Phytosterols/phytostanols (PSS) are bioactive compounds found in vegetable oils, nuts seeds(Reference Phillips, Ruggio and Ashraf-Khorassani1), which are also added to many commercial food products such as margarine and yoghurts(Reference St-Onge and Jones2). There are a wide variety of PSS variants found in nature(Reference Nes3), many of which are not yet fully characterised. Structural and functional differences between cholesterol and PSS derive from the presence of methyl or ethyl groups on the side chain, double bonds in the side chain at C22–C23, and in the case of phytostanols, saturation of the B-ring (Fig. 1). Just as cholesterol is a major component of mammalian cell membranes, PSS are major components of plant cell membranes where they control fluidity and permeability(Reference Ratnayake, L’Abbe and Mueller4). In addition, PSS are precursors to steroid hormones such as brassinosteroids that control the life cycle physiological processes of the plants(Reference Fujioka and Yokota5), and production can change in response to stress, developmental stage and osmotic pressure. Despite a relatively low absorption rate (<5 %) of dietary PSS(Reference Jimenez-Escrig, Santos-Hidalgo and Saura-Calixto6,Reference Ostlund, McGill and Zeng7) , compared with that of dietary cholesterol (30–80 %) in humans(Reference Bosner, Lange and Stenson8), PSS still reach micromolar concentrations in human serum(Reference Stiles, Kozlitina and Thompson9,Reference Genser, Silbernagel and De Backer10) and exert physiological and systemic changes to the body. For example, consumption of 2–3 g/d of PSS reduces circulating levels of total cholesterol by 5–10 %, LDL-cholesterol by 7·5–12 %, and triacylglycerol by 0·1 mmol(Reference Wu, Fu and Yang11–Reference Musa-Veloso, Poon and Elliot13). Therefore, for the treatment and management of hyperlipidaemia, PSS consumption is recommended by the National Lipid Association, the European Society of Cardiology and the European Atherosclerosis Society(Reference Jacobson, Ito and Maki14,Reference Catapano, Graham and De Backer15) . In addition, two meta-analyses have concluded that PSS consumption was linked to a reduced risk of developing some cancers in humans(Reference Jiang, Zhao and Xu16) and reduced cancer burden in preclinical models(Reference Cioccoloni, Soteriou and Websdale17). More broadly, PSS have also been proposed to have anti-inflammatory, anti-microbial, blood pressure lowering, anti-diabetic and anti-obesity properties (reviewed in(Reference Nattagh-Eshtivani, Barghchi and Pahlavani18)).
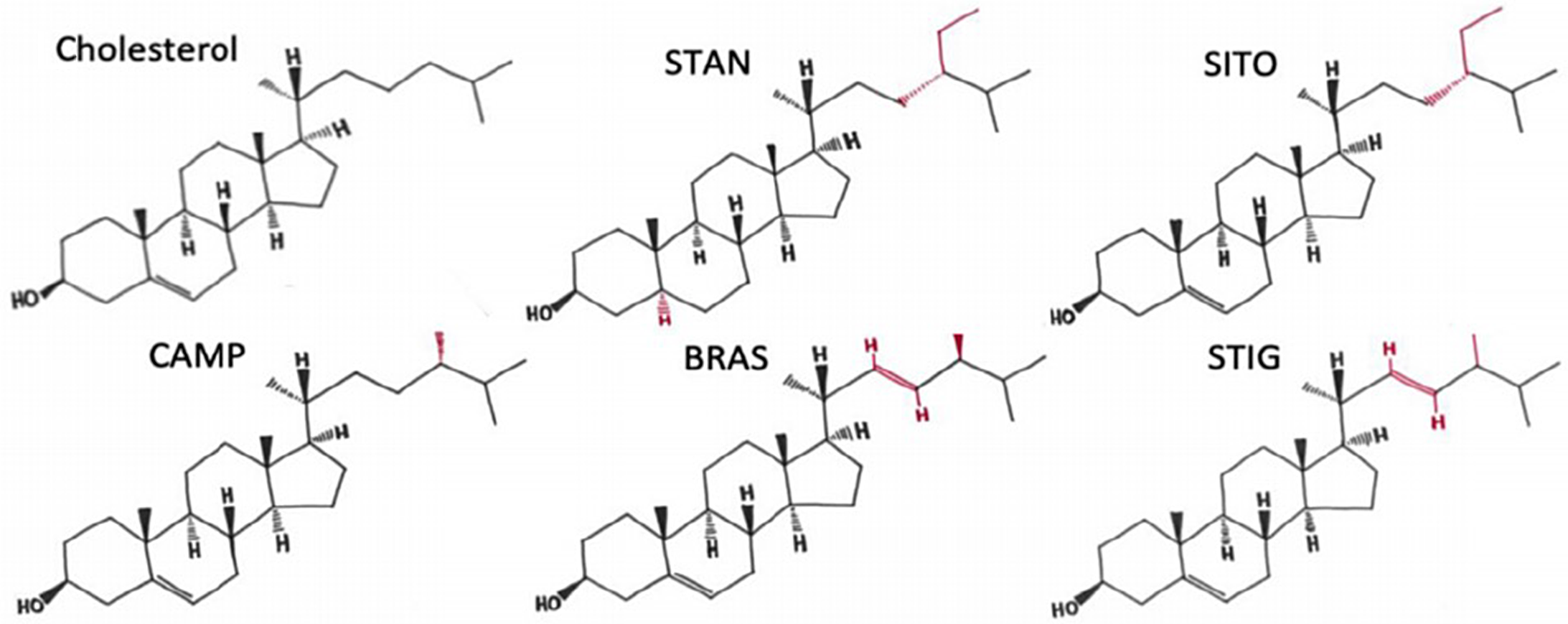
Fig. 1. The structures of cholesterol, sitostanol and phytosterols commonly consumed in a healthy human diet. STAN, sitostanol; SITO, β-sitosterol; CAMP, campesterol; STIG, stigmasterol. Regions of structural variability from CHOL are shown in red. Adapted from(Reference Hutchinson, Lianto and Moore32) and Licensed under CC BY 4.0.
The molecular mechanisms that convey inheritance of epigenetically regulated gene expression patterns include: post-translational modification of histones, DNA methylation and regulation of miRNA expression. Histone post-translational modifications through their interactions with transcription factors provide a nuanced level of control over gene expression. Balance in the levels of histone acetylation is maintained by opposing activities of acetyl depositing enzymes (histone acetyltransferases) and the histone deacetylase (HDAC) enzymes that remove the acetyl group. The eighteen HDAC enzymes are grouped into four classes by sequence homology and play important roles in regulating gene expression(Reference Ropero and Esteller19). Chemical inhibitors of HDAC (HDACi) are emerging as anti-cancer agents owing to their ability to induce cancer cell apoptosis(Reference Bao, Diao and Dong20), limit proliferation and modulate behaviour of immune system cells(Reference Kim and Bae21). For example, the HDACi givinostat is in phase II clinical trials for the rare blood cancer polycythaemia vera(Reference Rambaldi, Iurlo and Vannucchi22) and a phase III trial for Duchenne muscular dystrophy (NCT02851797). DNA methylation, where a methyl group is added to the CpG Island in the promoter region by DNA methyltransferase (DNMT) enzymes, is essential for fundamental processes such as transcriptional regulation, chromatin structure, embryonic development, X chromosome inactivation and genomic imprinting(Reference Meng, Cao and Qin23). Lastly, expression of micro-RNA (miRNA) may also be conveyors of epigenetic information when inherited during cell division. Often referred to as oncomirs, miRNA are widely reported to be dysregulated in cancers of the breast(Reference Verghese, Drury and Green24,Reference Thorne, Battaglia and Baxter25) , blood(Reference Calin, Ferracin and Cimmino26) and thyroid(Reference He, Jazdzewski and Li27). Interestingly, many miRNA are regulated by vitamins and other dietary components(Reference Thorne, Battaglia and Baxter25,Reference Thorne, Maguire and Doig28,Reference Zhang, Moon and Thorne29) .
The exact mechanisms through which PSS exert their effects remain unclear, but previous work has implicated induction of sterol metabolism and transport pathways(Reference De Smet, Mensink and Plat30,Reference Nakano, Inoue and Murakoshi31) , interference with ligand dependent transcription factor activity(Reference Hutchinson, Lianto and Moore32,Reference Plat, Nichols and Mensink33) and inhibition of oncogene signalling(Reference Cioccoloni, Soteriou and Websdale17). Given the change in steady-state levels of PSS in the body after long-term consumption, a key question is whether PSS, as for various other dietary compounds(Reference Tiffon34), may be dietary modifiers of epigenetic gene expression patterns. Therefore, the aims of this systematic review were to: (i) ascertain the depth of literature that has previously investigated links between PSS and epigenetic molecular mechanisms; (ii) understand the potential mechanisms through which PSS may influence epigenetic inheritance of gene expression patterns related to health and disease and (iii) identify high confidence PSS-regulated epigenetic pathways that could be used as controls in future studies.
Methods
Search strategy
A systematic search of titles and abstracts was carried out up to and including February 2023 in three online databases: PubMed, Scopus and Web of Science, using pre-defined criteria informed by PROSPERO. Keywords encompassed PSS and epigenetic-related terms (Table 1). Records were collected and duplicates removed using Endnote X9·3·3, and titles/abstracts were screened using Rayyan software (https://www.rayyan.ai/).
Table 1. Search terms syntax for three databases

Study selection
Titles and abstracts were screened for eligibility by two independent researchers (E. J, M. X) and a third researcher resolved any discordance. Inclusion criteria were (i) original papers; (ii) English, Chinese or Arabic languages; (iii) conducted on PSS or their derivatives and (iv) measured an epigenetic molecular mechanism. Epigenetic molecular mechanisms were defined as either: (i) changes to miRNA expression levels; (ii) changes to levels of histone post-translational modifications or expression of histone PTM enzymes or (iii) changes to CpG island methylation or expression of DNA methylation enzymes. We did not consider transience of founder event, or meiotic or mitotic heritability, as requisite factors for inclusion.
Data extraction
All data were extracted into Microsoft Excel. Data extracted included: PSS type, PSS delivery method, exposure time and concentration, epigenetic mechanism, molecular epigenetic factor such as regulated enzyme or miRNA, hypo/hypermethylation of CpG promoters and cell/tissue type (Table 2).
Table 2. Summary of the data extracted from the included studies (n 11) illustrating the effects of PSS on the epigenetic mechanisms

PSS, phytosterols/phytostanols; 231, MDA-MB-231; ATA, Antcin-A; BCa, breast cancer; BEC, bronchial epithelial cells; β-SITO, β-sitosterol; β-SDG, β-SITO-D-glucoside; CAMP, campesterol; COPD, chronic obstructive pulmonary disease; ERGO, ergosterol; HAEC, human aortic endothelial cells; ELISA, enzyme-linked immunosorbent assay; N/A, not applicable; NSC, neural stem cells; PCa, prostate cancer; qPCR, quantitative polymerase chain reaction; SITO, sitosterol; STIG, stigmasterol; γ-SITO, γ-sitosterol; WB, western blot.
Risk of bias assessment
The risk of bias analysis was performed using a designed multi-point questionnaire completed by two researchers working independently; disagreements were resolved by discussion with a third member of the research team. A total of twenty-five questions were created to assess the experimental, statistical and reporting transparencies. Questions related to qPCR data were created from the Minimum Information for Publication of Quantitative Real-Time PCR Experiments (MIQE) guidelines(Reference Bustin, Benes and Garson35); while those for immunoblotting data were created from the guidelines of the British Journal of Pharmacology(Reference Alexander, Roberts and Broughton36).

Fig. 2. PRISMA flow diagram showing numbers of records. The numbers of the included and excluded studies in each step; identifications from the three databases, titles/abstracts, full-text screening and the final numbers that are eligible for this systematic review.
Results and discussion
After duplicates were removed, 229 titles and abstracts were included for abstract screening, which left sixteen records for full-text screening; of which eleven were eligible for data extraction (Table 2). The PRISMA flow diagram summary is shown in Fig. 2. The PSS reported were sitosterol (SITO) in five studies(Reference Jiang, Yang and Yuan37–Reference Shen, Liu and Qu42), Antcin-A (ATA)(Reference Kumar, Vani and Hsieh43,Reference Kumar, Vani and Hsieh44) and stigmasterol (STIG)(Reference Ishola and Adewole45,Reference Hohoayi, Antwi and Amoah46) in two studies each and ergosterol (ERGO)(Reference Sun, Liu and Feng47), and campesterol (CAMP)(Reference Ishola and Adewole45) in one each. Four studies completed experiments in breast cancer cell lines(Reference Xu, Li and Han38,Reference Pradhan, Parbin and Kar39,Reference Kumar, Vani and Hsieh43,Reference Senthil Kumar, Gokila Vani and Hsieh48) , two in models of coronary obstructive pulmonary disease(Reference Shen, Liu and Qu42,Reference Sun, Liu and Feng47) , one in prostate cancer cell lines(Reference Pradhan, Parbin and Kausar40), one in neural stem cells(Reference Jiang, Yang and Yuan37), one in human aortic endothelial cells(Reference Jiang, Li and Niu41), one in BALB/c-nude mice(Reference Xu, Li and Han38), one was in guinea pigs(Reference Hohoayi, Antwi and Amoah46) and one in silico(Reference Ishola and Adewole45).
Phytosterols regulate expression and function of histone deacetylase enzymes
Five studies investigated the role of PSS on HDAC expression, activity or binding(Reference Pradhan, Parbin and Kar39,Reference Pradhan, Parbin and Kausar40,Reference Ishola and Adewole45–Reference Sun, Liu and Feng47) . Two studies by Pradhan and colleagues in cancer cell lines provided in vitro evidence that PSS promote histone acetylation via HDAC inhibition. SITO inhibited the expression of HDAC1 and HDAC2 in MCF7 and MDA-MB-231 breast cancer cells(Reference Pradhan, Parbin and Kar39) and in PC3 and DU145 prostate cancer cells(Reference Pradhan, Parbin and Kausar40). In a subsequent HDAC activity assay, HDAC activity was inhibited in a SITO dose-dependent manner that was separate from the effect of the PSS on HDAC expression(Reference Pradhan, Parbin and Kausar40). A relatively high concentration of 80 μM SITO was used in this in vitro analysis; median serum concentration levels of SITO were previously reported to be 2148 ng/ml (5·2 μM) with a range of 308 ng/ml (0·74 μM) to 19 476 ng/ml (47 μM) in a study of >3000 people(Reference Stiles, Kozlitina and Thompson9). Ishola and Adewole(Reference Sowemimo, Fakoya and Awopetu49) performed molecular docking experiments and predicted several phytosterols could physically interact with class I and class II HDAC leading to inhibition of activity(Reference Ishola and Adewole45). Forty-nine compounds isolated from Morinda Lucida, a plant found throughout central and southern Africa and used in traditional medicine for the treatment of cancer(Reference Daina, Michielin and Zoete50), were compared with the HDACi givinostat for their capacity to bind and inhibit HDAC(Reference Ishola and Adewole45). Of the forty-nine compounds tested, PSS were among the strongest HDAC-binding factors. Interestingly, CAMP and STIG were predicted to bind in the same HDAC pocket as givinostat and with higher binding affinity. An Absorption–Distribution–Metabolism–Excretion analysis using Swiss online Absorption–Distribution–Metabolism–Excretion web tool indicated that several PSS adhere to the Lipinski rules of five for drug development (Table 3), underlining their suitability for clinical translation(Reference Ras, van der Schouw and Trautwein51).
Table 3. The oral drug likeness of SITO, CAMP, STIG and ATA by Lipinski’s rule of five(Reference Ras, van der Schouw and Trautwein51)

SITO, sitosterol; CAMP, campesterol; STIG, stigmasterol; ATA, Antcin-A; MLOGP, Moriguchi octanol–water partition coefficient.
Contrary to publications above that found PSS inhibited HDAC expression and function were two publications exploring the effect of PSS in lung diseases that found PSS were associated with increased HDAC expression. In a model of steroid-resistant asthma, HDAC2 protein expression was measured in lung tissue of guinea pigs after the animals were given five daily intraperitoneal injections of 50 mg/kg or 100 mg/kg STIG(Reference Hohoayi, Antwi and Amoah46). Steroid-resistant asthma animals had fivefold lower expression of HDAC2 compared with healthy controls and the addition of STIG (50 mg/kg or 100 mg/kg) ameliorated this loss of HDAC2 expression. In a study using a mouse coronary obstructive pulmonary disease model and mouse macrophage cell line (Raw264·7), exposure to cigarette smoke extract inhibited HDAC3 expression and induced expression of the histone acetylation coactivators P300 and PCAF. When increasing doses of ERGO (2·5, 5 and 10 mg/kg) were added to the cigarette smoke extract treatment, the cigarette smoke extract mediated loss of HDAC3 was ameliorated(Reference Sun, Liu and Feng47). Neither the data presented by Hohoayi et al., regarding STIG mediated increases in HDAC2 expression, nor by Sun et al., regarding ERGO induction of HDAC3, included analysis of the effect of the PSS on HDAC in control animals, nor did they directly assess HDAC activity. Given that both disease models are associated with very high free-radical environments, HDAC degradation may be inhibited by PSS via protection from oxidative damage and adduct formation from the high levels of free radical characteristic of both these disease models; indeed, this was proposed by Hohoayi and colleagues as a reason why HDAC2 levels remained high.
The experimental observations showing PSS has HDAC modulatory properties may help explain why PSS are so potent at lowering LDL-cholesterol(Reference Ras, Geleijnse and Trautwein12,Reference Musa-Veloso, Poon and Elliot13) , yet have not been conclusively linked to a reduction in CVD risk(Reference Genser, Silbernagel and De Backer10,Reference Zhao, Zhuang and Li52–Reference Yoon and Eom55) . Whether HDACi are beneficial in terms of CVD prevention is context and end point dependent. In their 2016 review, Yoon and Eom described evidence regarding the use of pharmacological HDACi to reduce risk of several CVD outcomes, such as myocardial infarction and hypertension. However, HDACi is was also linked to worse vascular calcification and indeed, and thrombus was a side effect of significant clinical importance in patients taking vorinostat(Reference Soteriou, Kalli and Connell56). The molecular mechanisms of action of how PSS inhibit HDAC function in the context of CVD therefore require further elucidation. This would also shed light on the role of PSS in other diseases where HDAC activity is important, such as cancer.
Phytosterols decrease expression of DNA methyltransferase enzymes and reduce global DNA methylation
Two studies investigated whether PSS exposure led to changes in DNA methylation by affecting DNMT expression(Reference Pradhan, Parbin and Kar39,Reference Pradhan, Parbin and Kausar40) . In the first, H2O2 was used to induce expression of DNMT1 in MCF7 and MDA-MB-231 breast cancer cells(Reference Pradhan, Parbin and Kar39). However, in the presence of 40 μM or 60 μM SITO, DNMT1 induction was prevented and both mRNA and protein levels of DNMT were reduced by PSS in both cell lines. Moreover, H2O2 downregulated the expression of anti-epithelial to mesenchymal transition factor N-cadherin/CDH1, while lupeol and SITO reversed this pro-metastatic repression of CDH1 at mRNA and protein levels. In prostate cancer cell models, DNMT1 was also reduced following exposure of cell lines (PC-3 and DU-145) to methanolic extract of Paederia foetida leaves, lupeol or SITO, for 24 h or 72 h. The data show SITO inhibited DNMT1 expression and activity, decreased the expression of anti-apoptotic protein Bcl2 and upregulated the expression of pro-apoptotic protein Bax; and, again as for breast cancer cells, CDH1 expression was increased(Reference Pradhan, Parbin and Kausar40).
Given that DNMT play essential roles in activating or silencing oncogenes and tumour suppressor genes, it is interesting to note that PSS appear to have therapeutic actions in regulating these enzymes, in cell lines at least. Further molecular analyses are required to provide a mechanistic understanding of how PSS are able to regulate activity of DNMT enzymes. Specifically, the use of coarse grained and atomistic computational simulations, which have been applied previously to determine lipid–protein interactions (reviewed in(Reference Zhang, Moon and Thorne29)), molecular docking simulations (such as those in(Reference Ishola and Adewole45) or molecular binding analyses) would determine if physical interactions occur. Further work in pre-clinical models and ultimately dietary interventions in cancer patients will be critical to understanding if PSS-mediated changes to DNA methylation patterns can translate into clinically meaningful effects.
Phytosterols regulate miRNA expression
The link between miRNA and PSS exposure was investigated in six records(Reference Jiang, Yang and Yuan37,Reference Xu, Li and Han38,Reference Jiang, Li and Niu41–Reference Kumar, Vani and Hsieh43,Reference Senthil Kumar, Gokila Vani and Hsieh48) , of which two were screening studies(Reference Jiang, Yang and Yuan37,Reference Jiang, Li and Niu41) . The screening studies evaluated SITO in the context of rat neuronal stem cells(Reference Jiang, Yang and Yuan37), and human aortic endothelial cells(Reference Jiang, Li and Niu41). In neuronal stem cells, 40 μM SITO upregulated 30, and downregulated 84, miRNA(Reference Jiang, Yang and Yuan37). While in the HAEC cells, 4·8 μM SITO upregulated 579 and downregulated 112 miRNA, albeit this was in comparison to an ox-LDL treated group(Reference Jiang, Li and Niu41). Interestingly, despite being performed in different cell types from different species, and with a ten-fold difference in concentration of SITO utilised, four miRNA were significantly altered by SITO in both studies (miR-17-5p, miR-20a-5p, miR-210-3p and miR-532-3p). Although these were not in the top regulated lists in either study and thus were not validated by PCR. In NSC cells, several SITO-regulated miRNA were found to activate the IGF1 signalling pathway, and treatment was associated with increased cellular proliferation(Reference Jiang, Li and Niu41). Similarly in HAEC, signalling pathway analysis indicated that SITO treatment enriched for AKT signalling, forkhead box O transcription factors and cell cycle signalling(Reference Jiang, Yang and Yuan37). Contrary to this, the bronchial epithelial airway cell line model of chronic obstructive pulmonary disease (BEAS-2B) responded to very low concentrations of SITO (60 nM for 24 h) by induction of miR-196-5p and suppression miR-361-5p, leading to inhibition of mTOR signalling and reduced proliferation(Reference Shen, Liu and Qu42).
In cancer, like in the pulmonary model, PSS did not stimulate proliferative or cell survival signalling, but instead tended to suppress these pathways. In BCa cell lines MCF7 and MDA-MB-231 cells, 32 μM SITO-induced apoptosis via induction of the tumour suppressor miR-10a-5p that in turn limited the activity of PI3K-AKT signalling(Reference Xu, Li and Han38). Also, in MCF7 and MDA-MB-231 cells, 10 μM ATA upregulated miR-200c(Reference Kumar, Vani and Hsieh43) and miR-708(Reference Kumar, Vani and Hsieh44). Of note is that miR-708 was significantly downregulated by SITO in HAEC cells(Reference Jiang, Li and Niu41). ATA inhibited multiple components of the epithelial to mesenchymal transition pathway, a critical step in cancer metastasis. ATA induced miR-200c expression in a p53-dependent manner, which led to repression of transcription factor ZEB1, and molecular effectors of epithelial to mesenchymal transition such as E-cadherin and vimentin(Reference Kumar, Vani and Hsieh43). ATA suppressed the pro-inflammatory NFκB pathway via miR-708-dependent inhibition of IKKβ (Reference Kumar, Vani and Hsieh44).
These data support the hypothesis that PSS regulate important cell fate decisions such as proliferation and differentiation. Importantly, cell fate decisions can be driven in either direction, in cancer PSS are anti-proliferative, anti-metastatic and pro-apoptotic, but in the non-cancer models of NSC and HAEC, PSS-enhanced proliferation and in some cases via upregulation of the same pathways that are downregulated by PSS in cancer. This context dependence indicates the molecular effectors of PSS control over cell fate are yet to be fully elucidated.
Risk of bias and study limitations
The risk of bias score was generated by assessing experimental, statistical and reporting transparency for each included study. Questions regarding experimental protocols were created according to the Minimum Information for Publication of Quantitative Real-Time PCR Experiments guidelines(Reference Bustin, Benes and Garson35) and for reporting guidelines for immunoblotting data(Reference Alexander, Roberts and Broughton36). See the table in Supplementary (Table S1). Generally, all the studies received a minimal risk of bias rating (Fig. 3). In terms of statistical and experimental transparency, most studies had minimal risk of bias. Other aspects of reporting transparency assessed as low across the studies were: a lack of information on reagent catalogue numbers, reverse transcription and qPCR experimental variables, a lack of size marker reporting in immunoblot images and a lack of reporting across all studies about how PSS were stored and delivered to cell lines (e.g. use of carriers). Apart from these gaps, other criteria were generally sufficiently reported so risk of bias for the included studies was considered low.

Fig. 3. Risk of bias score. The risk of bias assessment for the included studies using a designed multi-point questionnaire. The score presented as green indicated the low risk of bias, red indicated the high risk of bias and yellow indicated the neutral risk of bias.
Perhaps the major limitation of this systematic review was the paucity of studies returned during the search phase. The corollary of which is that we have been unable to adopt previous examples of good practice when reviewing miRNA pathways; namely, requiring a miRNA to be both experimentally independently validated (e.g. QPCR, genetic or other functional confirmation of high-throughput expression data) and reported as differentially regulated in two or more publications to warrant inclusion(Reference Katan, Grundy and Jones57).
Conclusion
There is international consensus that the high consumption of seeds, nuts, legumes, fruits and vegetables reduces the risk of cardiometabolic disease and cancer. However, further studies characterising the bioactive compounds and mechanisms of action conferring health benefits are required. PSS enriched in plant-based diets lower pathogenic blood lipids(Reference Ras, Geleijnse and Trautwein12,Reference Jacobson, Ito and Maki14,Reference Windler, Beil and Berthold58) , are linked to reduced cancer burden and risk(Reference Jiang, Zhao and Xu16,Reference Cioccoloni, Soteriou and Websdale17) and a range of other diseases. Our systematic review has collated important knowledge regarding how PSS may act as epigenetic modifiers with the following notable observations.
PSS could be amongst the most potent naturally occurring HDACi reported to date(Reference Ishola and Adewole45). This potency, coupled with PSS’s suitability as orally administered agents as suggested by their high scores in the Lipinski test, indicates that further examination exploring their use as HDACi in clinic is warranted (Table 3)(Reference Ras, van der Schouw and Trautwein51). Nonetheless, clarity regarding their role in preventing and exacerbating different CVD clinical endpoints is required. As described recently, PSS are very unlikely to exacerbate CVD risk(60), but gaps remain in understanding the mechanism of action of how they reduce LDL-cholesterol and global histone acetylation analyses may shed light on this phenomenon. PSS inhibit expression and activity of DNMT that methylate CpG islands, leading to re-expression of genes silenced in disease states(Reference Pradhan, Parbin and Kar39,Reference Pradhan, Parbin and Kausar40) . PSS regulate perhaps hundreds of miRNA, but validation of individual miRNA in multiple studies was only possible for five miRNA in total. More screens in more cell and tissue types are required to understand the true scale of miRNA regulation by PSS across tissues.
These epigenetic gene expression regulatory mechanisms impinge on several diseases. In cancer, PSS induce miRNA that lower expression of factors such as ZEB1 that promote the epithelial to mesenchymal transition, a critical step in cancer metastasis(Reference Kumar, Vani and Hsieh43) and suppress miRNA allowing increase in expression of factors that maintain an epithelial phenotype, such as CDH1(Reference Kumar, Vani and Hsieh43). PSS also inhibit components of inflammatory pathways, including NFκB and mTOR signalling, in models of coronary obstructive pulmonary disease(Reference Shen, Liu and Qu42) indicating a potential dietary route to ameliorating these conditions. Investigations into the epigenetic effects of PSS on many cell types, including those of the immune system, are lacking from the literature thus far. Given the emerging understanding that PSS may ameliorate or even prevent a range of diseases, evaluating the role of PSS in epigenetic reprogramming is of increasing clinical and public health interest.
Acknowledgements
E. J. is supported by a grant from Saudi Arabian Cultural Bureau and Umm Al-Qura University. This work was in part supported by grant IIG_FULL_2021_019 obtained from World Cancer Research Fund (WCRF UK), as part of the World Cancer Research Fund International grant programme by J. L. T.
J. L. T. and E. J. contributed to the review conception and design. E. J. screened records, extracted and analysed data, created figures and tables and contributed to an initial manuscript draft. M. X. screened records and extracted data. J. L. T. and J. B. M. supervised the project. J. L. T. wrote the final manuscript. All authors critically reviewed the manuscript for intellectual content and approved final version. Fig. 1 is Adapted Material from(Reference Hutchinson, Lianto and Moore32) and shared under CC BY 4·0 License. The graphical abstract was created with BioRender.com under license by E. J.
All authors declare that they have no competing interests.
Supplementary material
For supplementary material/s referred to in this article, please visit https://doi.org/10.1017/S0007114523002532