Introduction
Problems with muddy pens and keeping cattle clean has been identified as one of the three most serious animal welfare issues related to outdoor feedlot beef production (Grandin, Reference Grandin2016). Precipitation and moisture excreted by the animals themselves can result in a wet substrate under foot, composed of manure and, depending on the pad base, may include eroded soil base.
A detrimental effect of muddy conditions on the growth and efficiency of cattle is well documented (Morrison et al., Reference Morrison, Givens, Garrett and Bond1970). From a survey of Australian feedlots, O'Keefe et al. (Reference O'Keefe, Mcdonald, Tucker and Watts2013) rated screened or graded woodchip as the most suitable bedding for alleviating muddy conditions in feedlots due to its durability, porosity, ease of handling and availability. Woodchips have a high capacity for water absorbance: spruce, for example, is able to absorb water to reach 690 g moisture/kg after 48 h soaking, without reaching an equilibrium (Kumar and Flynn, Reference Kumar and Flynn2006). The water holding capacity of various bedding types at room temperature, demonstrated sawdust and peat moss held three and two times more water than woodchip, respectively, whereas straw held half as much water as woodchip (Airaksinen, et al., Reference Airaksinen, Heinonen-Tanski and Heiskanen2001). Woodchip pads are commonly used for winter bedding of cattle intensively housed outdoors on ‘out-wintering’ or ‘stand-off’ pads in the UK and New Zealand (Smith et al., Reference Smith, Chadwick, Dumont, Grylls and Sagoo2010). These are generally engineered as a soil or concrete pad or metal grate with drainage systems installed at the base; whereas Australian feedlots are usually on gravel/clay base (Davis and Stafford, Reference Davis, Stafford, Watts, Davis, Keane, Luttrell, Tucker, Stafford and Janke2016)). Much of the research on bedding for outdoor cattle systems has thus compared woodchip-bedded outdoor pads with concrete or slatted pads, or with indoor housing (O'Driscoll et al., Reference O'Driscoll, Hanlon, French and Boyle2009), or under freezing conditions, and has focused on animal performance rather than pad condition. There is relatively little work examining the use of woodchip bedding for lot-fed beef cattle under conditions of cold, wet, but non-freezing conditions on soil underbases, rather than concrete or slatted pens. Furthermore, there is no research on the most effective application rate of woodchip bedding to manage the condition of the feedlot pad.
The use of woodchip bedding not only affects the conditions inside the feedlot pen, but also affects the weight and composition of manure removed from the pen at pen cleaning, with implications for its value for composting and the potential to recover woodchips for reuse. Where volatile solids (VS, i.e. the organic solids lost on ignition) are low this can be due to manure mixing with pen clay base or prolonged manure breakdown (Watts and McCabe, Reference Watts and Mccabe2015). This research thus sought to understand how different depths of woodchip bedding might affect the characteristics of the manure pad that affect cattle comfort, recovery potential of the pad substrate and its quality for composting. The experiment compared clay and gravel-based pens without bedding as a control, with two depths of woodchip bedding for responses in the pad, such as penetrable depth, moisture and force required to pull an animal leg analogue out of the substrate; bulk density, weight of substrate removed at the end of the feeding period; VS, carbon (C) and nitrogen (N) composition.
Methodology
Animals and housing
To test the effect of woodchip bedding at two different applications rates, a randomized block experiment was designed with three treatments, each with ten replicate pens of ten cattle, where the pen was the experimental unit. The study was conducted over a 109-day experimental period during winter (May–September 2018) at the University of New England's Tullimba Feedlot (30°28′49″S, 151°11′20″E, elevation 710 m), located in a summer rainfall area, with a mean annual rainfall of 767 mm. All procedures were approved by the University Animal Ethics Committee (AEC18-038).
Three hundred Bos taurus steers of various breeds, 12–18 months of age, were stratified by breed type (European/British), then weight strata, breed and weight, before allocation to ten blocks. At the start of the experiment, the cattle were individually weighed and allocated randomly to one of the three treatments. Each pen of ten cattle was housed in contiguous outdoor (no shade or shelter) feedlot pens of identical dimensions (6.25 m × 20 m; slope 3° west to east (from front of pen to back), and 1° north to south, along the row of pens; one fence line water trough shared between two pens = 3.0 × 0.7 m2), built on a clay interface. The pens were stocked at a density of 12.5 m2/head. If cattle needed to be removed from the experiment after the first week of the experiment, they were not replaced, and instead the area of the experimental pen was reduced by cutting out an area equivalent of ~12.5 m2 with cattle panels, so as to maintain the stocking density within the pen. Each pen had a concrete apron 3 m deep from the back of the feed bunk. The remainder of the pen surface was a manure interface above a compacted soil base of clay and natural rock. Each pen housed ten steers and was cleaned to the interface layer before the start of the experiment.
The weather conditions (air temperature, relative humidity, rainfall, solar radiation, wind speed and calculated evapotranspiration using Penman–Monteith equation) were automatically measured and logged hourly with an ICT Weather Hub (ICT International Pty Ltd, Armidale, NSW, Australia).
Treatments
The three experimental treatments were (1) no bedding (control), (2) woodchip bedding provided at approximately 150 mm depth (W15) and (3) woodchip bedding provided at approximately 300 mm depth (W30). The bedding was Eucalypt (hardwood) woodchip of average dimensions approximately 50 × 30 × 5 mm3 with mean initial moisture content of 507 g/kg. At a mean density of 480 kg/m3, this gave woodchip application rates of approximately 54 kg/m2 in W15, and 108 kg/m2 in W30.
The bedding was distributed over the entire pen surface, but not on the concrete apron in front of the feed bunk (an area 3.0 × 6.5 m2). To prevent woodchip spreading into adjacent pens, 400 mm rubber belting was installed along the sides of each pen. The back of each pen was uncontained, and free to drain out of the pen area.
Being a summer rainfall district, there was naturally low rainfall during the experimental period so a cold wet winter environment was simulated by irrigating the experimental pens. An irrigation system (Hunter MP3000, Hunter Industries®, San Marco, CA, USA) was installed with rotator sprinklers mounted on the pen dividing rails which provided overlapping coverage over the entire pen surface, the cattle in the pen and the feed bunks. The irrigation distribution was validated using 14 sample pans evenly distributed over the surface of each pen during an irrigation test, and showed that the mean application rate was 5.4 mm/h (±0.13 mm/h s.e.m.). In the absence of natural rainfall, irrigation was applied up to four times per week in 4 mm events, commencing 2 h after morning feeding. Total target precipitation (natural rainfall + simulated) was set at 74 mm of rainfall per 30 days, which was the 66.6th percentile of long-term monthly rainfall records for the nearest weather station (Bureau of Meteorology, 2017). Irrigation was reduced to account for natural rainfall events (Fig. 1), so that the target precipitation total was maintained (mean running 30-day average 76.3 ± 8.4 mm). The weather during the experiment was cold and mostly dry, with few days above 20°C maximum until the end of the experiment, and 65 days of minimum <1°C (Fig. 1).

Fig. 1. Average daily maximum (solid line) and minimum (dashed line) temperature (°C), total precipitation per week (rainfall (solid bars) and irrigation (hashed bars), mm) on pens and total evapotranspiration per week (open squares, mm). Note: Week 16 is 4 days.
Pen measures
Measures of pad moisture during feeding period
Samples from the pad were collected weekly, commencing 12 days after the cattle entered the experimental pens (once manure had begun to accumulate) and continuing until the day after the end of the feeding period. The collection day was fixed in relation to a 7-day sampling week, but varied in relation to the watering schedule. The pens were divided into equidistant front, middle and back sections, excluding within 1 m of the water trough and the concrete apron next to the feed bunks, and one sample was randomly taken from each section and bulked together for each pen.
The substrate was not homogenous in the woodchip treatments, and the structure of the woodchip layer caused difficulties in accurately estimating the full depth of the pen substrate with a straight-edged cleft in the pad. As a result, separate ‘surface’ and ‘deep’ pad samples were taken at each sample site, using a plumber's shovel. The ‘surface’ and ‘deep’ stratum were distinguishable from each other visually by the lack of manure mixed with the woodchip in the deep stratum. This reflected the animals' experience, in that their feet and legs tended to penetrate the surface stratum (which was a mix of woodchip and manure), but not into the deep stratum of woodchip. For sampling, the surface sample site was defined as 0–60 mm depth, and the deep sample was defined as from 60 mm depth to the interface layer. This definition was maintained for the duration of the experiment, despite the fact that towards the end of the experiment, the substrate in W15 became more homogenous. The three samples from each stratum in each pen were bulked for the pen-stratum, mixed thoroughly in a bucket with a hand trowel, and subsampled for a single ‘surface’ and a single ‘deep’ sub sample for each pen. The substrate in the control pens was homogenous, therefore at each site a single sample was taken from the substrate surface to the interface layer, bulked and subsampled for each pen. Subsamples were taken by random samplings from the bulked sample and sealing in a plastic bag. The subsamples were immediately transported to the lab, and gravimetric water content determined by oven drying until weights were stable. The samples collected from the control treatment pens in weeks 6 and 7 were discarded due to human sampling error.
Visual scores of apparent depth of pad substrate during feeding period
The apparent depth of the pad in each pen was scored visually using a 1–7 score, using the front legs of cattle as a relative measure (Table 1) between one and four times weekly by one scorer. One animal located in each sector of the pen (front, middle and back) was selected for scoring. The animal selected as the relative measure was the nearest, visible, standing animal in each sector, and depended on the visibility of animals among the group and varied from week to week. The ability to score in each sector of the pen was dependent on the location and body position of cattle in each sector at the time of scoring (e.g. standing or lying, orientation to the scorer), and so not every sector of every pen was able to be scored on every scoring day.
Table 1. Visual scoring system for the apparent depth of substrate in each pen in relation to the front legs of cattle

Sampling of pad substrate at end of feeding period
All pens were sampled using the weekly sampling procedure 24-h after the end of the feeding period. The sample was split into three subsamples. One subsample was analysed for moisture content. A second subsample was refrigerated at 4°C in a sealed package then tested for bulk density. Due to the large particle size of the woodchips, bulk density could not be sampled in situ so the maximum bulk density was measured using a standard Proctor Hammer method (AS 1289.5.2.1). Subsamples were compacted on an as-received moisture basis, as this is what the cattle would be experiencing in the pen, then dried at 105°C until no change in weight. A further 30 ml subsample was stored in a screw-top container for VS, C and N content. These samples were immediately placed on ice, and frozen at −4°C at the feedlot. They were later transported frozen to the laboratory and freeze dried at −50°C for 7 days in an Alpha1-4 LDplus freeze drier (Martin Christ Freeze Dryers, Osterode, Germany). As the woodchips were thick and hard, the samples were first ground to pass through a 5 mm sieve, then a cotton mill grinder with a 1 mm sieve. Samples were split until a homogenous sample was obtained, which was analysed for VS, C and N content (detailed below).
At the end of the feeding period the penetrable depth of the pen substrate was measured by insertion of a 2.5 mm steel spike to the interface at five points across a transect in each pen. At each of the transect points, total depth from the surface to interface layer was measured after excavating to the clay/rock interface layer using a plumber's shovel. Sampling proportion of the surface was calculated for W15 and W30 as the surface depth (60 mm) divided by the total depth of the substrate. The sampling proportion of each stratum in the woodchip-bedded treatments was used to upscale mean moisture, VS content and bulk density for the woodchip substrate into a whole pen basis.
Analysis of substrate composition at end of feeding period
A clean oven-dried crucible was placed in a 550 °C muffle furnace for 15 min, cooled in a desiccator, weighed. Then 2 g of substrate sample was loaded in crucible, oven-dried at 105 °C for 2 h, cooled in a desiccator and re-weighed, until the change was <4 % difference in weight. The sample was then placed in the muffle furnace where temperatures were raised to 550 °C for 1.5 h according to standard methods (APHA 2540E, 1999) facilitating complete combustion of the sample. The sample was removed and cooled in desiccator and weighed. Samples were performed in duplicate and mean values are presented. To check for any loss of VS between the end of the feeding period and pen cleanout, grab samples were collected from bulked manure samples cleaned from each treatment on the last day of pen cleaning, and analysed for VS using the same procedure. A sample of clean woodchip bedding was also analysed for VS.
For C and N, approximately 0.15–0.20 g of ground sample was weighed to four decimal places and analysed for C and N content via combustion in a TruMac determinator (LECO Corporation, St Joseph, MI, USA). The relative depth of each stratum in the woodchip-bedded treatments was used to calculate a mean C : N ratio for the woodchip substrate as a whole, in each pen.
Leg analogue measures of work requirement for cattle to walk through pen substrate
Five pens from the ten replicate pens were randomly chosen to perform the leg analogue experiment 24 h after the end of the feeding period. This experiment quantified the suction force that resulted from the removal of a bovine leg analogue that had previously been pressed into the pad substrate. A leg analogue made from cylindrical eucalyptus timber, with an end-surface area similar to a cattle hoof (80 cm2), was mounted (via metal brackets) to a universal tension-compression load cell (LPCH 10 000 kg, PT Ltd, Baulkham Hills, NSW, Australia; Fig. 2). A data logger (TMR-200 multi recorder, Tokyo Measuring Instruments, Tokyo, Japan) recorded force at 10 Hz frequency. Hanging from the hydraulic arm of a tractor, the leg analogue was lowered slowly until the force reading reached 400 kg of force, indicating the maximum penetration of an animal into the substrate. The leg analogue was then withdrawn using the tractor hydraulics and the peak suction force was recorded (Riskowski and DeShazer, Reference O'Keefe, Mcdonald, Tucker and Watts1976). Five replicate measures of the withdrawal force were recorded in random locations within each of the five pens from each bedding treatment.

Fig. 2. Schematic diagram of leg analogue experiment in pen during testing.
Weight of substrate removed
A front-end loader was used to remove the pen substrate after the end of the feeding period and once the moisture content had reduced sufficiently to allow handling. The fresh weight of substrate removed from each pen was recorded over a weighbridge. A representative sample was collected from each load of substrate removed, mixed and subsampled, and analysed for moisture content to allow the manure to be presented as a dry weight basis to take into account time when manure was removed. Where cattle had been removed from pens during the experiment, dry weight of substrate removed was adjusted for stocking rate (per head) × experimental days, so that dry weight removed was reported on a calculated kg/head/day basis.
Statistical analysis
Samples of the pad collected weekly for moisture content were considered separately as a single sample for the control pens, and separate surface and deep substrate samples for W15 and W30 pens (sample type). Median visual scores of apparent depth of pad substrate were calculated for the front, middle and back sector of each pen for each week. Weekly measures of visual pad depth scores were analysed in a generalized linear model with an ordinal distribution and logistic link function, and reported with the degrees of freedom, sample N, value of χ2 and P value. Repeated measures of moisture and bulk density was analysed as a linear mixed effects model (lme4; Bates et al., Reference Bates, Mächler, Bolker and Walker2014), with sample type and week and their interaction considered as fixed effects, and block as a random effect and pen as a random effect in a first-order autoregressive temporal correlation structure.
End of feeding period measures of substrate depth and penetrable depth, moisture, bulk density, VS, C, N, and weight and volume of substrate cleaned from the pen were analysed in a linear mixed model with treatment as a fixed effect and block as a random effect for least squared means. A linear model was used to test for difference in VS, and hence loss of substrate mass, between the end of the feeding period and the end of the cleaning period, separately for each treatment.
Suction force measures were analysed using linear mixed effects models (R package: lme; Bates and Watts, Reference Bates and Watts1988) with substrate treatments denoted as main effects and pen assigned to random effects to uphold assumptions of independence of pseudo-replicates. A variance structure was also used to normalize residuals (Zuur et al., Reference Zuur, Ieno, Walker, Saveliev and Smith2009).
Results
Visual score of pen substrate depth
There was no difference between pad scores of animals at the front, middle or back areas of a pen (P = 0.286) therefore median pad scores across the pen were compared between treatment and over time. The pad became increasingly wet and deep over the course of the experiment (Fig. 3). There was an interaction between treatment and week (χ2(28,2461) = 70.2, P < 0.001). Pad scores of control pens were higher from week 2 compared to W15 and W30 animals (Fig. 4). Individual pen pad scores reached 6 and 7 in the control pens, equivalent to approximately 600 mm of substrate.
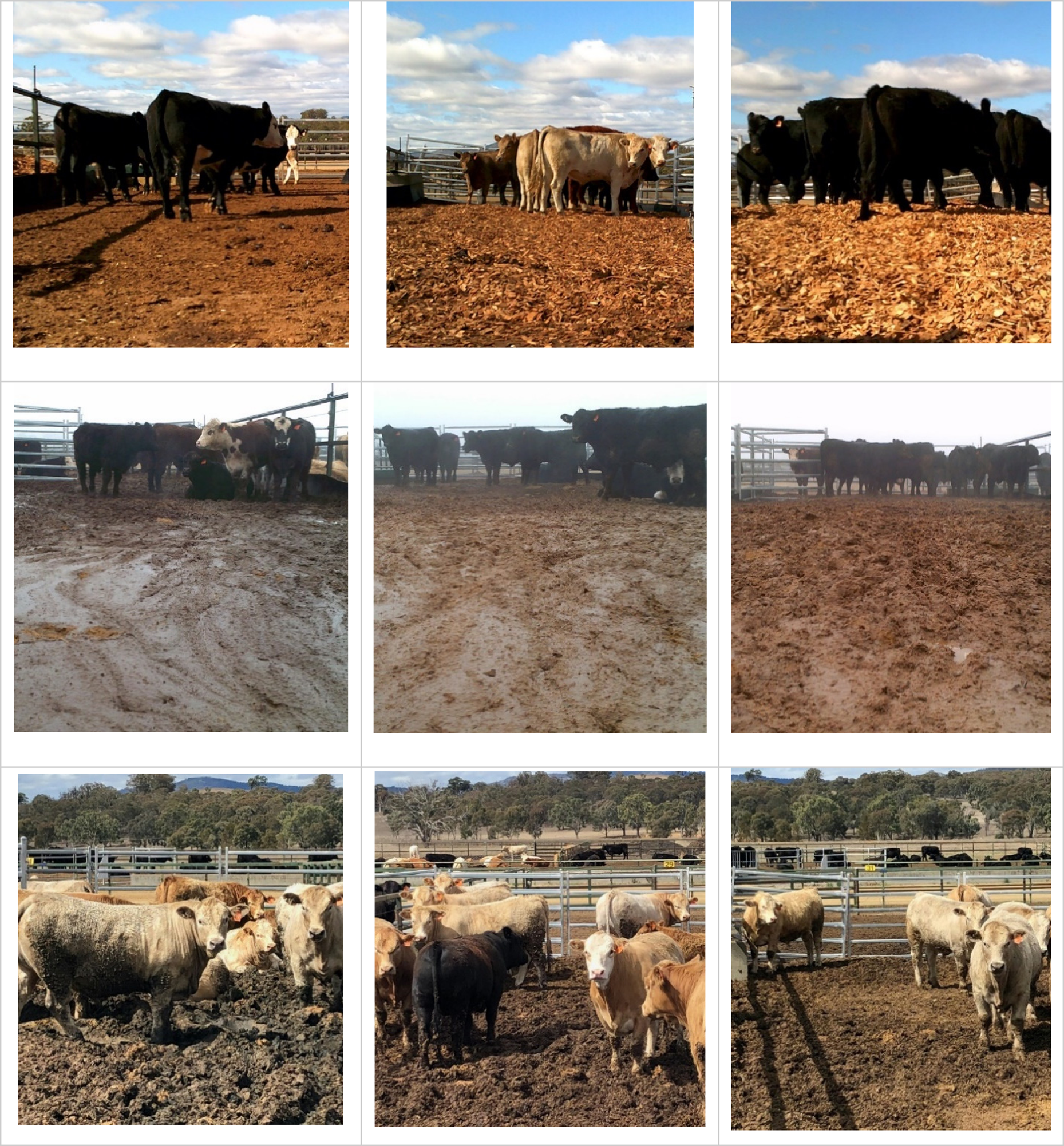
Fig. 3. Condition of the pad, taken from the feed bunk (from left to right, control, W15, W30) on days 3, 29 and 97 (top to bottom).

Fig. 4. Weekly mean visual score of pad substrate depth (mean ± S.D.) for unbedded pens (control, pale grey bars) and pens with woodchip bedding provided at 150mm (W15, medium grey bars) or 300mm depth (W30, dark grey bars). Differing letters denote a significant difference (P < 0.050) between treatment groups at each time point.
By experiment end, woodchip was well mixed with manure throughout the substrate in the W15 treatment, whereas the W30 treatment maintained a clean deep woodchip layer. This is reflected in significantly higher pen pad scores in W15 compared to W30 from week 10, and especially from week 13 onwards (Fig. 4).
Moisture content of pad substrate
Overall, the moisture content of the substrate in the control pens (654 ± 69 g/kg) was significantly (P < 0.001) higher than the surface or the deep samples of the woodchip-bedded pens (559 ± 90 g/kg (W15 surface), 537 ± 87 g/kg (W30 surface), 525 ± 90 g/kg (W15 deep) and 498 ± 81 g/kg (W30 deep)), for the entirety of the experimental period. The W30 deep samples were significantly drier than the W15 surface samples (P < 0.001). There was a significant interaction of sample type and week, whereby the control pens maintained a high and consistent moisture for the majority of the experiment, whereas the moisture content of the woodchip-bedded pens' substrate was initially low and increased gradually over the first 6 weeks of the experiment. In the last 2 weeks of the experiment, warmer temperatures, high winds and therefore higher evapotranspiration reduced the moisture content of the pen substrate (Fig. 5).
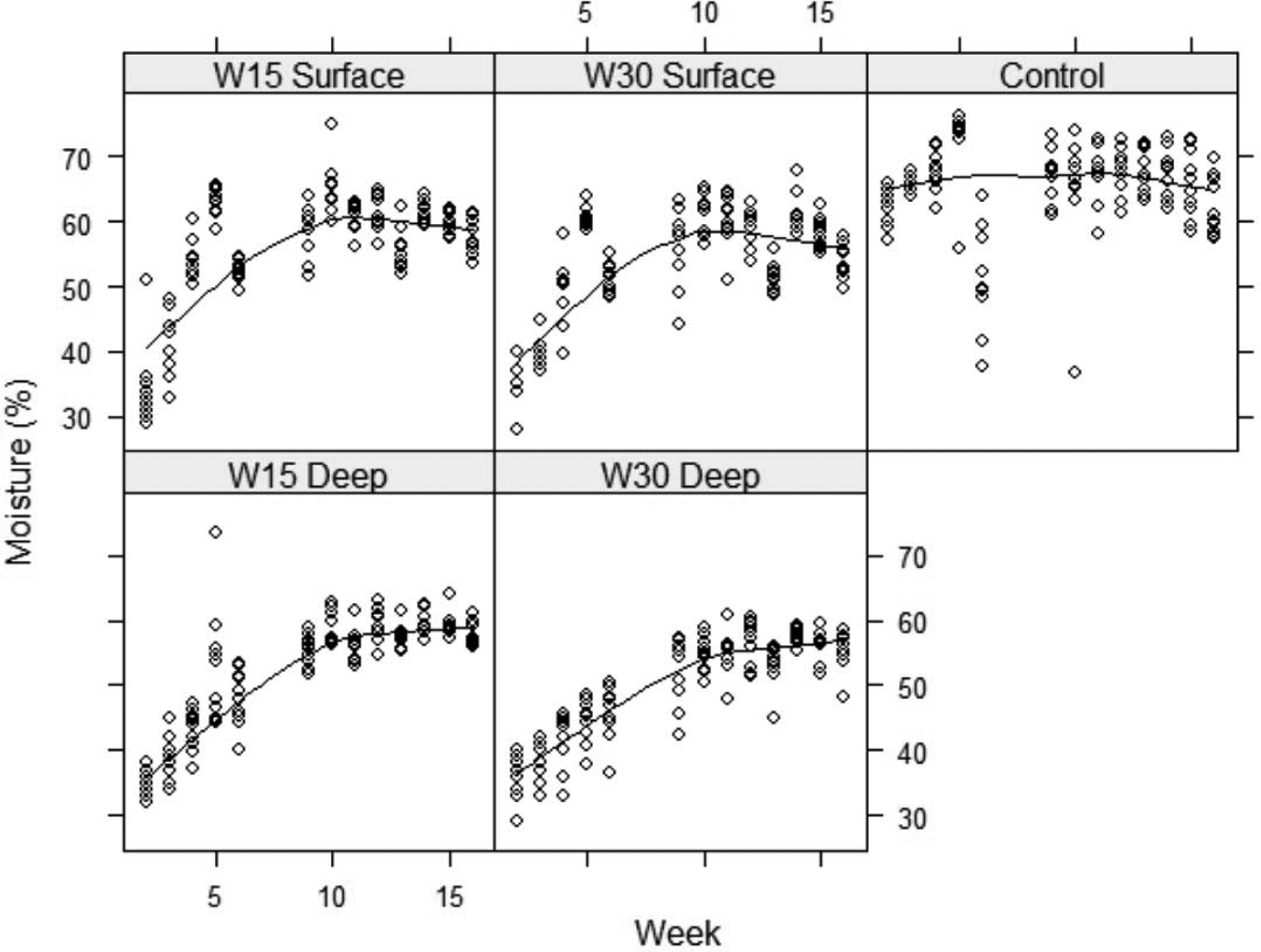
Fig. 5. Pen substrate moisture over the duration of the experiment. Line is smoothed mean, points are samples. A single moisture content sample was taken for the control pens, since the substrate was homogenous. The W15 and W30 pens were bedded with 150 and 300 mm woodchips respectively, with a distinct layering between the surface and deep stratum.
Characteristics of pad substrate at feeding period end
At the end of the feeding period there was no significant difference in bulk density between treatments for the surface to interface, and for the surface layer woodchip-bedded treatments for W15 and W30. However, there was difference between W15 and W30 for the deep layer (P < 0.001, Table 2). Measured depth from surface to interface showed a main effect of treatment such that W30 had the deepest substrate and W15 was shallowest, with the control intermediate (P < 0.001, Table 2). In contrast, penetrable depth (which was the depth the animal interacted with) showed a main effect of treatment, such that W30 was shallowest and the control the deepest, with W15 intermediate (P < 0.001, Table 2).
Table 2. Weight and characteristics (mean ± s.d.) of pen substrate at the end of the feeding periodd for unbedded pens (control) and pens with woodchip bedding provided at 150 mm (W15) or 300 mm depth (W30)

a,b,c Denotes significantly different means between all samples for each test.
d Sampled 24 h after cattle left the pen.
e There were difficulties in penetrating the full depth of the substrate to the interface through the deep woodchip layer in the W30 treatment using a spear, and so depth of substrate was measured by excavating to the interface with a plumber's shovel and using a metal ruler to measure depth.
f For control – as sampled; for W15 and W30 – calculated from ‘surface’ and ‘deep’ samples, according to sampling proportion.
g Substrate removed was sampled for moisture content, and dry-matter basis weight was adjusted for head × days in pens where cattle were removed during the experiment.
The VS content of the clean woodchip was 999 ± 0.7 g/kg (μ ± s.d.). The VS content of the substrate sampled from the pens at the end of the feeding period was significantly lower for the control (P < 0.001), and showed that clay material remained in the crucible. There was no significant change in VS between the end of the feeding period and the end of the cleaning period (P = 0.628 (control), 0.187 (W15), and 0.987 (W30), data not shown). The control pen substrate had a substantially lower C : N ratio than the woodchip-bedded pens, as a result of the high carbon content of the woodchip bedding (Table 3). The C : N ratio of the woodchip-bedded pens aligned with visual observations of the pen substrate at the end of the feeding period, with the strata in the W15 pens becoming mixed, and the W30 pens maintaining differentiation between the manure stratum and the clean woodchip stratum. The carbon content of the control pens had a higher variance than that of the woodchip-bedded pens. However, there was no relationship between ash content at end of the feeding period, and scores of pen damage (r = −0.02, P (two-tailed) = 0.900, data not shown).
Table 3. Carbon and nitrogen content (mean g/kg, DM-basis ± s.d.) and ratio (mean ± s.d.) of the pen substrate sampled after 109 days of feeding with woodchip bedding provided at 150 mm (W15) or 300 mm depth (W30) or no bedding (control)

The control pens had a homogenous substrate from which a single bulked sample was analysed. The W15 and W30 pens had distinct layers of manure and woodchip, and these strata were sampled and analysed separately.
a,b,c Denotes significantly different means between all samples for each test.
Leg analogue
Application of woodchip significantly (P < 0.001) reduced the force required to lift the leg analogue out of the pen substrate at the end of the feeding period (Table 2).
Discussion
Pen conditions relevant to animal experience during the feeding period
The stratification of the woodchip bedding in this experiment into a surface stratum principally composed of manure, and a deep stratum principally composed of woodchip reflected the observations of Watts et al. (Reference Watts, Yan, Sullivan, Luttrell, Davis and Keane2015). This stratification, and the penetrable depth of the pen substrate at the end of the feeding period, reflects the experience of the cattle, in that in control pens, the substrate was homogenous and the cattle sank deep into the manure to the interface layer, whereas in the woodchip-bedded pens, the cattle stood on a ‘solid’ sub-layer of woodchip. Larney et al. (Reference Larney, Olson, Miller, Demaere, Zvomuya and Mcallister2008) also found that woodchip bedding resulted in a drier surface, due to the ability of water to ‘infiltrate the bedding pack’, resulting in fewer applications of woodchip required in a 6–7 month feeding period compared to straw. In the present study, the results of penetrable depth aligned with the visual pad substrate depth scores. There were observed areas of the pen in all treatments that were particularly wet: this may be attributed to the pooling of liquid after rainfall or irrigation events or where the cattle were favouring places to sleep and therefore creating hollow areas for the liquid to pool. This was more pronounced in the control pens.
Visual observations showed the woodchip in the deep stratum was clean of manure, but there was an interaction of treatment and time. The cattle hooves did not penetrate the woodchip substrate in W30, which had a defined surface and deep layer, but in W15, towards the end of the experiment, there was visual evidence of ‘churning’ of the manure and woodchip strata with the woodchip being coated in manure, evidenced by N content being significantly higher in the deep W15 than deep W30 samples; and the W15 surface and deep samples having similar N content. This aligns with increasing visual pad substrate depth scores in W15 from week 10 onwards, and a penetrable depth equal to the substrate depth at the end of the feeding period. Decreased VS in control pens may be associated with degradation of the interface, and mixing of soil with the manure substrate in those pens, as also found by Watts and McCabe (2015).
Differentiation of the strata was considered important, particularly in relation to moisture and bulk density, as this reflected the condition of the area of the pad substrate where the cattle interacted. In the present experiment, the surface stratum was almost always wetter than the deep stratum. For both woodchip-bedded treatments the moisture increased over the first 4 weeks of the experiment, then the surface moisture fluctuated around 550–600 g/kg. The moisture content of the deep stratum of the woodchip-bedded substrate was on average 5–10 % lower than the surface stratum in both bedded treatments. The case studies of Watts et al. (Reference Watts, Yan, Sullivan, Luttrell, Davis and Keane2015) reported that moisture passed through the surface layer of woodchips, and drained in the deep stratum. As a result, the surface of the woodchip in the observations of Watts et al. (Reference Watts, Yan, Sullivan, Luttrell, Davis and Keane2015) was much drier than the underlying woodchip stratum. The reason for the higher moisture of the surface layer in the present experiment is likely to be a result of the consistent application of irrigation in addition to excreted moisture from the cattle, whereas the case studies of Watts et al. (Reference Watts, Yan, Sullivan, Luttrell, Davis and Keane2015) were conducted under much drier conditions. The substrate sampled on day 35 showed a temporary decline in the surface moisture of the woodchip-bedded treatment pens and the control pens and this most likely reflects time since the last irrigation event, and increasing evapotranspiration potential from solar radiation and wind velocity. In this experiment, precipitation (natural + artificial) was equivalent to 2.46 mm/day. By the end of the feeding period, the cattle themselves would have been contributing approximately 2.5 mm of moisture/day to the pad (Sweeten, Reference Sweeten1982). The 24 h which elapsed from the removal of the cattle from the pen to the end of feeding period sampling would have stopped the continuous addition of this endogenous moisture. This, combined with warm and windy conditions, explains why the moisture content of the substrate at the end of the feeding period was lower than the last weekly measurement.
The lower VS content of the substrate in the control pens showed there was an increase in pen clay base which may have affected the animal experience of the moisture content. Moisture is absorbed within the organic matter structure of VS, thus when VS content is reduced, a greater proportion of the water content of the substrate is adsorbed onto the surface of the mud particles. When considered with the structure and particle size of the woodchips, a greater proportion of the moisture content of the deep woodchip-bedded samples (and increasingly, the surface samples of W15 as the experiment progressed and the stratification of the substrate was reduced in that treatment), was absorbed within the woodchip particles, and thus ‘unavailable’ in the substrate. These combined factors may mean that the cattle experienced the control substrate as ‘wetter’ for similar crude moisture contents.
The force required for an animal to pull its foot out of mud is a combined effect of friction of mud along the surface of the hoof, a constant suction force created by the resistance of mud to flow into the void left by the moving hoof, and a small force due to accumulation of mud on the hoof (Riskowski and DeShazer, Reference Riskowski and Deshazer1976). Riskowski and DeShazer (Reference Riskowski and Deshazer1976) found a positive association between the energy required to pull a hoof out of the mud, and the depth (tested range 25–228 mm) and VS content (tested range 82 and 212 g/kg) of the mud; and a negative association with moisture content of the mud (tested range 450–990 g moisture/kg). In the woodchip-bedded pens, a lower penetrable depth is likely one of the main contributors to the lower work requirement to withdraw the leg analogue from the substrate. Decreasing moisture content increases the resistance of mud to flow into the void left by the withdrawal of the hoof, thus increasing the suction force against the pull of the leg. Although the surface of the woodchip-bedded pens had a lower moisture content than the control pens, this did not result in a higher force requirement, and it is possible that the heterogenous nature of the manure and woodchip-mixed substrate affected its ability to flow, and therefore the suction force. The moisture content of all pens at the time of the leg analogue experiment (24 h after removal of the cattle from the pens) was 5–10 % reduced from peak moisture content, potentially causing an overestimation of the force requirement. The pull force required in the control treatment (~44 kg) in the present experiment was much higher than that recorded for similar mud depth and moisture content in a silty clay loam soil (~11 kg) in the tests of Riskowski and DeShazer (Reference Riskowski and Deshazer1976). This is most likely because that earlier research tested soil samples with VS contents of 12–30 % of those in the present control treatment, possibly because of reduced or no manure content in the soil samples of the earlier research. However Riskowski and DeShazer (Reference Riskowski and Deshazer1976) concluded that the additional energy requirement for a feedlot animal walking through mud would not be a substantial contributor to decreased performance of feedlot cattle, this present experiment shows that that may be a significant underestimate.
Because the leg analogue hung from a tractor's hydraulic arm, vibrations translated to the load cell which resulted in a small amount of noise in the data, especially once the leg was free from the manure. This vibrational interference resulted in the retracted leg not always reaching a stable zero-point, whereby the only tensile force on the load cell once free from the suction tensile forces, was that of the leg analogue mass. To improve this for future experiments, a suitable heavy static testing platform could be lowered in place within each pen to remove the vibrations associated with the tractor's engine and hydraulic pump. The hydraulic piston that the leg analogue and load cell attach to could then be powered by a hydraulic pump external to the platform to further reduce such vibrations.
Substrate removed at end of feeding period and potential value
At the end of the feeding period, there was significantly more substrate (in volume and dry weight) in W15 than control, and in W30 than control or W15. This difference was attributable to the weight of the woodchip bedding added as part of the treatments. There was no overall effect of treatment on the bulk density of the pen substrate at the end of the feeding period, however bulk density of the deep stratum of the W15 pens was increased compared to W30 pens. This aligns with observations of manure becoming well mixed with the woodchip, reducing the porosity of the deep stratum in W15.
The ideal C : N ratio for composting is in the range of 15–30 (Smith et al., Reference Smith, Chadwick, Dumont, Grylls and Sagoo2010). This puts the cleaned manure from the control pens at the lower end of this range, and that from W15 at the upper end. The W30 manure would contain too little N to be an effective compost. Previous comparison of woodchip and straw bedding in feedlots has found that manure mixed with woodchip was suitable for composting when added at 27.2 kg/m2 for a 6–7 month feeding period where cattle stocking density was 18 m2/head, or 2.5 kg woodchip/head.day (Larney et al., Reference Larney, Olson, Miller, Demaere, Zvomuya and Mcallister2008). The present research tested woodchip application rates of 6.2 (W15) and 12.4 (W30) kg woodchip/head/day. For on-farm composting of feedlot manure, the W15 substrate may be a simpler, more cost-effective product to compost on-farm than uncontaminated manure, since it would not require other sources of C to be added. The W30 deep stratum may be suitable for reuse without composting, reducing the volume of woodchips required for the next pen establishment, however this requires further investigations including potential biosecurity consideration. Further research into the composting of woodchip-contaminated manure from feedlot pens is recommended so as to better understand the commercial value and opportunities of this product.
When commercial feedlots are considering the implication of similar application of woodchip as provided in the outcomes of this experiment, there are several factors that will need to be considered. It is important that the outlay cost of woodchip is considered in terms of the feeding period it will be used for, so that costs are amortized to $/head/day. Increasing the lifespan of the woodchip to 180 days or more would significantly reduce the cost of woodchip on a head-day basis. This experiment tested a 109-day feeding period, and it is possible that at the W15 level of woodchip application, the benefits of this strategy for cattle may have started to decrease beyond this. The observed increase in pen scores and mixing of manure through the woodchip substrate by week 10 in the W15 treatment pens indicates that a higher application rate may be required for longer-fed cattle. The increased volume of substrate removed from the pens will also incur additional costs for cleaning time and equipment. Since the deeper substrate in W30 remained clean, it may be possible to clean off the more heavily contaminated top stratum only, but this is unlikely to provide any savings compared to only applying woodchip at the W15 rate, and may increase risk of infectious foot disease. Any production benefits from reduced energy lost to walking through the mud, increased cattle comfort and reduced incidence of ‘cast’ heavy cattle will increase the returns of using woodchip bedding. This project was conducted on the east coast of Australia where eucalypt woodchip is available, but in other regions, different tree species such as pine may be available more, and so woodchip may vary in longevity and moisture absorption rate.
Conclusion
This research found that providing feedlot cattle with either 150 or 300 mm depth of woodchip bedding during a 109-day feeding period improved the condition of the pad substrate for cattle comfort by reducing penetrable depth and moisture content of the substrate surface stratum. Cattle provided with woodchip bedding did not sink as low into the substrate. When the W30 treatment was applied to the pens, the cattle stood on a relatively clean surface even at the end of the feeding period. This reduction in penetrable depth caused significant reduction in the force required to pull a leg analogue from the substrate in the woodchip-bedded pens. There was no additional benefit of W30 over W15 overall, however, for longer feeding periods a greater quantity of woodchip may be required than was provided by W15, as by 10 weeks on feed, the surface and deep substrate strata were mixed and penetrable depth was increasing in this treatment. The W30 pens were still in good order at the end of the trial and cattle could have been fed longer while maintaining good pen condition. The substrate removed from the pens after the 109-day feeding period was at the upper limit of the ideal range of C : N for composting in the W15 pens, and at the lower limit for the control pens, while the W30 substrate had too little N content to be effectively composted. It is important to match planned feeding period duration to woodchip application depth in order to maintain pad condition and the value of the substrate for composting.
Acknowledgements
This research was conducted on Anëwan land. The authors thank the staff of the University of New England Smartfarms Tullimba Feedlot and Anthony McKinnon for their contributions to this research.
Author contributions
J. W. and F. C. conceived the study. All authors contributed to design of the study in different parts. J. W., A. T., F. C., R. F. and J. T. conducted data gathering. J. W., A. T., F. C. and J. T. conducted sample analysis. J. W., F. C. and R. F. conducted statistical analyses. J. W., F. C., A. T. and R. F. contributed to interpretation of the data. J. W., F. C. and A. T. drafted the manuscript. J. W., F. C., A. T., J. T. and R. F. reviewed and revised the manuscript.
Financial support
This research was funded by Meat and Livestock Australia Pty Ltd (Grant no. B.FLT.0244).
Conflict of interest
The authors have no conflicts of interest to declare that are relevant to the content of this article.
Ethical standards
All animal procedures in this research were approved by the University Animal Ethics Committee (AEC18-038).