In 2004, researchers at the Ford Motor Company unveiled a software package that would revolutionize the development of new car engines. Up until then, making cast aluminum engine components involved designing and creating a prototype, testing it, and then reworking and repeating the process.
Everything from slight changes in an alloy’s chemical composition to the temperature at which it is heated cause subtle changes to its microstructure. Using commercial software and in-house codes, the researchers had developed simulations to capture the impact of manufacturing processes on the material’s structure across atomic, nano-, micro- and macroscopic dimensions. These materials computation models seamlessly integrated a wide range of approaches from ab initio calculations and phase field modeling to finite element techniques. Some models predicted how these structural changes affected strength and fatigue, while others predicted the effect of these physical properties on durability.
The resulting Virtual Aluminum Castings (VAC) package allowed engineers to design and test engine components on a computer. VAC has been used in more than 20 engine programs, where it has cut development time by 25%, saving Ford about USD$100 million.
The VAC project is an example of Integrated Computational Materials Engineering (ICME), which aims to design new products, materials, and manufacturing processes by linking models at different length scales. The Office of Naval Research’s Dynamic 3D Digital Structure program and DARPA’s Accelerated Insertion of Materials program are other successful ICME examples. They show the value of “bringing computational tools, experimental data, and heuristics and models together to streamline design,” said Julie Christodoulou, the director of the ONR’s materials division.
These ICME projects set the stage for the Materials Genome Initiative (MGI), announced in June 2011 by U.S. President Obama. The MGI aims to extend these ideas further by applying them to the discovery of critical new materials. While its precise details are still under development, the initiative’s goals are to “discover, develop, manufacture, and deploy advanced materials at least twice as fast as is possible today, at a fraction of the cost.”
VAC has been used in more than 20 engine programs, where it has cut development time by 25%, saving Ford about USD$100 million.
By accelerating advanced materials discovery, the MGI hopes to address some of the country’s most pressing needs: clean energy, national security, and human welfare. Experts believe it could have a critical, far-reaching impact on the global clean energy revolution. “Clean energy is driven heavily by advanced materials,” said Cyrus Wadia, the assistant director for clean energy and materials research and development at the White House Office of Science and Technology Policy. “Photovoltaics, lightweight alloys for vehicles and wind turbine blades, fuel cell membranes, all of these are advanced materials,” he said. If we are serious about scaling to terawatts of clean energy from such technologies, we need to recognize that they are materials-intensive and focus on ways to change that, he added. “We’re going to have to innovate quickly and get products to market faster, and the materials genome is a way to do that,” Wadia said.
Part of the MGI’s focus is to develop more accurate, powerful ICME tools to replace expensive, time-consuming experimental procedures. That would reduce the costs of designing and manufacturing new products using existing materials or their slight variations, said John Allison, a professor of materials science and engineering at the University of Michigan, who led the VAC project at Ford. Manufacturers could then, for instance, rapidly adopt lightweight aluminum and magnesium alloys in vehicle body parts, or optimize nickel- and titanium-based alloys in engines that operate at higher temperatures, both of which would improve fuel efficiency.
The initiative’s real value for energy, though, is in speeding up the discovery of novel materials tailored for specific applications. This concept, called inverse design, involves starting with a set of desired properties and deducing the structure that achieves those properties. A definitive goal of computational materials science is to design new materials by integrating first-principles methods—which determine materials properties by solving basic equations of quantum mechanics and statistical mechanics—with a wide array of computational techniques, such as ab initio, phase field, finite element, and Monte Carlo. The idea is to be able to eventually design, for example, a photovoltaic material that is 40% efficient and easily printable on a plastic sheet, much like an engineer would design a bridge to carry a specific load and withstand vibrations.
This ultimate goal of the MGI of designing materials from scratch could have a far-reaching impact across all sectors of clean energy: batteries, thermoelectrics, hydrogen storage, photovoltaics, catalysts, light-emitters, and structural alloys. “In so many critical energy areas, what we really lack at this moment in time is effective materials,” said Chris Wolverton, a materials science and engineering professor at Northwestern University. “In a lot of these problems, what we need is materials discovery.”
The six decades-old solar photovoltaic industry, for example, is built around a list of about 10 materials, most of which were discovered by accident, according to Alex Zunger, a researcher with the U.S. DOE’s Energy Frontier Research Center on Inverse Design. The fact that many high-tech sectors are focused on a very limited number of semiconductor materials is astonishing, Zunger said. “In bioengineering, the number of species is on the order of 105. It’s a striking difference,” he said.
Good thermoelectrics are few and far between because they require an unusual combination of mobility and low thermal conductivity, said David Singh, a researcher at the Oak Ridge National Laboratory. The 15% thermal-to-electrical power efficiency of bismuth telluride and lead telluride, two common thermoelectrics used today, is not high enough for solar thermal and waste-heat capture in vehicles. Singh hopes that the MGI will lead to the development of tools that help researchers to precisely predict thermal conductivity of materials; accurately screen for thermoelectric properties in classes of compounds, some of which are already known thermoelectrics; and better calculate the doping dependence of thermoelectric properties of known materials.
Storing hydrogen for fuel cells vehicles requires lightweight materials that can hold a lot of hydrogen by weight and volume, do not need high pressures to get it in or high temperatures to get it out, and store and release it quickly, said Wolverton. “Computation has allowed us to predict materials and reactions with suitable hydrogen capacity and thermodynamics to give good temperature and pressure characteristics,” he said. “However, what we need now is methods to predict kinetics for these reactions.”
Computationally designing and validating new materials is, for now, a vision. However, the exponential rise in computing power over the past few decades—a result of Moore’s Law, which holds that the number of transistors on an integrated circuit will double roughly every two years—has driven materials computation to a remarkable extent. Scientists have developed powerful algorithms to predict materials behavior and are able to use high-throughput computing to screen thousands of materials and pick out those with desirable properties.
One example of this sort of computational engineering is the Materials Project at the Massachusetts Institute of Technology.
By accelerating advanced materials discovery, the MGI hopes to address some of the country’s most pressing needs: clean energy, national security, and human welfare.
The project was previously known as the Materials Genome Project, but has no direct relation with the White House initiative that has borrowed the name. The goal of the MIT project, launched by materials science and engineering professor Gerbrand Ceder, is to find potential new materials for lithium-ion batteries. They explore this by computationally designing compounds, calculating their properties, narrowing down the many possible to a few that show the most promise, and then sharing the data with experimentalists, who decide which materials to synthesize.
Ceder’s group starts with the Inorganic Crystal Structure Database of over 100,000 compounds and uses artificial intelligence algorithms that perform chemical substitutions to predict novel compounds that are likely to exist. Their simulation predicts crystal structure stability using first-principles methods. To synthesize a class of compounds called lithium carbonophosphates, for instance, the algorithm began with one of very few known sodium carbonophosphates. “We modify it, calculate if it’s stable, and then modify further,” Ceder said. “As soon as we have something that looks stable, we would throw the idea to experimentalists.”
Wolverton believes that ab initio computing is critical to accelerate the discovery of energy-related materials. However, added Long Qing Chen, a materials science and engineering professor at Penn State University, “you have to integrate computation at different spatial scales, and need to have theorists closely working with experimentalists in order to iterate experimental and simulation results to validate each other.”
This is precisely what the MGI hopes to achieve, according to Steve McKnight, the director of the National Science Foundation’s civil, mechanical, and manufacturing innovation division, which is supporting the MGI. The initiative will promote research to “identify fundamental mechanisms that control material behavior; define mathematical models to capture those phenomena; code those mathematical models; and develop tools to validate the models across length scales,” he said.
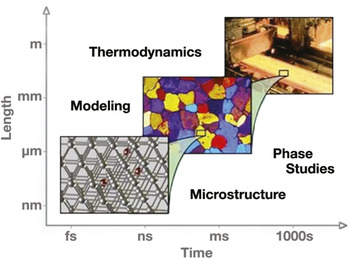
Advancing computational tools is the key to achieving the goal of the Materials Genome Initiative: to speed up the discovery and deployment of advanced materials that could impact energy.Credit: Department of Computational Materials Design, Max-Planck-Institute for Iron Research
What’s more, the MGI will encourage researchers to develop computational tools on an open platform. The intention is to create a digital data infrastructure by implementing standard methods for researchers to share algorithms and models, as well as large, open access databases that scientists can index and search. Instead of a student or researcher writing code at separate universities or national laboratories, this would allow the entire community to collaborate and pool their expertise, speeding up innovation. A product designer in the industry could then easily incorporate advanced models into their own software tools and apply it to real-world problems. “Part of the problem is we have masses of information but it’s all in publications, file folders, or lab notebooks,” said Allison.
This focus on digital data could have a tremendous impact on catalyst research, believes Jens Nørskov, a chemical engineering professor at Stanford University. Catalysts play a crucial role in energy conversion and storage. Nørskov and his colleagues are already trying to create an extensive public database of calculated values of reaction barriers and energies on surfaces, which should help to identify new catalytic materials. Such databases will promote materials informatics in much the same way as the Human Genome Project fueled the interest in bioinformatics. “The idea is to have data available to everyone in a simple, systematic way so they don’t end up spending time looking for numbers in journal papers,” he said. “We want to go from materials science being very data poor to being data rich.”
For the MGI to deliver on its promise of a far-reaching impact on clean energy, the materials research community will have to help define and shape it by working in an interactive, interdisciplinary fashion. Wadia said the federal government is playing a catalytic role to get the conversation started, but all stakeholders—private industry, national laboratories, academic institutions—should be actively involved. “This is an all-hands-on-deck approach,” he said. “There’s a cultural shift that has to happen in order to take full advantage of computation, data informatics, and new experimental techniques.”
“We’re going to have to innovate quickly and get products to market faster, and the materials genome is a way to do that.”–Cyrus Wadia
Anton van der Ven of the University of Michigan and Christopher M. Wolverton of Northwestern University served as Feature Editors for this article.