Glossary
- AFNOR
Association Française de Normalisation – French Standards Organization
- ALCA
Attributional Life-Cycle Assessment
- BOM
Bill of Materials
- CAPEX
Capital Expenditure
- CGE
Computable General Equilibrium
- CLCA
Consequential Life-Cycle Assessment
- EOL
End of Life
- GHG
Green House Gas
- LCA
Life-Cycle Assessment
- LCC
Life-Cycle Cost
- MFA
Material Flow Analysis
- OPEX
Operational Expenditure
- PLD
Product Line Design
- R&D
Research and Development
- ROI
Return on Investment
- SABEC
Stochastic-Activity-Based Energy Consumption
- SETAC
Society of Environmental Toxicology and Chemistry
- UNEP
United Nations Environment Programme
1 Introduction
Sustainability has steadily gained attention due to its overarching impact for evaluating and guiding designed systems and products across a wide variety of domains and disciplines. Sustainable design has become more complex as its field of applications has widened from consumer products to complex industrial systems. The scope of sustainability reaches beyond design and manufacturing stages to usage, maintenance and end-of-use stages, i.e., the entire life cycle leading to realization of circular economy. Design science – theory, methodology, guided tools etc. – has been central to recent advances, as evidenced in a wealth of literature and case studies (Papalambros Reference Papalambros2015).
While initial advances in sustainable design came from a desire to reduce environmental impacts during the life cycle, the recent focus has more comprehensively included all three aspects (‘pillars’) of sustainability, i.e., environmental, economic and societal (Kloepffer Reference Kloepffer2008). Rather than focusing on only one of the three, recent works combine economic and environmental aspects together or evaluate effectiveness from a society–community perspective.
Ecodesign serves as one of the main drivers for circular economy practices, whose connection to achieving the UN’s Sustainable Development Goals (SDGs) is documented in Schroeder, Anggraeni & Weber (Reference Schroeder, Anggraeni and Weber2018). Among the 17 SDGs, circular economy practices (enabled in part by ecodesign) have direct link to SDG 6 (Clean Water and Sanitation), 7 (Affordable and Clean Energy), 8 (Decent Work and Economic Growth), 12 (Responsible Consumption and Production) and 15 (Life on Land). In Perpignan et al. (Reference Perpignan, Robin, Baouch and Eynard2019), the authors also noted that the national effort in France to reduce green house gas (GHG) emission by 75% in 2050 has direct relevance to SDG 9 (Industry, Innovation, and Infrastructure) and SDG 12 (Responsible Consumption and Production).
Sustainability research has not only gained comprehensiveness by addressing all three pillars, it has also effectuated a shift in direction, for a number of reasons. First, the focus on product has been expanded to systems. Sustainability affects design and manufacturing processes for products and systems, which is expanded further in the areas of system operations, product usage, end of use, remanufacturing, etc. Second, new tools and themes are emerging with the advancement of computing capabilities and new awareness by policymakers and regulators. As a result, new areas of application are emerging for sustainability research and practice, such as design analytics, product take-back and recycling laws, regulatory guidelines and more.
Understanding a product’s usage in full detail has been a challenge due to the difficulties in gathering noisy and diverse real data. With advances in telematics systems enabled by manufacturers such as GM, Ford, Volvo, John Deere, Caterpillar and Rolls Royce, it is now possible to collect product usage data in real time. This kind of advancement has enabled new ways of approaching product design for sustainability, which in turn leads to new research themes, such as design analytics and data-driven design, for example.
This paper is intended as a position paper to identify new research themes in ecodesign (with concurrent environmental and economic focus) based on our recent observations from project experiences in diverse fields. We have compiled and studied a diverse set of cases to analyse and collect emerging themes and research areas. All these case studies have proven successful and meaningful as they have solved practical issues for companies, consortia or governments. They have used a diverse set of modelling, simulation and optimization methods, and most have resulted in academic publications. The paper is not intended as a systematic review and the case-study findings do not cover all relevant topics in sustainability research exhaustively, but our observations do provide relevant rationale for pursuing newly emerging research trends.
The paper is organized as follows. Section 2 describes the methodology process adopted for this research. Section 3 clarifies the identification of the 10 case studies and gives a brief description of each case by grouping them into three categories. Section 4 describes how our analysis of the case studies and relevant literature identified five ecodesign issues. Section 5 explores these five areas in detail. Section 6 discusses the results and summarizes a new set of newly emerging challenges. Section 7 sets out the conclusions and future perspectives.
2 Methodology
The methodology proposed in this paper is illustrated in Figure 1. It is composed of four steps, which are detailed in the following paragraphs.

Figure 1. Overview of the proposed methodology based on case-study analyses.
Step 1 – Identification of the 10 case studies. First, we identified 10 case studies considered as representative of their research activities in ecodesign in the past few years. These case studies concern different industrial sectors, scopes and objectives, as detailed in Section 3.
Step 2 – Identification of the 5 ecodesign issues. Second, we propose a framework to analyse the case studies based on five concurrent environmental and economic issues: (1) optimal resource use for less environmental impact, (2) managing lifespan, (3) understanding users and usage, (4) integrating data and analytics, and (5) defining system boundary and perimeter. These ecodesign issues form a common thread across many of the case studies, even though the cases are from diverse backgrounds and industries. The framework helps to understand the emerging issues in ecodesign research and serves to guide future directions. The choice of these 5 issues is justified and enriched with the literature. This step is described in Section 4.
Step 3 – Exploration of the 5 ecodesign issues. The 5 ecodesign issues are then explored in detail by illustrating the main scientific issues with the case studies. For each issue, we review the literature to confirm our vision with the state of the art. In this way, we identify what is being or has been done in each area and what issues remain for research. Section 5 details the exploration of the 5 issues.
Step 4 – Discussion of the main research challenges. On the basis of the 5 illustrated ecodesign issues, we deduce the main research perspectives in ecodesign from our point of view. We discuss each of these challenges and highlight whether they have already been discussed in the literature and whether our viewpoint is convergent. This discussion is proposed in Section 6.
3 Identification of the 10 case studies
3.1 Identification of the case studies
To shed light on emerging research trends in ecodesign, 10 recent ecodesign or sustainability case studies are presented in Table 1 and §§ A.1–A.10 and analysed according to five main ecodesign issues detailed in Section 4. These 10 case studies were selected from the authors’ past or current ecodesign research projects, from 2009 to 2017. We have limited the case pool to those with which we have in-depth prior or current experience and access to data and results. The case studies were chosen to be representative of our work, including our teams and collaborations. We set out to select case studies that demonstrate at least one of the following characteristics:
(i) Being ecodesign-centred with a strong stake in the environmental dimension leading to an assessment and improvement of environmental performance.
(ii) Dealing with business concerns and being representative of multiple industrial sectors.
(iii) Covering the entire life cycle.
(iv) Connected with the economic and social dimensions of sustainable design if possible.
(v) Being representative of multiscale ecodesign issues, from end user to entire value chain.
(vi) Covering different ecodesign approaches, methods and tools.
The case studies also span the three environmental, economic and social pillars of sustainable development (Kloepffer Reference Kloepffer2008). The case studies extensively contribute to the environmental and economic pillars (all of them for the environmental aspect; eight of them for the economic aspect), while less than half contribute to the social aspect. This imbalance in contributions to the three pillars may be due to different availabilities of metrics to assess them. The metrics to assess the environmental and economic aspects are well documented and widely adopted by many in academia and industry. However, the metric to assess the social aspect is not readily available in a quantitative form. This imbalance could present a potential opportunity for future research to link the social aspect to the other two aspects. Here, however, we focus only on the environmental and economic dimensions, leaving the social dimension as a perspective.
Table 1. Overview of the 10 case studies. The information in brackets is a perspective for addressing the issue that has been identified but not yet done

The cases cover multiple industrial sectors (heavy industry with metallurgy, automotive, heavy-duty equipment, agrifood, consumer electronics, building and architecture) with different contexts, objectives and design methods and tools. Cases 1 and 2 deal with metallurgy. Case 1 (aluminium substation) concerns the development of an adapted ecodesign process for complex industrial systems such as AC/DC conversion substations for aluminium smelters. Case 2 (forge furnace) compares the sustainable performance of forge furnace alternatives by integrating environment, costs, clients and operation context. Cases 3 (automotive axle) and 4 (automotive recycling chain) concern the automotive sector. Case 3 assesses the economic and environmental impacts of automotive part replacement, while Case 4 analyses possible scenarios to develop an automotive glazing recycling chain. Case 5 (cotton harvesting) studies different harvesting systems to assess and compare their environmental and economic impacts. Cases 6 (olive packaging) and 7 (pork value chain) deal with sustainability-pressured agrifood products and systems. Case 6 compares environmental impacts of different packaging alternatives taking into account consumer usage and country context, while Case 7 considers the sustainability impacts and performances of a French pork value chain. Case 8 (consumer electronics) models the time-varying advantage of remanufactured consumer electronics products. Finally, Cases 9 (building and externalities) and 10 (building and usage) concern the building and architecture sector by integrating sustainable externalities with occupant behaviour.
An extended description of each case study is given in §§ A.1 to A.10. Each case study is also detailed in standard breakdown sheets available online. The web link is provided in appendix A. At this stage, we would urge the reader to carefully read at least the short case descriptions in §§ A.1 to A.10 if not the detailed descriptions found by following the URL links, to get a deeper understanding of this paper and process of coding and analysing the 10 use cases.
Next we group the 10 case studies into three categories as they cover all or some of the life-cycle phases of a product or system. Some of them concern the entire life cycle (Section 3.2), while some are mainly focused on the use phase (Section 3.3) and others mainly deal with end of life (EOL; Section 3.4). For each of these three categories, we give a ‘big picture’ to illustrate the case studies concerned, and a detailed picture in one particular case to highlight the issues raised and analysed in Section 4. Detailed descriptions of all 10 case studies can be found in appendix A, and further details can be found in Table 1. The whole life-cycle-oriented case studies (Cases 1, 2 and 7) investigate sustainability issues spanning the entire life of a product or system – design/manufacturing, distribution, usage, maintenance, and EOL management. They cover a wide variety of large-scale systems where the sustainability issues are considered not just for a particular life-cycle phase but more comprehensively for the entire life cycle. The scope of these case studies therefore tends to be larger than for those for a single product or system. The use-phase-oriented case studies (Cases 5, 6, 9 and 10) focus on how a product or system is used and how to capture the usage in the context of sustainability. Once a product or system is in operational use, it is important to understand the context and pattern of usage and gather data associated with diverse usage environments. Emerging technologies such as telematics systems have become increasingly important for capturing this usage data, as further explained in the case studies. The EOL-oriented case studies (Cases 3, 4 and 8) mainly focus on reuse, recycling and remanufacturing aspects of a product or system at the end of the life cycle. In line with the five sustainability issues in our framework, EOL operations carry clear benefits.
3.2 Life-cycle-oriented case studies
Life-cycle thinking, or the life-cycle approach (see for example the Life Cycle Initiative proposed conjointly by UNEP and SETAC; UNEP & SETAC 2012), considers the impacts generated throughout a product’s life cycle – from design/manufacturing through distribution, usage and maintenance and on to EOL management – in order to monitor and improve them in a structured way. Companies are increasingly implementing this paradigm, but considering and improving a product or system’s life-cycle-long environmental performances remains a challenge, so they often find it helpful to focus on particular phases. However, 3 of the 10 case studies presented in this paper consider every phase of the entire life cycle equally (Cases 1, 2 and 7).
Case 2 (forge furnace) deals with the selection of forge furnace technologies in a given context (location, client, energy supply). LCA results and life-cycle costs (LCC) are sensitive to the client’s profile (Leroy, Cluzel & Lamé Reference Leroy, Cluzel and Lamé2014). Different profiles have been defined to study how decisions could evolve according to these profiles, which are defined by environmental awareness and priority given to CAPEX or OPEX (does the client prefer a short-term perspective with little expenditure or prefer low operational costs?). Manufacturing, distribution and use phases are explicitly taken into account to estimate LCC and environmental impacts, but the shortage of data makes it difficult to include the EOL phase.
Case 7 explores the French pork value chain. Consumers increasingly want more information on the origin and quality of the food they buy: information on origin, breeding conditions and animal welfare, substances absorbed during the animal’s life (antibiotics, genetically modified organisms), carbon footprint etc. Case 7 shows which kinds of data are already captured in a food value chain and considers every phase of the life cycle, whether the product or system under study is the food source or the associated industrial value chain (Petit et al. Reference Petit, Bertoluci, Trystram, Lecomte and Chapdaniel2014). An LCA model has been developed and alternative scenarios for new solutions have been evaluated and used for decision-making between the actors of the value chain.
The life-cycle-oriented Case 1 (aluminium substation) considers the entire life cycle of a complex industrial system, namely an AC/DC conversion substation (described in Figure 2) used to convert energy for massive energy-consuming aluminium smelters. The design of these substations was independent of their future location, i.e., mainly where energy is abundant and cheap, namely in the Nordic countries with hydropower like Canada, Iceland or Russia, or in the Gulf countries or China, which use fossil energies. However, varied facility locations result in widely varied industry contexts: local electrical mix, distance to clients, client-led management of industrial systems (preventive versus corrective maintenance, updates and revamps), operating conditions in terms of temperature or humidity, regulations or practices for managing EOL components or systems and so on. Ecodesigning this type of industrial system requires consideration of industry-specific parameters and entire life-cycle scenarios.

Figure 2. Overview of mass and energy flows for an aluminium substation (Cluzel et al. Reference Cluzel, Yannou, Millet and Leroy2014).
First, a scenario-based LCA model was proposed to capture the environmental impacts of the entire life cycle despite the lack of data and the variability of exploitation contexts (Cluzel et al. Reference Cluzel, Yannou, Millet and Leroy2014). This semi-quantitative approach highlights for example that the best-case scenario in a particular context (substations supplied by hydroelectricity in a Nordic country) can decrease the environmental impacts by 70% compared to the worst-case scenario. The LCA model embracing the entire life cycle thus leads to different ecodesign actions (for example, decreasing masses of components but increasing electrical losses versus decreasing electrical losses by increasing masses of components) in a specific exploitation context.
Further, these LCA results were used to lead an eco-innovation approach based on a multidisciplinary working group composed of experts from different departments of the company (Cluzel et al. Reference Cluzel, Yannou, Millet and Leroy2016). The main deliverable of this working group was a portfolio of eco-innovative R&D to build the company’s strategic R&D road map. The method ensures a balanced portfolio, i.e., including projects with short-, mid- or long-term deadlines, projects dealing with components, subsystems, systems or supersystems, projects involving organizational, technological or methodological actions and projects considering different life-cycle phases of the system.
3.3 Use-phase-oriented case studies
In a number of cases, the use phase dramatically impacts a system’s ecodesign performance (Throne-Holst, Stø & Strandbakken Reference Throne-Holst, Stø and Strandbakken2007). However, real use emerges after the design and product launch phase. This is why user/usage profiles/patterns and sales prediction models are needed to integrate these nontrivial data models into LCA simulations.
Nine of the ten case studies highlighted in this paper, i.e., studies 2–10, directly consider – or could consider – users and usage to inform the ecodesign. This is especially true for case studies 5 (cotton harvesting), 6 (olive packaging), 9 (building and externalities) and 10 (building and usage).
The use-phase-oriented Case 10 is explained in more detail below. Building stock accounts for between 16 and 50 percent of national energy consumption worldwide (Saidur, Masjuki & Jamaluddin Reference Saidur, Masjuki and Jamaluddin2007; Hoes et al. Reference Hoes, Hensen, Loomans, De Vries and Bourgeois2009a,Reference Hoes, Hensen, Loomans, de Vries and Bourgeoisb; Masoso & Grobler Reference Masoso and Grobler2010). Governments around the world are thus rolling out energy directives, national regulations and energy-efficiency labels that set minimum requirements for building performances and promote the construction of ‘green’ buildings. Building stakeholders have thereby started dealing with buildings as products with services rather than just simple products. Services may for instance include energy monitoring or equipment maintenance during a building’s use phase. Moreover, new market expectations such as ‘energy performance contracts’ have started to emerge in a number of countries. Such services and offers thus require a better control of performance variability during a building’s life cycle. Consequently, a better understanding and consideration of the key determinants of energy performance has become essential for building design and marketing processes. Occupant behaviour is a substantial source of uncertainty in energy consumption, as inter-individual behavioural variations can account by as much as 100% for a given dwelling (Guerra-Santin et al. Reference Guerra-Santin, Romero Herrera, Cuerda and Keyson2016). The reasons are that different people generate different numbers of activities and may own more or less eco-efficient electrical appliances, both of which depend on household composition, lifestyle and socio-demographic background (Reinhart Reference Reinhart2004; Yun, Tuohy & Steemers Reference Yun, Tuohy and Steemers2009; Haldi & Robinson Reference Haldi and Robinson2011; Langevin, Gurian & Wen Reference Langevin, Gurian and Wen2015). This is why Zaraket et al. proposed an activity-based model for forecasting household energy and water consumption for residential building design (Zaraket, Yannou & Leroy Reference Zaraket, Yannou and Leroy2014). A user-centred statistics-driven approach correlates occupants’ profiles (socio-economic and -demographic) to quantities of domestic activities, appliance ownership and energy and water footprints. This model generates more accurate energy and water consumption forecasts and gives a means to assess usage-trend disparities in domestic consumption (Zaraket et al. Reference Zaraket, Yannou, Leroy, Minel and Chapotot2012). In total, 28 domestic energy- and water-consuming activities have been modelled as illustrated in Figure 3. These consumption models are expressed with approximately 20 parameters featuring household composition. Each activity was modelled in an Excel spreadsheet by a subset of influential household variables, a causal graph of influence starting from these household variables and ending with activity quantities via a number of quantitative intermediate variables (e.g., occupancy rate, number of weekly meals, laundry weight, etc.), modelling assumptions, data inputs from statistical databases or national consumption data and procedures used for model fitting to national consumption data. Figure 3 depicts the model architecture. These simulations of energy and water consumption based on different household profiles make it possible to
(i) refine or increase the accuracy of energy performance contracts;
(ii) simulate the influence of certain in-frame technical solutions (e.g., effectiveness or number of pre-installed washing machines);
(iii) obtain heat gain estimates per activity that are useful inputs for more accurate dynamic thermal simulations.
The originality of this work is the per-activity breakdown of domestic electricity consumption, which is vital, as occupants made aware can monitor and regulate their activities. This model could serve to build graphical displays helping occupants voluntarily reduce their consumption (via incentives, social-network emulation, diagnostics and action plan support etc.). Other similar approaches that link usage conditions to energy consumption can be found in the literature. As an illustration to highlight a couple of relevant use-phase-focused examples, Telenko (Telenko Reference Telenko2012; Telenko & Seepersad Reference Telenko and Seepersad2014) used probabilistic graphical models – a type of Bayesian network – to represent usage context as a network of factors characterized by local conditional probability distributions. Ma (Reference Ma2015) recently proposed collecting large-scale sensor data on product operation to mine usage patterns and build a usage model for LCA. He developed a predictive usage mining for life-cycle assessment (PUMLCA) algorithm to segment usage patterns and provide a more accurate estimation of environmental impact.

Figure 3. The SABEC model architecture (left side) applied to 28 activities of water and energy consumption.
Another project (Case 9) noted that ambitious building retrofits – positive externalities – to improve energy performance are often barely justified by energy savings alone. Indeed, the ROI to halve a building’s energy consumption is more than 25 years, which often discourages investors. Energy efficiency thus needs to be considered differently to be economically justified, which prompted a consortium of big French construction companies and academic partners to co-develop a new methodology called DECADIESE (Cluzel, Yannou & Costa Reference Cluzel, Yannou and Da Costa2015). Broader than an energy-efficiency focus only, DECADIESE aims to capture the sustainable value of a building with an original focus beyond the scope of classical analytic methods, by extending the perimeter of associated stakeholders to enable ambitious building projects.
Going further, Bertoluci, Leroy & Olsson (Reference Bertoluci, Leroy and Olsson2014) showed how collecting product use-phase information is crucial to determine whether a product or system is sustainable and eco-friendly. Abi Akle, Bertoluci & Minel (Reference Abi Akle, Bertoluci and Minel2013) showed that concentrated detergents branded as ‘green’ are actually slightly less environmentally friendly in practice as uninformed consumers tend to overdose them. In Case 6 (Bertoluci et al. Reference Bertoluci, Leroy and Olsson2014), it was shown that the environmental impact of olive packaging type was different in different countries depending on their packaging recycling rates, consumer food habits and olive loss rates related to packaging types.
Last, in Case 5, John Deere developed a new cotton harvesting system with a revolutionary cotton-stripping and module-making design (Quan et al. Reference Quan, Kim, Knight, Nelson and Finamore2015) that reduces harvest loss while enabling flexible configuration of cotton collection modules. Quan et al. (Reference Quan, Kim, Knight, Nelson and Finamore2015) showed that the environmental impact of a cotton field harvest depends on a series of configurations, i.e., composition of multiple machinery and equipment to ‘get the job done’ and harvesting task strategy, which is why harvester ecodesign is dependent on the ability to simulate all usage configurations and strategies.
3.4 EOL-oriented case studies
Environmental regulations have urged stronger product stewardship in product retirement and maintenance, which has resulted in a wealth of literature and industrial practices in the area of product recovery for reuse, repair, refurbishment and recycling. Successful recovery operations enable companies to gain economic advantage while complying with environmental regulations. In other words, the objective of the recovery operations is usually twofold: to maximize recovery profit and minimize adverse environmental impacts.
The nature of recovery operations, however, depends on an array of factors including product design, assembly/disassembly processes, buyback, reverse logistics, production planning, pricing of new and remanufactured products, managing product take-back timing, design upgrades etc. If remanufacturing is pursued as a viable recovery operation, for example, setting the prices for buyback and for remanufactured products directly affects total profit. In addition to pricing decisions, production planning – matching supply plan to demand plan, details of disassembly operations and production quantity – affects total profit as well.
The EOL-oriented case studies (Cases 3, 4 and 8) mainly focus on the reuse, recycling and remanufacturing aspect of a product or a system at the end of the life cycle. Cases 3 and 4 are from the automotive industry, where a part replacement decision is investigated in the remanufacturing context (Case 3) and a large-scale national level of automotive glass recycling is studied and optimized along with recycling network design (Case 4). Case 8 is for consumer electronics where product design and timing of remanufacturing were co-considered to gain economic and environmental advantages. These case studies illustrate the wide variety of products and relevant manufacturing and logistics issues considered in the EOL study category. While keeping the EOL context as the common thread in this subsection, additional details of Case 8 are provided next for illustration.
One of the core questions in EOL operations is whether product recovery operations truly benefit the company when evaluated by the sustainability pillars. A typical question could be Is a remanufactured product better than a brand-new product? (Kwak and Kim Reference Kwak and Kim2016). Case 8 offers an approach to estimating the economic and environmental benefits of EOL operations, in particular remanufacturing. Remanufacturing has time-varying benefit (i.e., timing of product take-back and remanufacturing) due to physical deterioration and technological obsolescence.
The framework in Case 8 provides a quantitative link between the nature of the product (e.g., product specification, physical and technological characteristics of each part, production costs) and the time-varying value of remanufacturing from the remanufacturer’s perspective. More specifically, the case answers the following questions:
(i) Is a remanufactured product better (both economically and environmentally) than a brand-new version of the product?
(ii) How does timing of remanufacturing affect the advantages of a remanufactured product?
(iii) How do market conditions (e.g., market preferences for a remanufactured product and customer requirements for product specifications) influence any advantages from remanufacturing?
The model incorporates two critical aspects – physical deterioration and technological obsolescence – to construct the framework for optimal production planning. It considers both economic and environmental aspects by capturing unit production cost and environmental impact savings from remanufacturing in comparison to manufacturing brand-new products. The perceived utility by customers diminishes from initial sales to EOL. After remanufacturing, the utility is increased to the level of significant improvement, although it may be lower than that at the time of the initial sale. When the product becomes a remanufactured product, the pattern of diminishing utility repeats, but possibly with a different rate of change. The logic of this model in explained in Figure 4.

Figure 4. Two components of optimal product design for life-cycle profit: initial product design and design upgrade at the EOL stage (Kwak & Kim Reference Kwak and Kim2015a).
The model specifically introduces the price ratio ($\unicode[STIX]{x1D6FD}$;
$0\leqslant \unicode[STIX]{x1D6FD}\leqslant 1$) of the remanufactured product to the equivalent brand-new product, which was simulated under various product recovery operations. Two distinct scenarios can be optimized with this model. First, if the price ratio is set as a certain number (e.g.,
$\unicode[STIX]{x1D6FD}=0.7$, i.e., the price of the remanufactured product is 70% of the brand-new price), the model calculates the product generation up to which the net-profit advantage is maintained (e.g.,
$t=7$), i.e., if a product is older than 7th generation ago, do not remanufacture. Second, if the age of the product is given as a fixed value, the model calculates the
$\unicode[STIX]{x1D6FD}$ ratio for remanufactured product pricing. Additional sensitivity analysis can also be performed depending on cost and environmental impact changes for each part or manufacturing operation.
The next section proposes five ecodesign issues abstracted from the case studies and the literature as a framework to analyse the 10 case studies.
4 Identification of the five ecodesign issues
4.1 Analysis of the case studies
After sharing in-depth knowledge about each case listed above, we identified the common and major issues. We consider the following issues as representative of recent developments and new trends in ecodesign research, although we do acknowledge there may be others that are outside the scope of the case studies. We do not claim these five issues are exhaustive nor fully independent. Rather, the intent is to provide an overview to help identify common threads and lay the ground for future research topics. The five ‘ecodesign issues’ that we have identified are as follows:
(1) Optimal resource use to reduce environmental impact
(2) Managing lifespan
(3) Understanding users and usage
(4) Integrating data and analytics
(5) Defining system boundary and perimeter
4.2 Confirmation with the literature
Four of these ecodesign issues (numbers 1–3 and 5) were also identified by Pigosso, Mcaloon & Rozenfeld (Reference Pigosso, Mcaloon and Rozenfeld2015) who characterized the state of the art and future trends in ecodesign from a bibliometric analysis covering the period 1996–2015. Managing lifespan had already started to surface in the literature in the period 2006–2010, but these four issues are reported by Pigosso et al. as a subset of nine trends for future ecodesign research. Resource efficiency (issue 1) and managing lifespan (issue 2) were classified in the trend ‘Development of products and services’. Development of product–service systems is assumed to lead to high dematerialization. The main consequences will be an increase of product lifespan and optimized resource consumption. Concern to use resources optimally (issue 1) is also covered by the ‘Circular economy’ trend, where waste management and design for EOL are predominant. Pigosso et al. (Reference Pigosso, Mcaloon and Rozenfeld2015) reports issues 3 and 4 as being part of the ‘Systems thinking’ trend. The necessity to characterize interactions among subsystems requires first defining or redefining system boundaries, and second acquiring more robust information on users, usages and user behaviours. These five issues are detailed in Section 5 and then discussed in Section 6.
The fact that the case studies presented in this paper match the state of the art by Pigosso et al. serves to validate the major categories of sustainable design reported in the literature. Here, in addition to validation, we intend the in-depth knowledge and insight from our case studies to provide a more specific picture of current ecodesign practices and trends along with the perspectives for future efforts described in Sections 5 and 6. Sections 5 and 6 bring core contributions of this position paper for future research directions based on in-depth analysis of the five ecodesign issues.
5 Exploration of the five ecodesign issues
After analysis of the 10 case studies and following the analysis framework proposed in Section 2, we identified five main ecodesign issues that we go on to explain in more detail in the next subsections. Table 2 gives a roll-up of all the contributions of the 10 case studies to these five ecodesign issues.
Table 2. Contribution of the 10 case studies to the five ecodesign issues

5.1 Optimal resource use for less environmental impact
5.1.1 Context
Using resources – materials and energy – optimally is one of the most obvious concerns when ecodesigning products, systems and services. As resources are finite, the simplest way to make more sustainable products and systems is to use less resource, but also to reuse and recycle existing resources where and when possible. Doing more and better with less is broadly the core concept of the circular economy, defined as an ‘economic system of exchange and production that aims to efficiently reduce the environmental impact of resource use throughout the product/service life cycle’ by the French environment and energy management agency (ADEME 2014), which subdivides the circular economy into seven axes – one of which is ecodesign (ADEME 2014):
(i) Offer of economic actors
(1) Sustainable extraction, supply, procurement
(2) Ecodesign (products and processes)
(3) Industrial and territorial symbiosis
(4) Functional economy
(ii) Demand and consumer behaviour
(5) Responsible consumption
(6) Longer duration of use
(iii) Waste management
(7) Recycling and waste recovery
Axes (5) and (6) related to consumption, demand and behaviour are directly connected with other sustainable design issues proposed in this paper: Integrating data and analytics, Understanding users and usage and Managing lifespan. From a design perspective, the other axes emerge spontaneously when we consider the question of how to promote optimal use of resources at the product or system design stage. Different strategies can be envisioned to answer this question. First, ecodesign (Axis 2) aims to minimize the environmental impact of a product throughout its life cycle. Ecodesign approaches may be seen as being mostly internal to one company and as involving one product or process – through lean and green approaches for example (Cluzel et al. Reference Cluzel, Yannou, Leroy and Millet2012) – that the company generates or uses. However, a company is likely part of a larger ecosystem encompassing other companies, territories, sectors etc. The industrial ecology (Axis 3) perspective reaches outside the traditional perimeter of a company to envision the optimization of material and energy flows across a territory. Sustainable supply (Axis 1) is another perspective that considers the origin of resources used to produce goods. Significant environmental burdens are then generated later at product EOL. Another perspective dealing with optimal resource use is of course waste management, with the ability to reintroduce secondary resources to produce new products and then to close the loop (Axis 7). The last strategy, a functional economy (Axis 4), consists in fostering a move from ownership to usage with the idea of selling services instead of products, which would hopefully mean less material intensiveness. All these strategies are closely linked and interdependent.
Decreasing resource use answers two main challenges. The first is the fact that Earth’s resources, in particular, metal and fossil resources, are finite yet depleting. Using less resources and promoting circular economies is manifestly a necessity. The second challenge is to minimize the environmental impact of products throughout their life cycle to address major environmental concerns such as climate change, ozone layer depletion, soil acidification and water eutrophication. Identifying the environmental impacts generated by products is thus a crucial task, for which there are numerous methods and tools, the most widely used being Life Cycle Assessment (LCA; International Organization for Standardization 2006).
5.1.2 Analysis of the case studies
Figure 5 shows the positioning of the 10 case studies on the seven ‘Circular Economy’ axes proposed by the ADEME. Ecodesign is of course the common denominator of these 10 cases, but this graph shows that all other axes are involved in at least two case studies, highlighting a high variety of possible strategies for ecodesign.
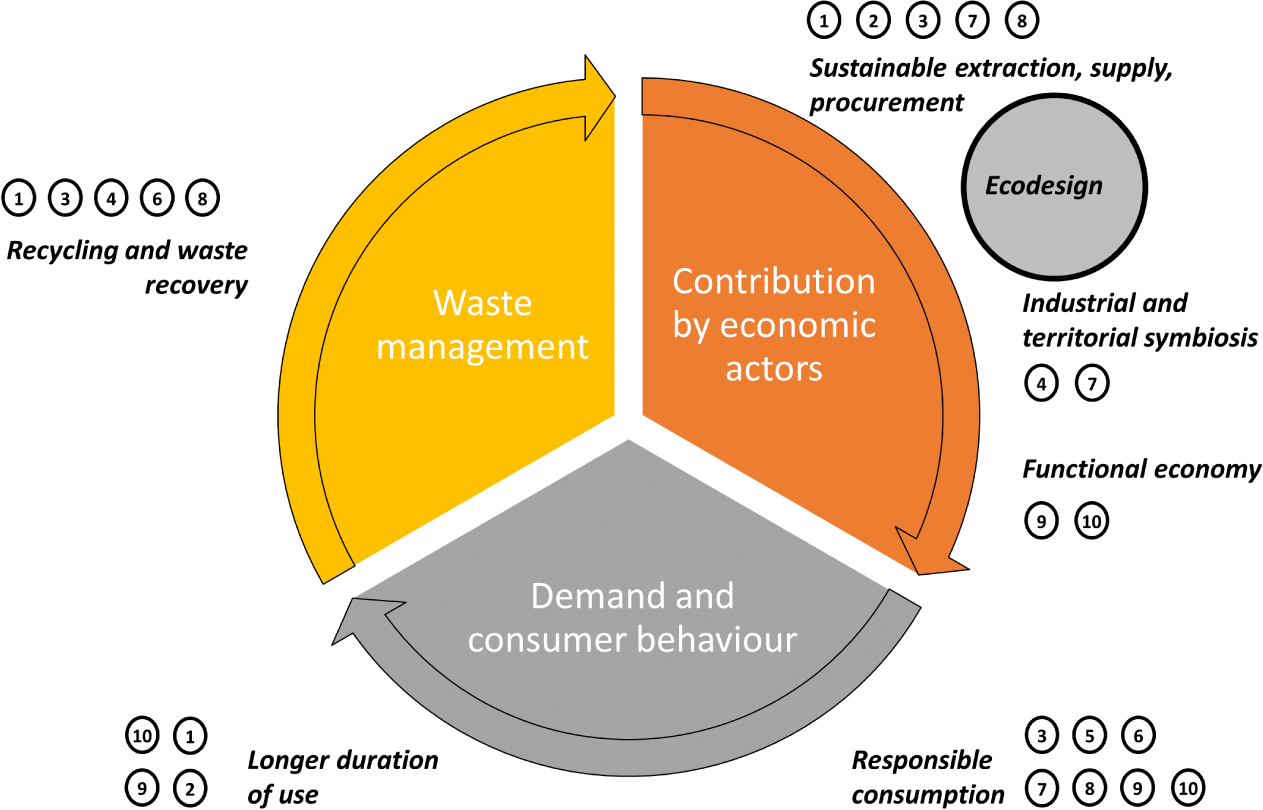
Figure 5. Positioning of the 10 case studies on the ADEME’s circular economy diagram.
It shows that Optimal resource use for less environmental impact is a major consideration in all 10 studies. Along with Defining system boundary and perimeter, it is the only common issue shared across all the case studies, and is thus an inescapable part of sustainable design. The other issues may be seen as directions that are complementary or emerging – but not necessarily less important – to complement or reinforce a product’s sustainable performance.
Going further, LCA is clearly predominant in case studies dealing with optimal resource use. LCA has become the major method for quantifying environmental impacts and then identifying critical areas to improve environmental performance (Millet et al. Reference Millet, Bistagnino, Lanzavecchia, Camous and Poldma2007), as done in Cases 1, 2, 3, 5, 6, 8 and 9. For example, LCA is used in Case 1 with a scenario-based approach to evaluate the environmental impacts of a set of operations scenarios (best case, most likely scenario, worst case) for large AC/DC conversion substations for aluminium smelters (Cluzel et al. Reference Cluzel, Yannou, Millet and Leroy2014). LCA results are then used as inputs to inform an eco-innovation process whose goal is to identify a set of eco-innovative R&D projects that could be deployed in the coming years (Cluzel et al. Reference Cluzel, Yannou, Millet and Leroy2016). Another example is Case 6 where LCA is used to compare three olive packaging alternatives (doypack, glass jar and steel can) in different countries (Bertoluci et al. Reference Bertoluci, Leroy and Olsson2014). Usages are taken into account to identify environmental impacts and set recommendations for the most ecodesigned solution in a specific country.
Other methods for considering optimal resource use employ simulations centred on specific indicators such as energy consumption (Cases 7 and 10), GHG emissions (Case 4) or environmental externalities that are broader than (but also include) classical LCA impact categories (Case 10). For instance, Case 4 simulates the emergence of a recycling value chain for automotive glazing. Performance indicators used to evaluate the model include not only economic aspects and material recovery but also lowering GHG emissions via energy savings. Simulations are performed using linear programming and system dynamics (Farel, Yannou & Bertoluci Reference Farel, Yannou and Bertoluci2013a; Farel et al. Reference Farel, Yannou, Ghaffari and Leroy2013b). Case 9 goes beyond the classical scope of LCA by considering more general environmental, social and economic externalities associated with a building construction or retrofit (Cluzel et al. Reference Cluzel, Yannou and Da Costa2015; Nösperger, Mazoyer & Vitt Estelle Reference Nösperger and Mazoyer2015). Positive externalities can be considered to extend the perimeter of stakeholders ready to finance part of the building, leading to more ambitious sustainable buildings with optimal use of resources and less environmental impact.
5.1.3 Confirmation with the literature
Optimal resource use for less environmental impact is undoubtedly the core concept or the raison d’ être of ecodesign. However, in classical design perspectives, the use phase is rarely considered in detail and rarely fully integrated in studies. Considering usage is an emerging field of research in building ecodesign for example (Hoes et al. Reference Hoes, Hensen, Loomans, De Vries and Bourgeois2009a,Reference Hoes, Hensen, Loomans, de Vries and Bourgeoisb). The use phase of a wide range of products (energy-using products, buildings etc.) is characterized by high variability of users’ behaviour, leading to large uncertainties.
For many authors, ecodesign has now become a core strategy of the circular economy, which is an economic system that represents a ‘change of paradigm in the way that human society is interrelated with nature and aims to prevent the depletion of resources [and] close energy and material loops’ (Prieto-Sandoval, Jaca & Ormazabal Reference Prieto-Sandoval, Jaca and Ormazabal2018). Ecodesign is now seen as an essential design strategy guided by LCA to diminish the environmental impacts often located at the distribution and use phases (Prieto-Sandoval et al. Reference Prieto-Sandoval, Jaca and Ormazabal2018) in the broader scope of the circular economy. The scientific literature has now moved towards strategies, methods and tools for developing the circular economy (see for example Gaustad et al. Reference Gaustad, Krystofik, Bustamante and Badami2018; Kalmykova, Sadagopan & Rosado Reference Kalmykova, Sadagopan and Rosado2018) to use resources optimally, especially through product design (Bocken et al. Reference Bocken, De Pauw, Bakker and van der Grinten2016). Researchers recently started to observe how ecodesign fits and serves the transition towards a circular economy (den Hollander, Bakker & Hultink Reference den Hollander, Bakker and Hultink2017; Richet et al. Reference Richet, Perry, Vautier and Roche2019). While the scholarship’s central message is still to decrease environmental impacts by decreasing or at least rationalizing resource use, this shift of vocabulary also shows that decision makers (in the industrial and political spheres) have started to more seriously consider these issues, even if the road is still long. Cultural barriers are considered the main bottlenecks for the circular economy in terms of consumer interest (driven by markets) and company culture (Kirchherr et al. Reference Kirchherr, Piscicelli, Bour, Kostense-Smit, Muller, Huibrechtse-Truijens and Hekkert2018). While Optimal resource use for less environmental impact is intuitively an obvious issue that the research community has long been tackling, constant effort is still needed to make it more tangible in industry.
While optimal resource use now appears to be a well-established direction in sustainable design approaches, Integrating data and analytics, Understanding users and usage and Managing lifespan are emerging research fields with the potential to fully incorporate the use phase in sustainable design. Finally, in order to make environmental impact assessments more reliable, particularly when involving increasingly complex systems, research on Defining system boundary and perimeter is also crucial. These four issues are expanded below.
5.2 Managing lifespan
5.2.1 Context
Managing lifespan, i.e., the lifetime of a product or system, is critical to achieving sustainability for economic and environmental reasons. Two main stakeholders – manufacturer and customer – have different perspectives that need to be evaluated when making lifetime decisions. These lifetime decisions include the following concerns:
(i) When is it time to retire a product by declaring the end-of-use stage?
(ii) What are the end-of-use options (reuse, remanufacturing, recycling or disposal) to choose from after retirement?
(iii) What are the benefits (economic or environmental) to consider?
(iv) What is the role of regulators?
(v) What are the indications from the usage data?
(vi) What are the product-specific considerations (e.g., size, weight, normal lifespan etc.)?
Take the example case of end-of-use product management: ‘Cash For Clunkers’, a government-driven car allowance rebate programme in the United States in 2009 (US DOT 2015). Different cash rebates were allowed depending on vehicle type and specifications. While the programme was widely popular, it is not clear how the rebate criteria were determined, particularly in terms of sustainability, which underlines the importance of managing a product or system’s lifespan.
One of the main challenges in managing a product or system’s lifespan is the transient aspect of time or pseudo-time (e.g., product generation; Guide et al. Reference Guide, Souza, Van Wassenhove and Blackburn2006; Bras Reference Bras2014). The nature of the product drives end-of-use decisions such as remanufacturing, recycling or disposal (Hauser & Lund Reference Hauser and Lund2003; Kwak Reference Kwak2012). However, the optimal lifetime decision may change as the advantage evolves over time (or over product generation). Remanufacturing may be more profitable and environmentally friendly at one point in time, but this profitability may shift as the value and benefit of remanufacturing change over time. At the end of product usage, a carefully planned remanufacturing scheme, with upgrades for example, could increase the utility perceived by customers. If no action is taken with prolonged usage of product, the utility diminishes without prolonging the product’s useful life. Results show the benefit of lifetime management in the context of sustainable design.
5.2.2 Analysis of the case studies
Individual case studies show different aspects of lifespan management and its challenges: uncertainties in defining the lifetime of a system (Case 1), time-dependent nature of value and preference (Case 3), uncertainty in demand evolution for recycled cullet from automotive glazing (Case 4), normalizing the lifetimes of multiple systems for a fair comparison (Case 5), trade-off between waste reduction and energy consumption (Case 7), optimal lifetime identification for higher economic value recovery (Case 8) or a long time horizon to realize the ROI (Case 9). In that sense, Figure 6 positions the 10 case studies according to the type of consideration they lend to lifespan management. Most of the cases (2, 5, 6, 9 and 10) broadly seek to extend the duration of the use phase. Cases 3 and 8 are more focused on taking the right decisions when the system product is reaching the end of a ‘first’ use phase (second life or not). Case 4 is dedicated to recycling scenarios to imagine reusing glazing in other applications. Finally, Cases 1 and 7 attempt to optimize the entire life cycle of a system or a value chain, including considerations on lifespan optimization.

Figure 6. Main concerns surrounding lifespan decisions with the 10 case studies positioned.
The importance of managing a product’s lifespan is evident when a product or system shows the following characteristics:
(i) Uncertainty in end-of-use status and timing to retire a product due to obsolescence or part failures.
(ii) Deterioration of economic or environmental characteristics: continuing usage has a significant economic or environmental downside, often due to regulations or wear and tear.
(iii) Potential benefit of end-of-use treatment: remanufacturing may bring economic benefit for the manufacturers by expanding market coverage for remanufactured products while sustaining new product sales.
Following these observations, a few of the case studies are further detailed below to highlight the importance of managing lifespan.
In the harvester case (Case 5), product lifespan tends to be long (usually more than 10 years). The life cycle of a harvester consists of manufacturing, usage, maintenance, and end-of-use treatment, where economic and environmental impact is present at every stage. There has been a wealth of LCA literature where the primary goal is to analyse the environmental impact of a product or a system (Hauser & Lund Reference Hauser and Lund2003; Smith & Keoleian Reference Smith and Keoleian2004; Gutowski et al. Reference Gutowski, Sahni, Boustani and Graves2011; Kwak Reference Kwak2012; Bras Reference Bras2014). However, few studies have compared two or more heterogeneous systems on their environmental impact due to the additional layer of complexity needed for a fair comparison. The harvester case study brings a unique contribution in this regard (Quan et al. Reference Quan, Kim, Knight, Nelson and Finamore2015). Two different sets of product systems (old versus new harvester models) sharing the same objective (harvesting cotton crops) were compared on environmental impact to show which system is better and why. Old and new systems are composed of different sets of machinery and equipment, which makes it difficult for a fair comparison between the two. The harvester case study provides a common task to be completed, and each contributing machine’s impact is measured with consideration of the entire life cycle.
In the consumer electronics case (Case 8), product lifespan tends to be short (e.g., 12–24 months for cell phones). Compared to the harvester case, technological obsolescence has a much more significant role to play in sustainable product design and remanufacturing decisions (Bras Reference Bras2014; Kwak Reference Kwak2016). New generations of consumer electronics with emerging technological features make it to market at a much faster pace than for agricultural equipment. As a result, in making end-of-use treatment decisions, it is critical to factor in the time-varying aspect of product value (Guide et al. Reference Guide, Souza, Van Wassenhove and Blackburn2006; Kwak & Kim Reference Kwak and Kim2011). For example, remanufacturing may be desired for a cell phone, but if the phone is too old, it will be difficult to sell at a profit in the remanufacturing market. Both consumers and manufacturers therefore need to recognize that there is a window of opportunity in making product retirement decisions. The consumer electronics case study provides a quantitative framework to guide optimizing this type of remanufacturing decision by ensuring economic profitability and environmental friendliness (Kwak and Kim 2016).
The automotive axle case (Case 3) showed that the time frame of a maintenance operation determines the type of sustainable options available and the role of regulators. Unlike the harvester and consumer electronics cases, the automotive axle case concerns replacing a part rather than a whole product. Vehicle owners tend to prefer a new replacement part when the product is relatively new but prefer a remanufactured part when the product’s residual value is low (i.e., on older vehicles; Ovchinnikov Reference Ovchinnikov2011; Ovchinnikov, Blass & Raz Reference Ovchinnikov, Blass and Raz2014). The challenge is that a remanufactured product brings environmental benefit but with a loss of economic benefit, i.e., a remanufacturer loses money by selling a remanufactured axle due to its lower price (Mitra Reference Mitra2007; Vadde, Kamarthi & Gupta Reference Vadde, Kamarthi and Gupta2007; Kwak & Kim Reference Kwak and Kim2012). For this particular case study, the data was collected for the Korean automotive market, where the government is looking to promote the remanufacturing business. With remanufacturers clearly losing money, the government needs to determine the amount of subsidy to give them, which is calculated based on sensitivity analysis. The axle case study provides a quantitative framework by which regulators can decide whether any economic incentive should be provided and how much subsidy to allocate for a particular industry or market.
5.2.3 Confirmation with the literature
In addition to the cases described above, recent papers confirm the importance of managing product lifespan for sustainable design. Product lifespan management for sustainability includes reuse of the product itself, maintenance, repair, and technology upgrades for remanufacturing (and/or refurbishment) (Bocken et al. Reference Bocken, De Pauw, Bakker and van der Grinten2016). Below we cite the notable recent work on this topic.
Aydin, Kwong & Ji (Reference Aydin, Kwong and Ji2015) provided a methodology to consider new and remanufactured products simultaneously in product line design (PLD) to acquire maximum profit and product market share. The method determines the specifications of new and remanufactured products and the time for launching remanufactured products. Aydin, Kwong & Ji (Reference Aydin, Kwong and Ji2016) also focused on PLD for which supply chain partners work with remanufacturers to maximize total profit from new and remanufactured products.
Bobba, Ardente & Mathieux (Reference Bobba, Ardente and Mathieux2016) proposed a set of indicators, called ‘Pro-EnDurAncE’ to assess product durability from both environmental and economic perspectives. The indicators capture various relevant aspects such as impact and cost of keeping and replacing products, maintenance and repair, lifetime extension and use of energy and auxiliary materials during operation. Based on the indicators, the decision whether to replace a product or extend its life was evaluated in terms of environmental and economic benefits.
Iraldo, Facheris & Nucci (Reference Iraldo, Facheris and Nucci2017) investigated whether extended durability is beneficial both environmentally and economically. LCA and LCC were applied to compare scenarios of durable versus standard options for managing life-cycle length. For the case studies of a refrigerator and electric oven (i.e., energy-using products), increased durability (i.e., extending life) was shown to be practically always economically beneficial, while the environmental impact of manufacturing and EOL treatment determines whether increased durability is a preferred option.
Kwak (Reference Kwak2016) proposed an index to measure the ‘greenness’ of product lifetime extension. The greenness index reflects the nature of a product, including the intensity of remanufacturing, technological trends and their influence on product design and user satisfaction, and the intensity of customer use in terms of environmental impact. The index provides a more comprehensive assessment as to the effectiveness of extending product lifetimes, which could be used to evaluate a variety of products.
Kim & Moon (Reference Kim and Moon2017) utilized the benefits of a product family to prolong the lifespan of a product by sharing new and remanufactured modules. The sustainability was mathematically modelled by considering the three pillars of sustainability: economic, environmental and social performance. Also, Wang et al. (Reference Wang, Tang, Li, Yang, Salido, Giret and Zhu2019) presented an optimization model to determine product family design and remanufactured products’ configuration designs to maximize the total profit and the total GHG emission from production.
Kwak (Reference Kwak2018) proposed an optimal way to differentiate new and remanufactured products’ configuration designs that can overcome the effects of potential cannibalization of new product sales and technological obsolescence of used products, which are barriers to remanufacturing, while maximizing total profit and market share.
Ma & Kremer (Reference Ma and Kremer2015) provided a systematic method for evaluating the EOL options of components considering the sustainability and subjective perception of the designer’s sustainability. Cong, Zhao & Sutherland (Reference Cong, Zhao and Sutherland2019) proposed a methodology to determine recovery strategies for end-of-use components and optimal new product design to improve product recyclability for circular economy.
Wang et al. (Reference Wang, Wang, Mo and Tseng2017) showed the impact of component commonality decisions in product family design on component reuse in remanufacturing with respect to introduction time of the second generation of product family and durability of components. Kim & Kim (Reference Kim and Kim2019) applied the benefits of generational commonality to prolong the lifespan of components by sharing components between successive generations. This study investigated the impact of generational commonality of short-life-cycle products in manufacturing and remanufacturing processes.
Mesa, Esparragoza & Maury (Reference Mesa, Esparragoza and Maury2018) proposed a set of indicators to measure sustainability performance in product families for a robust framework for sustainable product design. The indicators were expanded to cover product families, unlike those for a single product that was presented in previous studies, which are mainly classified as sustainability performance indicators and functional performance indicators. Such indicators are measured sustainability of product families that encourages the implementation of the circular economy model.
This recent literature demonstrates that managing product lifespan is a relevant issue. The highlights above provide insight into the breadth and depth of lifetime management. While the insights come from observations of recent case studies, there are also other interesting research questions and opportunities. Section 5 identifies and expands on a new set of research challenges.
5.3 Understanding users and usage
5.3.1 Context
Understanding users and usage contexts is hugely important for making sure that designed products and services are effectively ecodesigned. Due to various restrictions and limitations, companies usually have little information available to answer the following questions:
(i) What is the average (or distribution of) product lifespan? Are products always as reliable and used as much as expected?
(ii) Are products disposed of due to real obsolescence or perceived obsolescence?
(iii) What are the reasons for EOL: not repairable, repairable but too expensive, and real/perceived/planned obsolescence? Does the market assign some value to a second life of the product? Are the products properly returned by users and effectively collected for adequate dismantling and recycling?
(iv) Do users properly maintain their products, i.e., follow maintenance guidelines?
(v) Do users responsibly eco-operate their high-energy-consumption products?
But the sustainability property of a product or service often surfaces later on in real usage, which mirrors the durability and recyclability conditions with a high degree of variability and uncertainty. This is why product sustainability requires investigation into users, usage situations and product usage modes.
5.3.2 Analysis of the case studies
Usage scenarios are known to be made of usage context and user profile to simulate product performance in the specific issue settings described above in the questions on modelling and simulating product lifespans, perceived or real/planned product obsolescence, product maintenance, product use and reasons for product end of use and product disposal. Each of these issues warrants consideration to assess the environmental impact of a product line in its category and to determine whether a product family is ecodesigned. These issues appear in Figure 7, where the 10 case studies are positioned. Note some cases deal with maintenance and obsolescence considerations. There is explicit reflection on lifespan in 6 out of the 10 case studies, in accordance with Section 4, while EOL and disposal are considered in 5 case studies. However, 8 of the 10 case studies explicitly give focus to user habits and how they influence the environmental impacts of a product or system, revealing a real focus for research.

Figure 7. Main concerns dealing with usage, and position of the 10 case studies.
Most of the 10 case studies presented in this paper directly consider users and usage. However, we can distinguish two different perspectives. The first considers end users, consumer behaviours and preferences. The approaches concerned deal for example with user observation, preference elicitation and statistical models in order to make products more sustainable regardless of their usage context. This is shown in case studies 3, 5, 6, 7, 8, 9 and 10.
The second is a manufacturer (or company) perspective, as shown in case studies 2 and 4, where the companies consider client data and market conditions. This perspective therefore deals with more collective, large-scale user and usage data in the intermediate value-chain stage.
In the automotive axle case study (Case 3), both new and remanufactured parts (CV joints) are available to customers, at different prices (i.e., cheaper for remanufactured parts). For CV joints, consumer preference was captured through market demand data in Korea. Remanufactured CV joints bring environmental benefit, but at lower profit as remanufactured CV joints are cheaper to buy. Current market data on sales prices of new and remanufactured CV joints shows that without regulatory subsidization, pursuing remanufacturing business is beneficial for the environment at the expense of profitability. Market sensitivity analysis in Case 3 shows how customers’ preferences affect potential business strategy and regulatory policy.
In the forge furnace case study (Case 2), different customer profiles were modelled to study the variability of potential environmental impacts in different operation contexts. Three profiles were considered, from a customer with no environmental stakes and a short-term economic vision to another considered as an environmental champion with a long-term economic vision, and a third profile midway between the two. These profiles impact the importance customers give to the costs (CAPEX and OPEX) and different environmental categories (global warming potential, ozone layer depletion, human toxicity, fossil depletion, and recycling/reuse). Even if these profiles are theoretical, results show that the manufacturer would recommend different decisions on the best technology to choose according to these profiles, showing the importance of considering industrial usages in the exploitation of industrial systems.
5.3.3 Confirmation with the literature
In addition to the cases described above, the recent literature confirms the importance of understanding users and usage for sustainable design. Below we cite the most notable recent works on this topic.
The recent scholarship has started modelling user habits. Green et al. (Reference Green, Tan, Linsey, Seepersad and Wood2005) emphasized the importance of understanding and representing usage contexts for simulating a product’s design performances. Yannou et al. (Reference Yannou, Chen, Wang, Hoyle, Drayer, Rianantsoa, Alizon and Mathieu2009) and He et al. (Reference He, Chen, Hoyle and Yannou2012) showed how to identify a series of key variables for featuring usage scenarios that influence performance and utility. This new usage-centred market segmentation approach was used to build a market share prediction model for a hybrid car model by the choice modelling technique in He et al. (Reference He, Chen, Hoyle and Yannou2012) and by collecting human appraisals in different usage contexts, which was named usage-context-based design. Yannou et al. (Reference Yannou, Yvars, Hoyle and Chen2013) proposed the usage coverage model based on physics-based performance simulations under usage scenarios. Constraint programming techniques were used to compute usage coverage indicators under physical and behavioural uncertainties and usage segment domains.
Tuarob & Tucker (Reference Tuarob and Tucker2015) presented an automated approach to lead user product-feature identification. Their study assumed that the product features desired by lead users do not yet exist but will be desired by the general customer. It is also assumed that the lead users can be found through social media. The authors collected social media messages from the United States during a period of 31 months to determine whether lead user preferences are relevant to next-generation cell phone designs.
Zhou, Jiao & Linsey (Reference Zhou, Jiao and Linsey2015) proposed a two-layer model for latent customer needs elicitation through use case reasoning. The first layer is to identify explicit needs from online product reviews. The second layer is to identify implicit needs by reasoning the semantics of use cases.
Fuge & Agogino (Reference Fuge and Agogino2015) led a study on identifying appropriate design methods to uncover user needs and preferences in an unfamiliar context, especially in developing regions. They applied a series of statistical techniques to learn how user research methods are used in practice in the developing regions, and found that certain methods are consistently used in certain types of problems and that certain methods complement each other.
Wang & Chen (Reference Wang and Chen2015) introduced a data-driven network analysis based approach to predict individual choice sets for customer choice modelling in engineering design. The analysis consists of the following major steps: (1) classify customers into clusters based on their profile attributes; (2) construct a product association network that reflects the similarity of products in customers’ perceptual space; and (3) predict choice sets for individual customers using a probabilistic sampling approach.
Zaraket et al. (Reference Zaraket, Yannou, Leroy, Minel and Chapotot2015) proposed an activity-based model for forecasting household energy and water consumption and discussed how such an occupant-focused model could integrate a user-focused design for residential buildings. The consumption prediction is closer to reality compared to the standard averaged energy consumption profiles.
Cor et al. (Reference Cor, Domingo, Brissaud and Zwolinski2014), Cor & Zwolinski (Reference Cor and Zwolinski2015) developed a protocol to integrate user behaviour into the ecodesign of low-complexity consumer products. The protocol is composed of the following steps: (1) identify critical environmental aspects in use; (2) support designers to select a design strategy for sustainable behaviour after analysis of the use phase; and (3) test the selected strategy with product-in-use observations. Polizzi di Sorrentino, Woelbert & Sala (Reference Polizzi di Sorrentino, Woelbert and Sala2016) also used behavioural science to better model use phase in LCA and come up with realistic scenarios as basis for a behaviour-driven ecodesign and eco-innovation.
Sabbaghi & Behdad (Reference Sabbaghi and Behdad2017) investigated the impact of component deterioration profiles and consumer repair decisions on device lifespans and assessed the anticipated life-cycle environmental impact. The life-cycle characteristics were estimated by Monte Carlo simulation. In the same manner, Popoff, Millet & Pialot (Reference Popoff, Millet and Pialot2016) investigated the impact of usage ecodrifts, i.e., nonoptimal use of a product by the users, on energy overconsumption (real-time impacts) and abnormal wear and tear of parts of the product (delayed impacts).
Many studies have explored the effects of behavioural changes while the development of digital devices has increased (Pothitou, Hanna & Chalvatzis Reference Pothitou, Hanna and Chalvatzis2016). Others have studied how design could be used to influence consumer behaviour towards more sustainable practices. For instance, Lilley (Reference Lilley2009) proposed three strategies to incentivize sustainable behaviour: eco-feedback, behaviour steering and persuasive technology. The expression Design for Sustainable Behaviour (DfSB) is used by Boks (Reference Boks, Matsumoto, Umeda, Masui and Fukushige2012) and Daae & Boks (Reference Daae and Boks2015).
Picon et al. (Reference Picon, Yannou, Zaraket, Minel, Bertoluci, Cluzel and Farel2013) proposed a principle of residence-level mapping and storing of household consumption data as well as indications on geo-localized complaints on a residence map. This mapping may be useful for monitoring energy and water consumption of any household in (real) time and comparing it to that of comparable households for potential renovation projects by building designers. Capturing a household’s electricity and water usage data in the cloud or organizing a residence memory may in return provide useful information feedbacks to the consumers themselves. All the actors – residents, building administrator, construction/renovation company – need to voluntarily adopt the proposed renovation and design for the next generation of buildings. Kuo et al. (Reference Kuo, Tseng, Lin, Wang and Lee2018) used smart meters to provide energy information on real-time consumption and explore energy consumer behaviour with socio-demographics, pro-environmental behaviour and goal-directed behaviour.
We are undoubtedly at the beginning of an era in which data will be managed for designing adapted and energy-frugal products (i.e., zero energy use) and for monitoring product usage phases. This takes us to the next subsection.
5.4 Integrating data and analytics
5.4.1 Context
Data from various life-cycle stages of products and systems are becoming readily available, and data analytics is now more critical than ever for sustainable design. Measuring performance along the three sustainability pillars is becoming more affordable due to advancements in sensor technologies, which in turn leads to further improvement in sustainable product and system designs. Several approaches, such as carbon footprint measurement, LCA, LCC, activity-based costing, social life-cycle assessment or hybridization of tools, all provide useful solutions. However, the downside of measuring sustainable performance is that it creates issues with acquiring, managing and analysing the data. The main issues in dealing with data are charted in Figure 8, where the case studies are positioned according to the analysis proposed below.

Figure 8. Main problems arising from data acquisition in the 10 case studies with different approaches (LCA, value-chain assessment or other types of eco-evaluation).
Life-cycle decisions usually integrate the entire life cycle of a product, service or system. In order to support such decision-making processes, several kinds of information need to be collected throughout the life cycle. First, bills of materials are a requisite for assessing the environmental impact of a system. Nature and amount of materials contained and consumed or emitted by the system are used to calculate the environmental impact. Cases 1, 2, 3, 5, 6, 8 and 9 evaluated this environmental impact, as all of them performed LCAs. In an open and global market, where components come from several locations to be assembled and where systems are sold in other locations, it is difficult to know the exact material content of components and the associated manufacturing processes. The information required essentially spans raw material extraction through to manufacturing life-cycle stages. Traceability and data exchange between stakeholders, e.g., producers/suppliers, suppliers/suppliers or producers/end users, is a prerequisite for more systematic evaluation. The next subsection briefly summarizes the case studies in terms of data and analytics context and then looks at other recent papers on this topic.
5.4.2 Analysis of the case studies
Data traceability is particularly challenging when it involves consumer consumption and demographic data. This issue is particularly significant for food production and its final clients (Case 7). One of the main challenges revolves around how to share confidential information and sustainable benefits between economic partners in a value chain or their stakeholders (Sarkis, Zhu & Lai Reference Sarkis, Zhu and Lai2011; Grimm, Hofstetter & Sarkis Reference Grimm, Hofstetter and Sarkis2014). The issue of data availability is a common thread across all the life-cycle stages, including packaging, transport, usage and EOL management. Public or commercial databases usually help overcome the data availability issue by providing specific life-cycle inventories (Finnveden et al. Reference Finnveden, Hauschild, Ekvall, Guinée, Heijungs, Hellweg, Koehler, Pennington and Suh2009). This practice will gain more traction in coming years thanks to increased awareness of environmental issues, animal well-being, local production or sustainable fair trade. In order to accommodate the demand for more transparency and environmental information, industries are increasingly leveraging environmental communications instruments such as ecolabels (Thøgersen, Haugaard & Olesen Reference Thøgersen, Haugaard and Olesen2010). These approaches force them first to evaluate their own products and processes, second to communicate with their economic partners and third to generate and disseminate up-to-date information.
Although data may be accessible for existing systems, acquiring massive-scale data is still bottlenecked (Reap et al. Reference Reap, Roman, Duncan and Bras2008; Pryshlakivsky & Searcy Reference Pryshlakivsky and Searcy2013), chiefly by the static aspect of the data, the lack of knowledge or detailed information about some life-cycle stages and relevancy when moving from generic to specific data and from global to local context.
The static aspect of data generated by most of the existing tools and approaches and the major data collection effort required for tackling sustainability issues are often sources of data inaccuracy. Information may be obsolete and limited to a specific system, area or operational conditions. In addition, as collecting information is expensive, it is not unusual to only have access to a single value for one parameter instead of a complete statistical dataset (Leroy & Froelich Reference Leroy and Froelich2010). As a result, uncertainty is still high, which has prompted practitioners to develop several strategies to overcome the uncertainty. In Case 1 for example, where the lifespan of the substation and its use phase are uncertain, the authors performed scenario-based analysis to capture the range of environmental performances (Cluzel et al. Reference Cluzel, Yannou, Millet and Leroy2014). The use of expert data, assumptions, estimations and approximations is also common practice to overcome data gaps. The life-cycle inventory, i.e., the energy and material streams consumed and emitted by the system during its entire life cycle, for example the oxy-fuel system in Case 2 or the profitability model in Case 3, is also based on estimations.
These practices stem largely from an undercomprehension of specific life-cycle phases or influential parameters. While upstream phases are well understood and documented, the use phase and the EOL phase require more understanding. Case 9 addresses this issue by proposing a stochastic-activity-based model to evaluate inhabitants’ domestic water and electricity consumption. By combining 29 daily activities, the SABEC model integrates the occupants’ socio-professional attributes, electrical appliance ownership and performances and finally the service unit per activity. This offers a new way to characterize users’ behaviours instead of considering them via a daily occupancy rate or adopting an averaged behaviour (Zaraket et al. Reference Zaraket, Yannou, Leroy, Minel and Chapotot2015). Case 7 also highlights the importance of getting more information on consumers as they have a huge influence on value-chain performances. This issue is shared among most of the case studies. Cases 1 and 2, for example, envision the way the systems operate according to geographic location (Cluzel et al. Reference Cluzel, Yannou, Millet and Leroy2014; Leroy et al. Reference Leroy, Cluzel and Lamé2014). Sustainable performances are connected to resource availability, accessibility and prices.
Finally, the use of generic data may undermine the accuracy of results. In all the cases except 3 and 5, specific context knowledge was crucial to define an optimal system architecture, as was the case for understanding usage behaviours (Cases 3, 8 and 10). Indeed, in these studies, more detailed and specific information is needed because sustainable systems are highly end-user-dependent. Generic information is usually collected from surveys, and estimations or expert judgement are often too vague and insufficient to capture the variability in performance introduced by users. Geographic location is also an extremely important factor when investing in a specific technology. Cases 1 and 2 highlight different trade-offs between economic and environmental impacts for the same system according to its geographical location. Factors such as regulations, environmental sensitivity, distribution of resource accessibility, availability and price, and clients’ awareness of sustainability play a major role in decision-making and are highly influential in sustainability performances. These factors must be characterized and documented as much as possible, which was found to be critical in Cases 4, 6, 7 and 9, where the subject under study is assessed or designed in a specific geographic context. The automotive recycling value chain and the pork value chain are both located in France. Most of the stakeholders have to comply with the same regulations, work with homogeneous production conditions and exploit or use EOL management. Case 6 requires the integration of real EOL performances per country, e.g., waste collection rate and recycling and incineration efficiency, in order to allow comparison between five European countries. Finally, Case 9 needs to characterize the direct building environment to define and assess the externalities and choose the most promising solutions.
5.4.3 Confirmation with the literature
Key issues identified for data and analytics are data availability, accuracy and uncertainty. In addition to summarizing the case studies presented here, we also cite recent sustainability literature on data and analytics to show the universal nature of the issue.
Chen & Fuge (Reference Chen and Fuge2017) proposed a data-driven adaptive sampling technique called $\unicode[STIX]{x1D700}$-margin sampling in an attempt to identify feasible domains in an unbounded design space. In comparison with random and active sampling, the advantage of
$\unicode[STIX]{x1D700}$-margin sampling is that the data is efficient due to the principled probabilistic trade-offs. Furthermore, this adaptive sampling technique manifolded with a coupled design can avoid the exponential increase of samples with increasing scale in real-world designs.
Ramanujan et al. (Reference Ramanujan, Bernstein, Chandrasegaran and Ramani2017) reviewed research into using visual analytics (VA) tools in sustainable life-cycle design. Sustainable life-cycle design needs product life-cycle data for the environmental impact assessment and human expertise for the design changes. VA tools are the platform needed to combine data-driven and user-driven methods.
Luo, Yan & Wood (Reference Luo, Yan and Wood2017) developed a data-driven VA system called InnoGPS, borrowing an analogy from global positioning systems, which allows designers to set their own innovation position and directions in the technology space according to the technologies that they can design in the pool of 5 million technological patent records.
Huang, Kwok & Zhou (Reference Huang, Kwok and Zhou2017) developed a novel algorithm called Volumetric 4-Points Congruent Sets (V4PCS) for global registration that finds an optimal rigid transformation to align two three-dimensional (3D) shapes without any assumptions on their initial positions. The alignment enables the desired reuse information for similarity identification in data-driven designs and engineering applications such as quality control and mass customization. The authors claim that compared to Super 4-Points Congruent Sets (S4PCS), their algorithm greatly reduces computational complexity by incorporating volumetric information from 3D models.
Suryadi & Kim (Reference Suryadi and Kim2018) investigated the relations between online customer reviews and sales rank, aiming to identify the design features significantly related to sales rank. Their proposed methodology essentially consists of the following stages: (1) produce product-feature words by employing a combined word embedding–X-means clustering approach; (2) identify sentiment words and their intensity, and connect feature with sentiment words using a dependency tree; (3) determine the features that significantly affect sales rank. This methodology was successfully applied to wearable technology products and laptops.
Ma & Kim (Reference Ma and Kim2016) proposed a model for predictive data-driven product family design that expands clustering-based approaches to incorporate a market-driven approach. The execution of the model will determine optimal product family architectures with customer preference data.
Ma & Kim (Reference Ma and Kim2014) developed a new predictive trend mining algorithm for product and design analytics. Compared to a traditional static data mining algorithm, the new algorithm can dynamically extract valuable customer knowledge over time with higher prediction accuracy, while enabling dynamic data selection, avoiding overfitting, identifying model performance and allowing numerical prediction. The algorithm is demonstrated on tablet PC design.
Lim & Tucker (Reference Lim and Tucker2017) proposed an unsupervised model to mitigate online product rating biases. These biases come from the fact that different reviewers could give the same product a significantly different rating. To eliminate biases and identify true product quality, the study observed the significance of reviewers’ rating histories and tendencies. The model developed is thus based on reviewers’ history data instead of human-labelled training data.
Chaklader & Parkinson (Reference Chaklader and Parkinson2017) introduced a weighted phrase rating method to determine preliminary design specifications from consumer text reviews. They claim the method is applicable to larger review pools and provide useful information to help determine design specification in a faster and more economical way than traditional methods such as surveys, experiments and observational studies.
Ranscombe, Kinsella & Blijlevens (Reference Ranscombe, Kinsella and Blijlevens2017) developed holistic styling analysis (HSA) to help designers assess differences in product appearance objectively rather than by instinct and intuition. In contrast with traditionally abstract descriptions of appearance, digital shape comparison tools work on 3D product geometries and generate data on product shape.
Under the theme of ‘data-driven design’, the data and analytics area is gaining more attention among design community scholarship. For sustainability research, data and analytics is set to take on an increasingly prominent role to overcome the challenges tied to data availability, accuracy and uncertainty.
5.5 Defining system boundary and perimeter
5.5.1 Context
An ecodesign project consists of two major stages. The first stage involves analysing the environmental performance of the existing system (before the one we want to ecodesign), and the second stage is to design new solutions, assess their environmental impacts and then select the solutions that contribute to the project strategy (AFNOR 2013). The element that links these two major stages of an ecodesign project is the model used to represent the system to be ecodesigned. This model is an assembly of unit processes. Its environmental performance depends on how these unit processes represent the reality of the system evaluated but also on which of these processes or their input–output flows the modeller chooses to include in their model. Indeed, the set of these unit processes describes both what the system is and the input and output flows that its life cycle generates. The analysis systematically involves clarifying both what system we want to improve and the context – the environment – in which it operates.
LCA is one of the methods used on a microeconomic scale to increase system eco-efficiency. In terms of objectives associated with LCA modelling, the European Guide (European Commission 2010) defines three kinds of possibilities that correspond to different decision-context situations of relevance in LCA to gauge whether the design study helps to support a product or strategy decision. The three possibilities are: (1) a design change on the product that does not modify production capacities or characteristics, (2) a design change that modifies production capacities/characteristics and consequently the company’s business model and (3) the focus is on methodological issues, which is generally for improving research or public policies.
As stated by Frigg (Reference Frigg2003), a model is only an artificial representation of reality whose characteristics are defined according to the modeller’s objectives. In parallel, a model is an intermediate and reflexive design object, not just for the modeller but for the whole project team (Boujut & Blanco Reference Boujut and Blanco2003). In this sense, the information that is gathered to create the model and that emerges from running it adds to the modeller and project team’s knowledge. This new knowledge leads them to gradually grasp situations of increasing complexity not only within the project itself but also from project to project. In the case studies analysed here, we show ecodesign induces continuous meso-/macroscale changes related to the observation of the interactions of the studied system with its environment. The analysis of these interactions, particularly in the case of consequential LCA, implies considering other dimensions beyond the environmental pillar alone when judging the project sustainability, and thus to extend the perimeters considered for modelling.
5.5.2 Analysis of the case studies
Here we show how the changing system perimeter surfaces in case studies 1–10 from different environmental, economic and social-dimension perspectives, and then give more detailed descriptions of case studies 5 and 6. These perspectives are shown in Figure 9.

Figure 9. Main factors influencing the perimeter of a study and raised by certain case studies.
The nature of the system analysed and the objectives of the study often modify the perimeter and criteria integrated in the model. As with many LCA-based studies, assessment of technical systems for professional use tends to only account for the environmental criterion (Case 1) (Sarkis et al. Reference Sarkis, Zhu and Lai2011; Brandenburg et al. Reference Brandenburg, Govindan, Sarkis and Seuring2014). However, some studies show that a better understanding of user practices and context could help to evaluate the performances of these technical systems differently (Case 2) (Chapotot et al. Reference Chapotot, Abi Akle, Minel and Yannou2011; Abi Akle et al. Reference Abi Akle, Bertoluci and Minel2013). Accounting for realistic user behaviours becomes even more important when assessing the impacts of products and systems in direct relationship with consumers (Case 6) or individuals (Cases 9 and 10). Taking users’ actual behaviours into account can completely change a preferred design solution by measuring appropriate sustainability performances.
Moreover, it is vital to consider a solution’s economic consequences for all stakeholders as an element of the system’s sustainable performance in order to establish the financial sustainability of the solutions evaluated (Shi et al. Reference Shi, Wang, Fan, Mo and Jin2018; Zhang et al. Reference Zhang, Dong, Jin, Li and Ren2019). The economic aspect is considered for product analyses on mass-market sustainability (Cases 2, 3 and 10) (Heise & Theuvsen Reference Heise and Theuvsen2014; Baudry, Macharis & Vallée Reference Baudry, Macharis and Vallée2018) and also in more global approaches dedicated to value-chain analyses (Cases 4 and 7) (Carter & Rogers Reference Carter and Rogers2008; Petit et al. Reference Petit, Yannou-Le Bris, Trystram, Lallmahomed and Cadiz2017a; Petit, Yannou-Le Bris & Trystram Reference Petit, Yannou-Le Bris and Trystram2017b,Reference Petit, Yannou-Le Bris and Trystramc). In particular, the inclusion of the life-cycle players’ own economic evaluation (calculated margins or production costs) is a supporting element informing public policy decision-making (Cases 3 and 4).
Finally, models developed for sustainability assessment increasingly need to integrate the social dimension, especially for mass-market products. This social assessment introduces a new challenge for modellers, as it involves a wide range of indicators concerning not only the specific raw materials used and the social and economic difficulties of the countries in which they are sourced, but it also engages the complexity and opacity of the industrial sectors they belong to and, ultimately, of the individual end users’ expectations.
Systems perimeters are increasingly being extended in an effort to increase the sustainability of systems in their use context. An inadequate perimeter definition can result in a solution that is not ultimately sustainable, even if it seems to be the better solution in the initial evaluation. The case studies feature examples illustrating this potentially misleading simplification. Case 5 (cotton harvesting) is a case in point. A comparison limited to only the two machines would show that a newer machine has higher environmental impact due to its heavier mass and higher fuel consumption. However, as cotton harvesting involves multiple tasks, a machine-versus-machine comparison would be too narrow to produce a fair environmental impact assessment. The real need is to compare two cotton harvesting ‘systems’ composed of other pieces of equipment such as tractor and transportation system. Therefore, extending the perimeter by including all relevant pieces of machinery allows a fair comparison of environmental impacts.
A second example is Case 6 that compares the environmental impacts of three different types of olive packaging (glass, metal box and multilayered plastics). Assessments were conducted according to country-specific performances (five different European countries considered) where their EOL stages are treated and valorized. An environmental evaluation of the packaging would identify plastic as the solution with the lowest environmental impact. The advantages of plastics are seriously diminished when the assessment perimeter encompasses the impact of consumer behaviours. The study conducted on French consumers showed that plastic packaging increases olive waste rates. After considering the impacts of food waste by each packaging, the initial worst environmental solution (glass) ultimately emerges as the better global option.
5.5.3 Confirmation with the literature
Ongoing development of LCA practices and knowledge is progressively redrawing the perimeters of product and system sustainability studies. Developments in telematic systems and analytics capabilities now make it possible to forecast different scenarios of possible future life cycles. Consequential LCA (CLCA) is thus growing in relevance, as it serves to compare the environmental impacts of different scenarios. This trend requires the creation of scenarios that include probabilistic data. It also requires a clear definition of the objectives of the modelling in order to clearly define the perimeter of the model. The credibility of future research should be based on the robustness of the parameters considered in the scenario and the statistical representativeness of the data used in the model of user behaviours.
Plevin, Delucchi & Creutzig (Reference Plevin, Delucchi and Creutzig2014) pointed out that the simplification inherent to attributional LCA (ALCA) rules out using ALCA results as evidence for policymaking. In contrast, CLCA is better, as CLCA models actual real-world change and CLCA results are scenario-dependent.
Yang & Heijungs (Reference Yang and Heijungs2018) reviewed the major assumptions of LCA models (linear and nonlinear optimization models) and found that linear models assume fixed coefficients and unlimited input supply whereas nonlinear models like computable general equilibrium (CGE) assume rationality. The authors also question whether LCA estimates are verifiable or falsifiable. They concluded that the estimates are largely unverifiable due to the complexity of human–environment system interactions. Their suggestion for policymakers is to check the convergence of different models. If estimates from models point to the same direction, then it is highly likely that the LCA predictions are sound.
Kawajiri et al. (Reference Kawajiri, Ihara, Hatayama and Tahara2018) developed a novel analytical framework to visualize the environmental impacts of customer consumption patterns in a CLCA setting. They found that some products/services, e.g., meat and dairy products with relatively low $\text{CO}_{2}$ intensities, have high net
$\text{CO}_{2}$ emissions per unit price.
Khoo, Sharratt & Isoni (Reference Khoo, Isoni and Sharratt2018) highlighted that LCA estimates are highly dependent on the relevance and accuracy of data selection in the chemical industry. Eight criteria were suggested and tested on 12 chemicals/solvents by a multidisciplinary research team.
Sevigné-Itoiz et al. (Reference Sevigné-Itoiz, Gasol, Rieradevall and Gabarrell2015) were conscious of the challenge of taking pro-environmental decisions for waste management when the system boundary is the international market. They integrated material flow analysis (MFA) and CLCA to examine the consequences of increasing paper and cardboard recycling on GHG emissions in Spain.
Vázquez-Rowe et al. (Reference Vázquez-Rowe, Marvuglia, Rege and Benetto2014) developed a combined CLCA–partial equilibrium approach to evaluate the environmental impact of Luxembourg attaining 14% bioenergy in the national grid by 2020 [which is a European Union (EU) objective]. The proposed method considered the impact of land use change due to maize production for energy and different consequential decision contexts.
These are a few examples from the recent literature that showcase the depth and breadth of research on rethinking changes to system boundary and perimeter and on LCA-related uncertainties across different regions and countries.
6 Discussion of the main research challenges
Our analysis of the case studies and their findings led to a shortlist of five big issues for ecodesign (Section 5). After a reflection on the five ecodesign issues, we go on to list the main research challenges in pursuing them. These challenges are grouped into three categories – short term, medium term and long term.
6.1 Reflection on the five ecodesign issues
In light of the 10 case studies, and in accordance with Pigosso et al. (Reference Pigosso, Mcaloon and Rozenfeld2015), the five ecodesign issues presented earlier offer promising research perspectives. Some have already been amply explored in detail, while others remain ‘future’ research areas. Without pretending to be exhaustive, as no additional issues or themes emerged from our analysis of the 10 case studies (themselves chosen for their diversity), we feel that these five issues offer a good overview of current trends in ecodesign.
Table 2 gives a roll-up of how each case study deals with the five issues. Some of the case studies deal with all the issues, while others only address some of them.
Ecodesign research has made huge strides forward over the last couple of decades. As shown in Table 1, its spans a variety of focal areas with applications from single products to large-scale industrial complexes. Optimal resource use and internalizing environmental impact consideration are ubiquitous in all the case studies, and they have a wealth of methods and tools available to choose from. Much of the research effort has been devoted to developing a new set of sustainable design methods and tools for separate application areas, and the complexity and intricacy of the methods devised is increasingly prominent in various cases. While the concept of sustainable design is pervasive to many domains, the adoption and implementation of sustainability is less visible in many industrial practices.
A more in-depth analysis of the topics raised in the case studies has led to the emergence of other research questions. Our list of emerging research areas may not be exhaustive, but it is based on a deep understanding of the case studies and expert interpretation of the findings. We identified six major areas that are still key challenges when trying to make ecodesign more accurate and more widely disseminated: massive adoption of existing ecodesign methods and tools by industry, development of long-term industrial vision by companies, integration of environmental aspects to the same level as economic considerations, expanding the sustainability spectrum by integrating the social dimension, data and design analytics integration and wider adoption of the systems perspective. These fields are shown in Figure 10 and are detailed in the following paragraphs.

Figure 10. Future research agenda for sustainable design.
6.2 Short-term challenges
Slow uptake by industry may be due to lack of economic rationale and difficulties using the tools available. Adding the economic aspect and making more tools available is relatively straightforward to implement, and is thus considered a short-term challenge.
First, in most industrial practices, sustainability often requires a long-term perspective, which may not necessarily translate into immediate economic value (i.e., profitability). Short-term thinking for profitability often trumps long-term sustainability vision. In Europe, like elsewhere in the world, despite the development of environmental regulations, design decisions are inextricably linked to the short-term economic benefits they bring. Ecodesign methods that focus on economic viability could improve this situation. Most research has started with methods and tools that improve the environmental performance of product or systems, i.e., reduction of the environmental impact, often at the expense of economic profitability. Recent advances in ecodesign methods considering better economic rationales will ensure both economic and environmental improvement in sustainable design practices.
Second, tools for ecodesign are not readily integrated in product design practices (Stark et al. Reference Stark, Buchert, Neugebauer, Bonvoisin and Finkbeiner2017). For example, LCA tools are more readily available for product and service environmental impact assessments. However, the existing LCA tools often require an exhaustive data gathering step, analysis of product bills of materials and setting numerous parameters spanning manufacturing, usage, maintenance and end-of-use recovery. This type of data and information is often unavailable at the design stage, even before it becomes an additional barrier to the adoption of new tools by design engineers. Making the tools easy to implement and adopt will be essential for a wider adoption of ecodesign practices.
6.3 Medium-term challenges
A strong approach to sustainability is proposed by the field of ecological economics (as pioneered by the work of Daly (Reference Daly1990)). In this approach, sustainable development leads to zero compensation between the three pillars of sustainability (economic, social and environmental). In other words, creation of technical value cannot be allowed to compensate for a loss of natural resources. This sustainable development paradigm requires harvest rates to equal regeneration rates and waste emission rates to equal the natural capacities of the ecosystems to absorb these wastes, and natural capital and human-made capital are conjointly managed at optimal levels under which they can be maintained over a very long time. The approach also demands no compensation between the environmental damage caused by a system and the economic value that it creates.
If we are to consider that each pillar of sustainability must be protected, how do we define the perimeter of the analysed system in order to understand these exchanges of impacts between damage to the natural ecosystem, society and the living beings that make it up? This kind of objective necessarily implies measuring the indirect environmental impacts caused by the ecodesigned system, and it also implies addressing the other dimensions of sustainability.
Among the three pillars of sustainability, the economic and environmental aspects have been widely investigated in many design cases, although these two aspects are often modelled separately rather than in an integrative manner (Kim & Moon Reference Kim and Moon2017). An integrative modelling of both economic and environmental dimensions is therefore a challenge for industry to adopt sustainable design as a predominant factor in the long haul. At the design stage of products or systems, the two competing objectives – economic and environmental – need to be co-considered simultaneously in an integrative manner. A good starting point may be a multi-objective optimization approach, where a Pareto frontier is constructed for simultaneous consideration of both objectives rather than a sequential approach.
Also, for the reasons mentioned earlier, models developed for sustainable assessment increasingly need to integrate the social dimension, especially for mass convenience goods products. This social assessment introduces a new challenge for modellers as it involves a wide range of indicators, not only on the specific raw material used and the social and economic difficulties of the countries in which they are sourced, but also because of the complexity and opacity of the industrial sectors they belong to and, ultimately, of the individual end users’ expectations. This third pillar has been underinvestigated in the design context, possibly due to the difficulty in quantifying the social aspect of sustainable design and translating it into design practices. In line with the user-centred sustainable design paradigm, the social aspect should be more widely adopted in design practices (Kim & Moon Reference Kim and Moon2017). Once a quantitative model of the social dimension is available, integrating the objective as an additional input to the dual-objective formulation (economic and environmental) is straightforward. In this case, the Pareto frontier becomes a 3D space to explore.
Modelling each objective and integrating them requires more than adopting/implementing existing tools, as noted in our section on short-term challenges. Modelling therefore demands a long-term industrial vision and a development effort, thus posing a medium-term challenge.
6.4 Long-term challenges
Data generated by customers are now more readily available, and the effort to understand the data is becoming more prominent in many sustainable design methods. Also, the scale of product or system should be expanded from a single product or system to large-scale ‘system of systems’, and a fresh look at system boundary and perimeter should be pursued to link B2B relationships in addition to B2C relations. For some mass-produced products, the ability of traceability/telematic systems to transmit specific information (location of production steps, animal feeds in livestock, working conditions in textile mills etc.) is expected to become prevalent in the future, and thus become part of an expanded scope of sustainable design.
One of the key decisions in managing the lifespan of a product or system is how to determine the lifetime in economic and environmental terms. Rather than following a uniform set of scheduled maintenance operations, for example, each machine or system will be able to follow individualized diagnosis and prescriptive maintenance treatment for end-of-use decision-making. As a result, sustainable design may become more customizable and pervasive in a variety of products and systems.
Design analytics is a new way of designing a product or system in conjunction with data analytics and systems design (Ramanujan et al. Reference Ramanujan, Bernstein, Chandrasegaran and Ramani2017). Analytics capability will benefit sustainable design in the following ways. First, design analytics will enhance the economic aspect of sustainable design. Design engineers will be able to detect user preferences and changing market dynamics much more accurately than before. As a result, the manufacturer can initiate product take-back in an optimal time window where the potential economic benefit is maximum. Second, the environmental aspect will also benefit from analytics capabilities. As products and systems age (Guide et al. Reference Guide, Souza, Van Wassenhove and Blackburn2006), their performance tends to degrade and so their environmental impact usually increases. Analytics could help retire the product at the right time, long before it reaches an obsolete and environmentally inferior state (Bras Reference Bras2014), which would in turn reduce the environmental impact.
Research efforts in sustainable design have shown great potential with a wide range of potential application areas in consumer electronics, heavy-duty equipment, industrial complexes, agriculture and food chain networks, as shown in the case studies. While the traditional ‘design for environment and sustainability’ started with a single product or system, the observations in this paper highlight a large-scale systems approach to the research effort. The systems perspective provides an approach for handling trade-off situations where sustainable system design can benefit one component of a system economically but not ‘environmentally’. Also, one design may be sustainable at a given point in time but become less so as the time horizon evolves, as was shown in the case dealing with the time-varying value of remanufacturing (Aydin et al. Reference Aydin, Kwong and Ji2015, Reference Aydin, Kwong and Ji2016; Bobba et al. Reference Bobba, Ardente and Mathieux2016; Kwak Reference Kwak2016; Iraldo et al. Reference Iraldo, Facheris and Nucci2017). Consideration of multiple systems and lifespans and the resource management aspect of sustainable design will require a new set of thinking in the area of sustainable design for long-term future research.
7 Conclusions and perspectives
Based on insights and findings from material presented here, we suggest that research efforts in ecodesign should be directed towards a set of topics raised in sections of this paper.
The 10 case studies summarized as illustrations of current ecodesign research projects do not exhaustively cover every topic, but they serve to highlight the major ecodesign issues covered to varying degrees in current research:
(1) Optimal resource use for less environmental impact
(2) Managing lifespan
(3) Understanding users and usage
(4) Integrating data and analytics
(5) Defining system boundary and perimeter
Working up from these issues, we identified new emerging directions: massive adoption of ecodesign tools and methods in industry, expansion of the sustainability spectrum, data and design analytics integration and adoption of the systems perspective. We see these directions as the next major challenges for sustainable design research.
Among these challenges, extending the sustainability spectrum is a crucial issue. Among the 10 case studies presented in this paper, integration of the social dimension is rarely explicit, other than in Case 7 (pork value chain, including animal welfare or farmer satisfaction indicators) or cases in the building sector (Cases 9 and 10, including social externalities or occupants’ social attributes). Integrating the social dimension to the same extent as the environmental and economic dimensions remains a major research perspective to deal with in the coming years.
Acknowledgments
The authors thank all the companies and institutions involved in the 10 case studies used in this paper. Any opinions, findings, conclusions or recommendations expressed in this paper are those of the authors and do not necessarily reflect the views of these companies and institutions.
Appendix A. Description of the case studies
All the case studies are detailed with more elements in standard breakdown sheets in a separate file available online at https://hal.archives-ouvertes.fr/hal-02084355.
A.1 Case 1 – Aluminium substation
An AC/DC conversion substation for aluminium smelters is a large electrical system that converts alternative current to direct current usable for aluminium electrolysis. Substations are characterized by huge environmental impacts linked to the complexity of the system [high number of subsystems and components, highly uncertain operational service phase (up to 30–40 years), EOL almost unknown, close links to the macrosystem (aluminium smelter), tailor-made design, multiple suppliers and more]. The general objective of this project was to develop and implement an adapted framework to ecodesign complex industrial systems, through three research questions:
(i) How to ecodesign complex industrial systems?
(ii) How to assess the environmental performance of complex industrial systems?
(iii) How to generate and select a powerful portfolio of eco-innovative R&D projects for complex industrial systems?
A.2 Case 2 – Forge furnace
Sustainability is an increasingly pivotal issue, especially when designing large energy-consuming industrial systems. As they are implanted worldwide, they are subject to high variability in terms of operational conditions and thus in economic, environmental or social impacts. Decision-making in such a context is a hard task.
The main objective of this study was to connect environmental assessment, economic evaluation and clients’ expectations while defining the optimal solution according to the geographical context. To do so, environmental impacts of three alternative burner solutions for a forge furnace were compared by LCA in four locations differing in terms of energy mix, fuel prices and accessibility. The economic evaluation is performed by combining CAPEX and OPEX. Finally, we addressed trade-offs between environmental gain and financial effort through three client expectation profiles.
The main objectives of this study was to highlight the high geography-dependent variability in environmental and economic impact.
A.3 Case 3 – Automotive axle
Remanufacturing is generally perceived as environmentally friendly, but it comes with an economic cost. The automotive axle case study is about a framework to assess economic effects and environmental impacts based on a remanufacturing industry case in Korea. Depending on the residual value of the product, there is a change in preference for a new or remanufactured for regular maintenance part. There is potential growth opportunity for the remanufacturing business, but only if there is compensation to offset the economic loss due to the low retail price of remanufactured parts. The research aims to identify what level of economic incentive should be provided to encourage remanufactured part use for regular maintenance and replacement of a major automotive part. The study entails the following tasks:
(i) Calculate economic and environmental impact based on estimated demand for replacement parts
(ii) Calculate the amount of economic subsidy required to compensate for potential loss of profit in pursuing remanufacturing business
A.4 Case 4 – Automotive recycling chain
Recycling end-of-life vehicle (ELV) glazing is defined as the process of dismantling, collection, storage, transport, treatment and ultimately reuse of recycled glass called cullet. The objective of this study was to model, simulate and size an ELV glazing recycling value chain or network to avoid landfilling 70,000 tons of glazing per year in France while (1) creating value with cullet production and (2) avoiding governmental penalties for each car glazing not recycled by a given date imposed by EU regulation. The profitability of such a network system was investigated along with its sizing – quality of cullet flows, type of glazing collection, type of transport etc.
First, a linear programming model was employed to obtain the optimal material flow of ELV glazing, going through the activity alternatives in the dismantling procedure, collection and storage sites, glass treatment units and the final cullet product. This is a scenario-based simulation of optimal recycling networks based on three variables: landfill cost, cullet price on the market and penalty per nonrecycled car glazing. Second, a system dynamics approach was used to simulate the net economic balance of the recycling network under different future scenarios.
A.5 Case 5 – Cotton harvesting
Cotton harvesting involves a series of tasks that have to be performed using a diverse set of machinery and equipment configurations. A new machine system is to be brought to market and a fair comparison is needed to guide product design and manufacturing decisions. Two systems (old vs. new) perform a common task (harvesting cotton crops) where the environmental impact is generated differently for each system. The objective of the project is to develop a comparative systems LCA framework to assess the environmental impact of multiple heterogeneous systems. The project is to answer the following research questions:
(i) How can we provide an ‘apple-to-apple’ comparison of the environmental impacts of two heterogeneous systems when each system has multiple constituent elements that contribute to the environmental impact?
(ii) How can we characterize a common task for two systems so that the output (environmental impact) is assessed for the same number of tasks to be completed?
A.6 Case 6 – Olive packaging
The EU advocates recycling as the preferred option for dealing with EOL packaging management. However, the recycling chains in each European country are not equally efficient. The main objective of this paper is to define the most sustainable packaging solution according to its material content and country of sale.
We thus compared the environmental performances of three different olive packaging options: a steel can, a glass jar and a doypack. First, we used LCA to gauge the environmental impacts. The LCA used bills of materials, transformation processes and generic recycling rates in French. Second, we considered five European countries and their respective waste collection and EOL recovery chain efficiencies.
Finally, the results were tested against consumer expectation. Thirty-nine questionnaires were collected and analysed to measure alignment between environmental assessment and consumers’ purchasing choices.
A.7 Case 7 – Pork value chain
Food consumers want more information on the origin and quality of the food they buy, including information on its origin, animal breeding conditions, substances absorbed during the animal’s life, carbon footprint and more.
This study shows which types of data are already captured in a food value chain. The analysis also shows that to answer consumer expectations, we need to account for new types of information on social conditions. We also found that another issue was the lack of data sharing between the actors. To be able to really evaluate and improve product sustainability, it is necessary to develop a common database of values.
A.8 Case 8 – Consumer electronics
The consumer electronics case study provides time-dependent economic and environmental rationales for remanufacturing. Depending on the timing of remanufacturing (or product age, physical conditions and technological obsolescence), there may be optimal manufacturing strategies, which in turn lead to optimal product designs for economic profitability and environmental sustainability. Our approach can be applied to a broad range of products – from consumer electronics to automotive parts etc. – where there is an active and widely accepted market for remanufactured products.
The research validates and answers the following questions on two factors: physical deterioration and technological obsolescence:
(i) What is the cost advantage of remanufactured products over producing the equivalent brand-new product?
(ii) What is the environmental advantage of remanufactured products over producing the equivalent brand-new product?
(iii) What is the optimal pricing of remanufactured products compared to brand-new products from the net-profit perspective?
(iv) What is the minimum allowable pricing for remanufacturing to be economically viable?
A.9 Case 9 – Building and externalities
Ambitious building retrofits to improve energy performance are often barely justified by energy savings only. Indeed, the ROI to halve a building’s energy consumption is more than 25 years, which often discourages investors. Energy efficiency thus needs to be considered differently to be economically justified, which prompted a consortium of big French construction companies and academic partners to co-develop a new methodology called DECADIESE (Cluzel et al. Reference Cluzel, Yannou and Da Costa2015). Broader than an energy-efficiency focus only, DECADIESE aims to capture the sustainable value of a building via an original focus going beyond the scope of classical analytic methods, by extending the perimeter of associated stakeholders in order to enable ambitious building projects. DECADIESE considers an extended value of a building by incorporating economic, social and environmental aspects through elicitation of externalities and integration with a multi-stakeholder perspective, and also by recentring the value created by a building around the benefits brought to its users through a functional approach.
A.10 Case 10 – Building and usage
The building sector is currently a major driver of environmental impacts. In France, it was responsible for 44% of the total French energy consumption, 75% of waste and 23% of $\text{CO}_{2}$ emissions. Most new buildings now integrate sustainability by reducing energy consumption during the use phase or using greener materials. In this context, the major share of energy consumed during the use phase depends on inhabitants’ behaviours. The first focus of work on buildings and usage was to capture the influence of user behaviours on energy performance in residential buildings. To do so, we used the SABEC model (stochastic-activity-based energy consumption). We identified 29 daily activities characterized by user needs and a target service unit per activity. Behaviours were derived from social and economic attributes of the residents, dwelling characteristics and the availability and performance of home appliances. The SABEC model serves to forecast occupant-related energy consumption in residential buildings while accounting for variability in consumption patterns due to occupants’ varying socio-demographic and -economic profiles. Second, we developed a use-phase memory model for residential buildings designed to storing energy consumption and usage patterns. These data collection and analysis tools are useful for building experts designing new sustainable buildings and retrofitting existing building stock.