Menarche (first menses) is a complex physiological event that occurs after full reactivation of the hypothalamic gonadotropin releasing hormone secretory system, which initially develops and is temporarily active during fetal and the first 6 months of life (DiVall & Radovick, Reference DiVall and Radovick2008). Age at menarche (AAM) has important health and behavioral implications. Early menarche is known to be a risk factor for obesity (Ong et al., Reference Ong, Northstone, Wells, Rubin, Ness, Golding and Dunger2007), type 2 diabetes (He et al., Reference He, Zhang, Hunter, Hankinson, Buck Louis, Hediger and Hu2010), adolescent depression (Kaltiala-Heino et al., Reference Kaltiala-Heino, Kosunen and Rimpela2003), conduct problems (Burt et al., Reference Burt, McGue, DeMarte, Krueger and Iacono2006), and precocious sexual activity (Jorm et al., Reference Jorm, Christensen, Rodgers, Jacomb and Easteal2004), whereas late menarche is associated with an increased risk of osteoporosis (Naves et al., Reference Naves, Diaz-Lopez, Gomez, Rodriguez-Rebollar and Cannata-Andia2005) and low fertility (Komura et al., Reference Komura, Miyake, Chen, Tanizawa and Yoshikawa1992; Presser, Reference Presser1978).
Although the downward trend in AAM in Asian and European countries is well established (Ahn et al., Reference Ahn, Lim, Song, Seo, Lee, Kim and Lim2013; Hosokawa et al., Reference Hosokawa, Imazeki, Mizunuma, Kubota and Hayashi2012; Hur, Jin et al., Reference Hur, Jin and Lee2019; McDowell et al., Reference McDowell, Brody and Hughes2007; Talma et al., Reference Talma, Schönbeck, van Dommelen, Bakker, van Buuren and Hirasing2013), our understanding of the trend of AAM in African countries remains relatively poor because systematic studies based on large samples are scarce. Recently, using 1,222,338 women between the ages of 15 and 49 years in 27 low- and middle-income countries, including many Africans, Leone and Brown (Reference Leone and Brown2020) demonstrated that the mean AAM declined from 14.66 years (95% CI [14.34, 14.98]) for the 1932 birth cohorts to 12.86 years (95% CI [12.64, 13.07) for the 2002 birth cohorts. On the basis of a large set of data (N = 47,356; birth years from 1935 to 1965) from the World Fertility Survey conducted in eight sub-Saharan African countries, Garenne (Reference Garenne2020) found that the mean AAM ranged from 14.11 years (Nigeria) to 15.26 years (Rwanda). In all eight countries, decreasing trends in AAM were statistically significant and averaged 0.47 years per period of 30 years (1935−1965), comparable to the rate of decline in Europe in the 19th century. Especially, in Nigeria, the mean AAM decreased by 0.6 years from 1930 to 1960 birth cohorts (14.5 years to 13.9 years), indicating a decline of 2.4 months per decade. These decreases have been attributed mostly to improved nutrition and changes in body mass index, as well as improvements in public health and socioeconomic status in African countries (Garenne, Reference Garenne2020).
Although secular decreases are likely driven by environmental influences, twin studies and genomewide association studies (GWAS) have demonstrated that AAM is significantly influenced by genes. About 45% (Snieder et al., Reference Snieder, MacGregor and Spector1998) to 95% (Loesche et al., Reference Loesch, Huggins, Rogucka, Hoang and Hopper1995) of the variance in AAM have been reported to be associated with additive genetic influences, with the remaining variance being attributable to nonshared environment plus measurement error. GWAS have shown that genes for hormonal regulation, cellular development, body weight regulation, and other biological functions are associated with AAM (Day et al., Reference Day, Thompson, Helgason, Chasman, Finucane, Sulem, Ruth, Whalen, Sarkar, Albrecht, Altmaier, Amini, Barbieri, Boutin, Campbell, Demerath, Giri, He, Hottenga and Perry2017; Delahanty et al., Reference Delahanty, Beeghly-Fadiel, Long, Gao, Lu, Xiang, Zheng, Ji, Wen, Cai, Zheng and Shu2013; Elks et al., Reference Elks, Perry, Sulem, Chasman, Franceschini, He, Lunetta, Visser, Byrne, Cousminer, Gudbjartsson, Esko, Feenstra, Hottenga, Koller, Kutalik, Lin, Mangino, Marongiu and Murray2010; Perry et al., Reference Perry, Day, Elks, Sulem, Thompson, Ferreira, He, Chasman, Esko, Thorleifsson, Albrecht, Ang, Corre, Cousminer, Feenstra, Franceschini, Ganna, Johnson, Kjellqvist and Ong2014), although genes discovered to date explained only about 7.4% of the population variance in AAM, corresponding to approximately 25% of the estimated heritability (Day et al., Reference Day, Thompson, Helgason, Chasman, Finucane, Sulem, Ruth, Whalen, Sarkar, Albrecht, Altmaier, Amini, Barbieri, Boutin, Campbell, Demerath, Giri, He, Hottenga and Perry2017). As twin studies and GWAS of AAM conducted so far mostly focused on Asians and Europeans (Day et al., Reference Day, Thompson, Helgason, Chasman, Finucane, Sulem, Ruth, Whalen, Sarkar, Albrecht, Altmaier, Amini, Barbieri, Boutin, Campbell, Demerath, Giri, He, Hottenga and Perry2017; Delahanty et al., Reference Delahanty, Beeghly-Fadiel, Long, Gao, Lu, Xiang, Zheng, Ji, Wen, Cai, Zheng and Shu2013; Elks et al., Reference Elks, Perry, Sulem, Chasman, Franceschini, He, Lunetta, Visser, Byrne, Cousminer, Gudbjartsson, Esko, Feenstra, Hottenga, Koller, Kutalik, Lin, Mangino, Marongiu and Murray2010; Perry et al., Reference Perry, Day, Elks, Sulem, Thompson, Ferreira, He, Chasman, Esko, Thorleifsson, Albrecht, Ang, Corre, Cousminer, Feenstra, Franceschini, Ganna, Johnson, Kjellqvist and Ong2014; Pyun et al., Reference Pyun, Kim, Cho, Koh, Lee, Shin and Kwack2014; Shi et al., Reference Shi, Zhang, Choi, Gao, Li, Lu, Long, Kang, Xiang, Wen, Park, Ye, Noh, Zheng, Wang, Chung, Lin, Cai and Shu2016), heritability of AAM in Africans remains largely unknown.
The goal of the current article was to report genetic and environmental influences on AAM in adolescent twins attending public schools in Lagos State, Nigeria. Lagos State is located in the southwestern part of Nigeria, where urbanization and industrialization are rapidly ongoing. Lagos State includes Lagos, the most prosperous and populous city in sub-Saharan Africa. However, there are considerable variations in economic distribution among people living in Lagos State (World Bank Group, 2013). As our twin participants were those attending public schools in Lagos State, they were mostly of lower socioeconomic class in Nigeria. For example, the Lagos State Ministry of Education reported that in 2014, on average, junior secondary public school students suffered from 39% shortage of classrooms, 74% shortage of school furniture, and 45% shortage of toilet facilities; and senior secondary school students, from 23% shortage of classrooms, 62% shortage of school furniture, and 21% shortage of toilet facilities (Lagos State Ministry of Education, 2019). Prior studies found that females who reside in rural areas and come from lower socioeconomic classes experienced significantly delayed menarche as compared to urban dwellers and females from middle or high social class families (Irewole-Ojo et al., Reference Irewole-Ojo, Senbanjo, Oduwole and Njokanma2018; Karapanou & Papadimitriou, Reference Karapanou and Papadimitriou2010).
The incidence of teenage pregnancy is high (about 23% on average) in Nigeria, which is a major public health concern because of its association with the risk of sexually transmitted diseases and reproductive tract infections, and mortality for both the mother and the child (National Population Commission and IFC International, 2014). Given the significant association between early menarche and precocious coital activities, it is important to study the etiology of AAM in Nigerian females to develop effective interventions to prevent teenage pregnancy and improve reproductive health.
Methods
Sample and Measure
The sample includes 1803 adolescent female twins aged from 12 to 18 years, consisting of 454 monozygotic (MZ) twins (226 complete pairs and 2 cotwin missing cases), 823 same-sex dizygotic (DZ) twins (408 complete pairs and 7 cotwin missing cases), and 526 female members of opposite-sex (OS) DZ twins drawn from the Nigerian Twin and Sibling Registry (Hur Jeong et al., Reference Hur, Jeong, Kang, Ajose, Kim, Beck, Hottenga, Mbarek, Finnicum, Ehli, Martin, de Geus, Boomsma, Davies and Bates2019). The mean (SD) age of twins was 14.57 (±1.70) years. In terms of year of birth, the oldest twin in this study sample was born in 1995, and the youngest in 2002. Details of the recruitment procedure of the study sample can be found in Hur et al. (Reference Hur, Jeong, Chung, Shin and Song2013) and Hur, Jeong et al. (Reference Hur, Jeong, Kang, Ajose, Kim, Beck, Hottenga, Mbarek, Finnicum, Ehli, Martin, de Geus, Boomsma, Davies and Bates2019). In brief, twins were recruited from 272 public junior and senior secondary schools in Lagos State, Nigeria in 2013 and 2014. These 272 schools represented 42.6% of the total public junior and senior secondary schools in Lagos State, and were selected for their large enrollment size (typically, greater than 500 students in each school). A questionnaire book was given to twins in a library or a special classroom in each school. The questionnaire book included a question on AAM where female twins were asked to write the year and the month of their first menstruation if they had started menstruation. As most postmenarcheal girls provided the year but not the month of menarche, the current report defined AAM by age in years only.
During the testing session, a saliva sample was taken and analyzed to determine twins’ zygosity. Eighteen microsatellite markers of DNA, including amelogenin, were analyzed to test zygosity of twins. Opposite-sex twins were assigned as DZ twins without zygosity testing. A much larger number of DZ than MZ twins in the present sample likely reflected twin birth rates in Nigeria (MacGillivray, Reference MacGillivray1986).
Statistical Analysis
Of 1803 twins, only 898 answered the menarche question, leaving 905 for missing values. The missing cases included those who had not experienced menarche as well as those who did not recall AAM. Unfortunately, the two groups could not be separated clearly because we did not make separate options in the question. However, the number of missing depended on the age of twins (r =−.44) such that at age 12, the number of missing was 221, while at age 18, it was only 26. These suggested that younger twins likely skipped the question because they had not experienced menarche, while older twins skipped the question for they did not recall. Age of twins was also a strong covariate of AAM (r = .49).
To impute missing values, we employed the Expectation–Maximization (EM) algorithm (Dempster et al., Reference Dempster, Laird and Rubin1977), in which an iterative method was used to find maximum likelihood estimates of parameters on the basis of existing data on AAM, age, year of menarche, and zygosity. To estimate the mean and median values of AAM, Kaplan–Meier’s survival analysis was conducted on the basis of imputed data.
Twin studies make use of the difference in the proportion of genes shared between MZ and DZ twins. Utilizing this difference, the total variance of AAM is partitioned into three components: additive genetic (A; MZ = 1.0, DZ = 0.5), shared environment (C; MZ = 1.0, DZ = 1.0), and nonshared environment plus measurement error (E; MZ = 0, DZ = 0). Using a raw data approach in Mx, we computed maximum likelihood twin correlations and performed univariate model-fitting analysis to estimates parameters, A, C, and E and compare alternative models. Twin correlational and model-fitting analyses were conducted based only on MZ (82 complete pairs and 38 cotwin missing cases) and DZ twins (157 complete pairs and 99 cotwin missing cases) who reported AAM. Data were adjusted for age to control the main effect of age using a regression analysis.
Using the difference in twice the negative log-likelihood (-2LL) of the models, which is distributed as a chi-square, the fit of the full ACE model was compared to that of submodels to determine the best fitting, most parsimonious model for the data. A significant (p < .05) change in chi-square in the submodel indicates that elimination of the parameters from the full model significantly worsens the fit of the model, and therefore, the parameters should be retained. However, a nonsignificant change in chi-square indicates that the parameter should be eliminated from the full model as models with fewer parameters are more parsimonious and therefore preferable. When submodels are not nested to each other, Akaike’s information criterion (AIC = −2LL, −2df) was used to evaluate alternative models. A model with lower AIC is considered as a better one (Akaike, Reference Akaike1987).
Results
Descriptive Statistics
Figure 1 displays cumulative distribution of AAM in the present sample. Kaplan–Meier analysis yielded 13.23 years (95% CI [13.18, 13.28]) for the mean value and 13.00 years (95% CI [12.96, 13.04]) for the median of AAM. Kaplan–Meier survival distributions showed that means were not significantly different across zygosity (χ2 (1) = 2.11, p = .15; 13.17 years vs. 13.25 years) or between the first- and the second-born twins (χ2 (1) = 2.94, p = .09; 13.27 years vs. 13.19 years).
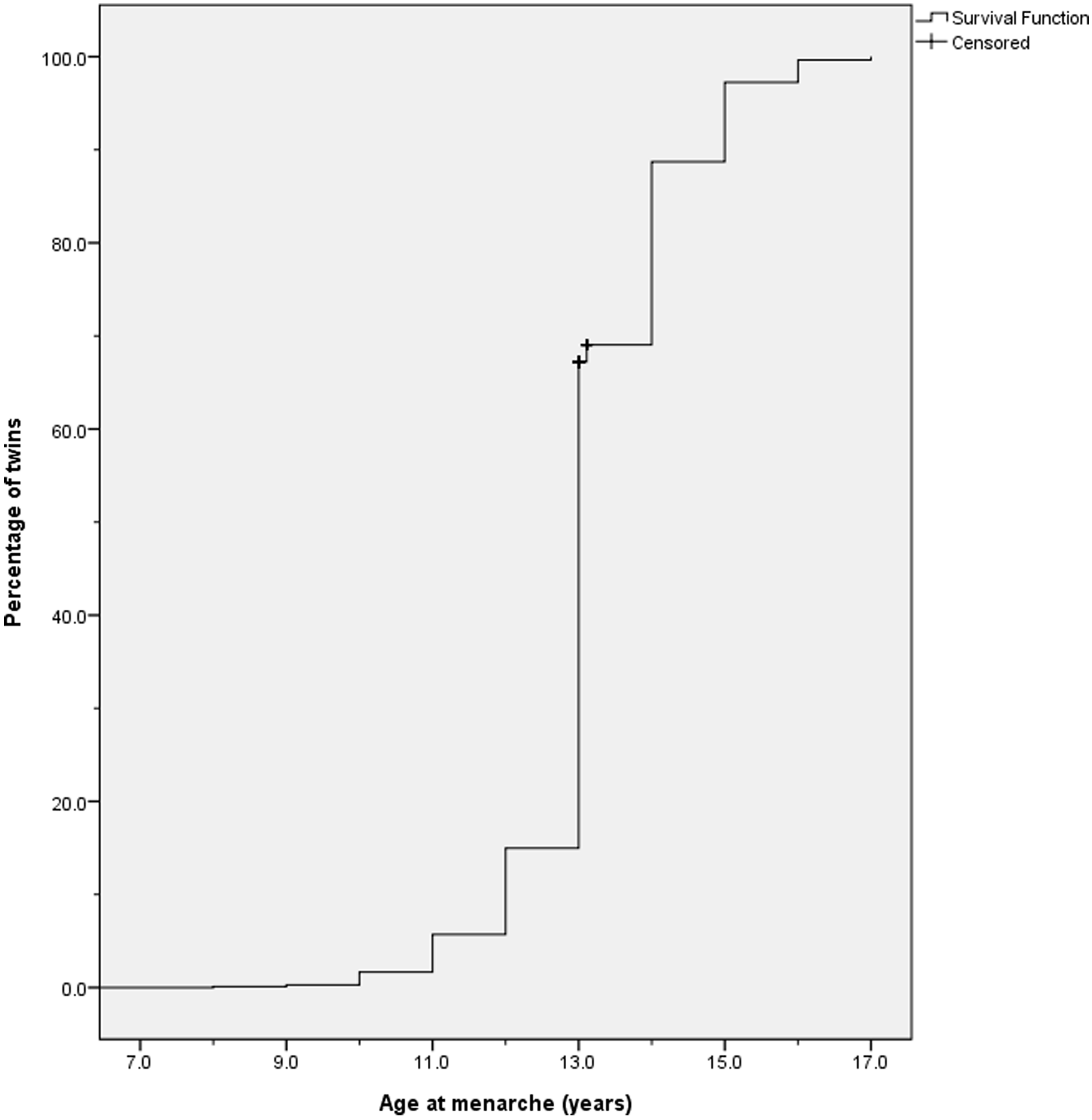
Fig. 1. Cumulative distribution of age at menarche (N = 1803).
Twin Correlations and Model Fitting
Maximum likelihood MZ and DZ twin correlations for AAM were .63 (95% CI [.48, .74]) and .33 (95% CI [.19, .45]) respectively. The MZ twin correlation was significantly higher than the DZ twin correlation without an overlap there of 95% confidence intervals. This pattern of twin correlations suggested significance of additive genetic influences and little shared environmental influences on AAM.
Table 1 presents the results of univariate model-fitting analysis. Consistent with twin correlations, the AE model was the best-fitting one, where additive genetic and nonshared environmental influences were 58% (95% CI [46, 67]) and 42% (95% CI [33, 54]), respectively. Shared environmental influences were not significant, consistent with findings from Asian and western twin samples (Hur, Jin et al., Reference Hur, Jin and Lee2019; Treloar & Martin, Reference Treloar and Martin1990).
Table 1. Results of model-fitting analysis for age at menarche in Nigerian adolescents

Note. a95% CI incalculable. −2LL = −2 log likelihood. A, additive genetic influence; C, shared environmental influence; E, nonshared environment and measurement error. The best-fitting model is indicated in bold.
Discussion
To our knowledge, this study is the first to report heritability of AAM in African adolescents. The mean AAM (13.23 years) in our sample was between 13.13 years reported by Ajah et al. (Reference Ajah, Onubogu, Anozie, Lawani, Iyoke, Onwe and Ajah2015) and 13.44 years found by Onyiriuka and Egbagbe (Reference Onyiriuka and Egbagbe2013). Both studies were based on Nigerian secondary school girls whose birth years were similar to ours. However, our mean was much lower than the mean AAM (13.9 years) found in a large sample of Nigerian females in the birth cohort of 1965 (Garenne, Reference Garenne2020). This discrepancy suggests that the mean AAM continued to decline in Nigerians like in many countries in the world. As our twins were born between 1995 and 2002, our results suggest that the mean AAM decreased by 0.62 years during the past three decades. The rate of decline was nearly identical to that found in the Garenn’s study.
Heritability of .58 (95% CI [.46, .67]) found in our sample is within the range of heritability estimates found in Asian and Western twin samples. Recently, in a metaanalysis of GWAS of AAM in African-American women (N = 18,089), Demerath et al. (Reference Demerath, Liu, Franceschini, Chen, Palmer, Smith, Chen, Ambrosone, Arnold, Bandera, Berenson, Bernstein, Britton, Cappola, Carlson, Chanock, Chen, Chen, Deming and Haiman2013) showed that 60% of menarche loci identified in European women contained variants significantly associated with AAM in African-American women. Given genetic and environmental differences between African and African-American women, however, a GWAS of AAM needs to be conducted on the basis of African women living in African countries to better understand the etiology of AAM and reproductive health in Africans.
There are a few limitations in our study. First, our data had many missing responses, and we were not able to differentiate between censored observations and missing responses resulting from forgetting or other reasons. In Nigeria, the process of menstruation is still a social taboo and dealt with secretly (Goon et al., Reference Goon, Toriola, Uever, Wuam and Toriola2010), which may have increased missingness in our data. For the purpose of comparison, we computed heritability based on the imputed data (N = 1803), which yielded a higher estimate of nonshared environmental influence (52%; 95% CI [44%, 61%]) and a lower estimate of heritability (48%; 95% CI [39, 56), as compared to those found in MZ and DZ twins who reported their AAM. We think the discrepancies were due to increased measurement error associated with the process of imputation, given that measurement error is confounded with nonshared environmental influence in our model-fitting analysis (Neale & Cardon. Reference Neale and Cardon1992). Overall, however, as our twins were adolescents (12–18 years), the intervals between the actual menarche and the time of report were likely short in most twins. Thus, the recall error in those who responded may be quite small. Another limitation is that our study sample was based on twins attending public schools covering poor regions in Lagos state in Nigeria, and our results therefore may not be generalized to those residing in upper socioeconomic neighborhoods in Nigeria or other countries in Africa.
Acknowledgments
This research was supported by Ulster Institute for Social Research (UK) to the first author (YMH). We would like to thank twins who participated in this study and schoolteachers and principals in public schools in Lagos State, the staff members in the Ministry of Education in Lagos State, and many research assistants who kindly assisted this study.