discarded any information not relevant for the purpose of early warning. The potential signals were further screened by gathering additional information from relevant sources to ensure that they differed from known events in terms of disease manifestation, location, number of cases, suspected causal agent and the circumstances under which they occurred. The final step of triage involved selection of signals from a screened list of potential signals presented by the designated epidemiologist. This was determined through discussion with a team of epidemiologists who used baseline epidemiological data, reliability of the source, indications of severity, risk of international spread or the potential to cause large-scale outbreak as the basis for selection. The selected signals were then shared with the respective member states via WHO Country Offices for verification or continued to be monitored for any change in the situation. A confirmed signal was a signal found to be true after investigation or verification by the member state and WHO, while a signal was considered discarded if not verified during the investigation. Confirmed signals that met the requirements of the IHR 2005 Annex 2A decision-making instrument for notification to WHO were deemed as new public health events. Other signals concerning the spread of an already notified outbreak to new geographic areas were monitored, used to gauge the need for a rapid risk assessment and inform public health response actions.
Risk assessment of acute public health events
The WHO guide on rapid assessment of acute public health events [36] was used by AFRO to conduct rapid risk assessments (RRA). These were conducted for ‘large-scale events potentially exceeding the response capacity of the affected country, for events with international implications, and those requiring a WHO response’ [18]. They assessed the likelihood and consequences associated with three main risk determinants: the potential risk to human health, its potential to spread, and the risk of insufficient control capacities. RRA were systematically undertaken internally by WHO staff to reflect its independent assessment of an event and evidence for decision making. The overall public health risk was categorised as either ‘very high’, ‘high’, ‘moderate’ or ‘low’ at the global, regional and national levels based on the findings from the RRA. An outbreak was assessed as low risk if it required routine response through the disease control programme while a moderate risk was assigned if the outbreak required some roles and responsibilities in addition to routine response. A high-risk outbreak was an outbreak needing a range of additional control measures including the need to establish command and control structures. A very high risk was the highest level of risk assigned and denoted that the implementation of control measures with serious consequences was highly likely.
Data sources and collection
Data on signals triaged, events detected and RRA performed during the studied period were recorded in the public health event (PHE) database maintained at AFRO as well as the WHO Events Management System (EMS) [37]. The PHE database and the EMS were the primary sources of data for the study.
Data measurement and analysis
We selected only infectious disease outbreaks reported during the studied period for our analysis and categorised them based on their modes of transmission, a similar type of pathogen or pathogenesis, or requiring a similar type of public health intervention (e.g. vaccination). We performed a descriptive analysis of the infectious disease outbreaks detected through the various modes of epidemic intelligence activities. We analysed the frequency of these outbreaks and their geographic distribution across the African region.
We also analysed the proportion of outbreaks detected and positive predictive values (PPV) of the media monitoring implemented through the internet-based epidemic intelligence platforms for the top 16 infectious disease outbreaks reported. An outbreak was considered as detected if a signal concerning the outbreak was found via the epidemic intelligence platforms before they were officially reported to WHO. This metric measured the ability of the epidemic intelligence platforms to detect an outbreak and was calculated as the total number of outbreaks detected divided by the total number of outbreaks for a disease. The results were expressed as a percentage. The PPV measured whether a signal selected through the triage process could or could not be confirmed and was calculated as the number of confirmed signals divided by the total signals selected, expressed as a percentage. The frequency and risk level categorisation were analysed for the top 11 frequent infectious disease outbreaks with finalised RRA.
R version 4.0.3 [38] was used for statistical analysis and ESRI 2017 ArcGIS Pro 2.1.0 [39] for mapping of the data.
Results
A total of 415 substantiated infectious disease outbreaks were detected through epidemic intelligence activities and reported to WHO from 2017 to 2019, with 25% (n = 104) initially detected through event-based surveillance and 75% (n = 311) through indicator-based surveillance (Table 1). During the studied period, about 100 infectious disease outbreaks were reported each year, although the number of new outbreaks decreased slightly over time. The most frequent outbreak categories were vaccine-preventable diseases (n = 108), followed by food-and-water-borne (n = 88) and vector-borne diseases (n = 77) (Table 1). The most frequent diseases causing outbreaks were cholera (n = 62), COVID-19 (n = 47), measles (n = 37), dengue fever (n = 29) and Crimean-Congo haemorrhagic fever (CCHF) (n = 26). Cholera (40.3%; n = 25) had the most outbreaks initially detected by event-based surveillance, followed by measles (27.0%; n = 10), COVID-19 (17.0%; n = 8), dengue fever (27.6%; n = 8) and CCHF (19.2%; n = 5). The types of surveillance used for the initial detection of each outbreak reported are seen in Table 1.
Table 1. Infectious disease outbreaks and conditions detected by epidemic intelligence activities (event-based and indicator-based surveillance) and reported to WHO in the African region, 2017–2020

Figure 2 shows a spot map of the geographic distribution of infectious disease outbreaks reported to WHO during the studied period, with at least one outbreak reported from each member state in the African region. Cholera and measles were the most frequent infectious disease outbreaks reported from 2017 to 2019. Additionally, circulating vaccine-derived poliovirus type 2 (cVDPV2) outbreaks emerged among the three most frequent across the region in 2019, while COVID-19 was the most frequent in 2020, with SARS-CoV-2 detected in all the member states of the WHO African region (Fig. 2).

Fig. 2. A spot map of the geographical distribution of infectious disease outbreaks reported to WHO in the African region, 2017–2020.
Over 2.8 million unverified media articles were published on the epidemic intelligence platforms from 2017 to 2020 (Fig. 3). Of these, 20 405 media signals were screened with 924 selected and sent to member states for verification. Of the selected media signals, 780 (84.4%) were either confirmed or remained under monitoring while the rest were either discarded or unverifiable. From the confirmed media signals, 104 new public health events were detected (Fig. 3).

Fig. 3. Results of event-based surveillance (media monitoring) undertaken at WHO Regional Office for Africa leading to detection of new infectious disease public health events in the African region, 2017–2020.
Of the 358 outbreaks caused by the 16 most frequent diseases reported to WHO from 2017 to 2020, 24% (n = 86) were initially detected via the epidemic intelligence platforms before official notification to WHO (Table 2). Detection ranged from 4.3% for yellow fever to 53.8% for anthrax and malaria. The most frequent disease outbreak detected was cholera (n = 25) followed by measles (n = 8). Table 2 further showed that of 1018 media signals selected for the top 16 diseases reported, 33% were confirmed with positive predictive values ranging from 8.5% for COVID-19 to 57.6% for measles. EVD (n = 100) had the highest number of signals confirmed (Table 2).
Table 2. Percent of events detected and positive predictive values of the most frequent events identified through epidemic intelligence in the WHO African region, 2017–2020
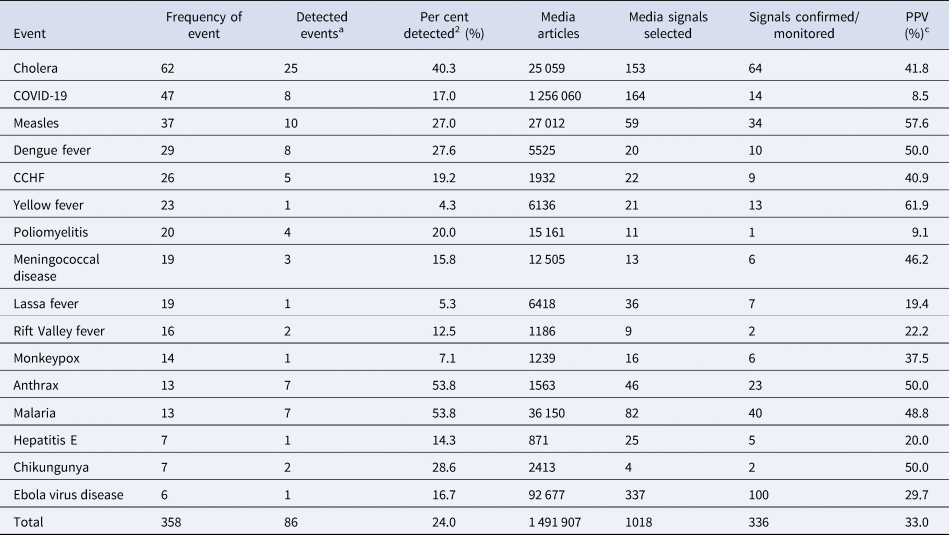
a Detected events are events detected via epidemic intelligence platforms before they were officially reported to WHO.
b Per cent of events detected via the epidemic intelligence platforms before being officially reported to WHO.
c PPV, positive predictive value, is defined as the proportion of selected media signals that were confirmed or monitored after verification.
A total of 178 RRA were conducted for infectious disease outbreaks during the studied period with cholera (24.2%; n = 43) being the most frequent, followed by EVD (13.5%; n = 24), yellow fever (10.1%; n = 18), dengue fever (6.1%; n = 11) and Lassa fever (5.6%; n = 10) (Fig. 4). Further, RRA found most outbreaks to have high risk (47.7%; n = 83) at national level, moderate risk (42.5%; n = 74) at regional level and low risk (92.9%; n = 169) at global level (Fig. 4). Only outbreaks of cholera and EVD reached very high risk at both national and regional levels. All outbreaks were assessed as low risk at the global level except for COVID-19, which was considered very high due to the ongoing pandemic.

Fig. 4. National, regional, and global levels risk characterization for the top eleven infectious disease outbreaks with frequent rapid risk assessments, WHO African region, 2017–2020 (N = 178).
Discussion
Epidemic intelligence activities have detected and verified a large number of infectious disease outbreaks in the WHO African region, highlighting the frequency of outbreaks of cholera and measles over the whole study period. In 2019 outbreaks of cVDPV2 increased in frequency, while in 2020 the global pandemic of COVID-19 was reflected in the frequency of its detection and wide geographical spread across the region. Our results have shown that epidemic intelligence activities have contributed to the detection and reporting of infectious diseases across the region.
Although IDSR specifies the use of both indicator and event-based surveillance, we found that the member states mainly relied on formal reporting sites for the detection of outbreaks, thus the high number initially detected through indicator-based surveillance. According to Fall et al., implementation of event-based surveillance was inadequate despite the wide adoption and scale-up of IDSR across the African region [Reference Fall40]. The initial detection of a quarter of all outbreaks through event-based surveillance from media sources showed that this mode of epidemic intelligence is a useful complement in the early detection of outbreaks. This point has been reinforced in the third edition of the IDSR technical guidelines, recently adopted by the WHO African region [41].
Although vaccines are known to be one of the most efficient, high-impact public health measures to prevent diseases, immunisation rates across most of the WHO African region have been sub-optimal, mainly challenged by issues related to funding, stock-outs, logistics and accessibility, among others [Reference Mihigo42]. Our finding of high frequency and recurrence of vaccine-preventable disease outbreaks in the region during the studied period could be attributed to these challenges. Food-and-water-borne disease outbreaks have also remained prevalent in the region, with cholera being the most frequently detected and reported. Poor water, sanitation and hygiene (WASH) infrastructures are the most cited key factors driving the recurrence of food-and-water-borne disease outbreaks, however, the role of environmental reservoirs as well as anthropogenic and hydroclimatic drivers have also been highlighted [Reference Gwenzi and Sanganyado43].
Additionally, the changing environment, particularly changes in the socio-ecological systems and climate, have undoubtedly influenced the recurrence of outbreaks of some emerging and reemerging diseases, which we categorised as vector-borne and viral haemorrhagic fevers [Reference Campeau44]. There are recurrent outbreaks of these diseases, such as dengue fever, Rift Valley fever, CCHF, Lassa fever and EVD. Also, the emergence of several outbreaks of cVDPV2 in 2019 and the spread of SARS-CoV-2 globally in 2020 in all members states of the WHO African region highlights the effects of novel diseases and conditions on human health and development in the region.
With the increasing threat of emerging and reemerging infectious diseases, the role of epidemic intelligence remains crucial for preparedness and response to these events. Integrated surveillance systems such as IDSR, when implemented holistically, are central to early detection of outbreaks and, most importantly, can help mitigate the devastating social and economic consequences of epidemics. The ability of media monitoring to detect several outbreaks not only confirm the invaluable contribution of internet-based sources to early outbreak detection, but also emphasise how adopting standardise and thorough processes for triaging rumours can reduce noise and improve the PPV of media monitoring.
Moreover, the epidemic intelligence activities undertaken at AFRO may have contributed to the early detection of outbreaks and indirectly influenced the timeliness of WHO response actions to support member states in mounting public health response to outbreaks with potential for international spread. Impouma et al. found that the timeliness of detection and control of outbreaks across the African region improved from 2017 to 2019, attributed to enhanced epidemic intelligence, among other factors [Reference Impouma45]. For example, in 2017 WHO received initial information on a cluster of cases presenting with EVD-like symptoms in the Democratic Republic of the Congo three days before the event was confirmed and officially notified by the country. A verification request from WHO to the member state triggered field level investigation and within 24 h of confirmation and official notification, an RRA was finalised, which provided the basis for further operational actions. The rapid response mounted by the member state with support from WHO and partners cannot be discounted among factors that led to an eventual control of the outbreak within two months.
The WHO emergency response framework requires evidence for decision making when supporting member states’ response to epidemics [18]. The RRA provided the evidence base with which to inform the scale of WHO support in order to implement appropriate and timely public health response measures. The high frequency of RRA for some outbreaks such as cholera, EVD, dengue fever and Lassa fever highlighted the potential of these outbreaks to cause significant impact to human health. Most outbreaks were assessed as high risk at national level, indicating that these outbreaks could quickly overwhelm national capacities and spread to new sub-national areas, with high morbidity and mortality. While most outbreaks had moderate risk at the regional level, outbreaks of cholera and EVD were found to have high and very high risk at regional levels indicating their potential to cross national boundaries and affect other member states in the region, resulting in strain on their health systems. COVID-19 was assessed as very high risk at the global level in light of the ongoing pandemic and its impact across the globe.
Implementing epidemic intelligence activities in the African region has not been without challenges. First, event-based surveillance has not been fully scaled-up across the African region, partly due to the additional logistical, human and financial resources that are required for its implementation. While media monitoring undertaken at AFRO have been useful, in-country strengthening of event-based surveillance activities, for example, community-based surveillance and media monitoring, would have further enhanced the timeliness of outbreak detection and response at sub-national levels. Secondly, the internet-based epidemic intelligence platforms generated a high volume of noise, resulting in hundreds or thousands of articles, making triaging laborious. Additionally, while articles from formal media sources have been invaluable, the increasing popularity and use of social media platforms such as Facebook and Twitter in the region could have contributed much more to the pool of epidemic intelligence information if they were included as sources of information on the epidemic intelligence platforms. Thirdly, for some events such as EVD and COVID-19, the heightened awareness, concerns and increased rumours associated with outbreaks meant that many more signals needed to be investigated to rule out new flare-ups or events in order to ensure that the population was protected from any eventual spread of these diseases. The importance of increasing sensitivity to capture all possible signals resulted in lower positive predictive values and increased human and financial resources needed to investigate additional signals. There was a higher importance to acquire sufficient sensitivity at the cost of lower PPV.
There are four main limitations of our analysis. First, our list of outbreaks may not be exhaustive since we considered only those required for notification to WHO as per IHR 2005 Annex 2A decision instrument. Second, the detection metrics is not an ideal measurement for sensitivity. The relatively low detection measured for most of the top 16 infectious disease outbreaks were due to the exclusion of signals appearing on the epidemic intelligence platforms after the outbreaks were officially reported to WHO through other channels. This means that the detection metrics cannot be used as a proxy for sensitivity of the epidemic intelligence platforms, that is, the ability of the platforms to detect outbreaks irrespective of whether first reported to WHO or not. Third, several signals remained unverified as of the time of our analysis and therefore could not be classified as confirmed or discarded. This may have led to underestimation of the positive predictive values reported. Finally, we did not systematically capture data on the number of duplicate articles filtered during the triaging process, hence, we were unable to exclude duplicates from the total number of unique media articles populating the platforms. While the epidemic intelligence platforms automated the de-duplication of some of the articles that were nearly identical in text, there were articles which provided duplicative information which were not filtered out.
Conclusion
Despite these challenges and limitations, epidemic intelligence activities have proven useful to the early detection and response to outbreaks in the African region. The adoption of standardised processes and tools for epidemic intelligence, including rapid risk assessments, not only enhanced outbreak detection in the region but also provided an objective means of monitoring the evolution of outbreaks and generating evidence to guide WHO in the deployment of its resources and technical expertise. The implementation of media monitoring using internet-based platforms in addition to routine indicator-based surveillance, shows that in an increasingly digital world, there is growing reliance on unofficial sources for first reports about outbreaks.
Recommendations
We recommend that AFRO continues to invest in strengthening epidemic intelligence as part of preparedness for and response to events of public health concern through building countries’ capacities to implement event-based surveillance including the scale-up of the use of platforms such as EIOS in the African region. Expanding sources on the current epidemic intelligence platforms to include social media would further increase the chances of detecting events early and should be prioritised. There is also a need to strengthen routine disease prevention programmes by addressing factors that limit their effectiveness to prevent recurrent outbreaks.
Acknowledgements
The authors would like to thank all colleagues, particularly WHO staff, partners and Ministries of Health, whose tireless efforts have shaped the epidemic intelligence landscape across the African region and save many more lives from the scourge of epidemics.
Data availability statement
The authors confirm that the datasets used for this study are available on request.