Introduction
The relationships between fertility management and integrated weed management have long been recognized but remain difficult to combine into a single decision-making framework. Fertility amendments frequently influence weed–crop competition (Buhler Reference Buhler2002; DiTomaso Reference DiTomaso1995; Zimdahl Reference Zimdahl2018), but these effects vary between and within systems. Fertility management is also guided by many considerations other than weeds, including direct effects of nutrients on crop yield and environmental health over short and long timescales. These considerations have sometimes dominated decision making, with any undesirable impacts on weed–crop competition being addressed through herbicide applications. However, overreliance on herbicides has become increasingly problematic with the global rise in herbicide-resistant weeds (Délye et al. Reference Délye, Jasieniuk and Le Corre2013; Mortensen et al. Reference Mortensen, Egan, Maxwell, Ryan and Smith2012; Norsworthy et al. Reference Norsworthy, Ward, Shaw, Llewellyn, Nichols, Webster, Bradley, Frisvold, Powles, Burgos, Witt and Barrett2012; Powles and Yu Reference Powles and Yu2010). The growing market for organic food has also increased the number of cropping systems in which aggressive weed competition must be either prevented or addressed with alternative methods (Badgley et al. Reference Badgley, Moghtader, Quintero, Zakem, Chappell, Avilés-Vázquez, Samulon and Perfecto2007; Hughner et al. Reference Hughner, McDonagh, Prothero, Shultz and Stanton2007; Seufert et al. Reference Seufert, Ramankutty and Foley2012). At the same time, efforts to reduce negative environmental consequences of fertilization, such as groundwater contamination and eutrophication (Carpenter et al. Reference Carpenter, Caraco, Correll, Howarth, Sharpley and Smith1998; Conley et al. Reference Conley, Paerl, Howarth, Boesch, Seitzinger, Havens, Lancelot and Likens2009; Spalding and Exner Reference Spalding and Exner1993), often focus on increasing partial factor productivities for added nutrients. Thus, the effects of nutrient addition on weed–crop competition may be increasingly relevant to both integrated weed management and fertility management.
The study of weed–crop competition draws on broader theories of competition. One foundational idea is the principle of competitive exclusion, which states that two functionally identical species competing for the same limited resources will not achieve stable coexistence (Gause Reference Gause1934). This principle not only explains dominance (suppression) but also predicts that competition will result in niche differentiation. Both species, particularly the lesser competitor, will undergo selection for traits that reduce niche overlap as well as traits that increase competitive ability. Although there are limits to the extent to which plants can differentiate their resource requirements, niche differentiation (e.g., the use of different soil nutrient pools) may be an important influence on competitive dynamics in agroecosystems (Smith et al. Reference Smith, Mortensen and Ryan2010). Niche-based perspectives have been opposed by neutral theories, which argue that differences between individuals and/or species have weak impacts on ecological dynamics (Caswell Reference Caswell1976; Hubbell Reference Hubbell2001). Priority effects play a prominent role in modern neutral theories (as in the work on island biogeography that preceded them [MacArthur and Wilson Reference MacArthur and Wilson1967]) and in studies of weed–crop competition with variable emergence timing. Niche and neutral theories are not mutually exclusive (Adler et al. Reference Adler, HilleRisLambers and Levine2007; Leibold and McPeek Reference Leibold and McPeek2006; Matthews and Whittaker Reference Matthews and Whittaker2014) but sometimes differ in their applicability to different topics. For example, niche theory underlies the C-S-R theory of Grime (Reference Grime1977, Reference Grime1979), which claims that species adapted to low-stress, low-disturbance habitats may be effective competitors for multiple limiting resources. Although agricultural weeds are well adapted to disturbed habitats, many weeds also exhibit traits associated with this group of competitors, such as dense leaf canopies or extensive root systems. An alternate hypothesis, the R* theory, argues that species have a competitive advantage when they require less of a limiting resource than their competitors (Tilman Reference Tilman1982). This theory helps contextualize studies in which crops or weeds with high nutrient optima are less competitive in low-fertility soil and may be extended to describe phenomena such as luxury consumption. All these theories emphasize outcomes of competition rather than mechanisms, which are difficult to generalize. A crucial exception is the association between asymmetric competition for light and competitive dominance (Weiner Reference Weiner1990), which is central to a trait-based view of weed–crop competition (Gaba et al. Reference Gaba, Perronne, Fried, Gardarin, Bretagnolle, Biju-Duval, FColbach, Cordeau, Fernández-Aparicio, Gauvrit, Gibot-Leclerc, Guillemin, Moreau, Munier-Jolain, Strbik and Reboud2017).
The effects of fertility treatments on weed–crop competition can vary according to the placement, timing, rate, and source of nutrients supplied. In the last review to focus exclusively on this subject, DiTomaso (Reference DiTomaso1995) surveyed research on all four factors. In the 25 years since his review, there has been little change in the scientific consensus on general trends in nutrient placement and timing, although best practices are still being developed in many cropping systems. Regarding nutrient placement, DiTomaso (Reference DiTomaso1995) concluded that banded and/or deep fertilizer applications reduced weed growth relative to broadcast applications. This idea has received support from diverse studies in which banded or injected applications of inorganic or organic fertilizer were preferable to broadcast applications for weed control (Blackshaw Reference Blackshaw2005; Blackshaw et al. Reference Blackshaw, Semach and Janzen2002, Reference Blackshaw, Molnar and Janzen2004b; Blackshaw and Molnar Reference Blackshaw and Molnar2009; Kirkland and Beckie Reference Kirkland and Beckie1998; Mesbah and Miller Reference Mesbah and Miller1999; Petersen Reference Petersen2003; Rasmussen Reference Rasmussen1995, Reference Rasmussen2002; Rasmussen et al. Reference Rasmussen, Rasmussen and Petersen1996; Santos et al. Reference Santos, Dusky, Stall and Gilreath2004b; Sengxua et al. Reference Sengxua, Jackson, Simali, Vial, Douangboupha, Clarke, Harnpichitvitaya and Wade2019). Outstanding questions largely concern the circumstances under which savings from reduced fertilization rates and weed pressure can offset the cost of standard banding equipment and/or technologies allowing deeper applications. Weed species and density both affect these cost–benefit analyses. Unlike DiTomaso’s (Reference DiTomaso1995) conclusions about nutrient placement, his observation that application timing can affect weed–crop competition remains true in theory but difficult to apply in practice. For example, later (within- or across-season) nitrogen (N) applications may decrease (Angonin et al. Reference Angonin, Caussanel and Meynard1996; Blackshaw et al. Reference Blackshaw, Molnar and Janzen2004b), increase (Ball et al. Reference Ball, Wysocki and Chastain1996; Dhima and Eleftherohorinos Reference Dhima and Eleftherohorinos2001), or have little effect on (Johnson et al. Reference Johnson, Ott, Gibson, Nielsen and Bauman2007; Sanusan et al. Reference Sanusan, Polthanee, Audebert, Seripong and Mouret2010; Terry et al. Reference Terry, Marquardt, Camberato and Johnson2012) weed competitiveness. Further work on this topic must evaluate whether effects on weed–crop competition ever outweigh other determinants of application timing. If so, it will be important to establish whether factors such as seed size (Liebman and Davis Reference Liebman and Davis2000) or weed responsiveness to nutrients are useful in predicting the weed management implications of application schedules.
This review focuses on the effects of nutrient rate and source on weed–crop competition. Both DiTomaso (Reference DiTomaso1995) and Kaur et al. (Reference Kaur, Kaur and Chauhan2018), who recently described the effects of both fertilizer and water availability on weed–crop competition, noted that the effects of nutrient rate on weed growth, weed–crop competition, and crop yield are highly variable. Here, we identify trends underlying this variability. To facilitate useful generalization, we focus on the effects of macronutrient addition on weed growth, crop growth, and crop yield in annual cropping systems within a single growing season. Our treatment of this topic begins with a survey of studies on weed responses to fertility in monoculture, then addresses competition with crops. We present a new conceptual framework for evaluating the effects of nutrients on weed–crop competition. Finally, we address research on nutrient sources and particularly on organic fertility amendments, which provide unique opportunities and risks for weed management. This review is intended to illuminate mechanisms underlying complex data sets, guide extrapolations from published studies to field situations, and suggest questions and design considerations for future work.
Weed Growth in Monoculture: How Are Common Weed Species Affected by Soil Fertility?
Studies on the effects of nutrient availability on the growth of weeds in monoculture demonstrate species’ inherent responsiveness to nutrients without the confounding effects of interspecific competition. Species characteristics evaluated may include responsiveness of germination, emergence, growth, and reproduction to increasing fertility; tolerance of low fertility, including ability to extract nutrients from soil; nutrient-use efficiency; and tissue nutrient accumulation, including luxury consumption. Many frequently measured traits are relevant to both vegetative growth, the focus of this review, and reproductive output. For example, nutrient-induced increases in plant size may be accompanied by dramatic increases in seed production in Rumex spp. (Hejcman et al. Reference Hejcman, Křišťálová, Červená, Hrdličková and Pavlů2012; Hrdličková et al. Reference Hrdličková, Hejcman, Křišťálová and Pavlů2011).
N addition can stimulate germination and emergence in many weed species, such as Powell amaranth (Amaranthus powellii S. Watson), redroot pigweed (Amaranthus retroflexus L.), common lambsquarters (Chenopodium album L.), and false chamomile [Tripleurospermum inodorum (L.) Sch. Bip.] (Baskin and Baskin Reference Baskin and Baskin2014; Brainard et al. Reference Brainard, DiTommaso and Mohler2006; Sardi and Beres Reference Sardi and Beres1996; Sweeney et al. Reference Sweeney, Renner, Laboski and Davis2008; Williams and Harper Reference Williams and Harper1965). The stimulatory effect of N may interact with other dormancy inhibitors or stimulators (Gallagher and Cardina Reference Gallagher and Cardina1998; Sweeney et al. Reference Sweeney, Renner, Laboski and Davis2008). The effect of N on germination may also be dependent on the maternal environment of the seed (Fawcett and Slife Reference Fawcett and Slife1978), although Brainard et al. (Reference Brainard, DiTommaso and Mohler2006) found no maternal effect. In addition, fertility conditions in the maternal environment can affect seedling growth after germination through effects on seed resources (Hrdličková et al. Reference Hrdličková, Hejcman, Křišťálová and Pavlů2011; Wulff and Bazzaz Reference Wulff and Bazzaz1992).
Insofar as agricultural weeds share a distinct set of traits, they tend to exhibit increased aboveground growth (often measured as biomass, height, or leaf area) in response to fertilization when environmental nutrient levels are low. The high-fertility amendments commonly used in agriculture tend to increase the prevalence of weed species that are both capable of responding to fertility and most competitive under high-fertility conditions (Grime and Hunt Reference Grime and Hunt1975; Pyšek and Lepš Reference Pyšek and Lepš1991). Consequently, species with very low nutrient optima have become increasingly rare over recent decades of high nutrient inputs and are generally not strong competitors in fertilized cropping systems (Fried et al. Reference Fried, Chauvel and Reboud2009a, Reference Fried, Petit, Dessaint and Reboud2009b; Lososová et al. Reference Lososová, Chytrý and Kühn2008; Storkey et al. Reference Storkey, Moss and Cussans2010). However, variation in responsiveness among weed species is a subject of ongoing research and active management concern. Widespread interest in this topic has encouraged the development of new reportable measures, including Moreau’s nitrophily index, an ecophysiological (leaf area–based) alternative to the Ellenberg N scores traditionally derived from natural species distributions (Moreau et al. Reference Moreau, Milard and Munier-Jolain2013). Moreau et al. (Reference Moreau, Milard and Munier-Jolain2013) used the index to report that slender meadow foxtail (Alopecurus myosuroides Huds.), barnyardgrass [Echinochloa crus-galli (L.) P. Beauv.], curlytop knotweed (Polygonum lapathifolium L.), and T. inodorum are equally or more nitrophilic than wheat (Triticum aestivum L.), providing proof of concept for this limited but reproducible metric.
Several large-scale surveys of weed species have indicated that the growth of certain species is particularly sensitive to N, phosphorus (P), and potassium (K) availability. Blackshaw et al. (Reference Blackshaw, Brandt, Janzen, Entz, Grant and Derksen2003) measured shoot biomass, root biomass, and N uptake across 23 agricultural weeds under multiple N treatments (up to 240 mg N kg−1 soil). They found substantial species-by-dose interactions; for example, the increase in shoot biomass with increased N ranged from less than 25% in some species to greater than 700% in others. The most responsive species included A. retroflexus and wild mustard (Sinapis arvensis L.). The height and biomass of A. retroflexus (Berger et al. Reference Berger, Mcdonald and Riha2007) and leaf area and biomass of S. arvensis (Harbur and Owen Reference Harbur and Owen2004) have been independently reported to increase with N. A similar study by Blackshaw et al. (Reference Blackshaw, Brandt, Janzen and Entz2004a) demonstrated variation in the responses of shoot biomass, root biomass, and P uptake to five P treatments (up to 60 mg P kg−1 soil) across 22 agricultural weeds. Shoot biomass (2- to 20-fold increase) was more sensitive to increasing P than root biomass (0 to 3-fold increase). Hoveland et al. (Reference Hoveland, Buchanan and Harris1976) measured the biomass of 10 warm-season and 7 cool-season weeds under several P and K treatments. Among warm-season species, A. retroflexus, jimsonweed (Datura stramonium L.), and Florida beggarweed [Desmodium tortuosum (Sw.) DC.] showed the greatest responses to both P and K. Among cool-season species, common chickweed [Stellaria media (L.) Vill.] was most responsive to P, while S. arvensis and annual bluegrass (Poa annua L.) were most responsive to K. Grant et al. (Reference Grant, Derksen, Blackshaw, Entz and Janzen2007) applied similar rates of K (up to 200 kg K ha−1) to a different group of 19 weed species. Seven species showed increased biomass under high K, especially C. album, kochia [Bassia scoparia (L.) A. J. Scott], and henbit (Lamium amplexicaule L.). Grant et al. (Reference Grant, Derksen, Blackshaw, Entz and Janzen2007) also found that weeds in the Brassicaceae were particularly likely to increase their biomass in response to sulfur, which is an interesting result that should encourage further investigation into the responses of weed species to nutrients other than N, P, and K. Finally, monoculture studies involving multiple species have demonstrated that the species with the most extreme growth responses to added fertility are often the species most negatively affected by low fertility (Harbur and Owen Reference Harbur and Owen2004; Hoveland et al. Reference Hoveland, Buchanan and Harris1976; Qasem and Hill Reference Qasem and Hill1995; Shipley and Keddy Reference Shipley and Keddy1988).
Multispecies data sets on nutrient responses can provide insight into mechanisms underlying interspecific variation and niche differentiation. For example, Ellenberg N values may be used to draw general conclusions about nutrient uptake and productivity (Hill and Carey Reference Hill and Carey1997). These values have been linked to diverse traits, including seed weight, relative growth rate, specific leaf area, and root weight ratio (Bartelheimer and Poschlod Reference Bartelheimer and Poschlod2016). More specialized traits may be associated with nutrient responses within taxa of interest, such as legumes. Among young (pre-fixation) legumes, which rely on N from the seed and environment, interspecific variation in N uptake and environmental variation in N supply jointly influence growth and nodulation (Dayoub et al. Reference Dayoub, Naudin, Piva, Shirtliffe, Fustec and Corre-Hellou2017). Another example comes from Moreau et al. (Reference Moreau, Busset, Matejicek and Munier-Jolain2014), who reported an association between nitrophily and high N uptake per unit of root biomass at high N levels in an analysis of 12 weed species. The sole exception to this rule, E. crus-galli, did not achieve high uptake efficiency at high N despite being nitrophilic. The authors speculated that this departure from the trend might reflect the fact that E. crus-galli was the only C4 species included. Plants that employ the C4 photosynthetic pathway require less of the rubisco enzyme to photosynthesize. Because rubisco is an N-rich protein, C4 plants generally fix more carbon (C) and thus produce more biomass per unit of N taken up than C3 plants (Ehleringer and Monson Reference Ehleringer and Monson1993). Based on this increased efficiency, some authors have proposed that C4 plants should be less limited by N and less responsive to increasing N availability than C3 plants (Ehleringer and Monson Reference Ehleringer and Monson1993; Gastal and Lemaire Reference Gastal and Lemaire2002). A competing hypothesis focuses on the fact that C4 species tend to have high maximum relative growth rates, which may enable them to take advantage of high fertility (Chapin Reference Chapin1980). Some tests of these hypotheses have found no significant difference in N responsiveness between C3 and C4 species (Harbur and Owen Reference Harbur and Owen2004; Wong Reference Wong1979) or greater tolerance of low N in C3 species (Sage and Pearcy Reference Sage and Pearcy1987). In one such study, Harbur and Owen (Reference Harbur and Owen2004) also found that species with high relative growth rates at high N exhibited greater declines in relative growth rate with low N, supporting the idea of a trade-off between the ability to take advantage of high fertility and the ability to thrive under low fertility stress.
Monoculture nutrient response studies can also be used to formulate hypotheses about the effects of nutrients on competition between species. For example, the results of broad surveys of weed responsiveness to N and P conducted by Blackshaw et al. (Reference Blackshaw, Brandt, Janzen, Entz, Grant and Derksen2003, Reference Blackshaw, Brandt, Janzen and Entz2004a) were used to guide selection of representative species with varying responses for replacement series experiments testing the effects of N and P on competition (Blackshaw and Brandt Reference Blackshaw and Brandt2008, Reference Blackshaw and Brandt2009). As hypothesized, species with minimal growth responses to N and P in monoculture did not take advantage of increasing fertility in the replacement series experiments (Blackshaw and Brandt Reference Blackshaw and Brandt2008, Reference Blackshaw and Brandt2009). In the N study, the (biomass-based) competitive abilities of the least-responsive species, Persian darnel (Lolium persicum Boiss. & Hohen. ex Boiss.) and Russian thistle (Salsola tragus L.), were not affected by increasing N (Blackshaw and Brandt Reference Blackshaw and Brandt2008). In the P study, the competitive abilities of B. scoparia and L. persicum decreased with increasing P (Blackshaw and Brandt Reference Blackshaw and Brandt2009). Also as predicted, species that were highly responsive to N (A. retroflexus) or P (common mallow [Malva neglecta Wallr.]) in monoculture usually became more competitive against wheat with increasing N or P, respectively (Blackshaw and Brandt Reference Blackshaw and Brandt2008, Reference Blackshaw and Brandt2009). However, wild oat (Avena fatua L.) was an exception to this trend. In the monoculture studies, A. fatua showed high responsiveness to N and moderate responsiveness to P, relative to wheat’s low responsiveness to both nutrients (Blackshaw et al. Reference Blackshaw, Brandt, Janzen, Entz, Grant and Derksen2003, Reference Blackshaw, Brandt, Janzen and Entz2004a). However, competition between A. fatua and wheat was unaffected by N or P addition (Blackshaw and Brandt Reference Blackshaw and Brandt2008, Reference Blackshaw and Brandt2009). While this result did not have an immediate explanation, it underscores the need to follow monoculture studies with competition studies that evaluate the effects of nutrient and weed treatments on crops in realistic environments.
Weed–Crop Competition: How Does Fertility Rate Affect Crop Yield in the Presence of Weeds?
The relationship between nutrient availability and crop yield reflects not only direct fertility effects but also indirect effects mediated by changes to weed–crop competition. The coexistence of these response mechanisms, both of which interact with other environmental factors, represents one reason why the literature on yield responses to nutrient addition can seem contradictory (Supplementary Table 1). Negative correlations between fertilization (at nontoxic rates) and crop yield can often be attributed to increased competitive pressure from weeds (Supplementary Table 1), for which weed biomass serves as a reasonable (albeit imperfect) proxy (Colbach and Cordeau Reference Colbach and Cordeau2018; Milberg and Hallgren Reference Milberg and Hallgren2004). However, it is difficult to predict when yield losses could occur. Many variables, such as weed density, weed and crop nutrient response curves, relative emergence times, and the availability of other resources, interact to determine whether added fertility will increase, decrease, or have no effect on the yield of a crop in competition with weeds. Because these dynamics are so complex, it is important to acknowledge the species and context specificity of observed fertility effects on weed–crop competition (Kaur et al. Reference Kaur, Kaur and Chauhan2018). However, it may also be helpful to identify broad patterns linking observations from multiple systems.
Conceptual Framework
We propose a conceptual framework to help explain the effects of fertility addition on crop yield in the presence of weeds. This framework is intended to contribute to three goals. First, it may function as an intuitive tool to help explain complex data sets, particularly when the observed yield trend reflects factors emerging from multiple spatiotemporal scales. Second, the framework may help guide extrapolations from existing data. For example, it highlights factors that cannot differ between the study system and a system of interest if study data are to be used for prediction or decision making in the system of interest. Third, the conceptual framework may help with components of experimental design, such as the choice of species and fertility treatments. On a related note, the framework could provide a useful perspective for more quantitative modeling projects, especially weed–crop competition models seeking to represent the effects of soil fertility (Colbach et al. Reference Colbach, Collard, Guyot, Mézière and Munier-Jolain2014; Renton and Chauhan Reference Renton and Chauhan2017).
The framework divides the set of possible weed–crop competition dynamics into four scenarios, each of which is further divided into several cases (Figure 1). The four-way division into scenarios isolates systems in which fertility does not affect weed–crop competition (Scenario 1; Table 1) and categorizes the remaining systems in terms of competition for light. Specifically, we distinguish between systems with limited asymmetric competition for light (Scenario 2; Table 2), strong asymmetric competition for light after canopy closure (Scenario 3; Table 3), and strong asymmetric competition for light by the time of canopy closure (Scenario 4; Table 4). The major role of aboveground dynamics in this framework reflects their powerful influence on outcomes of weed–crop competition, particularly in high-nutrient environments (DiTomaso Reference DiTomaso1995; Saberali et al. Reference Saberali, Modarres-Sanavy, Bannayan Aval, Aghaalikhani, Haghayegh and Hoogenboom2016; Tang et al. Reference Tang, Wan, Cheng, Li, Wang, Pan, Tao, Xie and Chen2014; Weiner et al. Reference Weiner, Andersen, Wille, Griepentrog and Olsen2010). The subdivision into cases within scenarios draws on a combination of species-specific characteristics, such as height and responsiveness to nutrients, and determinants of competitiveness, such as density and emergence timing (Figure 1). It is important to recognize that many of these factors are sensitive to environmental and management conditions, particularly those affecting the availability of other limiting resources.

Figure 1. A conceptual framework for evaluating the effects of added fertility on weed–crop competition. (A) The four scenarios within the framework. In Scenario 1, the added nutrients are not limiting, or the weed is a poor competitor. The other scenarios are distinguished by whether asymmetric competition for light does not occur (Scenario 2), occurs at or after canopy closure (Scenario 3), or occurs before canopy closure (Scenario 4). (B) Key factors used to define cases within scenarios. These factors include influences on relative competitiveness (e.g., emergence timing, density), relative responsiveness to the added nutrients, and relative shading ability under high fertility.
Table 1. Scenario 1: crop and weed competition is unaffected by the added nutrient.
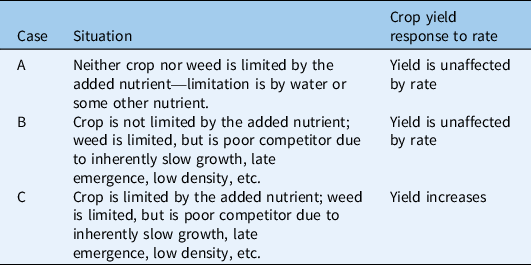
Table 2. Scenario 2: no strong asymmetric competition for light.
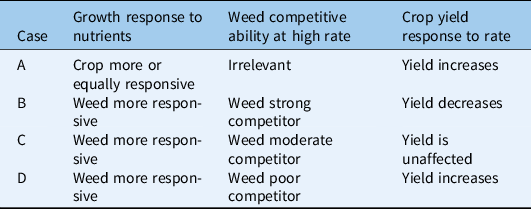
Table 3. Scenario 3: symmetric competition for light before canopy closure, asymmetric competition after canopy closure.
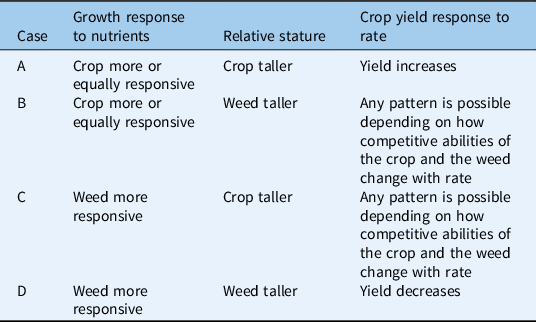
Table 4. Scenario 4: strong asymmetric competition for light by the time of canopy closure.

Several features of the framework require special note. First, the intended output variable is crop yield. A common input is nutrient responsiveness, which some authors report in terms of non-yield parameters (e.g., biomass in grain crops). Variation in yield may be decoupled from variation in other parameters according to trends in resource allocation that vary by species and environment (Donald and Hamblin Reference Donald, Hamblin and Brady1976; Heggenstaller et al. Reference Heggenstaller, Moore, Liebman and Anex2009; Serrano et al. Reference Serrano, Filella and Peñuelas2000; Smith et al. Reference Smith, Rao and Merchant2018; Weih et al. Reference Weih, Asplund and Bergkvist2011). If harvest index depends on nutrient level, the resulting yield adjustment should be (qualitatively) considered at the final step of the framework, after placement into scenario and case. Alternatively, the framework can be used to explain or predict trends in crop biomass or other growth variables in which nutrient responses have been measured. Fertility rate can also affect crop quality, but quality traits will not be addressed in this review because relatively few studies (Blackshaw et al. Reference Blackshaw, Semach, Li, O’Donovan and Harker2000, Reference Blackshaw, Semach and Janzen2002; García-Martín et al. Reference García-Martín, López-Bellido and Coleto2007; Kirkland and Beckie Reference Kirkland and Beckie1998; Lindsey et al. Reference Lindsey, Renner and Everman2013) have examined the effects of fertility on both crop quality and competition with weeds. Second, the framework focuses on the effects of fertility on yield in the presence of weeds. Unlike many previous groups, we do not address the effects of fertility on the percent of potential weed-free yield lost to weed competition. Third, the framework in its basic form is applicable only to crop competition with a single weed species or with functionally similar weed species that do not differ along the axes responsible for placement into scenario and case. Lastly, the framework is relevant to treatments consisting of a single nutrient or amendments containing multiple nutrients.
Applying the Framework to Research Findings
The conceptual framework can be used to better understand how species characteristics and environmental factors contribute to variation in fertility effects on weed–crop competition. These effects contribute to neutral, positive, or negative trends in crop yield with increasing fertility.
No Yield Response to Added Fertility
Some examples of crop yield failing to respond to fertility addition fall under Scenario 1 (Table 1), in which fertility addition does not affect weed–crop competition. Several studies have reported that in at least 1 site-year, water appeared to be more limiting than the added nutrient (Scenario 1, Case A: Ball et al. Reference Ball, Wysocki and Chastain1996; Hellwig et al. Reference Hellwig, Johnson and Scharf2002; Ruf-Pachta et al. Reference Ruf-Pachta, Rule and Dille2013; Ugen et al. Reference Ugen, Wien and Wortmann2002). Weed–water–fertilizer interactions are a complex topic requiring further study (Kaur et al. Reference Kaur, Kaur and Chauhan2018). In other cases, weed growth responded to added fertility but had a limited effect on crop yield, apparently because the weed emerged late relative to the crop (Scenario 1, Case B). For example, Menalled et al. (Reference Menalled, Liebman and Buhler2004) found that soybean [Glycine max (L.) Merr.] yield did not increase with the addition of composted swine manure (synthetic N was applied at higher rates in the no-compost treatment). Although the compost did increase the biomass and competitive ability of waterhemp [Amaranthus tuberculatus (Moq.) Sauer], soybean was still able to suppress this weed when the weed was planted at or after the soybean second-node stage. Liebman et al. (Reference Liebman, Menalled, Buhler, Richard, Sundberg, Cambardella and Kohler2004) reported similar effects of compost addition (treatments as in Menalled et al. Reference Menalled, Liebman and Buhler2004) on competition between corn (Zea mays L.) and velvetleaf (Abutilon theophrasti Medik.). Corn yield did not increase with compost addition in monoculture, presumably because sufficient fertility was available from the soil without compost amendment (averaging N = 5 to 15 mg soil NO3-N kg−1 + 143 kg fertilizer N ha−1; P = 59 mg kg−1; K = 164 mg kg−1). When corn and A. theophrasti were grown together, neither weed biomass nor corn yield varied with compost addition in years in which weeds began to emerge 7 or 11 d after the crop. However, compost increased weed biomass and decreased corn yield when weeds began to emerge only 2 d after the crop, suggesting that the competitive disadvantage associated with late emergence had prevented weeds from causing yield loss in other years’ compost treatments (Scenario 1, Case B).
Lack of a crop yield response to added fertility can also occur when the added nutrient benefits a moderately competitive weed more than the crop in the absence of strong asymmetric competition for light (Scenario 2, Case C). For example, Mesbah and Miller (Reference Mesbah and Miller1999) reported that broadcast application of 45 kg N ha−1 increased winter wheat yield when grown weed-free, but yield was unaffected by N addition when the wheat competed with jointed goatgrass (Aegilops cylindrica Host), a winter annual. Although the weed remained shorter than the crop, the increase in weed pressure at high N apparently prevented a net N response in wheat yield (Scenario 2, Case C). Barker et al. (Reference Barker, Knezevic, Martin, Walters and Lindquist2006) reported similar effects of N on yield in corn grown with A. theophrasti. Although corn yield increased with N in the weed-free control, yield failed to respond to increasing N rates under competition with A. theophrasti that had emerged shortly after the corn. Although weed height never surpassed corn height, increasing competition from the weed was apparently sufficient to cancel the potential increase in corn yield resulting from nutrient addition (Scenario 2, Case C). A third example comes from Blackshaw and Molnar (Reference Blackshaw and Molnar2009), who reported that a broadcast application of 18 kg P ha−1 increased spring wheat yield under weed-free conditions, but not when the wheat was grown with redstem filaree [Erodium cicutarium (L.) L’Hér. ex Aiton], A. fatua, or S. arvensis. In the case of the (prostrate) E. cicutarium and perhaps also S. arvensis, this result suggests that weeds were more responsive to P addition than wheat and prevented an increase in wheat yield through more or less symmetric competition (Scenario 2, Case C). Spring wheat and A. fatua were more similarly responsive to P. However, A. fatua sometimes overtops wheat after canopy closure (Cudney et al. Reference Cudney, Jordan, Bendixen, Holt, Hall, Corbett and Reints1991), so the added P may also have promoted asymmetric competition after A. fatua reached its maximum height (Scenario 3, Case B).
Increased Yield with Added Fertility
An increase in yield with fertility rate can be unaffected by weed competition if the weed pressure is low (Scenario 1, Case C). For example, in one particularly dry year, yield of spring wheat in competition with the perennial weed foxtail barley (Hordeum jubatum L.) increased with N rate in a tilled treatment but not in a no-till treatment (Blackshaw et al. Reference Blackshaw, Semach, Li, O’Donovan and Harker2000). The weed’s biomass was high and responsive to N rate in the no-till treatment, preventing wheat yield from increasing with N. In the tilled treatment, H. jubatum exhibited reduced growth across N treatments, enabling wheat to respond to the increasing N. In another study demonstrating interactions between weed pressure and fertility, Appleby et al. (Reference Appleby, Olson and Colbert1976) reported that winter wheat grain yield tended to increase with a doubled N application rate (56 to 112 kg ha−1) despite competition from low densities of Italian ryegrass [Lolium perenne L. ssp. multiflorum (Lam.) Husnot] (Scenario 1, Case C). At a higher weed density (up to 118 plants m−2), winter wheat grain yield generally did not benefit from increasing N. Because both the winter wheat and L. perenne ssp. multiflorum were fall-emerging, competition was likely symmetric (Scenario 2, Case C).
Yield can also increase with fertility application rate despite a simultaneous increase in weed pressure. This situation may occur when competition between the crop and weed is symmetric and the crop is equally or more responsive to the added nutrient (Scenario 2, Case A). For example, Ruf-Pachta et al. (Reference Ruf-Pachta, Rule and Dille2013) reported that irrigated corn yield and Palmer amaranth (Amaranthus palmeri S. Watson) biomass were both highly responsive to increasing N rate. Because both species benefited from the added N, the percentage of potential corn yield lost due to A. palmeri did not increase with N application rate, and actual yield did increase with rate. Even if the weed is more responsive to the added nutrient, crop yield may still increase with nutrient rate if the weed is a poor competitor. For example, Santos et al. (Reference Santos, Dusky, Stall, Bewick and Shilling2004a) showed that common purslane (Portulaca oleracea L.) reduced lettuce (Lactuca sativa L.) yield primarily through competition for P and secondarily through light interception. Competition for P appeared to explain the finding that P. oleracea biomass was higher in mixture than in monoculture. Lettuce yield increased with P despite increased growth of P. oleracea. In addition to indicating that lettuce was limited by P, this result may suggest that the weed’s growth form did not allow it to exert very strong asymmetric competition for light. These findings constitute an unusual example of Scenario 3, Case B, because P. oleracea did become taller than the lettuce but demonstrated limited shading ability.
An increase in crop yield with added fertility is likely to occur when the crop’s competitive ability increases with added fertility. For example, in Scenario 3, Case A, the crop has a greater maximum height than the weed and is similarly or more responsive to added fertility, so fertility addition facilitates canopy closure and weed suppression. The results of Hellwig et al. (Reference Hellwig, Johnson and Scharf2002) may fall under this scenario and case. This group tested the response of no-till corn to a side-dressed application of 45 kg N ha−1 (in addition to 112 kg N ha−1 added to all plots before planting). In a year with above-average precipitation, the additional side-dressed N increased corn yield despite competition from three grass weeds: large crabgrass [Digitaria sanguinalis (L.) Scop.], E. crus-galli, and giant foxtail (Setaria faberi Herrm.). The corn achieved greater heights than the weeds and likely shaded them asymmetrically (Scenario 3, Case A).
Added fertility may increase crop competitiveness and yield if crop growth increases with rate but weed growth does not. For example, Lindsey et al. (Reference Lindsey, Renner and Everman2013) found that potato (Solanum tuberosum L.) tuber yield increased with cured dairy manure compost rate (added P, K, and micronutrients). Biomass of the weeds C. album, S. faberi, and hairy nightshade (Solanum physalifolium Rusby) was unresponsive to compost rate. Although the weeds overtopped the crop toward the end of the season, regardless of compost treatment, weed–crop competition was symmetric until the late tuber bulking phases and vine senescence (Scenario 2). Before senescence, potato vine biomass increased with increasing fertility, making the crop more competitive and facilitating the increase in tuber yield (Scenario 2, Case A). Similarly, Santos et al. (Reference Santos, Dusky, Stall, Bewick and Shilling2004a) found that lettuce growth increased with P rate despite competition from the inherently taller smooth pigweed (Amaranthus hybridus L.), because the weed’s biomass and height were unresponsive to P addition (Scenario 3, Case B). In two other studies, crop yield increased with fertility rate when spring barley (Hordeum vulgare L.) (Andreasen et al. Reference Andreasen, Litz and Streibig2006) or spring wheat (Blackshaw and Molnar Reference Blackshaw and Molnar2009) emerged a few days before the weed. In both studies, the cold-tolerant crop gained an early-season advantage against the weed, and that advantage was further increased by the added fertility (Scenario 4, Case A).
The framework may be used to compare cases with similar outcomes to determine whether the underlying competitive dynamics were different and might suggest different management needs. In two studies, Awan et al. (Reference Awan, Chauhan and Cruz2014, Reference Awan, Sta Cruz and Chauhan2015) tested the growth of rice (Oryza sativa L.) in competition with wrinkled grass (Ischaemum rugosum Salisb.) or itchgrass [Rottboellia cochinchinensis (Lour.) W.D. Clayton] under four rice seeding rates and four N rates (up to 150 kg ha−1). In both cases, the weed was taller than the rice at all N rates and rice biomass increased with N (Awan et al. Reference Awan, Chauhan and Cruz2014, Reference Awan, Sta Cruz and Chauhan2015). In the I. rugosum study (Awan et al. Reference Awan, Chauhan and Cruz2014), the response of rice was greater than the response of the weed, indicating that N addition improved the relative competitiveness of the crop (Scenario 3, Case B). Increased fertility decreased the height advantage of the weed over the rice. In contrast, the R. cochinchinensis study (Awan et al. Reference Awan, Sta Cruz and Chauhan2015) found that increased fertility led to larger increases in weed height and biomass than crop height and biomass. These data indicate that R. cochinchinensis may be more responsive to nutrient addition than rice. The fact that rice biomass still increased with fertility suggests that R. cochinchinensis was not highly competitive under the study conditions and weed density (Scenario 1, Case C), but changes to weed pressure or management could result in an inverse relationship between fertility rate and yield (Scenario 3, Case D).
Decreased Yield with Added Fertility
Finally, nutrient addition can have negative effects on crop yield if the added nutrient benefits the weed more than the crop. The composted swine manure studies introduced in the subsection on unchanged yields (Liebman et al. Reference Liebman, Menalled, Buhler, Richard, Sundberg, Cambardella and Kohler2004; Menalled et al. Reference Menalled, Liebman and Buhler2004) both describe such outcomes. In addition to the corn and A. theophrasti case described earlier (Liebman et al. Reference Liebman, Menalled, Buhler, Richard, Sundberg, Cambardella and Kohler2004), Menalled et al. (Reference Menalled, Liebman and Buhler2004) reported that compost addition decreased soybean yield when A. tuberculatus was sown at soybean planting or emergence. Under those conditions, compost addition increased A. tuberculatus height but not soybean height, suggesting that compost enhanced the weed’s ability to overtop the soybean and thereby increased asymmetric competition against the crop (Scenario 3, Case D). In a weed-free check, soybean yield did not respond to compost amendment, indicating that compost addition benefited only the weed. In another study, increasing N increased radish (Raphanus sativus L.) yield in the weed-free control but reduced yield when radish was grown with purple nutsedge (Cyperus rotundus L.) (Santos et al. Reference Santos, Morales-Payan, Stall and Bewick1998). The C. rotundus grew faster than the radish and quickly overtopped the crop. This shading ability was likely enhanced by N addition, which increased weed biomass (Scenario 4, Case D or E).
Studies reporting yield losses with increased fertility have sometimes used very high nutrient rates. Liebman et al. (Reference Liebman, Menalled, Buhler, Richard, Sundberg, Cambardella and Kohler2004) and Menalled et al. (Reference Menalled, Liebman and Buhler2004) chose compost rates several times higher than would normally be used by growers in their region (558 to 715 kg N ha−1 in compost + 118 kg N ha−1 as synthetic fertilizer) to ensure a rapid, significant increase in soil nutrient concentrations. Santos et al. (Reference Santos, Morales-Payan, Stall and Bewick1998) used N rates so high (110, 220, 330 kg N ha−1) that radish exhibited N-toxicity symptoms at the highest rate. The effect of fertility on weed–crop competition might have been different at lower rates (see Scenario 4, Case C). However, moderate N rates can also decrease crop yield under weedy conditions. For example, Carlson and Hill (Reference Carlson and Hill1986) found that the yield of a short-statured spring wheat variety increased with N rate (0, 67, or 134 kg N ha−1) only when A. fatua density was less than 1.6% of the weed–crop stand (Scenario 1, Case C). At slightly higher weed densities, spring wheat yield did not benefit from increasing N application rates; at the highest weed densities, spring wheat yield decreased with increasing N rate. This decrease in yield may have been an example of Scenario 3, Case D if A. fatua overtopped the short-statured wheat late in the season (Cudney et al. Reference Cudney, Jordan, Bendixen, Holt, Hall, Corbett and Reints1991).
Extending the Framework to Special Cases
The conceptual framework, like all models, is less complex than the reality of weed–crop competition in the field. However, it may be applied or extended to account for complex trends, including multifactorial effects of high management importance such as interactions between fertility and weed control, the competitive effect of multiple weed species, and weed pressure in intercropped systems.
Interactions between Fertility and Weed Control
The effects of nutrient addition on weed biomass and crop yield often reflect interactions between nutrients and other factors. For example, Scenario 1, Case A accounts for situations in which crops and weeds do not respond to the added nutrient because a different resource is limiting. Environmental and management factors can also affect the yield response to nutrient addition by changing the relative competitiveness of crop and weed at some or all fertility rates. The previous section (“Applying the Framework to Research Findings”) included several examples in which factors like planting density or emergence timing interacted with nutrient addition. Other factors affecting crop competitiveness, including genetic factors, may function similarly but have seldom been tested alongside fertilization rates in studies of weed–crop competition (Harker et al. Reference Harker, O’Donovan, Turkington, Blackshaw, Johnson, Brandt, Kutcher and Clayton2013). Here, we note that weed control measures occupy an important role in the framework (controlled weeds behave like uncompetitive weeds; Scenario 1, Case C) and may enable crop growth and yield to increase with fertility.
Interactions between fertilizer and weed control occur at both plant and field scales. Working at the plant scale, Cathcart et al. (Reference Cathcart, Chandler and Swanton2004) reported that the effects of atrazine, glufosinate, glyphosate, mesotrione, or nicosulfuron on A. retroflexus and green foxtail [Setaria viridis (L.) P. Beauv.] were higher at high N, but these interactions were not consistent in A. theophrasti. Sønderskov et al. (Reference Sønderskov, Swanton and Kudsk2012) observed reduced susceptibility to tribenuron-methyl at low N in T. inodorum and scarlet pimpernel (Anagallis arvensis L.) but clarified that management implications may be limited, as this effect was observed at lower N rates (equivalent to 0 to 20 kg ha−1, in addition to soil fertility of 30.37 mg NO3− kg−1 soil + 1.12 mg NH4+ kg−1 soil) than would be typical in the field. At the field scale, Abouziena et al. (Reference Abouziena, El-Karmany, Singh and Sharma2007) reported that control treatments involving fluroxypyr, bispyribac-Na, and/or hand-hoeing interacted with added fertility to minimize biomass of P. oleracea (at 338 kg N ha−1) and E. crus-galli (at 375 kg N ha−1), but not D. sanguinalis or prickly sida (Sida spinosa L.). Increasing N favored corn growth and yield more than weed growth across weed control treatments (Scenario 3, Case A). At the field scale, fertility can also interact with the timing of weed control. Some authors have demonstrated fertility effects on the critical period for weed control (Evans et al. Reference Evans, Knezevic, Lindquist, Shapiro and Blankenship2003; Tursun et al. Reference Tursun, Datta, Tuncel, Kantarci and Knezevic2015), although the generalizability of these findings remains to be determined.
Understanding of field-scale dynamics is limited by the fact that species-specific data may not be collected or reported in field experiments. Wang et al. (Reference Wang, Liu, Dong, Liu and Lu2019) grew oilseed rape (Brassica napus L.) under five N levels (up to 240 kg ha−1), with or without acetochlor. They found that higher N rates (up to 180 kg ha−1) increased crop yield and that the herbicide interacted with N to further increase yield. The combined biomass of a weed community dominated by A. myosuroides, giant chickweed [Myosoton aquaticum (L.) Moench], Semiaquilegia adoxoides (DC.) Makino, and garden vetch (Vicia sativa L.) was less sensitive to N than the oilseed rape, particularly when treated with acetochlor. These findings contrasted with the results of Kristensen et al. (Reference Kristensen, Olsen and Weiner2008), who reported that N (up to 80 kg ha−1) usually increased spring wheat biomass and yield. While a herbicide combination also increased wheat biomass and yield, the herbicides had a much larger effect in unfertilized plots and very little effect in fertilized plots at high wheat density. The results of both Wang et al. (Reference Wang, Liu, Dong, Liu and Lu2019) and Kristensen et al. (Reference Kristensen, Olsen and Weiner2008) suggest differences between crop and weed responsiveness and competitiveness, but these results would be easier to understand and apply elsewhere if species-specific data had been reported.
Crop Competition with Multiple Weeds
While measures of weed–crop competition may be calculated under the assumption that weed communities act as a single species (Ciaccia et al. Reference Ciaccia, Montemurro, Campanelli, Diacono, Fiore and Canali2015; Paolini et al. Reference Paolini, Faustini, Saccardo and Crinò2006), this approach can become misleading as weed communities increase in species richness and functional diversity. A more diverse weed community is more likely to include at least one species that is both a strong competitor and highly responsive to fertility. If a crop is competing against a diverse weed community, yield will only benefit from increasing fertility if the crop is limited by the added nutrient and at least one of the following conditions is true: (1) weed competitiveness is limited by late emergence, low density, or effective weed control (Scenario 1, Case C); (2) no weed species is as responsive to fertility as the crop (Scenario 2, Case A); (3) the crop is highly responsive to fertility and tall statured (Scenario 3, Case A); or (4) the crop asymmetrically suppresses nearly all weeds by the time of canopy closure, at least at high fertility rates (Scenario 4, Case A).
An early investigation into fertility effects on weed–crop competition tested the effects of P rate on corn competing against A. retroflexus, C. album, D. sanguinalis, and E. crus-galli (Vengris et al. Reference Vengris, Colby and Drake1955). In the weed-free treatment, corn growth response to P was not statistically significant in either of the 2 yr. In contrast, all four weeds increased their biomass with P rate when grown without corn. Significant biomass responses to P were later confirmed in these four species (Blackshaw et al. Reference Blackshaw, Brandt, Janzen and Entz2004a; Hoveland et al. Reference Hoveland, Buchanan and Harris1976). When corn and weeds were grown together, corn biomass was unchanged (first year) or decreased (second year) with increasing P (Vengris et al. Reference Vengris, Colby and Drake1955). In this study, the crop was not limited by the added nutrient, presumably because sufficient P was supplied by the soil and the sod plowed down before planting, but weed growth did benefit and inhibited corn growth at high P rates. This study is a good example of a case in which dissimilar weeds jointly contributed to increased crop suppression at high fertility.
Ampong-Nyarko and de Datta (Reference Ampong-Nyarko and de Datta1993) demonstrated that weed species such as spiny amaranth (Amaranthus spinosus L.), C. rotundus, goosegrass [Eleusine indica (L.) Gaertn.], and R. cochinchinensis may have distinct effects on rice under varying levels of N. When grown in monoculture, each species increased its dry weight with increasing N. However, only rice and A. spinosus increased their relative competitiveness (defined as a proportion of total biomass) with increasing N when all five species were grown together. Rice was also tested against each weed species in two-species mixtures. In competition with A. spinosus or R. cochinchinensis, rice was less competitive at all N levels. With C. rotundus, rice was less competitive at low N but equally competitive at high N. Finally, with E. indica, rice was more competitive at low N but less competitive at high N. Because the experimenters attempted to reduce interspecific shading, competitive dynamics within the latter two mixtures may have been examples of Scenario 2, Cases A and B, respectively. The fact that outcomes in the two-species mixtures were not easily predictable from outcomes in the five-species mixture, or vice versa, illustrates the principle that competitive dynamics between crops and entire weed communities may be more than a sum of two-species interactions.
Several other studies have showed increasing crop yield with N rate despite competition from multiple weed species (Anderson et al. Reference Anderson, Tanaka, Black and Schweizer1998; Juroszek et al. Reference Juroszek, Drews, Neuhoff and Köpke2004; Kolhe et al. Reference Kolhe, Mittra and Bhadauria1988; Swanton et al. Reference Swanton, Shrestha, Roy, Ball-Coelho and Knezevic1999). The study by Swanton et al. (Reference Swanton, Shrestha, Roy, Ball-Coelho and Knezevic1999) is an example of Scenario 1, Case C. The weed community included several weeds that are highly responsive to fertility and potentially strongly competitive against corn (e.g., A. retroflexus, C. album). However, the weed control methods used by Swanton et al. (Reference Swanton, Shrestha, Roy, Ball-Coelho and Knezevic1999), especially the use of herbicides, maintained low enough weed pressure that weed density and species composition were unaffected by side-dressed N rate and corn yield increased with N rate. In contrast, an example of Scenario 2, Case A can be found in Kolhe et al. (Reference Kolhe, Mittra and Bhadauria1988). They observed no significant effect of N rate on the combined biomass of a community of more than 16 weed species, whereas rice grain yield increased with N.
The studies by Anderson et al. (Reference Anderson, Tanaka, Black and Schweizer1998) and Juroszek et al. (Reference Juroszek, Drews, Neuhoff and Köpke2004) are likely examples of Scenario 4, Case A, in which fertility addition increases the crop’s ability to asymmetrically shade the weeds. Anderson et al. (Reference Anderson, Tanaka, Black and Schweizer1998) reported that in a no-till rotation of spring wheat, winter wheat, and sunflower (Helianthus annuus L.), the yield of all three crops increased with broadcast N rate. The naturally occurring weed community was dominated by B. scoparia, S. tragus, yellow foxtail [Setaria pumila (Poir.) Roem. & Schult.], and S. viridis. Density of the weed community decreased with N rate (from 142 to 58 weeds m−2), suggesting that wheat’s ability to suppress these weeds increased with N. Juroszek et al. (Reference Juroszek, Drews, Neuhoff and Köpke2004) used a winter wheat cultivar (‘Pegassos’) that has been selectively bred for competitive ability. In all 3 site-years, grain yield and ground cover of Pegassos winter wheat increased with the addition of an organic N amendment: horn meal (14% N) or fermented molasses (4% N). Ground cover of silky windgrass [Apera spica-venti (L.) P. Beauv.], L. perenne ssp. multiflorum, and S. media increased with N addition, whereas ground cover of catchweed bedstraw (Galium aparine L.), hairy vetch [Vicia hirsuta (L.) Gray], and volunteer alfalfa (Medicago sativa L.) was not affected by N addition. N application increased the wheat’s ground cover more than weeds’ ground cover, likely by increasing the ability of the competitive wheat variety to shade the weeds. Improved weed suppression may have helped enable the observed increase in yield.
Weed Competition against Intercropped Species
In some respects, fertility effects on competition between weeds and intercropped species resemble fertility effects on competition between crops and functionally diverse weed communities. For example, Liebman (Reference Liebman1989) studied the effect of N application on growth and yield of intercropped barley and small or large varieties of field pea (Pisum sativum L.) in competition with white mustard (Sinapis alba L.). Although the addition of 180 kg N ha−1 increased S. alba biomass dramatically (averaging 472%), barley grain yield also increased with fertility. However, grain yield of the small pea variety decreased with N addition. Because the N-fixing field pea was not strongly responsive to N addition (Scenario 3, Case D), N addition may have increased the growth and shading ability of barley and S. alba relative to the pea. Subsequent work on pea–barley intercropped systems confirmed the association between high N and intense competition for light, which may result in weed suppression rather than crop suppression (Corre-Hellou et al. Reference Corre-Hellou, Dibet, Hauggaard-Nielsen, Crozat, Gooding, Ambus, Dahlmann, von Fragstein, Pristeri, Monti and Jensen2011).
Like Liebman (Reference Liebman1989), Vrignon-Brenas et al. (Reference Vrignon-Brenas, Celette, Amossé and David2016) found that increased N (100 kg N ha−1) promoted growth and grain yield of the main crop, winter wheat, but decreased initial growth of the leguminous intercrop, red clover (Trifolium pratense L.) or white clover (Trifolium repens L.), in an organic relay-intercropping system. N also increased weed biomass. While the weeds did not exert so much competitive pressure that increased fertility decreased wheat yield, these results indicate a trade-off between fertilization of the main crop and weed suppression by the intercrop before wheat harvest. After wheat harvest, clover biomass became increasingly similar between the fertilization treatments. This trade-off was not apparent in a different system (Saudy Reference Saudy2015), in which large amounts of N (up to 288 kg N ha−1 top-dressed onto corn) increased corn yield without substantially affecting weed biomass in either a corn monocrop or a corn–cowpea [Vigna unguiculata (L.) Walp.] intercropping system (alternating ridges). Any effects of N on cowpea biomass were not reported, but the intercropping system suppressed weeds across all N levels, decreasing weed biomass by 49.5% relative to the corn monocrop. Without species-specific weed measurements, it is difficult to develop a mechanistic understanding of weed–crop competition dynamics in these studies. However, available data suggest that, from the perspective of the main crop, the intercrop may behave like any environmental factor that reduces weed competitiveness (Scenario 1, Case C), provided that competition between the main crop and intercrop is much less intense than weed competition with either species. From the perspective of the intercrop, some possible outcomes may fall into Scenario 3, Case D or Scenario 4, Cases B and D, because potentially tall weeds can escape from control by responding to N when leguminous intercrops do not.
Effects of Nutrient Source on Weed–Crop Competition
The source used to supply nutrients can affect weed–crop competition through differences in species’ preferences for particular chemical forms, rates of nutrient mineralization and availability, and ratios of added nutrients. Many organic fertility amendments also have additional characteristics (e.g., weed seed content, pathogen content, allelopathic compounds, and organic matter) that affect weed pressure but lie beyond the scope of this review.
Ammonium (NH4+) and nitrate (NO3−) differ in their metabolic costs of uptake and assimilation (Bloom et al. Reference Bloom, Sukrapanna and Warner1992), effects on soil pH (Thomson et al. Reference Thomson, Marschner and Römheld1993), and mobilities in soil solution (Binkley Reference Binkley1984). These differences may lead to variation in the N uptake and growth responses of crops and weeds supplied with NO3− or NH4+. For example, Teyker et al. (Reference Teyker, Hoelzer and Liebl1991) found that corn shoot growth was equally responsive to NO3− or NH4+, but A. retroflexus growth was significantly more responsive to NO3− than to NH4+ applied with a nitrification inhibitor. Another study found that S. faberi growth did not vary between NH4+ (with a nitrification inhibitor) and NO3− treatments (Salas et al. Reference Salas, Hickman, Huber and Schreiber1997). However, at the highest fertility rate, seed production was reduced when N was supplied as NH4+ with the nitrification inhibitor. In a different study, liquid urea was associated with a different weed community and lower total infestation than ammonium sulfate or calcium-ammonium nitrate (Pyšek and Lepš Reference Pyšek and Lepš1991). In some systems, it may be possible to capitalize on differences between crop and weed affinities for certain nutrient forms, essentially tailoring the N supply to minimize benefits to weeds. However, major differences between crop and weed nutrient affinities do not appear to be common.
Conventional and organic fertility amendments tend to differ with respect to timing of nutrient availability, as organic fertility amendments require time to decompose before they can release nutrients into the soil solution (Azam et al. Reference Azam, Malik and Sajjad1985b; Dyck et al. Reference Dyck, Liebman and Erich1995; Harris et al. Reference Harris, Hesterman, Paul, Peters and Janke1994; Ladd and Amato Reference Ladd and Amato1986; Varco et al. Reference Varco, Frye, Smith and MacKown1993; Westcott and Mikkelsen Reference Westcott and Mikkelsen1987). Among organic amendments, nutrient release rate largely depends on the forms of C in the amendment and amount of C relative to N (Azam et al. Reference Azam, Haider and Malik1985a, Reference Azam, Simmons and Mulvaney1993). The C:N ratio of cover crops may increase with plant maturity, contributing to slower nutrient release and even temporary immobilization of N upon termination of mature cover crops (Luna-Orea et al. Reference Luna-Orea, Wagger and Gumpertz1996). Similarly, mineralization rates vary across organic manures and fertilizers (Johnson et al. Reference Johnson, Colquhoun, Bussan and Laboski2012). Mineralization rates also depend on soil temperature and moisture (Havlin et al. Reference Havlin, Beaton, Tisdale and Nelson1999; Stanford and Epstein Reference Stanford and Epstein1974), so the extent of early-season nutrient limitation varies with environmental conditions.
Some researchers have hypothesized that differences in the timing of nutrient supply from organic amendments could be harnessed to improve synchrony between nutrient supply and crop demand (Myers et al. Reference Myers, Van Noordwijk and Vityakon1997). Improved synchrony may increase the nutrient uptake of the crop relative to weeds, especially when peak crop demand is not aligned with peak weed demand. Two reviews of studies related to this hypothesis have concluded that no single organic amendment releases nutrients in complete synchrony with crop demand (Crews and Peoples Reference Crews and Peoples2005; Palm et al. Reference Palm, Giller, Mafongoya and Swift2001). Palm et al. (Reference Palm, Giller, Mafongoya and Swift2001) noted that some organic amendments release nutrients quickly after incorporation, much like conventional fertility sources, which can lead to excess supply in the early growing season when crop demand is low. Other organic amendments release nutrients gradually over the season, which avoids excess supply early in the season but can result in inadequate nutrient supply at peak crop demand (Palm et al. Reference Palm, Giller, Mafongoya and Swift2001). Despite these limitations, apparent synchrony does occur in field situations. For example, Ketterings et al. (Reference Ketterings, Godwin, Mohler, Caldwell and Czymmek2012) found that in 3 of 4 yr, mid-May plow-down of a 1-yr-old red clover green manure combined with 8 to 10 kg N ha−1 of starter fertilizer resulted in peak NO3-N supply 5 to 6 wk after clover plow-down. This timing matched the peak N demand of the corn crop, which was planted in late May or early June.
Nutrient release dynamics may help explain cases in which organic amendments are associated with reduced weed–crop competition relative to inorganic fertilizers. For example, Saberali and Mohammadi (Reference Saberali and Mohammadi2015) found that the large and immediate N influx due to inorganic fertilization led to large soybean seed yield losses in the presence of high densities of A. retroflexus. Under high weed densities, soybean yield was maximized by a combination of inorganic and organic (composted dairy cattle manure and municipal waste compost) fertility sources. Another study on A. retroflexus (in monoculture) found that composted dairy manure increased weed growth, but fresh manure inhibited weed growth (Amisi and Doohan Reference Amisi and Doohan2010). Working in a different system, Naderi et al. (Reference Naderi, Edalat and Egan2017) reported that S. arvensis biomass and associated reductions in rapeseed yield at high weed density were lower in plots fertilized only with organic amendments (composted cattle manure or composted municipal waste) than in plots fertilized with urea or combined (inorganic + organic) treatments. However, rapeseed yield at high weed density was generally similar across fertility treatments. In other cases, fertility source does not affect the intensity of weed–crop competition. Jastrzębska et al. (Reference Jastrzębska, Kostrzewska and Saeid2019) found no differences in weed P accumulation associated with fertility source (superphosphate or phosphorite compared with eight recycled-P treatments), suggesting that recycled P would not increase the competitive effect of weeds on wheat. Knight et al. (Reference Knight, Everman, Jordan, Heiniger and Smyth2017) reported that N rate and source (urea ammonium nitrate, sulfur-coated urea, or composted poultry litter) did not interact with the timing of A. palmeri and D. sanguinalis control to influence corn grain yield. Data suggesting that fertility source does not affect weed–crop competition could indicate that crops and weeds require—or can make use of—similar nutrient influxes at similar times.
Data sets in which organic amendments and/or combinations of fertility sources promote weed control may be consistent with the resource pool diversity hypothesis proposed by Smith et al. (Reference Smith, Mortensen and Ryan2010). This hypothesis contends that the diversity of soil resource pools is inversely associated with the intensity of weed–crop competition. The hypothesis was tested in a greenhouse replacement experiment that measured overyielding in weed–crop mixtures grown in soil from an organic cropping system (diverse crop rotation and organic fertility inputs) or soil from a conventional cropping system (Poffenbarger et al. Reference Poffenbarger, Mirsky, Teasdale, Spargo, Cavigelli and Kramer2015). The resource pool diversity hypothesis would predict greater overyielding in the organic soil, but the authors uncovered limited evidence for this effect. Differences in resource partitioning may be greater under field conditions. However, field conditions make it difficult to differentiate possible effects of resource pool diversity from effects of other factors, such as resource pool size (Ryan et al. Reference Ryan, Mortensen, Bastiaans, Teasdale, Mirsky, Curran, Seidel, Wilson and Hepperly2010). The Poffenbarger et al. (Reference Poffenbarger, Mirsky, Teasdale, Spargo, Cavigelli and Kramer2015) result may also be interpreted as an indication that no single hypothesis can capture all sources of variation in weed–crop competition.
Organic fertility amendments may increase weed growth (Juroszek et al. Reference Juroszek, Drews, Neuhoff and Köpke2004; Little et al. Reference Little, Mohler, Ketterings and DiTommaso2015), sometimes to the detriment of crop yield (Liebman et al. Reference Liebman, Menalled, Buhler, Richard, Sundberg, Cambardella and Kohler2004; Menalled et al. Reference Menalled, Liebman and Buhler2004). In some cases, these trends reflect an imbalance between crop nutrient needs and the nutrients supplied by the amendment. For example, beef cattle feedlot manures and compost manures generally supply nutrients at lower N:P ratios than the N:P uptake ratios of grain crops (Eghball and Power Reference Eghball and Power1999). This imbalance can result in overfertilization of P and other nutrients within a single season and a gradual elevation of soil fertility levels over time (Hart et al. Reference Hart, Marx, Christensen and Moore1997). If responsive weeds can take advantage of this excess fertility, weed pressure may increase. For example, weeds such as A. powellii, C. album, and S. faberi may exhibit large biomass responses to composted poultry manure that are not explained by the amendment’s N content (Little et al. Reference Little, Mohler, Ketterings and DiTommaso2015). The risk of overfertilization with P and other nutrients is reduced at lower manure and compost application rates or when these fertility sources are combined with low-P sources of N, such as green manures. P levels in manure and compost may also be reduced through precision feed management (Ebeling et al. Reference Ebeling, Bundy, Powell and Andraski2002; Ghebremichael et al. Reference Ghebremichael, Cerosaletti, Veith, Rotz, Hamlett and Gburek2007) or manure processing (Westerman et al. Reference Westerman, Bowers and Zering2010).
Differences in supplied nutrient ratios do not always affect weed–crop competition. For example, Ciaccia et al. (Reference Ciaccia, Montemurro, Campanelli, Diacono, Fiore and Canali2015) reported that fertilizer type did not affect weed biomass, weed N uptake, or weed competition with organic zucchini (Cucurbita pepo L.). The fertilizers tested, commercial poultry manure fertilizer, anaerobic digestate fertilizer, and composted municipal solid organic wastes, contained N, P, and K in different ratios. However, differences in P and K content (the more dramatic differences between amendments) may not have affected weed–crop competition because these nutrients were not limiting. These findings show that fertility effects on weed–crop competition depend not only on nutrient supply but also on cropping system and environment. The importance of environmental conditions was also reflected in a study that measured early-season soybean–weed competition for 11 nutrients over 8 site-years (Harre and Young Reference Harre and Young2020). The nutrients N, P, K, iron (Fe), and copper (Cu) were most strongly affected by weed–crop competition; drought conditions increased the importance of weed–crop competition with respect to P, K, and Fe. This research contributes to the emerging consensus that the indirect, weed-mediated effects of fertility source on yield are both highly significant and highly context dependent.
Directions for Future Research
The conceptual framework for evaluating fertility effects on weed–crop competition provides some insight into features of experimental design that facilitate interpretation of yield outcomes and comparisons between studies. Important data to collect in competition studies include crop and weed emergence dates, relative heights of crop and weed at canopy closure and harvest, and weed density. These data are crucial to understanding relative competitiveness and determining whether it changes with fertility level. Because relative competitiveness also depends on management practices and weather conditions, both topics should be addressed in detail. Field studies involving a natural community of weeds require a summary of the most common and aggressive weeds observed. Whenever possible, weed biomass and density should be reported separately for each species. If some species are present at low frequency, it is reasonable to provide data on the dominant species and summary data on the entire weed community.
Other important parameters include weed growth response to fertility, crop yield response to fertility in a series of weed-free controls, and crop yield response to fertility under competition with weeds. Studies involving a larger number of fertility rates (more than three) tend to be particularly helpful for the construction of nutrient response curves and identification of optimal treatments for crops in competition with weeds. A control treatment with no added fertility must be included in both the weed-free and the weedy series. Including both controls enables the researcher to determine whether a lack of yield response to fertility application rate reflects weed competition or indicates that the added nutrients were not limiting. The claim that nutrients were not limiting should also be supported with soil fertility analyses.
Agricultural research on weed-mediated effects of fertility has often focused on competition between a crop and a single weed species or functional type. This approach remains a valuable method of gathering information about fertility effects on relative growth rates, height hierarchies, and shading. It is also a realistic representation of field conditions in which most competitive pressure comes from one type of weed. However, it can be difficult to translate data on pairwise interactions into predictions about the effect of nutrients on a crop in competition with a functionally diverse community of weeds. Mechanistic models of weed–crop competition are improving in their ability to predict the effects of resource availability on heterogeneous weed communities (Renton and Chauhan Reference Renton and Chauhan2017), but fertility dynamics are not easy to model accurately (Colbach et al. Reference Colbach, Collard, Guyot, Mézière and Munier-Jolain2014; Renton and Chauhan Reference Renton and Chauhan2017). Improved representations of fertility effects could be particularly useful if combined with models of aboveground dynamics that emphasize shading (Evers and Bastiaans Reference Evers and Bastiaans2016), which may also need to be expanded to account for multispecies interactions. Even outside quantitative modeling projects, it may be useful to interpret data on weed–crop competition under multiple fertility regimes in terms of the distributions of nutrient requirements and responsiveness associated with the weed community.
Methodological advances have increased the visibility of belowground processes and supported high-resolution data sets on root and soil traits. For example, the partially automated measurement of traits related to root size and architecture (Clark et al. Reference Clark, MacCurdy, Jung, Shaff, McCouch, Aneshansley and Kochian2011; Himmelbauer et al. Reference Himmelbauer, Loiskandl and Kastanek2004; Iyer-Pascuzzi et al. Reference Iyer-Pascuzzi, Symonova, Mileyko, Hao, Belcher, Harer, Weitz and Benfey2010) can decrease the time required to quantitatively analyze root samples, facilitating analyses of root morphology in crops and weeds. Alternatively, root growth patterns can be analyzed using minirhizotrons, which allow nondestructive observations of root growth and soil exploration over time in the field (Johnson et al. Reference Johnson, Tingey, Phillips and Storm2001). Another factor influencing weed–crop competition dynamics is the function of arbuscular mycorrhizal fungi (AMF), which may increase the availability of immobile nutrients such as P (Harley Reference Harley1989; Jeffries and Rhodes Reference Jeffries and Rhodes1987; Stanley et al. Reference Stanley, Koide and Shumway1993) and improve crop yield (Zhang et al. Reference Zhang, Lehmann, Zheng, You and Rillig2019). Most plants can host AMF, but several important weed species are characterized as weak or non-AMF hosts (Jordan et al. Reference Jordan, Zhang and Huerd2000; Li et al. Reference Li, Jordan, Koide, Yannarell and Davis2016; Vatovec et al. Reference Vatovec, Jordan and Huerd2005). AMF can have a negative effect on the growth of these weed species, especially in the presence of a crop (Veiga et al. Reference Veiga, Jansa, Frossard and Heijden2011). More generally, AMF colonization potential is one of several factors underlying interspecific differences in nutrient requirements and responses (Li et al. Reference Li, Jordan, Koide, Yannarell and Davis2016). Consequently, AMF can help mediate relationships between fertility and competitiveness. AMF community composition and infection rate in crop fields also vary with management practices such as tillage, cover crop use, and crop rotations (Douds et al. Reference Douds, Galvez, Franke-Snyder, Reider and Drinkwater1997; Gosling et al. Reference Gosling, Hodge, Goodlass and Bending2006; Vivekanandan and Fixen Reference Vivekanandan and Fixen1991). These effects may constitute one of many mechanisms responsible for interactions between fertilization and other management factors.
Nutrient effects on weed–crop competition can be considered on multiple temporal scales. Over periods of years or decades, increased fertility shifts weed communities in favor of the species most able to benefit from the supplied nutrients (Grime and Hunt Reference Grime and Hunt1975). There exists overwhelming evidence that nutrient inputs are key determinants of weed community composition, diversity, and function (Fried et al. Reference Fried, Chauvel and Reboud2009a; MacLaren et al. Reference MacLaren, Storkey, Strauss, Swanepoel and Dehnen-Schmutz2019; Pinke et al. Reference Pinke, Blazsek, Magyar, Nagy, Karácsony, Czúcz and Botta-Dukát2016; Storkey et al. Reference Storkey, Moss and Cussans2010; Tang et al. Reference Tang, Wan, Cheng, Li, Wang, Pan, Tao, Xie and Chen2014; Wan et al. Reference Wan, Tao, Li, Pan, Tang and Chen2012). These topics were not addressed in our review because few studies connect the within-season effects of fertility on weed–crop competition in annual cropping systems (our focus) to long-term trends. Although the disconnect is understandable, we advocate an increased focus on the long-term consequences of these within-season effects. One useful approach is the collection of data on weed seed production and/or seedbank characteristics (e.g., Blackshaw et al. Reference Blackshaw, Semach, Li, O’Donovan and Harker2000; Brainard et al. Reference Brainard, Bellinder and Kumar2011; Harker et al. Reference Harker, O’Donovan, Turkington, Blackshaw, Johnson, Brandt, Kutcher and Clayton2013; Liebman et al. Reference Liebman, Menalled, Buhler, Richard, Sundberg, Cambardella and Kohler2004; Lindsey et al. Reference Lindsey, Renner and Everman2013). Alternatively, researchers can collect data for several years and report yearly observations of weed community composition alongside measures of weed–crop competition. Although both strategies can be labor-intensive, the resulting data sets are likely to promote effective decision making for long-term weed management.
Conclusion
The rate and source of added macronutrients may influence the intensity and outcome of weed–crop competition. Many agronomic and horticultural weeds are highly responsive to nutrient addition, whether grown in monoculture or annual cropping systems. However, the relative responsiveness of crop and weed and the effect of nutrient addition on weed-associated yield losses are highly variable. Using a novel conceptual framework, we have explained some of this variation in terms of crop and weed traits. We have also surveyed the effects of alternative and organic fertility amendments on weed–crop competition, integrating emerging questions with existing knowledge on nutrient addition. Our findings illustrate the possibility of explaining and predicting the effects of fertility on weed–crop competition across contexts. They also highlight the increasing importance of these indirect fertility effects to both fertility management and integrated weed management.
Acknowledgments
This research received no specific grant from any funding agency or the commercial or not-for-profit sectors. No conflicts of interest have been declared.
Supplementary material
To view supplementary material for this article, please visit https://doi.org/10.1017/wsc.2021.1