Trans-fat has been linked with an increased risk of heart disease and various health organisations have recommended dietary intake be reduced(1, 2). However, trans-fatty acids (fats containing at least one double bond in the trans configuration) are a heterogeneous group of molecules, probably having differing effects on health. Trans-fats consumed in the diet are produced either during the partial hydrogenation of vegetable oils or are present naturally in ruminant products (beef and dairy) by the biohydrogenation of PUFA by micro-organisms in the rumen(Reference Eckel, Borra and Lichtenstein3, Reference Lock and Bauman4). Emerging evidence supports a distinction between industrially produced trans-fats (i.e. elaidic acid) and trans-fats produced naturally in beef and milk (i.e. conjugated linoleic acid (CLA) and vaccenic acid (VA)) based on their differing effects on health(Reference Chardigny, Destaillats and Malpuech-Brugere5). A number of different health effects have been ascribed to the ‘natural’ trans-fatty acid CLA (for a review, see Bhattacharya et al. (Reference Bhattacharya, Banu and Rahman6)) and producers have been successful at increasing the CLA content of milk and beef through various feeding strategies (for reviews, see Lock & Bauman(Reference Lock and Bauman4) and Mir et al. (Reference Mir, McAllister and Scott7)). However, as the CLA content of beef and dairy increases, so does the VA (trans-11-18 : 1) content of the product(Reference Cruz-Hernandez, Kramer and Kennelly8, Reference Gillis, Duckett and Sackmann9). Interest in VA has increased based on its ability to be converted to the cis-9, trans-11 form of CLA, including in humans(Reference Turpeinen, Mutanen and Aro10). It remains to be seen whether dietary VA shares the health benefits ascribed to CLA or the reputed deleterious health effects of the industrially produced trans-fatty acids.
Our group has recently reported hypotriacylglycerolaemic effects of dietary VA at 1·5 % (w/w) in the obese JCR:LA-cp rat(Reference Wang, Lu and Ruth11). We also reported lower serum IL-10 concentrations with dietary VA in obese rats, suggesting an alteration in T-cell function(Reference Wang, Lu and Ruth11). Obesity has been associated with both impaired immune responses and an exaggerated pro-inflammatory response(Reference Ruth, Taylor and Zahradka12, Reference Falagas and Kompoti13). Researchers have shown that in healthy subjects dietary VA at 3 g/d was incorporated into the cellular membranes of immune cells, but did not alter the phenotype of immune cells in the blood, phagocytic activity of granulocytes, or serum concentrations of cytokines, adipokines and eicosanoids(Reference Kuhnt, Kraft and Vogelsang14). Ruth et al. (Reference Ruth, Taylor and Zahradka12) have shown incorporation into membrane phospholipids and alterations in T-cell function after feeding CLA (cis-9, trans-11) in an obese rat model. The mechanistic effects of dietary VA on the immune abnormalities associated with obesity and insulin resistance are not known.
To explore the potential immunomodulatory properties of VA in a chronic inflammatory state we examined the effects of both obesity and dietary VA on splenic and mesenteric lymph node (MLN) immune cell phenotypes and function, using an established rat model of the metabolic syndrome, the JCR:LA-cp rat. The fatty acid composition of the two diets was designed in such a way that the major difference was in the presence or absence of VA. We were interested in whether there would be incorporation of VA and cis-9, trans-11-CLA (due to conversion from VA) into immune cell phospholipids and whether this would influence the concentration of proliferative (IL-2), inflammatory (IL-1β, IL-6, TNFα, interferon (IFN) γ) and regulatory (IL-10) cytokines produced from the VA-fed rats compared with controls. The JCR:LA-cp rat spontaneously develops obesity, insulin resistance, dyslipidaemia and atherosclerosis if homozygous for the autosomal recessive corpulent (cp) gene (cp/cp)(Reference Russell, Graham and Richardson15). Rats that are heterozygous (+/cp) or homozygous normal (+/+) are lean and metabolically normal(Reference Russell, Graham and Richardson15). Therefore, this animal model allows us to examine the effects of dietary VA in both a healthy animal and one with severe insulin resistance and at risk of atherosclerosis. We have recently reported that the JCR:LA-cp rat exhibits serum markers of chronic low-grade inflammation(Reference Wang, Lu and Ruth11) similar to that reported in humans with the metabolic syndrome(Reference Eckel, Grundy and Zimmet16); however, little is known about the impact of obesity on T-cell function in this animal model.
Experimental methods
Animals and diets
Male JCR:LA-cp rats, aged 6 weeks, were obtained from our established breeding colony maintained at the University of Alberta. Rats were housed individually and animal care was provided in accordance with a protocol approved by the Animal Policy and Welfare Committee of the Faculty of Agriculture, Forestry and Home Economics at the University of Alberta. For 2 weeks, rats were fed standard rat chow (Lab diet 5001; PMI Nutrition International, Brentwood, MO, USA) with 1 % (w/w) cholesterol (Sigma, Oakville, ON, Canada) to exacerbate inflammatory processes and accelerate atherosclerosis(Reference Wang, Lu and Ruth11). At age 8 weeks, obese (cp/cp; n 20) and lean rats (2:1 mix of heterozygous (+/cp) and homozygous normal (+/+); n 20) were randomly assigned to one of two nutritionally adequate basal diets: a control diet (0 % VA) or a diet supplemented with VA (1·5 %, w/w) for 3 weeks. Both diets were prepared at the University of Alberta using a nutritionally adequate basal diet (Harlan Teklad, Madison, WI, USA) with a 15 % (w/w) fat mixture as described previously(Reference Wang, Lu and Ruth11). Both diets contained (per kg): 267 g casein, 539 g carbohydrate (222 g starch, 231 g dextrose) and 150 g fat. Both diets provided a PUFA:SFA ratio of 0·6. The diets were carefully designed to have similar fatty acid compositions, differing only in VA (trans-11-18 : 1) and oleic acid (cis-9-18 : 1). Dietary fatty acid composition measured by GC is shown in Table 1. Both feed intake and body weight were recorded weekly over the 3-week feeding period.
Table 1 Fatty acid composition of diets (g/100 g total fatty acids)*
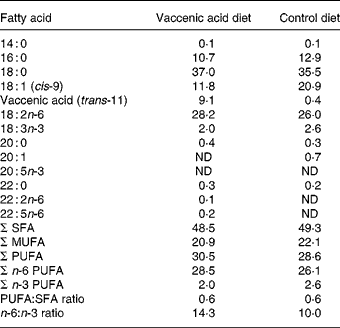
ND, not detectable.
* Diets contained 150 g fat/kg from a mixture of sources (sunflower-seed oil, flaxseed oil, soya, tallow, olive oil (control diet) and vaccenic acid (vaccenic acid diet)). Fatty acids not reported: 12 : 0; 16 : 1; 17 : 0; 17 : 1; 20 : 2; 24 : 0.
Tissue collection
Rats were anaesthetised with isoflurane and exsanguinated via cardiac puncture. The spleen and MLN were removed aseptically, placed in PBS and processed immediately.
Isolation of immune cells and estimate of proliferation
Immune cells were isolated from the spleen and MLN as previously described(Reference Field17). Briefly, single cell suspensions were obtained by disrupting tissue through a nylon mesh screen in Krebs–Ringer HEPES buffer with bovine serum albumin (5 g/l) (Sigma, Oakville, ON, Canada). Erythrocytes were lysed with ammonium chloride lysis buffer (155 mm-NH4Cl, 0·1 mm-EDTA, 10 mm-KHCO3; Fisher Scientific, Edmonton, AB, Canada). Cells were subsequently washed and re-suspended in complete culture media (Roswell Park Memorial Institute (RPMI) 1640 media supplemented with 5 % (v/v) heat-inactivated fetal calf serum, 25 mm-HEPES, 2·5 mm-2-mercaptoethanol and 1 % antibiotic/antimycotic (pH 7·4); Invitrogen, Burlington, ON, Canada). Cell concentration and viability were determined using a haemocytometer and trypan blue dye exclusion, respectively.
Plasma and mesenteric lymph node phospholipid fatty acid composition
Lipids were extracted from plasma and MLN cells by a modified Folch method as previously described(Reference Field, Ryan and Thomson18). C15 : 0 TAG standard (100 μg/ml) was added to plasma samples as an internal control. Total phospholipids were separated on silica G plates and visualised with 8-anilino-1-naphthalenesulfonic acid under UV light using the appropriate standards(Reference Layne, Goh and Jumpsen19). The phospholipid silica band was scraped and used to prepare fatty acid methyl esters. The base-catalysed method using sodium methoxide was used to prepare methyl esters as described elsewhere(Reference Cruz-Hernandez, Deng and Zhou20). Prepared phospholipid fatty acid methyl esters were flushed with N2 and stored at − 35°C until analysis by GC. Fatty acids were then analysed by automated GLC (Varian 3800; Varian Inc., Mississauga, ON, Canada) using a 100 m CP-Sil 88 fused capillary column (Varian Inc.) as described elsewhere(Reference Cruz-Hernandez, Deng and Zhou20).
Phenotyping
Monoclonal antibodies against rat CD3 (PE label, clone G4.18, isotype IgG3,k), CD4 (APC label, clone OX-35, isotype mouse IgG2,k), CD11b/c (PE label, clone OX-42, isotype IgG2a,k), CD25 (PE label, clone OX-39, isotype mouse IgG1,k), CD28 (FITC label, clone JJ319, isotype mouse IgG1,k), CD45RC (FITC label, clone OX-22, isotype mouse IgG1,k) and CD90 (PerCP label, clone OX-7, isotype mouse IgG1,k) were obtained from BD BioScience (Mississauga, ON, Canada). CD8 (RPE-Cy5 label, clone OX-8, isotype mouse IgG1,k) and CD152 (FITC label, clone WKH203, isotype mouse IgG1) were obtained from AbD Serotec (Raleigh, NC, USA). Immune cell phenotypes were determined by flow cytometry using isolated immune cells as previously described(Reference Layne, Goh and Jumpsen19). Fluorescent antibodies were used in the following combinations: CD28/CD3/CD8/CD4; CD152/CD25/CD8/CD4; CD45RC/CD3/CD90/CD4; CD45RC/CD8/CD4; CD11b/c; OX-12. Splenic and MLN cells were then washed twice with PBS supplemented with fetal calf serum (4 g/l) and fixed with 1 % (w/v) paraformaldehyde. Data were acquired on a FACSCalibur four-colour flow cytometer (Becton Dickinson, San Jose, CA, USA) and analysed using CellQuest software (Becton Dickinson, San Jose, CA, USA). Unstained cells were used to assess autofluorescence, while single colour samples were employed to adjust colour compensation.
Cytokine determination
Splenocytes (1·25 × 106 cells/ml) were incubated for 48 h with 5 % CO2 in either the presence or absence of concanavalin A (ConA; 2·5 μg/ml; MP Biomedicals, Montreal, Quebec, Canada) or lipopolysaccharide (LPS; 100 μg/ml, Sigma). Cells from the MLN were incubated in either the presence or absence of ConA. After the 48 h incubation, cells were centrifuged for 10 min at 1000 rpm. Supernatant fractions were collected and stored at − 80°C until analysis. The concentration (detection limits in parentheses) of IL-1β (62·5–4000 pg/ml), IL-2 (23·4–1500 pg/ml), IL-6 (78–5000 pg/ml), IL-10 (15·6–1000 pg/ml), IFNγ (31·25–2000 pg/ml) and TNFα (31·2–2000 pg/ml) in the supernatant fractions were determined using a commercially available ELISA kit (IL-1β, IL-2, Medicorp Inc., Montreal, Quebec; IL-6, IL-10, IFNγ, TNFα, BD Biosciences, Mississauga, ON, Canada). All samples were analysed in duplicate with CV < 10 %. All plates were read according to the manufacturer's specifications in a microplate reader (SpectraMax 190; Molecular Device, Sunnyvale, CA, USA).
Statistical analysis
All statistical analyses were conducted using SAS statistical software (version 9.1; SAS Institute Inc., Cary, NC, USA). Data are expressed as least squares means with their standard errors. Normality of the distribution was tested and data were log-transformed if necessary. Data were analysed by two-way ANOVA for main effects of diet and genotype as well as the interaction between diet and genotype. Differences among groups were identified using LSMeans. For all results, P < 0·05 was considered statistically significant.
Results
Effect of obesity and vaccenic acid on feed intake, body weight and organ weights
Obese (cp/cp) rats had significantly higher final body weight (447 (sem 5) v. 303 (sem 7) g; P < 0·0001) and average feed intake (38 (sem 0·6) v. 20 (sem 0·5) g/d; P < 0·0001) compared with lean rats. There was no effect of VA on body weight or feed intake in either obese or lean rats during the experimental treatment period. Absolute spleen weight did not differ between diet groups or genotype (0·77 (sem 0·02) g; n 40), but when corrected for body weight, obese rats had lower spleen weight compared with lean rats (1·8 (sem 0·04) v. 2·5 (sem 0·06) mg/g body weight; P < 0·001), respectively. There was no net effect of obesity on the number of cells per spleen. However, obese rats had a lower number of cells per g of spleen compared with lean rats (2·0 × 108 (sem 0·1 × 108) v. 2·5 × 108 (sem 0·2 × 108); P = 0·02), respectively. We also observed a significant interaction between diet and genotype (P = 0·01). Lean rats fed VA had higher spleen weights per g of body weight compared with lean rats fed the control diet (2·7 (sem 0·08) v. 2·4 (sem 0·07) mg/g; P = 0·007), while there was no significant difference between the obese rats fed the VA and control diets. Despite no effect of diet on absolute spleen weight, rats fed VA had a lower number of splenocytes per spleen compared with controls (1·5 × 108 (sem 0·1 × 108) cells v. 1·9 × 108 (sem 0·1 × 108) cells; P = 0·01), respectively.
Plasma and mesenteric lymph node phospholipid fatty acid composition
Effect of obesity
Obese rats had higher plasma phospholipid concentrations of all fatty acids compared with lean rats (except 24 : 1n-9) (Table 2). There was no difference in the plasma phospholipid PUFA:SFA ratio between obese and lean rats. Interestingly, obese rats had a lower n-6:n-3 PUFA ratio compared with lean rats. Despite equivalent dietary composition, obese rats had a higher proportion of MLN phospholipid fatty acids as 16 : 0, 16 : 1 (cis-9), 18 : 1 (cis-11), 20 : 4n-6, 20 : 1n-9, 22 : 5n-3, 22 : 6n-3, and a lower proportion as 18 : 1 (cis-9) and 18 : 2n-6 compared with lean rats (Table 3).
Table 2 The effect of dietary vaccenic acid (VA) on plasma phospholipid fatty acid composition in JCR:LA/cp rats
(Mean values with their standard errors of ten rats per group)

NS, P >0·05; ND, not detectable.
a,b,c Mean values within a row with unlike superscript letters were significantly different (P < 0·05; LSMeans).
* Significant main effects (diet and genotype) were determined by two-way ANOVA. There were no significant interactions between diet and genotype.
Table 3 The effect of dietary vaccenic acid (VA) on isolated mesenteric lymph node phospholipid fatty acid composition in JCR:LA/cp rats
(Mean values with their standard errors for ten rats per group)

NS, P>0·05; CLA, conjugated linoleic acid; ND, not detectable.
a,b,c Mean values within a row with unlike superscript letters were significantly different (P < 0·05; LSMeans).
* Significant main effects (diet and genotype) were determined by two-way ANOVA.
Effect of diet
VA-fed rats had a 1237 % and 1200 % higher concentration of VA in plasma and MLN phospholipids compared with rats fed the control diet (Tables 2 and 3). Rats fed VA also had 0·25 % of total fatty acids as CLA in MLN phospholipids, while CLA was not detectable in the MLN of control-fed rats. The VA-fed rats had higher concentrations of 18 : 0 in plasma phospholipids compared with rats fed the control diet.
Effect of vaccenic acid in lean v. obese rats
Obese VA-fed rats had higher concentrations of 16 : 0 in MLN and plasma phospholipids compared with obese control-fed rats, but there was no difference between dietary treatments in the lean rats. Lean VA-fed rats had higher concentrations of 16 : 1 (cis-9), 17 : 0 and 18 : 2n-6 in plasma phospholipids and lower concentrations of 18 : 1 (cis-9) in MLN phospholipids compared with lean control-fed rats, but there was no difference between dietary treatment groups in the obese rats.
Diet×genotype effect
There was no interaction between diet and genotype on plasma and MLN phospholipid fatty acid composition, except for the MLN phospholipid concentration of VA, 22 : 6n-3 and n-6:n-3 ratio. VA was incorporated into MLN phospholipids to a lesser extent in the obese rats fed VA compared with lean rats fed VA. The lean rats fed VA had a higher MLN phospholipid n-6:n-3 ratio compared with lean rats fed the control diet, but there was no difference in the MLN phospholipid n-6:n-3 ratio between the obese rats fed the VA and control diets.
Immune cell phenotypes
Effect of obesity
The proportion of splenic T-cells was 6 % higher in obese rats compared with lean rats due to a relative increase in both the proportion of helper (CD3+CD4+) and cytotoxic (CD3+CD8+) T-cells (Table 4). Unlike the spleen, the proportion of MLN T-cells was 5 % lower in obese rats compared with lean rats due to an 18 % decrease in the proportion of cytotoxic T-cells with no concomitant effect on the proportion of helper T-cells (Table 5). The ratio of helper:cytotoxic cells was 24 % higher in obese compared with lean rats. Moreover, obese rats had a 21 % higher proportion of splenic monocytes/macrophages (CD11b/c+) compared with lean rats, but obesity did not affect the proportion of splenic B-cells (OX-12+) (Table 4). We also observed that obesity in this animal model did not affect the proportion of B-cells (OX-12+), and macrophages (CD11b/c+) in the MLN.
Table 4 Splenic immune cell phenotypes
(Mean values with their standard errors for ten rats per group)

VA, vaccenic acid; NS, P>0·05.
a,b Mean values within a row with unlike superscript letters were significantly different (P < 0·05; LSMeans).
* Significant main effects (diet and genotype) were determined by two-way ANOVA.
Table 5 Mesenteric lymph node immune cell phenotypes
(Mean values with their standard errors for ten rats per group)

VA, vaccenic acid; NS, P>0·05.
a,b Mean values within a row with unlike superscript letters were significantly different (P < 0·05; LSMeans).
* Significant main effects (diet and genotype) were determined by two-way ANOVA. There were no significant interactions between diet and genotype.
Effect of diet
There was no effect of VA on the proportion of T-cells, cytotoxic T-cells, B-cells or macrophages in the spleen and MLN of obese rats (Tables 4 and 5, respectively).
Effect of vaccenic acid in lean v. obese rats
The lean rats fed VA had a 10 % lower proportion of splenic and MLN helper T-cells compared with the lean rats fed the control diet, but there was no difference in the proportion of helper T-cells between the dietary treatments in the obese rats.
Diet×genotype effect
There was no interaction between diet and genotype on immune cell phenotypes.
Immune cell activation markers
Effect of obesity
Obesity did not significantly affect the proportion of splenic helper or cytotoxic T-cells that expressed the co-stimulatory molecule CD28 (CD3+CD4+CD8− CD28+, 12·7 (sem 0·4) %, n 40; CD3+CD4− CD8+CD28+, 11·4 (sem 0·3) %, n 40) or CD152 (CTLA-4; CD4+CD8− CD152+, 0·36 (sem 0·04) %, n 40; CD4− CD8+CD152+, 3·52 (sem 0·17) %, n 40). There was also no difference in the proportion of MLN helper and cytotoxic T-cells that expressed CD28 (CD3+CD4+CD8− CD28+, 9·4 (sem 0·3) %, n 40; CD3+CD4− CD8+CD28+, 5·0 (sem 0·1) %, n 40) or CD4+ and CD8+ that expressed CD152 (CD4+CD8− CD152+, 0·12 (sem 0·01) %, n 40; CD4− CD8+CD152+; 1·17 (sem 0·12) %, n 40) between obese and lean rats. However, splenocytes from obese rats did have a 22 and 24 % higher proportion of splenic CD4+ and CD8+ cells that expressed the IL-2 receptor (CD25+), respectively (Table 4). Similarly, there was an 11 % higher proportion of CD4+ cells expressing CD25 in MLN cells, but unlike the spleen there was no difference in the proportion of CD8+ cells expressing CD25 in obese compared with lean rats (Table 5). Notably, there was no effect of obesity on the intensity of CD4 staining in the spleen, but obese rats had a 132 % higher proportion of CD4lo MLN cells compared with lean rats (Table 5).
Effect of diet
There was no effect of VA on the proportion of CD4+ and CD8+ cells expressing CD25, CD28 or CD152 in both the spleen and MLN. There was also no diet effect on the intensity of the CD4 staining in the MLN cells (Table 5) and splenocytes.
Diet×genotype effect
There was no interaction between diet and genotype on immune cell activation markers.
Immune cell maturation markers
Effect of obesity
There was no effect of obesity on the proportion of splenic T-cells that were new (CD3+CD90+), and helper T-cells that were naive (CD45RC+) (Table 4). However, obese rats had a 7 % lower proportion of MLN T-cells that were new (Table 5) and a 33 % higher proportion of MLN helper T-cells that were naive (CD4+CD45RC+) compared with lean rats.
Effect of diet
VA had no effect on the proportion of splenic and MLN T-cells that were new; however, VA-fed rats had 35–38 % lower proportions of splenic helper T-cells that were naive compared with rats fed the control diet (Table 4).
Effect of vaccenic acid in lean v. obese rats
VA normalised the proportion of MLN helper T-cells that were naive in obese rats to proportions similar to those seen in lean rats (Table 5).
Diet×genotype effect
There was no interaction between diet and genotype on immune cell maturation markers in the MLN or on the proportion of splenic helper T-cells that were naive. There was a significant interaction in the proportion of splenic T-cells that were new; however, no significant differences between means were found by LSMeans.
Ex vivo cytokine production
Effect of obesity
Unstimulated MLN from obese rats produced higher concentrations of IL-10 compared with lean rats (Table 6). Stimulation of splenocytes with the T-cell mitogen ConA resulted in a 43 % higher IL-6 concentration in supernatant fractions from obese compared with lean rats, but there was no difference between groups in the other cytokines measured (Table 6). Despite the lower proportion of MLN T-cells from obese rats, they produced 39–81 % higher concentrations of IL-2, IL-10, IFNγ and TNFα when stimulated with ConA compared with leans (Table 6). Although there was a higher proportion of splenic monocytes/macrophages, there was only a trend (P = 0·0518) towards a higher concentration of TNFα produced with LPS stimulation (Table 6).
Table 6 Cytokine production (pg/ml) by spleen and mesenteric lymph node (MLN) cells stimulated with concanavalin A (ConA) or lipopolysaccharide (LPS) for 48 h
(Mean values with their standard errors for nine or ten rats per group)

VA, vaccenic acid; NS, P>0·05; IFN, interferon.
a,b,c Mean values within a row with unlike superscript letters were significantly different (P < 0·05; LSMeans).
* Significant main effects (diet and genotype) were determined by two-way ANOVA. There were no significant interactions between diet and genotype.
Effect of diet
Splenocytes from VA-fed rats produced 16–23 % lower concentrations of IL-2, IL-10 and TNFα, with no difference in the concentration of IFNγ and IL-6 after ConA stimulation (Table 6). VA had no effect on the concentration of IL-1β, IL-6 and TNFα produced in response to LPS, but there was a 43 % lower concentration of IL-10 produced.
Effect of vaccenic acid in lean v. obese rats
Unstimulated cells from lean rats fed VA produced lower concentrations of IL-10 compared with those from lean rats fed the control diet, but there was no difference between dietary treatments in the obese rats. VA had no effect on cytokine concentrations produced by splenic and MLN cells after mitogenic stimulation from lean rats, except for a lower concentration of IL-10 by splenocytes stimulated with LPS (Table 6). However, obese rats fed VA produced 32 % less TNFα from ConA-stimulated splenocytes compared with obese rats fed the control diet. In addition, obese rats fed VA produced 34–38 % lower concentrations of IL-2 and TNFα (similar to concentrations produced by lean controls) compared with obese rats fed the control diet. Obese rats fed VA also produced 35–136 % higher concentrations of IL-10 and IFNγ compared with the other groups (Table 6).
Diet×genotype effect
There was no interaction between diet and genotype on ex vivo cytokine production.
Discussion
There is increasing evidence that obesity is a chronic inflammatory state with impaired immune responses(Reference Ruth, Taylor and Zahradka12, Reference Falagas and Kompoti13). The JCR:LA-cp rat has been shown to have higher circulating levels of haptoglobin(Reference Wang, Lu and Ruth11) (a systemic marker of inflammation) and we report here that abnormalities in immune function exist (Fig. 1). MLN cells of obese rats produced higher concentrations of IL-2, IL-10, IFNγ and TNFα in response to mitogenic challenge (Table 6), indicating a pro-inflammatory tendency. This observation is consistent with other reports of splenocytes from fa/fa Zucker rats(Reference Ruth, Taylor and Zahradka12) and peripheral blood mononuclear cells from humans with high BMI(Reference Tanaka, Isoda and Ishihara21). Inflammation involves activated T-cells(Reference Hansson, Robertson and Soderberg-Naucler22). An indicator of T-cell activation that has not been measured in other models of obesity is the down-modulation of CD4, indicated by a lower cell surface expression of CD4(Reference Marsh and Pelchen-Matthews23). Obese rats from the present study had a higher proportion of MLN CD4lo cells (Table 5), suggesting a higher proportion of activated cells, which is supported by a higher concentration of IL-10 produced by unstimulated MLN cells relative to lean rats. A higher production of cytokines by stimulated MLN cells (Table 6) is also consistent with a higher proportion of activated T-cells.

Fig. 1 Summary of the effects of obesity (A) and (B) and vaccenic acid (VA) (C) and (D) on immune cell phenotypes and cytokine production in the spleen (A) and (C) and mesenteric lymph nodes (B) and (D) of JCR:LA/cp rats. ConA, concanavalin A; IFN, interferon.
Experiments using fa/fa Zucker rats(Reference Tanaka, Isoda and Yamakawa24) and obese human subjects(Reference Nieman, Henson and Nehlsen-Cannarella25) have indicated a lower proliferative response of lymphocytes when stimulated ex vivo. The present results are not consistent with other rodent models of obesity that have shown lower proportions of CD8+CD25+ splenic cells(Reference Ruth, Taylor and Zahradka12), CD4+CD25+ MLN cells(Reference Kim, Lee and Kim26) and IL-2 production after mitogenic stimulation(Reference Ruth, Taylor and Zahradka12, Reference Mito, Hosoda and Kato27). The higher proportion of MLN helper T-cells that were CD25+ in combination with the higher IL-2 production in response to ConA suggest that MLN cells from the obese JCR:LA-cp rats may have a higher proliferative response compared with lean rats. It is unclear whether the differences among rodent models are due to environmental differences (i. e. housing or diet), genetic differences or experimental conditions (i.e. age), all of which influence immune function. Social isolation of male rats has been shown to be immunosuppressive(Reference Jessop and Bayer28); however, because all the rats in the present study were housed in the same manner, any effects of individual housing on immune function would be the same between dietary treatment groups and between genotypes.
CD45RC expression has been used to identify naive (CD45RC+) v. memory T-cells (CD45RC− )(Reference Bunce and Bell29) as well as T-helper (Th)1 (CD45RC+) v. Th2 (CD45RC− )(Reference Subra, Cautain and Xystrakis30, Reference McKnight, Barclay and Mason31) in rats. Obese JCR:LA-cp rats had a higher proportion of MLN helper T-cells that were CD45RC+ compared with lean rats, while the VA-fed rats had lower proportions of splenic and MLN helper T-cells that were CD45RC+ compared with controls (Table 5); however, the concentrations of cytokines produced were not consistent with a greater proportion of naive or Th1 cells in obese MLN or lower proportion of splenic Th1 cells with dietary VA.
This is the first study to report a lower proportion of new MLN T-cells in an obese animal model (Table 5). It has been hypothesised that, over time, fewer new T-cells would negatively affect the T-cell repertoire, leaving the rat more susceptible to disease(Reference La Gruta, Driel and Gleeson32), which is consistent with the reduced wound healing in the JCR:LA-cp rat(Reference Bauer, Ghahary and Scott33) and the observation that human obesity is associated with an increased risk of infection (for a review, see Falagas & Kompoti(Reference Falagas and Kompoti13)). Previous reports in fa/fa Zucker rats(Reference Ruth, Taylor and Zahradka12, Reference Tanaka, Isoda and Yamakawa24), diet-induced obese mice(Reference Kim, Lee and Kim26) and humans(Reference Tanaka, Isoda and Ishihara21) have all described T-cell lymphopenia in the obese state. Consistent with these studies, the obese JCR:LA-cp rat had a lower proportion of MLN T-cells (Table 5). However, in contrast with these reports, the obese JCR:LA-cp rat had a higher proportion of splenic T-cells (Table 5). This difference in the effect of obesity on the proportion of T-cells between organs could be due to the adipose tissue that surrounds the MLN, but not the spleen, a T-cell homing defect or an altered proportion of an unidentified population of MLN cells in the obese rats (i.e. dendritic cells).
MLN cells from obese rats produced higher concentrations of all cytokines measured (despite a lower proportion of T-cells), while there was no effect of obesity on the concentration of the cytokines produced by splenocytes with the exception of IL-6 in response to ConA (Fig. 1). A possible explanation for the differences in immune function between organs is that the MLN are embedded in visceral fat, while the spleen is not. Visceral fat produces adipokines which have various effects on the immune system (for a review, see Lago et al. (Reference Lago, Dieguez and Gomez-Reino34)). In the diet-induced obese mouse, visceral fat accumulation was linked with lymph node atrophy and lymphocyte apoptosis(Reference Kim, Lee and Kim26). Interestingly, CLA feeding in the fa/fa rat has been shown to alter concentrations of adipokines in both serum and adipose tissue(Reference Noto, Zahradka and Yurkova35). Our group has shown that VA-fed rats have a higher proportion of CLA within TAG isolated from adipose tissue relative to control-fed rats(Reference Wang, Lu and Ruth11). Adipokine concentrations in serum and adipose tissue were not measured in the present study; therefore, it is unknown whether MLN and spleen cells were exposed to different concentrations of adipokines. Although the function of the immune cells was assessed ex vivo without visceral fat, chronic exposure to adipokines in vivo may have permanently ‘programmed’ the immune cells to respond in a particular manner even in the absence of the adipokine signal.
Splenocytes from VA-fed rats produced lower concentrations of IL-10 when unstimulated, ConA-stimulated and LPS-stimulated compared with splenocytes from control-fed rats (Table 6). This is consistent with the lower serum IL-10 concentrations in obese rats fed VA in the study by Wang et al. (Reference Wang, Lu and Ruth11). However, there was no evidence of an increased splenic pro-inflammatory response with VA, indicating that the anti-inflammatory properties of IL-10 were not required to control inflammation. Conversely, stimulated MLN cells from VA-fed rats produced higher concentrations of IL-10 compared with control-fed rats (Table 6). It is likely that the inflammatory signals emanating from the adipose tissue affect immune cells in the resident MLN; therefore it would be beneficial to have more regulatory T-cells producing higher concentrations of IL-10 in the MLN to regulate or control the locally stimulated immune response. In fact, we did observe a normalisation of IL-2 and TNFα production with a higher production of IL-10 in MLN with VA feeding in the obese rats (Table 6). For all the other cytokines measured, there was not an opposite effect of VA in splenocytes compared with MLN; however, there was less of an effect in the splenocytes compared with MLN.
The effect of VA on IL-10 production in the MLN might be mediated by its conversion into CLA (cis-9, trans-11). Previous reports have shown that CLA (cis-9, trans-11) can enhance IL-10 mRNA and protein concentrations produced by stimulated dendritic cells(Reference Loscher, Draper and Leavy36). It is unclear whether CLA would have similar effects in different immune cell phenotypes. MLN contain mainly T-cells while the spleen contains a more even split between T- and B-cells. The differential effects of VA on the concentration of cytokines produced between splenocytes and MLN cells might be accounted for by the differences in the cell types present. To further support this hypothesis, after stimulation with the polyclonal T-cell mitogen, ConA, splenocytes from rats fed VA produced lower concentrations of IL-2 and TNFα compared with control-fed rats, but no difference in IL-2 or TNFα production was detected when splenocytes were stimulated with the B-cell/macrophage/dendritic cell mitogen LPS (Table 6). This suggests that feeding a diet enriched in VA may have altered T-cell function to a greater extent than that of antigen-presenting cells. There was no effect of diet on the proportion of splenic macrophages or B-cells; however, we did not measure the proportion of dendritic cells in this tissue.
In recent years, trans-fats have garnered interest due to concerns that dietary trans-fat increases the risk of CHD by favouring a pro-inflammatory state (for a review, see Mozaffarian(Reference Mozaffarian37)). However, it stands to reason that individual trans-fatty acids may have different immunological effects. Plasma markers of inflammation were positively related to trans-oleic acid (mainly elaidic acid)(Reference Lopez-Garcia, Schulze and Meigs38), while our group has reported that dietary VA did not increase systemic markers of inflammation (serum haptoglobin and IL-6 concentrations) in obese or lean rodents(Reference Wang, Lu and Ruth11). The present report is the first to examine the effects of VA on MLN and spleen cell immune function in the obese/insulin-resistant JCR:LA-cp rat. While other reports have shown hydrogenated fat increased peripheral blood mononuclear cell inflammatory cytokine production(Reference Han, Leka and Lichtenstein39), we report that feeding 1·5 % (w/w) VA did not increase the inflammatory response of splenic and MLN cells in both healthy rodents and those with the metabolic syndrome, but favourably altered the pro-inflammatory tendency of MLN cells in obese JCR:LA-cp rats.
Previous studies have shown that dietary CLA (cis-9, trans-11) has immunomodulatory effects in disease states, with no alteration of immune function in the healthy state(Reference Ruth, Taylor and Zahradka12, Reference Turpeinen, Ylönen and von Willebrand40). Therefore, we were not expecting to see an interaction between diet and genotype. Dietary VA did not alter the proportion of any of the immune cell phenotypes measured in lean rats, except for a lower proportion of helper T-cells. This decrease in helper T-cell proportions in VA-fed rats was associated with lower concentrations of cytokines produced after mitogenic stimulation; however, the physiological implications of these small changes are probably minor. Dietary VA did not normalise any of the altered immune cell phenotypes (including activation markers) in obese rats to levels similar to those seen in lean rats with the exception of the proportion of CD45RC+CD4+ MLN cells (Fig. 1). Therefore, the lower cytokine production by VA-fed rats after mitogenic stimulation (Table 6) cannot be explained by the proportion of activated cells in immune organs.
PPAR-α and PPAR-γ are expressed by lymphocytes (for a review, see Glass & Ogawa(Reference Glass and Ogawa41)). PPAR agonists, such as CLA (cis-9, trans-11), have been shown to inhibit inflammatory cytokine production(Reference Marx, Kehrle and Kohlhammer42, Reference Yu, Correll and Vanden Heuvel43); therefore, the lower inflammatory cytokine production with VA feeding may be due to its conversion to CLA (cis-9, trans-11). CLA (cis-9, trans-11) has shown little or non-significant effects on immune function in human studies(Reference Mullen, Moloney and Nugent44–Reference Burdge, Tricon and Morgan46). However, it is important to note that most of the trials have been done in healthy adults where we would expect to see very little effects on immune function. We have shown that VA can have different effects on the systemic immune response compared with the mesenteric immune response (Fig. 1). The human trials were not able to access the MLN where we observed the ‘normalisation’ of cytokine production.
The VA diets contained 9 % of total fatty acids as VA and 0 % CLA (Table 1). It is generally accepted that plasma phospholipid fatty acid composition reflects dietary intake. Therefore, it is interesting to note that despite a similar dietary content of 16 : 0, 18 : 2n-6 and 18 : 0 between the VA diet and control diet, VA-fed rats had higher concentrations of these fatty acids in plasma phospholipids compared with control-fed rats (Table 2). The plasma phospholipid concentration of VA in the lean VA-fed rats was similar to the dietary composition; however, the obese VA-fed rats had almost twice as much VA in plasma phospholipids compared with lean VA-fed rats (Table 2). This higher concentration of VA in plasma phospholipids in the obese state might be due to impaired incorporation of VA into tissues as evidenced by the lower concentration of VA in MLN phospholipids in the obese rats compared with lean VA-fed rats (Table 3). This lower concentration of VA in obese v. lean VA-fed rats is consistent with our report on VA proportions in adipose tissue TAG(Reference Wang, Lu and Ruth11). Similar to plasma phospholipids, the proportion of MLN phospholipid as 16 : 0 was also higher in obese compared with lean rats and with the VA compared with the control diets, which is consistent with a report showing a higher proportion of 16 : 0 in splenocyte phospholipids in Zucker rats fed CLA-containing diets(Reference Ruth, Taylor and Zahradka12).
VA-fed rats had 0·7–1·9 % of total MLN phospholipids as VA and 0·3 % CLA (cis-9, trans-11). Although the MLN phospholipid concentration of CLA (cis-9, trans-11) is small in the VA-fed rats, it is similar to the proportion of CLA (cis-9, trans-11) in splenocyte phospholipids in the study by Ruth et al. (Reference Ruth, Taylor and Zahradka12) who fed Zucker rats diets containing 5·3 % of total fatty acids as CLA (cis-9, trans-11). In that study, the cis-9, trans-11 isomer of CLA had beneficial effects on the proliferative and immunoregulatory response of T-cells in the Zucker rat(Reference Ruth, Taylor and Zahradka12). In the present study where we fed VA without CLA (cis-9, trans-11), the effects we observed suggest that some of the beneficial effects of VA on immune function may be mediated by its conversion into CLA. This is the first study to report the effect of VA on MLN phospholipid fatty acid concentrations and there are currently no published reports on the effect of dietary CLA (cis-9, trans-11) on MLN phospholipid concentrations. In vitro studies have shown that other dietary fatty acids, in particular EPA, can interfere with the conversion of VA into CLA (cis-9, trans-11)(Reference Renaville, Mullen and Moloney47); however, our diets were designed to contain a balanced polyunsaturated:saturated ratio, not high in PUFA, with no EPA. Overall, we achieved a higher proportion of VA and CLA (cis-9, trans-11) in MLN phospholipids (Table 3) compared with the proportion of VA (0·45 (sem 0·06)) and CLA (cis-9, trans-11) (0·16 (sem 0·03)) in mononuclear cell lipids from healthy humans consuming a dietary concentration of VA similar to the one used in the present study (about 8 % of dietary fat) for 42 d(Reference Kuhnt, Kraft and Vogelsang14). Both cell-culture studies(Reference Reynolds, Loscher and Moloney48) and animal feeding trials(Reference Lock, Corl and Barbano49) have shown that VA is converted into CLA (cis-9, trans-11); however, the anti-inflammatory and anti-cancer effects were specific to CLA (cis-9, trans-11). With the present study design, we are not able to separate out the effects of VA from CLA (cis-9, trans-11) on immune function.
In summary, the obese JCR:LA-cp rat, similar to other models of obesity, demonstrates a pro-inflammatory tendency when stimulated ex vivo. The present study is the first to report that unlike other industrially produced trans-fats, the natural trans-fat VA does not promote an inflammatory response by MLN and splenic cells in lean or obese JCR:LA-cp rats. Although the effects of dietary VA on immune cell phenotypes and function were small with few significant diet and genotype interactions, VA appears to favourably alter the pro-inflammatory tendency of MLN cells from obese JCR:LA-cp rats. The effects of VA on immune function in vivo on immunologically relevant markers of disease (i.e. arterial plaque formation) should be examined in future studies. In North America, CLA but not VA is excluded from the total trans content on packaged foods(Reference Moss50, 51), as there is insufficient evidence to conclude that VA does not negatively affect health. The present report provides evidence in an animal model that dietary VA at 3 % of energy may provide some health benefits.
Acknowledgements
The present study was supported by operating grants from the Beef Information Centre to C. J. F. and S. D. P. and the Alberta Agriculture Funding Consortium to the Alberta CLA Network to C. J. F.
We wish to thank Susan Goruk, Kristina MacNaughton and Tara Martin for their technical assistance, and Sharon Sokolik for animal care.
H. J. B. analysed data derived from the various experimental procedures, performed statistical analysis, wrote the manuscript and received feedback from the co-authors. C. A. G. prepared experimental diets, and performed and coordinated all laboratory analysis. M. R. R., S. D. P. and C. J. F. provided intellectual guidance at all stages.
There are no conflicts of interest to declare.