Introduction
California produces the majority of the almonds, pistachios, and walnuts in the United States and nearly 85% of the global almond production (CDFA 2024; USDA-NASS 2024). Almond, pistachio, and walnut are planted on 1 million ha in California, with a gross value of more than US$5 billion (CDFA 2024). Moreover, the Sacramento Valley of northern California is the second largest rice production region in the United States, with more than 0.2 million ha of premium-quality, water-seeded rice (USDA-NASS 2024). California rice systems have unique advantages, such as the Mediterranean climate, high solar radiation, and highly mechanized, developed, and precise production practices, which result in ∼20% higher yields than other regions in the United States produce (Hill et al. Reference Hill, Williams, Mutters and Greer2006). In the complex cropping systems of California’s Sacramento Valley, rice is often planted adjacent to almond, pistachio, and walnut orchards.
Most California rice is pregerminated, aerially seeded into 10 to 15 cm of standing water, and maintained under continuous flooding until ∼1 mo before harvest (Brim-DeForest et al. Reference Brim-DeForest, Al-Khatib and Fischer2017a, Reference Brim-DeForest, Al-Khatib, Linquist and Fischer2017b; Hill et al. Reference Hill, Williams, Mutters and Greer2006). This water-seeded system was initially developed to suppress weeds that pose a significant challenge for California rice growers (Hill et al. Reference Hill, Williams, Mutters and Greer2006). In general, many of the most problematic rice weeds are well adapted to continuously flooded growing systems (Brim-DeForest et al. Reference Brim-DeForest, Al-Khatib, Linquist and Fischer2017b; Galvin et al. Reference Galvin, Inci, Mesgaran, Brim-DeForest and Al-Khatib2022) and capable of reducing rice yields by up to 90% unless successfully controlled (Brim-DeForest et al. Reference Brim-DeForest, Al-Khatib and Fischer2017a). Nearly all California rice production heavily depends on complex herbicide programs to control weeds, and because of the continuous flood conditions, these are applied mostly by aircraft (Espino et al. Reference Espino, Greer, Al-Khatib, Godfrey, Eckert, Fischer and Lawler2023).
California rice growers use herbicides at planting and typically also apply at least one additional postemergence herbicide later in the season, between May and mid-July (Galla et al. Reference Galla, Al-Khatib and Hanson2018a). During this time of year, almond trees are actively growing from terminal and lateral buds, spurs and shoots emerge, nut and kernel growth occurs, and translocation of photosynthates from the leaves to kernels begins (Kester et al. Reference Kester, Martin and Labavitch1996). In addition, pistachio trees begin shoot growth at this time of year, and buds form and extend from late May to early July (Ferguson and Kallsen Reference Ferguson and Kallsen2016). Simultaneously, walnut trees in the Sacramento Valley are actively growing, the nuts generally reach their final hull and shell size, and the accumulation of assimilates, such as alcohol-soluble sugars and proteins in the kernels, begins (Galla et al. Reference Galla, Al-Khatib and Hanson2018b, Reference Galla, Hanson and Al-Khatib2019; Pinney et al. Reference Pinney, Labavitch and Polito1998). Consequently, most rice herbicide applications coincide with the important and sensitive growth stages of almonds, pistachios, and walnuts, when these crops are highly vulnerable to off-target foliar herbicide exposure.
Concerns over off-target crop exposure to herbicides by either drift or accidental direct application have been stated among growers, crop consultants, and researchers (Al-Khatib et al. Reference Al-Khatib, Claassen, Stahlman, Geier, Regehr, Duncan and Heer2003; Bhatti et al. Reference Bhatti, Al-Khatib, Felsot, Parker and Kadir1995; Egan et al. Reference Egan, Barlow and Mortensen2014; UCIPM 2024). Factors that affect off-target herbicide drift include wind speed and direction, relative humidity, air temperature, droplet size, herbicide volatility, applicator distance from the edges of the treatment area, and release height of the herbicides (UCIPM 2016). Under most circumstances, off-target herbicide drift occurs below 1/100X to 1/33X of the field use rates (Al-Khatib and Peterson Reference Al-Khatib and Peterson1999; UCIPM 2016). Even at these low levels of drift, some rice herbicides, such as photosystem II inhibitors (Galla et al. Reference Galla, Al-Khatib and Hanson2018a), acetolactate synthase inhibitors (Galla et al. Reference Galla, Al-Khatib and Hanson2018a, Reference Galla, Al-Khatib and Hanson2018b, Reference Galla, Hanson and Al-Khatib2019), and growth regulators used in rice (Haring et al. Reference Haring, Ou, Al-Khatib and Hanson2022), can be of concern due to their widespread use and potential for injury to highly sensitive tree and vine crops.
In plants, auxins are generally responsible for cell division, elongation, and growth and for the development of vascular tissue, floral meristem, leaf initiation, apical dominance, and shoot and root formation (Grossmann Reference Grossmann2010). The small quantities of auxins, such as 5 to 1,000 pg mg–1 plant tissue, impact the growth and development processes in higher plants like almond, pistachio, and walnut (Ferguson and Kallsen Reference Ferguson and Kallsen2016; Kester et al. Reference Kester, Martin and Labavitch1996; Pinney et al. Reference Pinney, Labavitch and Polito1998). However, auxins are toxic at high cellular concentrations, such as degradation, conjugation, transport, and sequestration (Taiz et al. Reference Taiz, Møller, Murphy and Zeiger2022). Synthetic auxins are more stable in plants than natural auxins, such as indole-3-acetic acid (IAA), indole-3-butyric acid, 4-chloroindole-3-acetic acid, and phenylacetic acid (Bishop et al. Reference Bishop, Sakakibara, Seo and Yamaguchi2015; Epp et al. Reference Epp, Alexander, Balko, Buysse, Brewster, Bryan, Daeuble, Fields, Gast, Green, Irvine, Lo, Lowe, Renga, Richburg, Ruiz, Satchivi, Schmitzer, Siddall, Webster, Weimer, Whiteker and Yerkes2016). Owing to their stable structures (Epp et al. Reference Epp, Alexander, Balko, Buysse, Brewster, Bryan, Daeuble, Fields, Gast, Green, Irvine, Lo, Lowe, Renga, Richburg, Ruiz, Satchivi, Schmitzer, Siddall, Webster, Weimer, Whiteker and Yerkes2016), and being much less subject to homeostatic control than natural auxins (Taiz et al. Reference Taiz, Møller, Murphy and Zeiger2022), synthetic auxins cause herbicidal damage, such as tissue swelling, growth inhibition, and epinasty, which can be highly injurious or lethal to susceptible plants. Developing plants are expected to be more sensitive to synthetic herbicides than developed plants (Taiz et al. Reference Taiz, Møller, Murphy and Zeiger2022).
Florpyrauxifen-benzyl [benzyl 4-amino-3-chloro-6-(4-chloro-2-fluoro-3-methoxyphenyl)-5-fluoropyridine-2-carboxylate; CAS: 1390661-72-9] is a synthetic auxin-type herbicide with a novel site of action for weed control in rice (Epp et al. Reference Epp, Alexander, Balko, Buysse, Brewster, Bryan, Daeuble, Fields, Gast, Green, Irvine, Lo, Lowe, Renga, Richburg, Ruiz, Satchivi, Schmitzer, Siddall, Webster, Weimer, Whiteker and Yerkes2016). Picolinate auxin-type herbicides like florpyrauxifen-benzyl have a carboxylic acid functional group, which involves a key binding interaction at the site of action (Epp et al. Reference Epp, Alexander, Balko, Buysse, Brewster, Bryan, Daeuble, Fields, Gast, Green, Irvine, Lo, Lowe, Renga, Richburg, Ruiz, Satchivi, Schmitzer, Siddall, Webster, Weimer, Whiteker and Yerkes2016) and mimics IAA to fill between the receptor and the co-repressor proteins at the cell nucleus. When exogenously applied to susceptible plants, growth disruption, leaf epinasty, tissue swelling, stem curling, excessive chloroplast damage, membrane and vascular system damage, wilting, and necrosis are commonly observed, ultimately leading to plant death (Grossmann Reference Grossmann2010). The low vapor pressure of florpyrauxifen-benzyl, <0.2 kPa (ChemSpider 2024), suggests that volatility is a very minimal factor for potential florpyrauxifen-benzyl nontarget drift.
Since the modern era of herbicides began after the commercialization of 2,4-D in the 1940s and dicamba in the 1960s, auxin-type herbicides became important tools that have been widely used on 2,4-D- and dicamba-resistant crops like corn (Zea mays L.), soybean [Glycine max (L.) Merr.], and cotton (Gossypium hirsutum L.) (Egan et al. Reference Egan, Barlow and Mortensen2014). Owing to extensive use, auxin herbicides are historically well known for their off-target injuries to soybean, cotton, sunflower (Helianthus annuus L.), vegetables, fruit and nut trees, field and forage crops, ornamentals, and vines (Al-Khatib et al. Reference Al-Khatib, Parker and Fuerst1993; Bhatti et al. Reference Bhatti, Al-Khatib and Parker1997; Dittmar et al. Reference Dittmar, Ferrell, Fernandez and Smith2016; Haring et al. Reference Haring, Ou, Al-Khatib and Hanson2022; Marple et al. Reference Marple, Al-Khatib, Shoup, Peterson and Claassen2007; Miller and Norsworthy Reference Miller and Norsworthy2018; Nunes et al. Reference Nunes, Albrecht, Albrecht, Lorenzetti, Danilussi, da Silva, Silva and Barroso2023; Ramos et al. Reference Ramos, Rzodkiewicz, Turcotte and Ashman2021; Sciumbato et al. Reference Sciumbato, Chandler, Senseman, Bovey and Smith2004; Serim and Patterson Reference Serim and Patterson2024; Sharkey et al. Reference Sharkey, Williams and Parker2021; Smith et al. Reference Smith, Ferrell, Webster and Fernandez2017; Warmund et al. Reference Warmund, Ellersieck and Smeda2022; Wells et al. Reference Wells, Prostko and Carter2019). The overall objectives of this research were to study the correlation between symptoms and residue of florpyrauxifen-benzyl in the leaf tissue of nut tree crops after plausible drift rates and to determine if florpyrauxifen-benzyl residue can be used as an indicator of the level of florpyrauxifen-benzyl exposure.
Materials and Methods
Study Site
Three simulated off-target drift experiments were conducted in 2020 and 2021 in newly planted almond (38.539°N, 121.794°W), pistachio (38.539°N, 121.793°W), and walnut (38.539°N, 121.794°W) orchards (elev. 18 m) at the University of California, Davis Plant Sciences Research Facility near Davis, CA. The orchards were established in March 2020 with ‘Nonpareil’ almond scion on ‘Empyrean 1’ rootstock, ‘Kerman’ pistachio scion on ‘UCB 1’ rootstock, and ‘Chandler’ walnut scion on ‘clonal RX1’ rootstock. Almonds and walnuts were planted 6 m apart within rows and 4.2 m apart between rows, while pistachios were 6 m apart within rows and 7 m apart between rows. The soil was classified as a Yolo silt loam with NO3–N 56 ppm, Olsen-P 25 ppm, K 348 ppm, Na 15 ppm, Ca 8 meq 100 g−1, Mg 10 meq 100 g−1, CEC 19 meq 100 g−1, OM 2.7%, and pH 6.7 at all experiments. Trees were maintained free of diseases and insects as recommended by the UCIPM guidelines (Ferguson and Haviland Reference Ferguson and Haviland2016; Micke Reference Micke1996; Ramos Reference Ramos1998; Strand Reference Strand2002, Reference Strand2003). Irrigation was made through a single-line drip irrigation system with emitters spaced every 30 cm. In all experiments, weeds between rows were managed with regular mowing and within rows with a mixture of rimsulfuron at 70 g ai ha–1, indaziflam at 50 g ai ha–1, oxyfluorfen at 560 g ai ha–1, and glufosinate-ammonium at 450 g ai ha–1. In addition, the spray solution for maintenance sprays included methylated seed oil (MSO) at 0.25% v/v and polyvinyl polymer drift control agent at 0.5% v/v.
Herbicide Applications
Florpyrauxifen-benzyl (Loyant® CA, 25 g ai L–1, Corteva Agriscience, Indianapolis, IN, USA) was applied on June 9, 2020, during calm weather conditions to avoid off-target movement, in all three experiments at plausible drift rates of 1/100X (1% drift) and 1/33X (3% drift) of the rice use rate of 29.4 g ai ha–1 (Espino et al. Reference Espino, Greer, Al-Khatib, Godfrey, Eckert, Fischer and Lawler2023; Galla et al. Reference Galla, Hanson and Al-Khatib2019). Four untreated check (UTC) plots were also included for comparison. All spray mixtures included MSO (Super Spread® MSO, Wilbur-Ellis, Fresno, CA, USA) at 584 ml ha–1. All florpyrauxifen-benzyl treatments were applied to one side of the tree canopy with a handheld, CO2-pressured backpack sprayer calibrated to spray 187 L ha–1 at 206 kPa pressure through AIXR8004 nozzles (TeeJet® Technologies, Wheaton, IL, USA). The spray boom had two nozzles spaced 50 cm apart, and treatments were applied in a single 3-s pass from top to bottom per tree. Environmental conditions at the time of application were 16 C air temperature, 58% relative humidity, and 0.4 m s–1 wind speed. All experiments were repeated on May 31, 2021, using the trees that were buffer trees during the 2020 growing season in the same orchards using the previously described methods. Environmental conditions during the second-year applications were 18 C air temperature, 50% relative humidity, and 0.6 m s–1 wind speed. No in-season auxin-type herbicides were used to avoid the potential confusion with florpyrauxifen-benzyl drift experiments.
Experimental Design and Data Collection
Experiments were set up in a randomized complete block design with four replicates, where an individual tree was an experimental unit. An untreated tree between treated trees was included as a buffer to prevent herbicide contamination. Trees were observed for visual symptoms at 6, 12, 24, 48, and 72 hr after herbicide treatment and at 7, 14, 21, 28, 35, 42, and 90 DAT. Symptomology descriptions of the treated foliage were made according to UCIPM herbicide symptoms guidelines (UCIPM 2024). At each observation, the florpyrauxifen-benzyl treated sides of almond, pistachio, and walnut trees were compared with UTC trees. Evaluations were made early in the morning, and photos of trees were taken from the treated side of the canopy throughout the growing season for consistency.
Analytical Methods
Randomly selected leaves from 1/100X and 1/33X florpyrauxifen-benzyl-treated almond, pistachio, and walnut leaves from the treated sides of the canopy and from the UTC were harvested at 7, 14, and 28 DAT. The approximately 50-g samples of harvested leaves were immediately rinsed in 50% methanol solution (SIGALD 439193, Sigma-Aldrich, St. Louis, MO, USA, CAS: 67–56–1) in the field to remove soil dust and unabsorbed florpyrauxifen-benzyl residues on the leaf surface (Al-Khatib et al. Reference Al-Khatib, Parker and Fuerst1992). The leaf samples were double-bagged and brought to the laboratory on dry ice, then frozen in liquid nitrogen and stored in an ultra-low-temperature freezer (MDF-DU901VHA, PHCbi, Wood Dale, IL, USA) until the leaf samples were processed.
To recover and quantify florpyrauxifen-benzyl from 7- and 14-DAT samples, leaf tissues were ground in liquid nitrogen to ∼5-mm-sized pieces, and 500 mg of ground tissue was placed in 7-ml tubes on dry ice. Tissues were fortified with an internal standard of similar hydrophobicity (SPEXQuE™ AOAC Internal Standard Mix, Thermo Fisher Scientific, Waltham, MA, USA) to check stability of instrument response to ensure the recovery accuracy, and 10 to 15 metal homogenizing beads (Fisherbrand™ Bead, Thermo Fisher Scientific) were added to the tubes. Florpyrauxifen-benzyl was extracted by adding 2.5 ml 90% w/v acetonitrile (CAS: 75-05-8, Sigma-Aldrich, St. Louis, MO, USA) and homogenized at 4,400 g n for sixteen 30-s cycles. The extracts were centrifuged at 1,100 g n for 5 min, and 500 ul supernatant was transferred to 2-ml tubes containing 300 mg QuE Verde dispersive SPE (dSPE) (Supel QuE Verde Tube Number 55442-U, Sigma-Aldrich). Extracts with dSPE were shaken on a rotary shaker at 0.5 g n for 15 min and centrifuged again at 17,700 g n for 5 min. The supernatant was directly analyzed using an ultra-high-performance liquid chromatographer coupled to an orbitrap fusion tribrid mass spectrometer (UltiMate 3000 UHPLC, Thermo Fisher Scientific). The liquid chromatography–mass spectrometry/mass spectrometry method was optimized to determine the quantity of florpyrauxifen-benzyl in the samples using an accurate mass and fragmentation pattern matching to a reference analytical standard (USEPA 2020). The concentration of florpyrauxifen-benzyl in each sample was quantified based on an external calibration curve of the analytical standard (Number 684721, HPC Standards, Atlanta, GA, USA) for florpyrauxifen-benzyl. Method validation was performed to determine the limit of florpyrauxifen-benzyl detection and the limit of quantitation (LOQ). To recover and quantify florpyrauxifen-benzyl from 28-DAT samples, the same protocols from 7 and 14 DAT were followed with increased method limits of quantitation (900-mg Supel QuE Verde Tubes) while maintaining acceptable method recoveries in leaf tissues due to the decreased florpyrauxifen-benzyl residue.
Statistical Analysis
Data for florpyrauxifen-benzyl residues were subjected to ANOVA using the agricolae package (de Mendiburu Reference de Mendiburu2024) in RStudio version 2024.04.2+764 (R Core Team 2024), and means were separated using Tukey’s HSD at α = 0.05, when applicable. Visual illustration was generated using the ggplot2 package version 3.5.1 in RStudio (Wickham et al. Reference Wickham, Navarro and Pedersen2024).
Results and Discussion
Florpyrauxifen-benzyl symptoms were apparent on all three nut tree species, and severity of symptoms increased as herbicide rates increased; however, the symptoms were more pronounced on pistachio than on almond and walnut at similar rates. Additionally, the time to develop symptoms was shorter with pistachio than with almond and walnut.
Symptoms on almond and walnut were initially observed at 7 DAT, and severity generally peaked at 14 DAT (data not shown). Although almond and walnut symptoms were observed mainly on the treated sides of the trees, some young walnut leaves on the nontreated side of the canopy also showed minor symptoms. Symptoms were most apparent on young leaves and shoots at all rates. Almond and walnut symptoms included chlorosis, chlorotic spot, epinasty, leaf curling, leaf narrowing, leaf crinkling, necrosis, necrotic spots, shoot curling, and twisting (Figure 1). Leaf curling, necrosis, and necrotic spots were more apparent at the 1/33X rate for almond than for walnut. Walnut symptoms were most apparent on young leaves, whereas old leaves were free of visual symptoms at any rate. Conversely, symptoms on almond leaves could be found throughout the treated part of the canopy regardless of leaf age. At the 1/100X and 1/33X rates, almond and walnut symptoms gradually dissipated, and trees appeared normal at the end of the growing season. Furthermore, almond appeared to recover more quickly from florpyrauxifen-benzyl drift rates than did pistachio and walnut (data not shown).
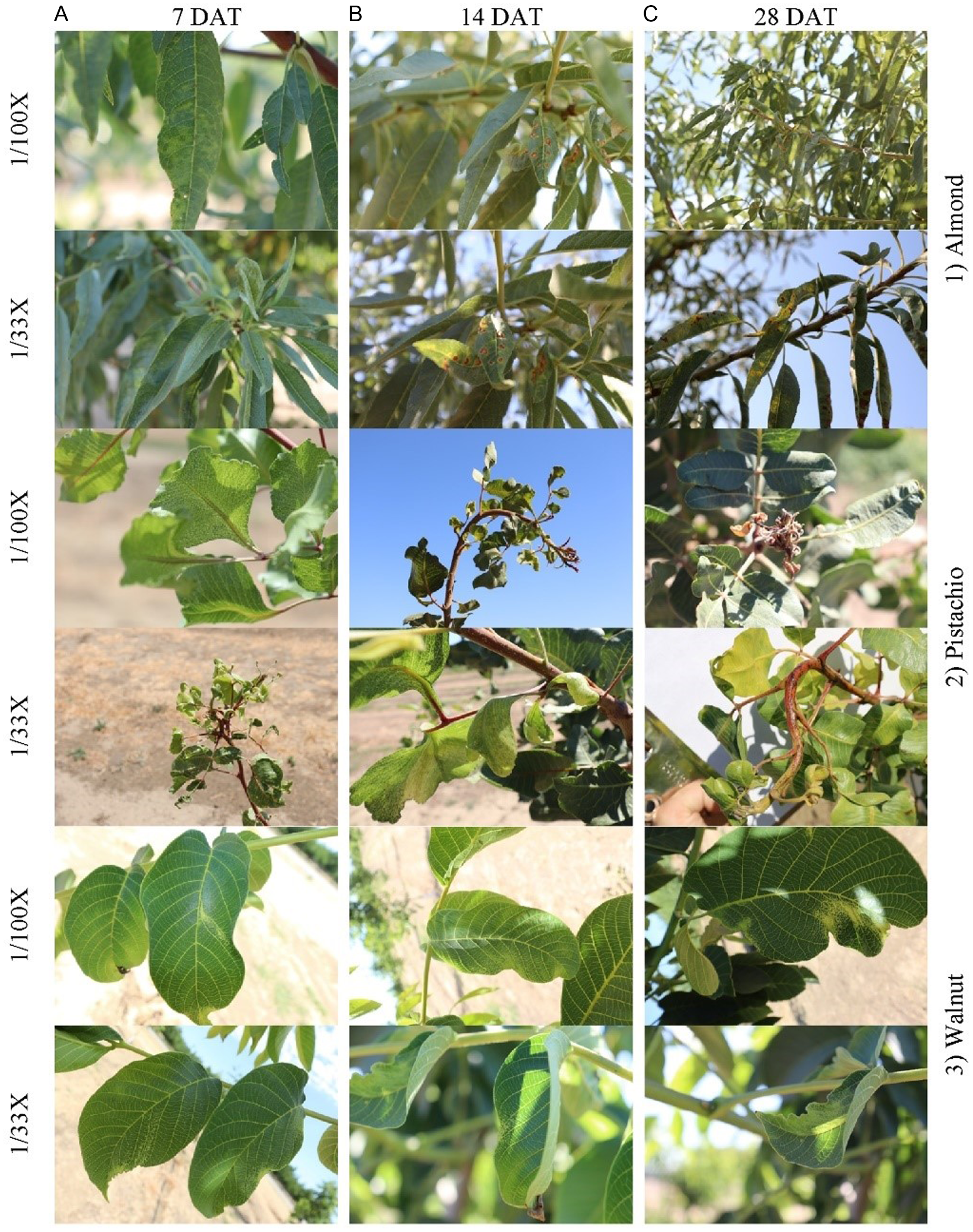
Figure 1. Characteristic symptoms of florpyrauxifen-benzyl on almond (top), pistachio (middle), and walnut (bottom) at 1/100X and 1/33X simulated drift rates of the rice use rate at 7 d after treatment (DAT) (A), 14 DAT (B), and 28 DAT (C) in 2021.
Pistachio was considerably more susceptible to florpyrauxifen-benzyl compared to almond and walnut. Florpyrauxifen-benzyl symptoms were visible at 3 DAT for 1/100X- and 1/33X-treated pistachio and generally peaked at approximately 14 DAT. Pistachio symptoms were observed throughout the canopy and included chlorosis, chlorotic spot, leaf curling, leaf narrowing, leaf distortion, leaf malformation, leaf crinkling, shoot curling, stem coloring with dark maroon-brown spots, stunting, terminal bud twisting, and terminal bud death (Figure 1). Shoot curling, stem coloring, stunting, and twisting were more apparent at the 1/33X rate than at the 1/100X rate. Pistachio symptoms slightly dissipated over time but remained visible throughout the growing season. Injury symptoms persisted into the following spring, when the trees leafed out during the 2021 and 2022 growing seasons. Stem curling, stunting, and twisting were most noticeable at the 1/33X rate for pistachio in the year after treatment.
Chemical analyses showed that the recovery of florpyrauxifen-benzyl residues from leaf samples was within the acceptable range: 82.2% for almond with 7% relative standard deviation (RSD), 103.6% for pistachio with 12% RSD, and 104.4% for walnut with 15% RSD at 14 DAT, and 74% for almond with 6% RSD, 79% for pistachio with 6% RSD, and 92% for walnut with 12% RSD at 28 DAT. The florpyrauxifen-benzyl recovery levels were between 70% and 120% of the known quantity of florpyrauxifen-benzyl with ≤20% RSD at appropriate residue analysis standards (USEPA 1996). In quantification tests, no residues were detected in any of the UTC leaf tissues sampled. At 7 DAT with the 1/100X rate, florpyrauxifen-benzyl residues were 2.31, 1.78, and 3.58 ng g–1 FW in almond, pistachio, and walnut, respectively. The residues in the 1/100X-treated plots were 1.10, 0.68, and 2.05 ng g–1 FW at 14 DAT and 0.04, 0.10, and 0 ng g–1 FW at 28 DAT for almond, pistachio, and walnut, respectively (Figures 2 to 4).
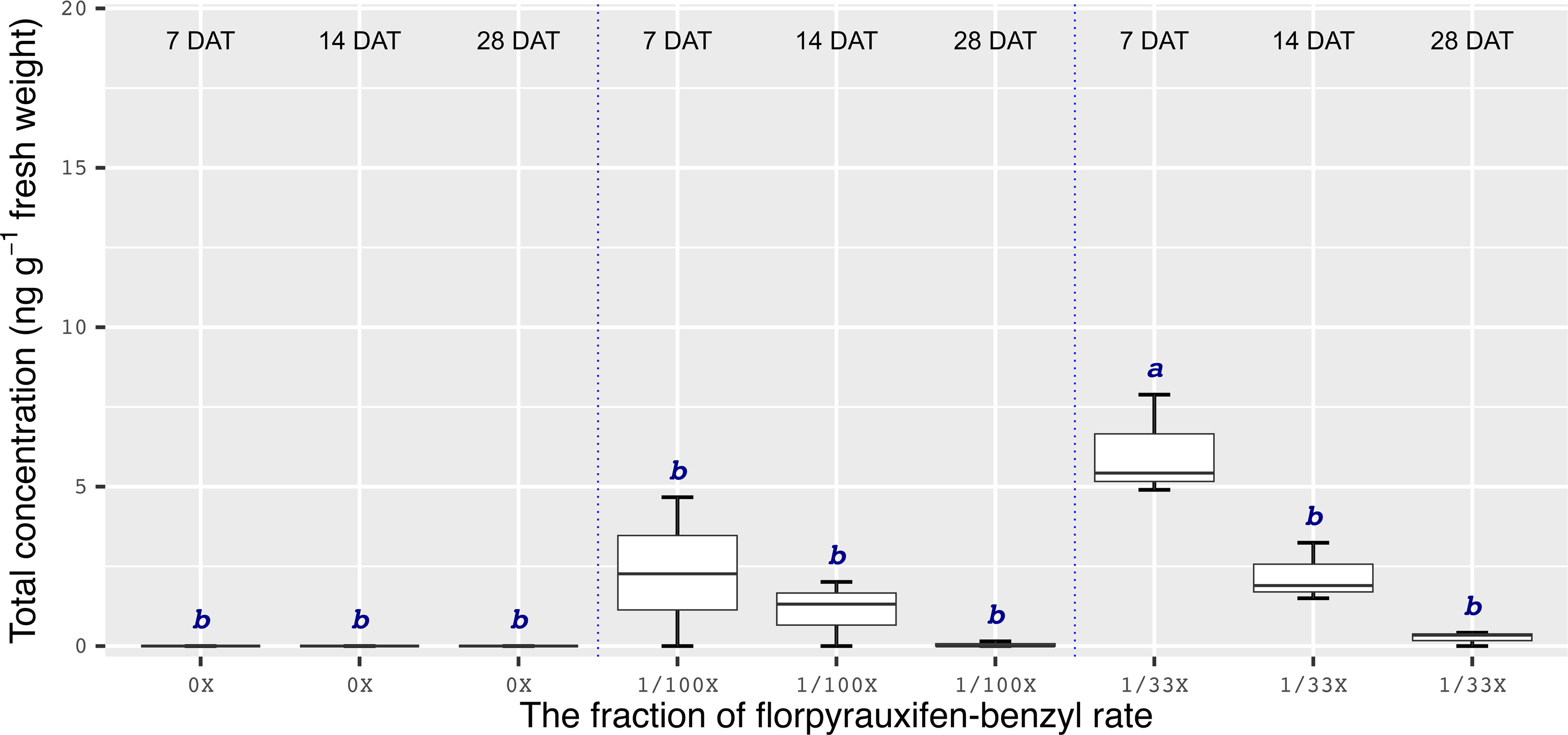
Figure 2. Florpyrauxifen-benzyl residues in almond leaf tissue 7, 14, and 28 DAT with 1/33X and 1/100X simulated drift rates. The rate is expressed as the fraction of the use rate in California rice of 29.4 g ai ha–1. Any two means not followed by the same letter are significantly different at P ≤ 0.05 using Tukey’s HSD.
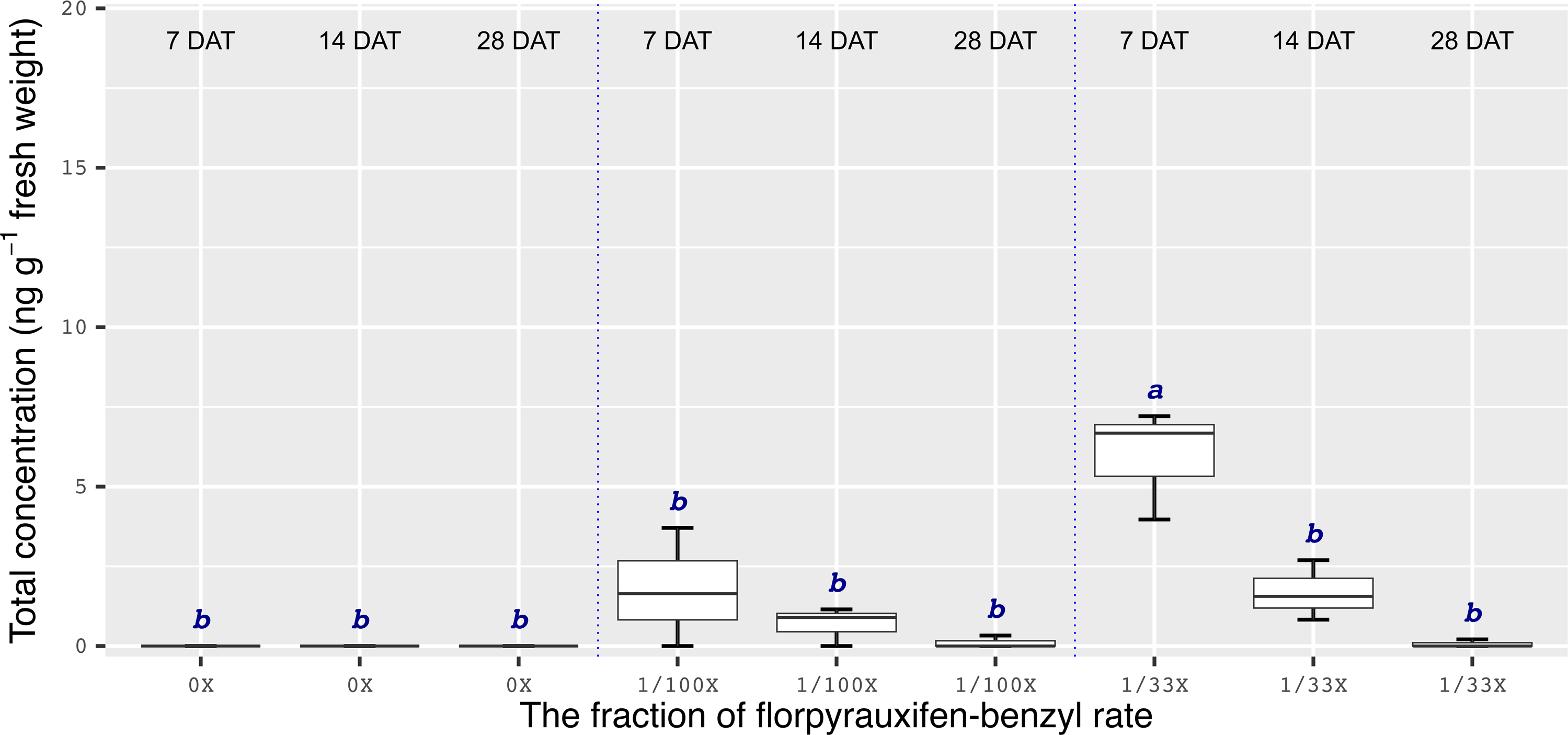
Figure 3. Florpyrauxifen-benzyl residues in pistachio leaf tissue 7, 14, and 28 DAT with 1/33X and 1/100X simulated drift rates. The rate is expressed as the fraction of the use rate in California rice of 29.4 g ai ha–1. Any two means not followed by the same letter are significantly different at P ≤ 0.05 using Tukey’s HSD.
In almonds treated with the 1/33X rate, florpyrauxifen-benzyl residues ranked from 6.06 to 0.25 ng g–1 FW at 7 through 28 DAT, respectively (Figure 2). In pistachio, the 1/33X florpyrauxifen-benzyl-treated leaf samples had 5.95 ng g–1 FW at 7 DAT; however, this decreased to 0.06 ng g–1 FW at 28 DAT (Figure 3). In walnut, the 1/33X-treated leaf samples ranked from 13.12 to 0 ng g–1 FW at 7 through 28 DAT (Figure 4). Walnut leaves had the highest residues at 7 and 14 DAT, but by 28 DAT, residues were below the LOQ (Figure 4).
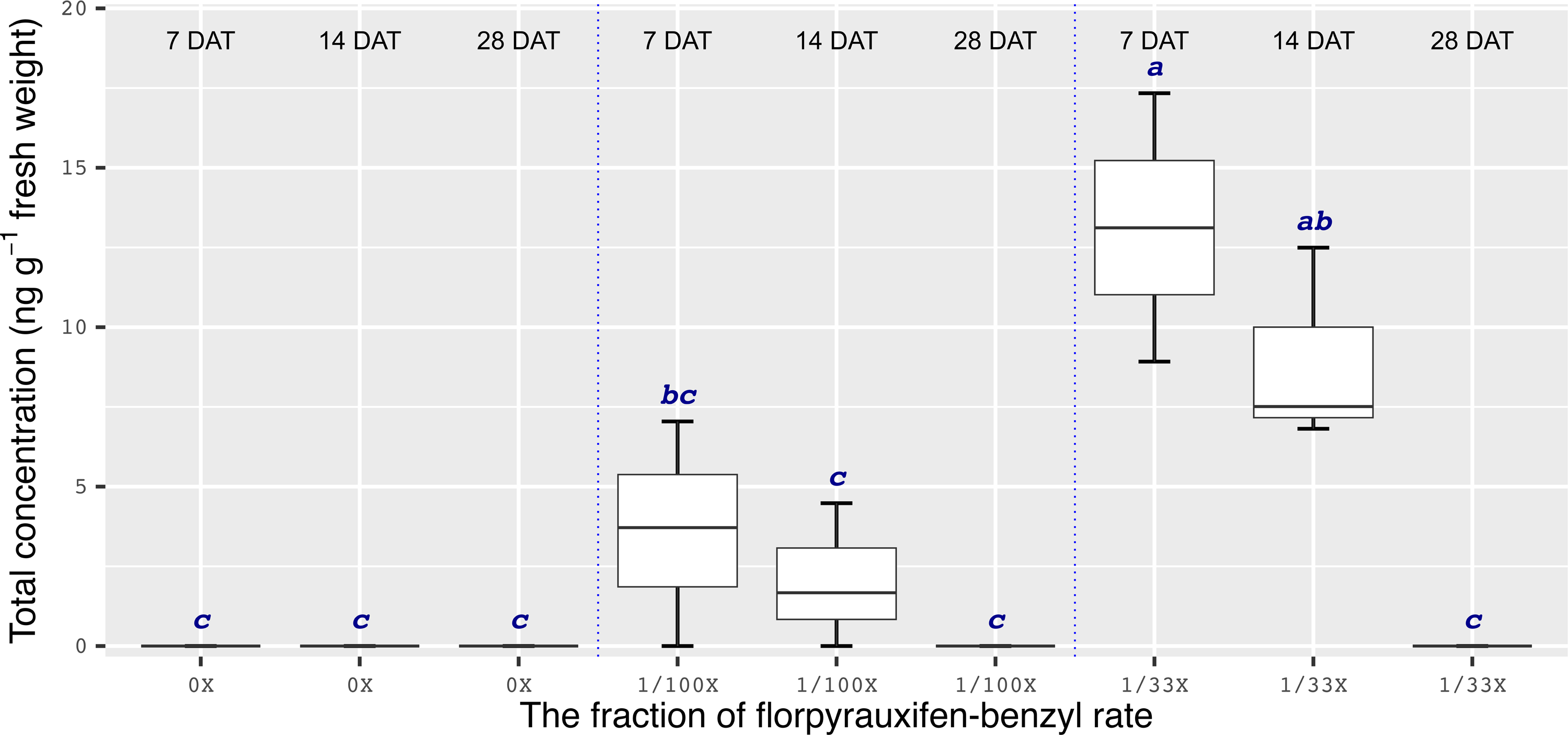
Figure 4. Florpyrauxifen-benzyl residues in walnut leaf tissue 7, 14, and 28 DAT with 1/33X and 1/100X simulated drift rates. The rate is expressed as the fraction of the use rate in California rice of 29.4 g ai ha–1. Any two means not followed by the same letter are significantly different at P ≤ 0.05 using Tukey’s HSD.
The results of this research suggest that the ideal time frame to quantify florpyrauxifen-benzyl residues is <14 d after a drift event. Florpyrauxifen-benzyl symptoms on nut crop trees generally started to appear within 3 to 14 d of exposure and were most severe from 14 to 21 DAT. Therefore, if florpyrauxifen-benzyl drift happens in an almond, pistachio, or walnut orchard, the symptoms may not be recognized until at least 14 d after the herbicide drift event occurred, but by this point, detectable residues would likely be decreasing. While a crop consultant, farm adviser, or grower with an auxin-type herbicide symptomology experience on trees may readily identify the symptoms, it may be too late for accurate residue quantification from the leaf tissues unless the drift exposure is extremely high. Under normal circumstances, drift rates are below 1/100X to 1/33X of the field use rate of an herbicide (Al-Khatib and Peterson Reference Al-Khatib and Peterson1999). The 1/100X drift rate of florpyrauxifen-benzyl caused symptoms on all crops tested in this research in the days and weeks after treatment, but the symptoms decreased gradually during the season for all crops and ultimately disappeared in almond and walnut, but not in pistachio. Florpyrauxifen-benzyl residues were not detectable in the leaf tissue at 28 DAT for walnut at any drift rate. Moreover, florpyrauxifen-benzyl at the 1/100X rate was detectable only near or below the lowest quantifiable standard concentration (0.2 ng ml–1 on the instrument) out of one sample for almond and pistachio at 28 DAT. This observation suggests that investigations of suspected florpyrauxifen-benzyl drift on almond, pistachio, and walnut should not be based entirely on florpyrauxifen-benzyl leaf residue, especially when tissue samples are taken 14 d or longer after suspected exposure.
Practical Implications
Increasing herbicide resistance has led to the necessity for complex herbicide programs with different modes of action in California rice. Florpyrauxifen-benzyl is becoming an important herbicide in season-long weed management programs owing to its activity on grass, sedge, and broadleaf weeds and to its broad application window. Florpyrauxifen-benzyl can be applied up to two foliar applications from the 2-leaf rice growing stage to 60 d prior to harvest at 40 g ai ha–1 within 14-d intervals. In the Sacramento Valley, these applications generally occur between May and mid-July, when almond, pistachio, and walnut are highly sensitive to herbicide drift. Pesticide applicators should use extra caution with florpyrauxifen-benzyl applications, particularly near pistachio orchards. Results from this research suggest that florpyrauxifen-benzyl residues in leaf tissue may decrease even before leaf symptoms reach peak severity. Therefore any florpyrauxifen-benzyl drift case investigations need to consider symptomology, weather conditions, and application records in the area and not rely solely on chemical residue analyses.
Acknowledgments
We gratefully acknowledge the efforts of Seth Watkins, Guelta Laguerre, and Maya Delong for their assistance with the fieldwork and Elizabeth Leonard and Nishanth Tharayil at Multi-User Analytical Lab, Clemson University, for the chemical analysis on this project.
Funding
This research was funded by the California Rice Research Board (grants RR20-7 and RR21-9), the Melvin D. Androus Endowment, and a University of California Henry A. Jastro–Shields Graduate Research Award.
Competing interests
The authors declare no conflicts of interest.