Introduction
The present shape of the coastline of the southern North Sea is the result of the interaction of various factors, for example, the glacial/periglacial Weichselian conditions (e.g. coversands, fluvial drainage systems) and deposits, the reorganisation of these conditions during the Holocene transgression, and – since the 9th century AD – coastal engineering (terps, land reclamation, diking) (Behre, Reference Behre2004, Reference Behre2007; Streif, Reference Streif2004; Vink et al., Reference Vink, Steffen, Reinhardt and Kaufmann2007; Vos & Knol, Reference Vos and Knol2015; Vos et al., Reference Vos, van den Meule, Weerts and Bazelmans2020). The reconstruction of the Quaternary evolution of this coastal zone is mainly based on core data from a variety of approaches (coastal engineering, hydrology, resources, research), which results in large data sets providing among others litho-, bio- and chronostratigraphic data and palaeoenvironmental, palaeoecological and sedimentological interpretations (for a review of the Dutch Wadden Sea data sets see Pierik & Cohen, Reference Pierik and Cohen2020; for Lower Saxony data sets see NIBIS® Kartenserver 2020a–c).
The existing palaeoenvironmental reconstructions of NW Germany have mainly focused on the Holocene. Vos & Knol (Reference Vos and Knol2015) and Karle et al. (Reference Karle, Bungenstock and Wehrmann2021) presented a comprehensive compilation of these results. To date, research on the Pleistocene deposits has mainly concentrated on the reconstruction of the locations of the ice margins for glacial periods, or coastlines for interglacials (e.g. Streif, Reference Streif2004; Ehlers et al., Reference Ehlers, Grube, Stephan, Wansa, Ehlers, Gibbard and Hughes2011). The reconstruction of Pleistocene surfaces, however, has been largely neglected as few reliable data are available. As a consequence, the presence of Eemian deposits in the study area is still unclear. According to Dechend (Reference Dechend1950, Reference Dechend1952, Reference Dechend1954, Reference Dechend1958), these deposits are present, but according to Sindowski (Reference Sindowski1973) and Streif (Reference Streif1990) they are not.
Within the frame of the joint WASA project (The Wadden Sea as an archive of landscape evolution, climate change and settlement history; Bittmann et al., Reference Bittmann, Bungenstock and Wehrmann2021) this study was initiated in order to reconstruct the regional Holocene coastal evolution in the light of environmental changes controlled by climate, local hydrology and sedimentary processes. An interdisciplinary data set, including hydroacoustic profiles, sediment cores and multi-proxy data (e.g. radiocarbon dating, marine micro- and macrofauna ecology, pollen analysis) was generated and analysed, in order to examine both the chronology and environmental changes, which occurred across the region within the determined time frame. To extend the detailed information inferred from point-based data sets (e.g. cores) to a broader area, we followed an integrated approach using hydroacoustic data (e.g. Wunderlich & Müller, Reference Wunderlich and Müller2003) to reduce the coarsening effect due to interpolation between points (cores). This is particularly true in the case of small-scale articulated stratigraphic sequences and palaeomorphologies, where the interpolation among point-based data can omit the local variability. In addition, the ability to resolve and describe the local stratigraphy at high resolution is an essential requirement for palaeoclimatological studies (e.g. Eriş et al., Reference Eriş, Çağatay, Akçer, Gasperini and Mart2011; Novak et al., Reference Novak, Busetti, Zgur, Baradello, Babich, Cova, Gordini, Romeo, Tomini, Poglajen, Diviacco and Vrabec2017) as it allows the evolution of sedimentary successions to be deciphered also in settings with low sedimentation rates and/or significant erosion or non-deposition.
Several authors have demonstrated that hydroacoustic data, and more specifically shallow sub-bottom profiling, can be successfully used to identify and describe glacial and interglacial deposits (e.g. Grant & Schreiber, Reference Grant and Schreiber1990; Bart & Anderson, Reference Bart, Anderson, Cooper, Barker and Brancolini1997; Wunderlich & Müller, Reference Wunderlich and Müller2003; Bart & Santis, Reference Bart and Santis2012; Lindeque et al., Reference Lindeque, Gohl, Henrys, Wobbe and Davy2016; Saleh & Rabah, Reference Saleh and Rabah2016) due to their contrasting lithologies. This is an essential requirement for the reconstruction of the Pleistocene/Holocene succession, for example, in the southern Baltic Sea (Gelumbauskaitė, Reference Gelumbauskaitė2000; Niedermeyer et al., Reference Niedermeyer, Verse and Bauerhorst2002). Ottesen et al. (Reference Ottesen, Dowdeswell and Bugge2014), Buckley (Reference Buckley2017), Lamb et al. (Reference Lamb, Huuse and Stewart2017) and Coughlan et al. (Reference Coughlan, Fleischer, Wheeler, Hepp, Hebbeln and Mörz2018) have already applied this technique to North Sea data sets, although mainly in studies of offshore areas.
The tidal inlets are considered to be highly dynamic systems mainly controlled by tidal prism and geological factors (FitzGerald, Reference FitzGerald2015). As they cut deep into the Holocene coastal sediment wedge, they may expose the underlying Pleistocene or older strata. The analysis and understanding of the entire stratigraphic succession is essential when estimating the effect of Pleistocene deposits and/or morphologies on the evolution of Holocene tidal channel systems. Up to now there have been no extensive investigations for this time interval (Pleistocene/Holocene) in the tidal inlets of the German Wadden Sea.
The main aim of this study is to analyse the Middle Pleistocene to early Holocene subsurface geology in the deepest part of the Norderney tidal basin using an interdisciplinary approach and a broad range of data sets. Additionally, we want to combine point-based core log data and high-resolution sub-bottom profiler transects (Fig. 1) to reconstruct on a broader scale the influence of glacial and interglacial depositional processes on the coastal development of the East Frisian coast, and, in particular, the spatial transition from the tidal inlet to the island of Norderney, namely the Riffgat channel. For the core database we have designed a sediment facies catalogue for the Pleistocene/Holocene depositional palaeoenvironments.
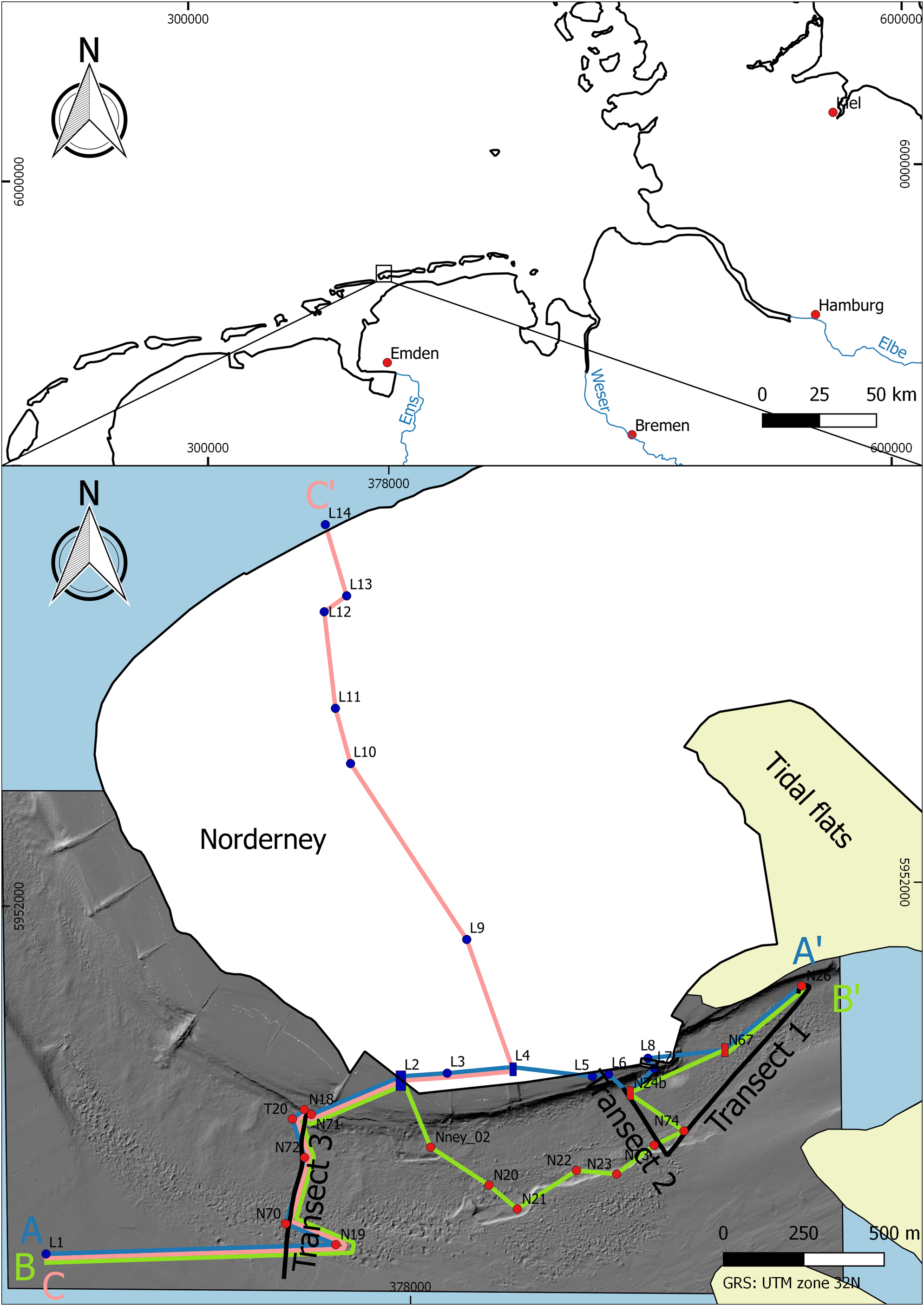
Fig. 1. Top: map of Northern Germany with the study area. Bottom: study area with the location of cores (red: WASA cores; blue: LBEG cores; L1 = Nordeney B13, L2 = Norderney I 26 BI Werfthalle, L3 = Norderney I 33 BVI Werfthalle, L4 = Norderney I 40 BXIII Werfthalle, L5 = Norderney 3/79 Hafen, L6 = Norderney 2/79 Hafen, L7 = Norderney 3 Hafen Fährbett, L8 = Norderney 1 Hafen Fährbett, L9 = Norderney Bohrlochreihe II. Nr. 1–2, L10 = Norderney H 51 Kinderheim Seldhausenstr., L11 = Norderney H 53 Kinderheim, L12 = Norderney H52 Kinderheim Luciusstr., L13 = Norderney B 6-1, L14 = Norderney B 7-1), sub-bottom transects and cross-sections. Data source: bathymetric data used with permission of the Lower Saxony Water Management, Coastal Defence and Nature Conservation Agency (NLWKN)
Geological setting
The North Sea is a marginal sea and forms part of the NW European shelf. The Quaternary-age geological history of the North Sea Basin was mainly influenced by the processes associated with glaciation, which resulted in the deposition of a thick sedimentary succession including a variety of glacial, periglacial, fluvial and marine deposits (Phillips et al., Reference Phillips, Hodgson and Emery2017). During glacial phases, ice masses extended from Scandinavia and Great Britain across Northern Germany (Ehlers et al., Reference Ehlers, Grube, Stephan, Wansa, Ehlers, Gibbard and Hughes2011), while the interglacial phases were characterised by sea-level rise and related transgressive depositional conditions (Streif, Reference Streif2004). During the last glaciation (Weichselian) the sea level dropped to 130 m below the current sea level. A marked rise in eustatic sea level followed the Last Glacial Maximum (LGM) at ∼11.7 ka BP and was related to the melting of the Fennoscandian and Laurentide ice sheets. Eustatic sea-level rise and glacio-isostatic adjustment induced a shift of the shoreline of almost 600 km to the south (e.g. Kiden et al., Reference Kiden, Denys and Johnston2002; Streif, Reference Streif2004; Vink et al., Reference Vink, Steffen, Reinhardt and Kaufmann2007; Hijma & Cohen, Reference Hijma and Cohen2010). At around 6–7 ka BP, when the North Sea reached the position of the present-day coastal area (e.g. Streif, Reference Streif2004; Flemming, Reference Flemming, Davis and Dalrymple2012), Holocene peat deposits and siliciclastic sediments accumulated on top of the Pleistocene sands, reaching thicknesses of up to 25 m (Streif, Reference Streif2004). Changes of the ratio of accommodation space and sediment supply led to the formation of prograding swamps, resulting in peat layers intercalated in the shallow subtidal to intertidal sediments of the Holocene-age succession (Streif, Reference Streif2004; Bungenstock et al., Reference Bungenstock, Freund and Bartholomä2021).
Glacial and interglacial history of Northern Germany
Two Elsterian ice advances (marine isotope stage (MIS) 10, 400–320 ka BP, cf. Ehlers et al., Reference Ehlers, Meyer and Stephan1984; Litt et al., Reference Litt, Behre, Meyer, Stephan and Wansa2007) have been recorded in Northern Germany, the older of which extends as far south as the low mountain range (Central Germany), while the second is of more limited extent (Stackebrandt, Reference Stackebrandt2009; Murton & Murton, Reference Murton and Murton2012). Tunnel valleys developed beneath the ice masses, as described by a number of authors (e.g. Ehlers & Linke, Reference Ehlers and Linke1989; Streif, Reference Streif1990; Lutz et al., Reference Lutz, Kalka, Gaedicke, Reinhardt and Winsemann2009; Stackebrandt, Reference Stackebrandt2009). In general, these tunnel valleys are 1–100 km long (average 30 km), and 1–4 km wide (some are up to 8 km). The maximum depth of substratum erosion by these tunnel valleys is between 120 and 400 m (Streif, Reference Streif1990; Lutz et al., Reference Lutz, Kalka, Gaedicke, Reinhardt and Winsemann2009; Stackebrandt, Reference Stackebrandt2009).
The Holsteinian Interglacial (MIS 9, 320–310 ka BP, cf. Geyh & Müller, Reference Geyh and Müller2005; Litt et al., Reference Litt, Behre, Meyer, Stephan and Wansa2007) occurred between the Elsterian and the Saalian. Holsteinian marine deposits have not been found in the study area, while terrestrial lacustrine deposits have been noted in the surrounding area (Streif, Reference Streif1990; Sierralta et al., Reference Sierralta, Urban, Linke and Frechen2017). Coastal conditions within the Holsteinian were quite similar to those of the Holocene, with the coast located to the north of its present-day position in the East Frisian region.
Three major ice advances have been recorded during the Saalian (MIS 8–6, 310–126 ka BP, cf. Litt et al., Reference Litt, Behre, Meyer, Stephan and Wansa2007), namely the Main Drenthe, the Younger Drenthe and the Warthe Stadium (Ehlers et al., Reference Ehlers, Grube, Stephan, Wansa, Ehlers, Gibbard and Hughes2011). Among them, only the Main Drenthe advance affected the area of the modern East Frisian Islands (Rappol et al., Reference Rappol, Haldorsen, Jørgensen, van der Meer and Stoltenberg1989; Streif, Reference Streif1990; Meyer, Reference Meyer2005; Litt et al., Reference Litt, Behre, Meyer, Stephan and Wansa2007; Graham et al., Reference Graham, Stoker, Lonergan, Bradwell, Stewart, Ehlers, Gibbard and Hughes2011), resulting in the deposition of outwash and moraine deposits (Streif, Reference Streif1990). A phase of warm, (sub-)continental climate conditions, which occurred during the Saalian, is termed the Dömnitz temperate stage (MIS 7, 227–180 ka BP, cf. Litt et al., Reference Litt, Behre, Meyer, Stephan and Wansa2007; Urban, Reference Urban2007). This temperate stage was characterised by the development of lacustrine conditions across Northern Germany, and the deposition of a series of muds and peats (Urban et al., Reference Urban, Thieme and Elsner1988; Urban, Reference Urban2007; Sierralta et al., Reference Sierralta, Urban, Linke and Frechen2017).
The subsequent climatic amelioration, which characterised the onset of the Eemian (MIS 5e, 126–115 ka BP, cf. Shackleton et al., Reference Shackleton, Sánchez-Goñi, Pailler and Lancelot2003; Schokker et al., Reference Schokker, Cleveringa and Murray2004) represents the final interglacial period of the Pleistocene (Caspers et al., Reference Caspers, Merkt, Müller and Freund2002; Litt et al., Reference Litt, Behre, Meyer, Stephan and Wansa2007). During this period the eustatic sea-level rise resulted in a higher sea level than that of the subsequent Holocene (Turner, Reference Turner2000; Streif, Reference Streif2004; Ehlers et al., Reference Ehlers, Grube, Stephan, Wansa, Ehlers, Gibbard and Hughes2011). However, the Central Wadden Sea region was less affected by the rising sea level, due to the coeval uplift related to the ongoing glacio-isostasic adjustment. According to Sindowski (Reference Sindowski1973), Eemian-age deposits in the East Frisian region can be subdivided into three facies: marine, fluvial, and limnic to semi-terrestrial. Eemian marine deposits in the area of Norderney and the Ems Estuary were investigated in detail by Dechend (Reference Dechend1950, Reference Dechend1952, Reference Dechend1954, Reference Dechend1958) and Dechend & Sindowski (Reference Dechend and Sindowski1956), who noted the presence of a sandy tidal-flat system. Dechend (Reference Dechend1950) recognised a transgressional and a regressional trend within the Eemian succession, with the average boundary (i.e. transgressional/regressional) described at a depth of −12.5 m NHN (Normalhöhennull, German standard elevation zero).
Climatic cooling marked the onset of the Weichselian (MIS 5d-2, 115,000–11,653 ± 99 a BP, cf. Behre & Lade, Reference Behre and Lade1986; Serjup et al., Reference Serjup, Haflidason, Aarseth, King, Forsberg, Long and Rokoengen1994; Huijzer & Vandenberghe, Reference Huijzer and Vandenberghe1998; Litt et al., Reference Litt, Behre, Meyer, Stephan and Wansa2007; Walker et al., Reference Walker, Gibbard, Head, Berkelhammer, Björck, Cheng, Cwynar, Fisher, Gkinis, Long, Lowe, Newnham, Rasmussen and Weiss2019), a period characterised by at least four major ice advances in the Middle Weichselian (first advance = LGM) alone. These advances did not extend as far as the maximums of the previous Elsterian and Saalian glaciations, nor did they extend as far as the study area. In the region of the East Frisian Islands, the Weichselian glacial conditions resulted in the deposition of periglacial sands. These sands, which have been referred to in the literature as ‘brown sands’ (e.g. Sindowski, Reference Sindowski1973; Streif, Reference Streif1990), are mainly related to the deposition of continental sediments. However, according to Streif (Reference Streif1990), the precise stratigraphic age of these continental sediments within the Weichselian is problematic due to the predominance of periglacial structures, such as cryoturbation and ice wedges, disrupting the stratigraphy, as well as a distinct lack of interbedded interstadial sediments. Sindowski (Reference Sindowski1973) and Streif (Reference Streif1990) have subdivided the East Frisian Weichselian deposits into three facies, namely fluvial, flood-basin–freshwater and aeolian, based on their lithology. During the LGM the sea level dropped to 110–130 m below the present-day level (Streif, Reference Streif2004). As a consequence, the coastline was located almost 600 km further north (see also Hepp et al., Reference Hepp, Romero, Mörz, de Pol-Holz and Hebbeln2019). Subsequent climate warming resulted in a sea-level rise, causing the development of the Wadden Sea. This relative sea-level rise was essentially driven by the melting of the ice masses, glacio-isostatic adjustment, ongoing plate tectonics, sediment compaction and changing tidal influences (Streif & Köstner, Reference Streif and Köstner1978).
Norderney Island
Norderney is part of a barrier island system within the Wadden Sea. The island is separated from the neighbouring islands by high wave-energy mesotidal inlet systems, with a mean tidal range of 2.4 m (BSH, 2020). In the western tidal inlet, the Norderneyer Riffgat channel runs along the backside of the island, parallel to its southern coastline (maximum depth: −15 m NHN) (Sindowski, Reference Sindowski1973; Streif, Reference Streif1990). The Riffgat channel deeply erodes the Pleistocene succession which underlies Norderney and, thus, exposes the stratigraphic transition from the Pleistocene to the Holocene.
Pliocene-age deposits occur at a depth of approx. −35 m NHN below Norderney (Streif, Reference Streif1990). These are unconformably overlain by preserved Pleistocene deposits. To the north of this Pleistocene high, the Pleistocene relief was flattened and eroded during rising sea level, leading to the development of a ravinement (Streif, Reference Streif2004). According to Flemming & Davis (Reference Flemming and Davis1994), the elevated Pleistocene relief in the area of the modern barrier islands was partially eroded by rivers, which later were transformed into estuaries by rising sea levels around 7500 years BP. The Pleistocene high is cut by a pre-Weichselian (possible Eemian-age) palaeochannel(-system) (Norderney–Hilgenrieder Rinne, Sindowski, Reference Sindowski1973).
On top of Pliocene deposits, the Pleistocene/Holocene sedimentary succession in the western Norderney area commences with Saalian outwash deposits, overlain by 5 m of sand and gravel representing the Saalian moraine (Main Drenthe) (Rappol et al., Reference Rappol, Haldorsen, Jørgensen, van der Meer and Stoltenberg1989; Streif, Reference Streif1990). No Holsteinian deposits have been described or recorded, which led Sindowski (Reference Sindowski1973) and Streif (Reference Streif1990) to conclude that the Holsteinian transgression did not extend as far south as the region of the East Frisian Islands. For Norderney, Eemian deposits were first described by Keilhack & Wildvang (Reference Keilhack and Wildvang1925) noting the presence of a marine sand unit, 1–1.5 km west of the island’s lighthouse, unfortunately without any depth information. They based their interpretation on macro-fossils. Eemian deposits (a peat layer at a depth of −43 to −41.9 m NHN) were also described from the ‘Wasserwerk, alter Brunnen II’ core, which were probably deposited in the Norderney–Hilgenrieder channel (cf. Dechend, Reference Dechend1952, Reference Dechend1954; Sindowski, Reference Sindowski1973). Dechend (Reference Dechend1958) identified the top of the Eemian marine deposits (based on pollen analysis, micro- and macro-fossils) in the Riffgat channel at a depth of −10.2 to −13.4 m NHN. Subsequent descriptions of Eemian deposits from Norderney by Sindowski (Reference Sindowski1973) and Streif (Reference Streif1990), however, suggest that they are missing from the western part of the island. Based on this, Streif (Reference Streif1990, Reference Streif2004) concluded that there had been extensive erosion of the Eemian deposits in the Norderney region. Thus, the Saalian moraine deposits are disconformably overlain by Weichselian fluvial and aeolian sediments, at a depth of −8 m NHN (Sindowski, Reference Sindowski1973; Streif, Reference Streif1990). The Pleistocene succession is overlain by Holocene peats and tidal-flat sediments, which were subsequently covered by aeolian dunes (Sindowski, Reference Sindowski1973; Streif, Reference Streif1990).
Methods
Within the framework of the WASA project, hydroacoustic (sub-bottom) and sediment core data were collected using two ships, RV Senckenberg and RV Burchana, from both the offshore and the back-barrier sides of Norderney Island between 2016 and 2018. In the back-barrier area, where the present study is located, three hydroacoustic transects were gathered along the Riffgat channel (Fig. 1): one central transect (broadly following the maximum depth of the channel), to investigate the lowest part of the sedimentary succession; and two lateral transects running parallel to the central one, to document the Pleistocene/Holocene transition and the Holocene development of the tidal-inlet/tidal-flat system. Several coring transects crossing the Riffgat channel were also collected. The coring locations were initially planned at the cross-points of the hydroacoustic grid. Additional locations were added later, based on the initial results provided by the hydroacoustic data. In the present study, a subset of cores and sub-bottom hydroacoustic data was selected, focusing on the Middle and Late Pleistocene to Holocene succession.
Sub-bottom profiles
The parametric echo sounder used for this study operates with non-linear acoustics for investigating at high resolution (nominal vertical resolution <6 cm with a primary frequency around 100 kHz) the near-bottom stratigraphy (down to 50 m below the sea surface; Wunderlich & Müller, Reference Wunderlich and Müller2003). A SES 2000 Standard Plus parametric sub-bottom profiler (InnomarTechnologie GmbH) (average cruising speed 4.5 kn, differential GPS-based positioning) was deployed in the study area. The acoustic system allows several secondary frequencies (between 4 and 15 kHz) to be selected. Preliminary investigations close to Norderney Island indicated that maximum signal penetration occurred between 4 and 9 m, depending on the local stratigraphy. After a day-long test in the Riffgat channel, the 8 kHz secondary frequency signal was selected as being the one which would provide the best combination of penetration and resolution. Three profiles (transects 1, 2 and 3 in Fig. 1; total length 2.2 km) were processed and analysed. The ISE software (InnomarTechnologie GmbH) was used for tide correction (tidal gauge data were provided by the WSV – Wasserstraßen- und Schifffahrtsamt), speed correction (1600 m s−1), filtering (water column removal, noise filter, stacking, and for the tracking of the hydroacoustic reflectors in specific layers. Sediment information derived from core data was imported and plotted in the ISE software. All of the depths are referred to as NHN. In some of the older studies (e.g. Keilhack & Wildvang, Reference Keilhack and Wildvang1925; Dechend, Reference Dechend1958; Sindowski, Reference Sindowski1973; Streif, Reference Streif1990) the depth referred to NN (standard elevation zero until 2016): these depths were converted to NHN using an average of ±28 mm (LGLN, 2019).
The sub-bottom data were classified into acoustic facies based on specific properties, such as amplitude (i.e. low, moderate or high; based on the impedance contrast between beds), lateral continuity, lateral variability, depth, thickness, presence of internal structures, and the geometry of the contacts. The resulting acoustic facies were subsequently combined with the sedimentary facies information derived from the core data.
Sediment cores and facies characterisation
In total, 16 cores were collected from the study area. The coring locations were chosen based on the crossing points of the acoustic grid and on the preliminary results of the sub-bottom data. A modified VKG 6 vibrocorer (med consultant GmbH) was deployed from aboard RV Burchana. The vibrocorer was used in full-load mode (1.1 t) and was equipped with PVC-liners of 5000 mm length and 100 mm diameter. The cores were cut into 1.1 m long sections, sealed, labelled and moved to the laboratory for further processing. A SeaPath System (Kongsberg Maritime) with RTK corrections (SAPOS-Service) was used for positioning.
The core sections were opened in the laboratory and high-resolution digital images were taken under constant light conditions. The cores were described following a protocol designed for the specific purposes of the WASA project (see Capperucci et al., Reference Capperucci, Enters, Bartholomä, Bungenstock, Karle and Wehrmann2021). The macroscopic description included sediment characterisation (e.g. sediment grain size, colour, sorting), sedimentary structures (e.g. lamination, lenses, evidence of reworking, bioturbation), macrofauna characterisation, presence and nature of organic matter (e.g. roots, peat layers, peat peebles), and presence of erosional contacts. The sediments were tested with dilute hydrochloric acid (10%) for carbonates.
Each core was subdivided into intervals (depositional units), based on the compositional (e.g. sediment composition, presence of fossils, carbonate content), stratigraphic (with reference to the same unit described in other cores) and geometric (e.g. sediment structures, erosional contacts) differences with the units above and below. The interpretation of the Pleistocene–Holocene deposits in the area of Norderney was based on the 140 cores collected from both offshore and from the back-barrier side of the island during the WASA project. A proper catalogue of the depositional environments and related facies was designed for this purpose, based on the detailed evidence provided by the high-resolution examination of the cores (sedimentological, stratigraphic, etc.) as well as the previously described onshore stratigraphic succession of the island. A literature review of the local deposits, extending in age from the Middle Pleistocene through to present, allowed the various depositional units to be assigned to specific depositional environments and time stages. Additionally, a fine-gravel analysis of the coarse fraction from the Pleistocene-age moraine succession was carried out in order to determine its relative age (see below).
The LBEG (Geological Survey of Lower Saxony) core database was used to extend the spatial interpretation of the Pleistocene/Holocene succession. 14 LBEG cores (available at the NIBIS® WEBSERVER website) were selected and integrated into the present study. As part of this, the sediment descriptions of the LBEG cores were reviewed and, where necessary, reinterpreted according to the WASA catalogue of the depositional environments and related facies.
Grain-size analysis
Grain-size analysis was performed on sediment samples collected from specific core intervals, in order to aid the recognition of the various depositional environments, as well as helping to distinguish the different sedimentary facies. Since much of the sedimentary succession comprises sand and mud, the gravel fraction was not used for the grain-size determination, except for the glacial deposits, where the gravel component was treated separately and analysed in order to determine the stratigraphic age of the specific intervals (see below). The cores were sampled every 20 cm (in general), and a volume of approx. 3 cm3 was used for grain-size analysis. Additional samples were collected from intervals of particular interest (e.g. corresponding to transition intervals between sub-bottom units). Samples were treated with H2O2 (15%) in order to remove the organic matter and then decalcified with HCl (10%). The samples were subsequently dispersed with sodium pyrophosphate (Na4P2O7 × 10 H2O; 1%) and placed for 24 hours on a rotation wheel. A Beckman Coulter Laserparticlesizer LS 13 320 was used for the grain-size analysis.
The results from the particle analyser were transferred into GRADISTAT (Blott & Pye, Reference Blott and Pye2001) to determine the grain-size distribution and the statistical values. Mean grain size was calculated using the method of Folk & Ward (Reference Folk and Ward1957). Sediments were then divided into grain-size classes (from clay to coarse sand) (Table 1).
Table 1. Mean grain-size distribution of facies F1–12 (S = sand, U = silt, C = clay; v = very, c = coarse, m = medium, f = fine)

Fine gravel analysis
In order to assess the stratigraphic age of the moraine, a fine gravel analysis according to TGL 25 232 (1971, 1980) was carried out. This analytic method is mainly used in Eastern Germany and Poland (cf. Bussemer, Reference Bussemer2002; Börner et al., Reference Börner, Hrynowiecka, Kuznetsov, Stachowicz-Rybka, Maksimov, Grigoriev and Niska2015; Obst et al., Reference Obst, Nachtweide and Müller2017). According to Erd (Reference Erd1994) and Nowel (Reference Nowel2003), however, this method can be used more generally. For example, Ehlers (Reference Ehlers1978) successfully used a similar analysis (cf. Zandstra, Reference Zandstra1976, Reference Zandstra1978) on successions from Germany, the Netherlands and Denmark, suggesting that it could be used to determine the stratigraphic age of tills.
The fine-gravel analysis consists in sampling particular grain-size fractions, which are believed to represent specific bed load fractions. For this purpose, approx. 5 kg of material (corresponding to a core length of approx. 70 cm) was taken from selected cores. The samples were first dried at room temperature and weighed. Subsequently, the material was put into 30°C warm water to loosen and disaggregate the sediment. The fractions corresponding to 4–10 mm and >10 mm were then selected by wet-sieving for further investigations. The 4–10 mm fraction was used to carry out a stratigraphic analysis by separating at least 200 granules per sample into specific groups (NK = Nordic crystalline rocks; PK = Palaeozoic limestone; PS = Palaeozoic shale; D = dolomite; F = flint; MK = Mesozoic limestone; S = sandstone; Q = quartz; SO = other), where the ratio of PK, PS*10 and Ffr*10 (= unweathered flint) is used to determine the stratigraphical age (Fig. 2). The clasts larger than 10 mm (‘indicator stones’ in Smed & Ehlers, Reference Smed and Ehlers2002) were used to determine the origin, and therefore to confirm the stratigraphic age, of the till. In order to be able to achieve a statistically reliable conclusion, three WASA cores in addition to core N70 were also sampled and analysed (i.e. cores N20, N22, and Nney_02).

Fig. 2. (A) Results of the fine-gravel analysis after TGL 25 232 (1971, 1980), presented as a ternary diagram, and the crinoid Isocrinida. sp. (B) Percentages of gravel classes, after TGL 25 232 (1971, 1980). (C) Stratigraphy of Lower Saxony, modified from Streif (Reference Streif2004).
GIS and graphic software
QGis v.2.14.1 was used to plot, visualise/analyse the data (e.g. existing data sets, sub-bottom transects, core locations), and to produce the maps. The EasyCore software (The EasyCopy Company) was used to sketch the cores, and the graphic correlation of the cores was done by means of the EasyCopy software (The EasyCopy Company). The GIMP v2.8.22 and CorelDRAW Graphic Suite 2020 software were used to draw the figures.
Results
Transects
Based on the compiled lithological and hydroacoustic data (Table 2), the three high-resolution transects allowed the determined stratigraphic scheme for the Pleistocene/Holocene succession (cf. Sindowski, Reference Sindowski1973; Streif, Reference Streif1990) to be extended along the western part of the East Frisian barrier island system.
Table 2. Facies description and stratigraphic interpretation of hydroacoustic and core data, mean grain size (S = Sand, U = silt, C = clay; v = very, c = coarse, m = medium, f = fine) after Folk & Ward (Reference Folk and Ward1957). Ages from radiocarbon dating. Ages of peat were provided by Schlütz et al. (Reference Schlütz, Enters and Bittmann2021)

Hydroacoustic and lithological facies
The detailed analysis of the hydroacoustic transects and the close examination of the sediment cores allowed 12 different facies types (F1–12) to be identified (Table 2). The facies types include deposits related to glacial and periglacial processes (i.e. moraine and outwash deposits), continental environments (i.e. lacustrine and fluvial settings), as well as marine and transitional environments (i.e. from brackish-lagoonal to intertidal and subtidal deposits). None of the Pleistocene sediments reacted with the hydrochloric acid (carbonate content = 0).
F1
Concave and oblique (divergent to planar) reflectors characterise some of the stratigraphically oldest facies (transects 2 and 3) in the acoustic data. The high-amplitude acoustic reflector at a depth of between −18 and −19 m NHN has a lateral extension of nearly 500 m on transect 3. Two cores (N24b, transect 2; N72, transect 3) correspond to this acoustic reflector. The pale brown fine sandy sediments (C: 5.5%; U: 30.8%; vfS: 47.5%; fS: 13.6% in bulk sample) contain highly organic-rich fine-grained layers (vfS with mud), the occurrence of which decreases towards the top of the depositional unit, and suggests that deposition occurred in a low-energy environment. The base of this facies is characterised by coarser sandy sediments (C: 1.4%; U: 5.9%; vfS: 25.2%; fS: 48.5%; mS: 18.2%; cS: 0.7% in bulk sample).
F2
Oblique planar reflectors characterise this facies, which suggest the possible presence of cross-stratification with transport towards the SE. A high-amplitude reflector at a depth between −17 and −18 m NHN can be laterally traced along transects 2 and 3 for approx. 350 m and 500 m, respectively. F2 was found in three cores (N74, transect 1; N24b, transect 2; N72, transect 3) and comprises pale to medium brown sands, without any internal structures (although some grading was noted). The larger amount of relatively medium to coarse-grained material within the samples (C: 2.5%; U: 7.5%; vfS: 30.3%; fS: 43.6%; mS: 12.6%; cS: 3.5% in bulk sample) and the fining-upward nature would suggest that sedimentation occurred in a moderate- to high-energy depositional system, with a decrease in energy levels towards the top of the unit. Single clasts (flint stone and quartz, average size 2.5–3.5 cm) were also found in the facies.
F3
This particular facies is delimited by two of the most prominent reflectors observed in the acoustic data set and was mapped on all three transects, with a lateral extent of more than 750 m (transect 1), 350 m (transect 2) and 850 m (transect 3). F3 was found in five different cores (N74, transect 1; N24b, transect 2; N70, N72 and T20, transect 3). The sediment composition includes a variety of sediments (from light grey to light green in colour), ranging from clays to cobble-grade gravels, without any evident sedimentary structure. Thus, the sediment is depicted as a diamicton. The matrix is made by sands and muds (C: 7.8%; U: 20%; vfS: 12%; fS: 31.6%; mS: 21.6%; cS: 7% in bulk sample). The fine-gravel analysis of core N70 was based on 282 granules (NK: 42.6%; PK: 28%; PS: 1.1%; D: 1.4%; F: 11.4%; MK: 3.2%; S: 6.7%; Q: 4.3%; SO: 1.4%) (Fig. 2). A rare Mesozoic-age crinoid Isocrinida sp. (Fig. 2) was found in the F3 facies of N20.
F4
Oblique planar acoustic reflectors characterise this facies. A high-amplitude reflector is recognised at a depth between −11 and −13 m NHN, and this can be laterally traced along transects 1, 2 and 3 for approx. 350 m, 150 m and 60 m, respectively. Two cores, both located within the Riffgat channel (N26, N74, transect 1), extended as far as this reflector. The sediments are characterised by planar-bedded, mica-bearing very fine-grained sands (C: 2.6%; U: 11.3%; vfS: 47.2%; fS: 38.7%; mS: 0.2% in bulk sample). Mud couplets are also present, suggesting a low-energy depositional environment. The sediments are enriched in organic content. Marine diatoms were found in core N26. The NE end of transect 1 lacks any recognisable acoustic reflectors. However, core N26 documents the presence of this facies (Fig. 3).

Fig. 3. Transect 1 sub-bottom profile (top) crossing the Riffgat channel and its interpretation (bottom). The Pleistocene sequence starts with the Saalian moraine (F3). Eemian tidal flat deposits (F4 and F5) are well shown in this transect. Core N26 confirms the presence of a thick Eemian succession for the NE end of transect 1, though no reflector was detected. Depth refers to NHN. For the description of the facies, see Table 2.
F5
This acoustic signal is quite similar to F4 and F10 and shows oblique planar geometries. On transects 1, 2 and 3 a high-amplitude reflector at a depth of between −10 and −13 m NHN can be traced laterally for approx. 1000 m, 120 m and 60 m, respectively. Four cores, located within the Riffgat channel (N26 and N74, transect 1; N18 and N71, transect 3) extend as far as the high-amplitude reflector. The sediments are characterised by planar- to cross-bedded mica-bearing fine-grained sands (average composition: C: 1.5%; U: 3.6%; vfS: 33%; fS: 56.1%; mS: 5.5%; cS: 0.2% in bulk sample), which suggests a low- to medium-energy depositional environment. The bedding, which mainly occurs in the lower part of the sequence, shows an alternation of sand layers (e.g. sample N18-1_89–90 cm, C: 1.9%; U: 6.4%; vfS: 54.3%; fS: 37.4%; mS: 0%; cS: 0% in bulk sample) with intercalated mud to sandy mud layers (sample N18-1_90–91 cm, C: 11.6%; U: 46.2%; vfS: 33%; fS: 29.9%; mS: 11%; cS: 0.5% in bulk sample). F5 can be distinguished from F4 due to its bedding type, its slightly higher content of fine- and medium-grained sands, and the negligible organic content.
F6
The main characteristic of this facies is the planar to concave, broadly continuous reflectors. The acoustic record shows a high-amplitude reflector at a depth of between −8.5 and −12 m NHN, which can be laterally traced along transects 1, 2 and 3 for approx. 125 m, 120 m and 50 m, respectively. Concave reflectors possibly represent channel structures. The facies is present in two cores (N18; N71, transect 3). Planar interbedded deposits of fine-grained sandy sediment (C: 2.5%; U: 14.5%; vfS: 50.7%; fS: 30.9%; mS: 1.2%; cS: 0.2% on bulk sample) suggest a moderate- to high-energy depositional environment. The sediments are cryoturbated and include evidence of root structures.
F7
This facies has been mainly correlated between the three transects on the basis of its stratigraphic position. On both transects 1 and 2, the facies lacks any clear reflectors, while on transect 3 concave reflectors are recognised. F7 is present in cores N18 and N71 of transect 3. The highly organic-enriched fine-grained sands (C: 1.5%; U: 3.5%; vfS: 32.9%; fS: 54.8%; mS: 6.5%; cS: 0.9% in bulk sample) decrease in grain size toward the top of the unit (fining upward) and are mostly structureless, indicating a depositional system with varying or decreasing energy levels. The facies includes highly decomposed plant fragments and also shows evidence of root penetration. The amount of organic content increases towards the top, as shown by the change in colour, which ranges from golden brown (basal part of F7) grading to dark brown (humic fine sand, top of the unit). The top of the unit is usually in direct contact with F8.
F8
The facies is marked by a highly recognisable high-amplitude reflector in all three transects at a depth of −6 m and −7 m NHN in transects 1 and 2, respectively, and at a depth of −9 and −10 m in transect 3. It can be traced laterally along transects 1, 2 and 3 for approx. 125 m, 120 m and 20 m, respectively, and is often associated with the intercalated deposits of F9. Two cores (N18 and N71) showed the presence of this facies in transect 3. The deposits comprise mainly peat, with a varying amount of sand (S: 5.6–83.7% in bulk sample) and the presence of reed rhizomes.
F9
Continuously parallel-planar reflectors characterise this facies along all three transects. The facies is intercalated with the peats of F8 and can therefore easily be identified at depths of −6 m and −7 m NHN in transects 1 and 2, and at a depth of −9 and −10 m in transect 3. It can be laterally traced along transects 1, 2 and 3 for approx. 125 m, 120 m and 20 m, respectively. Cores N18 and N71 (transect 3) contain this facies, which is characterised by organic-enriched laminated muddy sediments (C: 9.3%; U: 44%; vfS: 22.1%; fS: 21.7%; mS: 2.3%; cS: 0.3% in bulk sample), suggesting a low-energy depositional environment.
F10
This facies is similar to F4 and F5 and is characterised by oblique planar reflectors. These are noted at the NE end of transect 1. The facies is associated with the deposits of F11 and F12 and can therefore easily be localised at depths of between −11 and −13 m NHN with a lateral extension of almost 350 m. F10 is only present in core N26. The sediments are characterised by cross- to planar-bedded, fine-grained sands (C: 1.6%; U: 4%; vfS: 29.3%; fS: 63.8%; mS: 1.3%; cS: 0% in bulk sample), which indicate a low- to medium-energy depositional environment. The facies is distinguished from F4 and F5 by the presence of crumbled peats and the lack of mica. A specimen of the cockle Cerastoderma edule was found in life position and was dated at 2260 ± 30 yrs BP, using the radiocarbon method.
F11
The facies comprises a high-amplitude reflector in transect 1 at a depth of −12 to −13 m NHN, which can be laterally traced along the transect for approx. 150 m. The facies is present in four cores (N26, transect 1; N70, N72, T20, transect 3). The transition to F11 is usually marked by the presence of a chaotic coarse-grained layer, with reworked shells and shell fragments in a matrix of sand to gravel (fine fraction: C: 2.8%; U: 8.1%; vfS: 6.7%; fS: 40%; mS: 29%; cS: 13.5% in bulk sample).
F12
Broadly continuous reflectors, which are both planar and concave, are characteristic of this facies (e.g. transects 1 and 3). The depth of the high-amplitude reflector varies from −7 to −15 m NHN, and it can be laterally traced along transects 1 and 3 for approx. 350 m and 600 m, respectively. The base of the facies comprises high-amplitude reflectors, which may represent a lag deposit (i.e. AF11). Three cores (N26, transect 1; N70; T20, transect 3) were collected, which contain this facies. The sediments are characterised by massive to cross- to planar-bedded, fine-grained sands (C: 3.6%; U: 11.5%; vfS: 12.3%; fS: 47.7%; mS: 22.8%; cS: 1.9% in bulk sample) as well as shells and some small cobbles, indicating deposition in a moderate- to high-energy depositional environment.
Discussion
The compilation of new data of high-resolution sub-bottom hydroacoustics and vibrocores (up to 5 m length) collected as part of this study has allowed a better understanding of the stratigraphy of such key area for the reconstruction of the Middle Pleistocene to early Holocene history of the region. This builds on work carried out by a number of studies which commenced in the 1950s (e.g. Dechend, Reference Dechend1950, Reference Dechend1952, Reference Dechend1954, Reference Dechend1958) and is still ongoing (e.g. Streif, Reference Streif1990, Reference Streif1998, Reference Streif2004; Hoselmann & Streif, Reference Hoselmann and Streif2004). The new and extremely detailed data set also demonstrates the complexity of the Pleistocene palaeorelief and the lateral heterogeneity of the Holocene deposits for the western part of the East Frisian Wadden Sea.
Facies interpretation
A total of 12 different facies have been identified from the three sub-bottom transects and a range of core data (see above for details). In contrast to the historical published data (e.g. Sindowski, Reference Sindowski1973; Streif, Reference Streif1990), the use of hydroacoustic data allows to extend the knowledge about the presence of the Middle Pleistocene- and Eemian units across a much broader area. In this subsection the facies types are discussed in detail.
F1 – lacustrine-deltaic deposits
This facies represents the oldest deposits found in the cores. The stratigraphic position of these sediments (underneath the Saalian moraine, see F3), together with their sedimentological characteristics, differs from those of similar deposits described in the literature for the same area (e.g. Sindowski, Reference Sindowski1973) and would suggest that they represent Middle Pleistocene (most likely Holsteinian or Dömnitz temperate stage) lacustrine-deltaic deposits. In F1, the presence of both coarse- and fine-grained sediments suggests a variety of depositional settings, coexisting on a small scale during the transition between the temperate stage (either Holstein or Dömnitz temperate stage) and the subsequent Saalian glacial phase. The coarser-grained sediments may therefore represent either a distributary channel infill or a sandy mouthbar deposit, while the fine-grained deposits may represent deposition within an interdistributary bay, which developed as a swampy delta plain under subaerial conditions (cf. Lang et al., Reference Lang, Winsemann, Steinmetz, Polom, Pollok, Böhner, Serangeli, Brandes, Hampel and Winghart2012, Reference Lang, Böhner, Polom, Serangeli and Winsemann2015; Hepp et al. Reference Hepp, Romero, Mörz, de Pol-Holz and Hebbeln2019). Lacustrine deltas within Elsterian tunnel valleys have been recorded by a number of authors from Northern Germany (Ehlers & Linke, Reference Ehlers and Linke1989; Streif, Reference Streif1990; Lutz et al., Reference Lutz, Kalka, Gaedicke, Reinhardt and Winsemann2009; Stackebrandt, Reference Stackebrandt2009).
F2 – outwash deposits (sandur plain)
The facies is considered to represent Saalian-age proglacial outwash deposits (cf. Ehlers, Reference Ehlers1990; Eissmann, Reference Eissmann2002; Hambrey, Reference Hambrey2007). The sediments are broadly fining upwards and show cross-stratification with transport towards the SE. Thus, the sediments are interpreted as channel deposits. Furthermore, the observed clasts (e.g. flints) within the sediments are very similar to the fine-grained gravels of the overlying till deposits of F3, indicating a coherent evolution (i.e. a sandur plain located at the ice margin). In the Saalian period, three glaciations occurred, although only the older Main Drenthe advance (Older Saalian Glaciation) affected the area of Norderney (Ehlers, Reference Ehlers1990). During this phase, a glacio-fluvial system developed (Streif Reference Streif1990) and the outwash sands have been described as containing flints and siliceous gravels (Eissmann, Reference Eissmann2002). It is therefore highly probable that the deposits of this unit were formed on the sandur plain of the Main Drenthe advance, particularly since the overlying moraine is also identified as the Drenthe Main Till.
F3 – moraine
The facies was interpreted as the Older Saalian Till (i.e. Drenthe Main Till), the deposits of which can be found extensively in Northern Germany (cf. Rappol et al., Reference Rappol, Haldorsen, Jørgensen, van der Meer and Stoltenberg1989; Eissmann, Reference Eissmann2002; Meyer, Reference Meyer2005; Lambeck et al., Reference Lambeck, Purcell, Funder, Kjær, Larsen and Möller2006). The F3 facies closely resembles the boulder clay described by Streif (Reference Streif1990) as greyish-green to brownish-grey sediments with an average thickness of 1–5 m (maximum thickness 10 m). The moraine in the core data shows a thickness between 1.4 and 3.2 m. The fine-gravel analysis conducted on the coarser fraction of the moraine confirmed the age as Saalian (Fig. 2). Based on the proven efficacy of the similar methodology of Zandstra (Reference Zandstra1978) for Northern Europe (see Ehlers, Reference Ehlers1978, Reference Ehlers1980 for Germany; Zandstra, Reference Zandstra1976 for Northern Netherlands; and Ehlers, Reference Ehlers1979 for Denmark), we would suggest that the TGL 25 232 (1971, 1980) is a suitable method to determine the stratigraphic age of tills in Lower Saxony. The >10 mm fraction consists of crystalline detritic material with an origin in central to eastern Sweden (cf. Smed & Ehlers, Reference Smed and Ehlers2002).
F4 – tidal flat deposits I
This facies was interpreted as representing Eemian-age mixed tidal-flat deposits, based on the presence of interbedded tidal bundles. This interpretation differs from the more generalised description of a sandy tidal flat of previous authors (e.g. Dechend, Reference Dechend1954; Sindowski, Reference Sindowski1973; Streif, Reference Streif1990). In tidal-flat systems, energy conditions are variable, with energy levels generally increasing towards the open sea. With such an increase in energy, the levels of organic matter and mud within the sediment decrease as a result of winnowing (cf. Fan, Reference Fan, Davis and Dalrymple2012; Flemming, Reference Flemming, Davis and Dalrymple2012). Based on the results of the grain-size analysis (i.e. low percentages of mS and a lack of cS) the sediments may represent a mixed-flat environment (cf. Flemming, Reference Flemming, Davis and Dalrymple2012). Within this facies, the sand/mud ratio (6:1) is very high. Thus, it can be concluded that the sediments were deposited in a more exposed environment, which could have been either an external part of a mixed flat or a transition zone from a mixed flat towards a sand flat. The sediments in F4 correspond to modern tidal-flat deposits observed in the same area, suggesting the presence of similar depositional conditions (cf. Sindowski, Reference Sindowski1973; Streif, Reference Streif1990; Caspers et al., Reference Caspers, Merkt, Müller and Freund2002; Shackleton et al., Reference Shackleton, Sánchez-Goñi, Pailler and Lancelot2003; Schokker et al., Reference Schokker, Cleveringa and Murray2004; Leduc et al., Reference Leduc, Schneider, Kim and Lohmann2010).
F5 – tidal-flat deposits II
The facies was interpreted as Eemian-age sand-flat deposits. F5 is quite similar to F4, though the sediments of F5 are slightly more coarse-grained. The mud content is >5% while the amount of organic matter is negligible. The sediments of F5 are considered to have been deposited under medium energy conditions, and represent either an external part of the Eemian sand-flat environment or a transition zone from a sand flat towards a mixed-flat environment (cf. Flemming, Reference Flemming, Davis and Dalrymple2012).
F6 – fluvial deposits
This facies is interpreted as Weichselian-age fluvial deposits. Internal concave reflectors are interpreted as channel structures. Additionally, the sediments show evidence of normal grading, which is also typical for channel deposits. This facies is associated with the deposits of F7, which are interpreted as crevasse splay deposits. These combined characteristics led to the interpretation that these deposits represent a meandering river system. The presence of cryoturbation features provides evidence that the sediments were deposited under permafrost conditions. The channel (or channels?) was oriented EW based on the orientation of the concave reflectors in all three transects. During the Weichselian, although the area of Norderney was not covered by ice, glacial climatic conditions were active (Boulton, Reference Boulton2001; Knies et al., Reference Knies, Kleiber, Matthiessen, Müller and Nowaczyk2001). Thus, periglacial processes were common (Huijzer & Vandenberghe, Reference Huijzer and Vandenberghe1998). Meandering river systems with crevasse splays (F7) developed in Northern Germany as noted by previous authors (van Huissteden et al., Reference van Huissteden, van der Valk and Vandenberghe1986; van Huissteden, Reference van Huissteden1990; Vandenberghe & Woo, Reference Vandenberghe and Woo2002). The deposits of these rivers have been described by Streif (Reference Streif1990) as fine sands and silts, which are brownish-grey and are interbedded with medium-grained sands, gravels and plant fragments. In addition, periglacial features such as ice wedges or solifluction are quite common (Streif, Reference Streif1990), both of which were also recognised as part of this study.
F7 – crevasse splay deposits (with palaeosol)
The facies was interpreted as Weichselian-age continental deposits (i.e. semi-terrestrial, as a result of the transition from the Eemian shallow-marine environments to the Weichselian proglacial conditions). Van Huissteden (Reference van Huissteden1990) described similar Weichselian-age sediments from the Northern Netherlands, and interpreted them as crevasse splay deposits. His interpretation is consistent with the sedimentological characteristics observed in F7 (sandy sediments, fining upward) and with the interpretation given for F6 (fluvial deposits). Several authors (e.g. Dury, Reference Dury1964; van Huissteden et al., Reference van Huissteden, van der Valk and Vandenberghe1986; van Huissteden, Reference van Huissteden1990; Vandenberghe & Woo, Reference Vandenberghe and Woo2002) showed that crevasse splays develop within a fluvial system, independent of the climatic conditions. The topmost part of this facies is rich in organic matter (humus, detritus, roots). The organic enrichment was probably a result of post-diagenetic pedogenic processes, which occurred during the final stage of the Weichselian/early Holocene phase. According to Kraus & Aslan (Reference Kraus and Aslan1993) and Collinson (Reference Collinson and Reading2009) the top of F7 can be described as simple alluvial palaeosols (weak development of horizons, A-horizon very rare, colours from grey to yellow-brown, diffuse presence of roots). It has been noted that such palaeosols may develop in crevasse splays (Collinson, Reference Collinson and Reading2009; Gulliford et al., Reference Gulliford, Flint and Hodgson2017).
F8 – peat
This facies was interpreted as Holocene peats. The Holocene succession of the Wadden Sea commences with the formation of a basal peat, which developed due to the rising groundwater table, and which was associated with an increase in swampy conditions and the development of mires (cf. Streif, Reference Streif1990, Reference Streif2004). Based on the presence of reed rhizomes within F8 sediments, it was possible to identify the peat of core N71 as a fen peat. These peat deposits are partly intercalated with fine- to very fine-grained sands and muds. The clastic material may have been deposited as a result of aeolian sediment transport (loess or wind-blown sand). The basal peats in cores N18 (7630 yrs BP), N71 (7780 yrs BP) and N23 (6850 yrs BP) were dated by Schlütz et al. (Reference Schlütz, Enters and Bittmann2021).
F9 – brackish-lagoonal deposits
Brackish-lagoonal or lacustrine sediments are deposited under similar conditions and are not easily distinguishable (Nichols, Reference Nichols2012). However, analysis of diatoms from this facies implies a brackish environment, and thus it can be suggested that this facies represents a Holocene brackish-lagoonal depositional system. Such settings were quite common in the North Sea area during the early Holocene, when fens and bogs started to develop (Streif, Reference Streif1990, Reference Streif2004).
F10 – tidal flat deposits III
The facies was interpreted as Holocene sand-flat deposits. As noted above, the marine bivalve Cerastoderma edule (dated at 2260 ± 30 yrs BP), also known as the common cockle, was found in life position in core N26. This bivalve is typically found in shallow-marine environments. According to Fan (Reference Fan, Davis and Dalrymple2012), the presence of mud couplets is one of the main criteria for recognising tidal-flat environments. A second criterion, according to Fan (Reference Fan, Davis and Dalrymple2012), is the presence of cross-bedding structures. F10 comprises mud couplets and shows evidence of weak cross-bedding, which changes to parallel bedding towards the top of the facies. The change in bedding type can result from variations within the energy levels. In a sand-flat environment only <5% of mud is generally present (Flemming, Reference Flemming, Davis and Dalrymple2012). This corresponds to the sediment characteristics of this facies.
F11 – channel lag deposits
This facies was interpreted as a channel lag deposit comprising coarse-grained sediments, mainly sands and gravels, as well as chaotic deposits. Such deposits are typically present at the base of subtidal channels within the tidal-flat environment (e.g. Fan, Reference Fan, Davis and Dalrymple2012; Flemming, Reference Flemming, Davis and Dalrymple2012). Streif (Reference Streif1990) noted the presence of coarse-grained sands and gravels at this stratigraphic level. He also noted that the unit contained shell fragments. Such lags are typical of tidal-flat successions (cf. Flemming et al. Reference Flemming, Schubert, Hertweck and Müller1992; Scasso et al. Reference Scasso, Dozo, Cuitiño and Bouza2012).
F12 – channel fill deposits
Channel fill sediments overlie the channel lag deposits (F11) and, together, form a typical tidal channel sequence (cf. Flemming, Reference Flemming, Davis and Dalrymple2012). The extensive presence of cross-bedding and planar bedding structures in the cores, together with the grain-size characteristics of these sediments (fine-grained sandy sediments with mud layers, occasionally interbedded with coarse-grained sediments and shell/gravel lags), suggests that deposition occurred in an environment characterised by alternating high- and low-energy conditions, which is consistent with a channel fill system (cf. Fan, Reference Fan, Davis and Dalrymple2012; Flemming, Reference Flemming, Davis and Dalrymple2012).
Stratigraphic succession: from Middle Pleistocene to early Holocene
Based on the analysis of the 12 sedimentary facies and on their correlation with the hydroacoustic profiles, a new and much more detailed reconstruction of the stratigraphy of the back-barrier area of Norderney from the Middle Pleistocene through to the early Holocene has been performed.
The deep erosion of the main tidal Riffgat channel into the Holocene and Pleistocene sediments has allowed the entire succession extending from pre-Saalian (possibly Holsteinian) lacustrine deposits through to Saalian periglacial and moraine sediments to be examined (Figs 3–5). Along the channel margins, the depositional transition from interglacial Eemian sediments to periglacial and fluvial Weichselian sediments was followed by the deposition of a transgressive sequence corresponding to the first stages of the early Holocene sea-level rise (Figs 3–5). The stratigraphic succession from the northern side of the Riffgat channel is complete (i.e. from F1 to F12), extending from the Middle Pleistocene up to the early Holocene (transect 3, Fig. 5). The successions from the southern side of the Riffgat channel (i.e. transects 1 and 2, Figs 3 and 4) are less complete, but very similar.
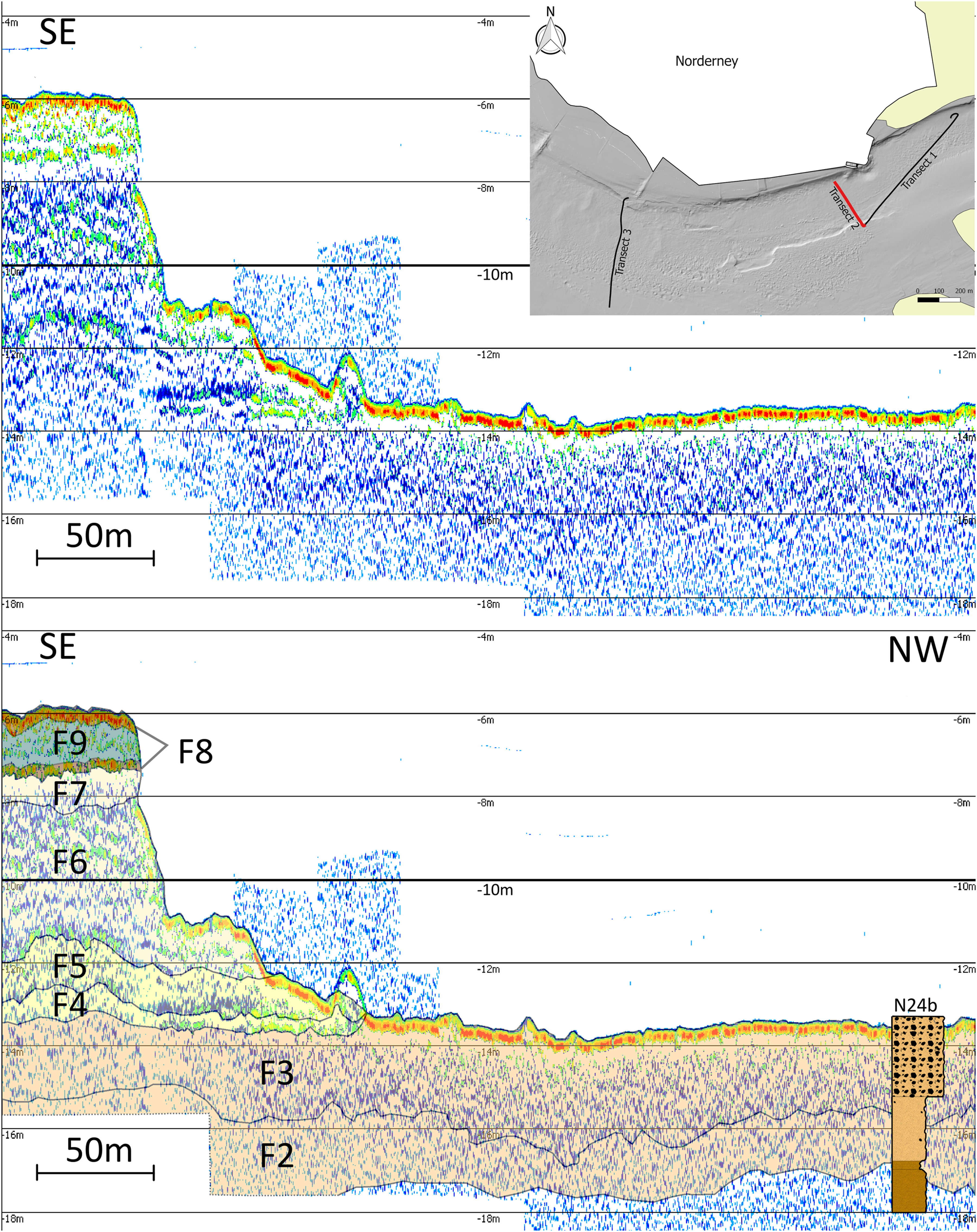
Fig. 4. Transect 2 sub-bottom profile (top) perpendicular to the Riffgat channel, and its interpretation (bottom). The Saalian outwash deposits (F2) are followed by the Saalian moraine (F3), which works as a limiting erosional element for the evolution of the Riffgat channel. Core N24b confirms the presence of Middle Pleistocene sediments (F1) below the Saalian deposits, though no related reflector was detected. Depth refers to NHN. For the description of the facies, see Table 2. For legend see Figure 3.

Fig. 5. Transect 3 sub-bottom profile (top) perpendicular to the Riffgat channel. Middle Pleistocene deposits (F1) commence the Pleistocene sequence. The Saalian moraine (F3) builds up parts the present sea floor, which works as a limiting erosional element for the evolution of the Riffgat channel. It is covered by a thick succession of Holocene subtidal channel fill deposits (F12) in the southern part of transect 3. Depth refers to NHN. For the description of the facies, see Table 2. For legend see Figure 3.
The reconstructed stratigraphic succession commences with lacustrine-deltaic sediments (F1) at a depth of −18 m NHN (transect 3 and lowest section of core N72), and –17 m NHN (transect 2 and core N24b), interpreted as Middle Pleistocene deposits. F1 is capped by a semi-horizontal erosional contact and overlain by around 2 m of Saalian outwash deposits (F2). The top and bottom boundaries of F2 are clearly visible in the hydroacoustic data in transect 3, while on the other transects the presence of this facies is mainly deduced from the core sediment analysis (core N24b, transect 2 and core N74, transect 1). The Saalian moraine deposits (i.e. Drenthe Main Till, F3) are present in all three transects. Indeed, much of the sea floor in the main channel is covered by this coarse-grained material (i.e. medium- to coarse-grained sand and gravels). The acoustic reflector of the Saalian moraine is one of the most prominent acoustic elements, and can be identified by its clear upper and lower boundary reflectors. The Saalian moraine is overlain by 2 m of Eemian mixed-flat (F4) and sand-flat deposits (F5). The Eemian deposits are overlain by 2 m (transect 3) to 5 m (transect 2) of Weichselian fluvial (F6) and crevasse splay (F7) sediments on the margins of the Riffgat channel. A basal peat (F8) overlies the Weichselian deposits at a depth of −7.5 m (transect 1) to −10 m (transect 3) NHN, followed by brackish-lagoonal deposits (F9) and a second peat layer (F8).
In order to trace the lateral extent of our new detailed reconstruction of the stratigraphy of the western head of the island of Norderney, core data from the LBEG were included (Fig. 6). In contrast to the interpretation of Sindowski (Reference Sindowski1973), the Middle Pleistocene lacustrine facies and an Eemian shallow-marine facies are now also documented beneath the western part of Norderney. This is a significant finding, since it suggests that the profile of Sindowski (Reference Sindowski1973) is erroneous, at least in terms of describing the stratigraphy in the western part of Norderney. In addition, our high-resolution analysis of sedimentological data, such as tidal bedding and bundles, and the occurrence of marine diatoms, proves the presence of Eemian-age sediments at a depth of −10.6 m (N18) to −12,3 m NHN (N26). These new results confirm the conclusions of Dechend (Reference Dechend1950, Reference Dechend1952, Reference Dechend1954, Reference Dechend1958) while disproving the interpretation of Sindowski (Reference Sindowski1973) and Streif (Reference Streif1990) who had suggested that Eemian deposits were completely eroded in the western area of Norderney.

Fig. 6. Cross-section of the Riffgat channel (A–A′, B–B′) and the western head of Norderney (C–C′), derived from both WASA- and reinterpreted LBEG cores. For orientation of cross-sections see Figure 1.
Middle Pleistocene deposits and the presence of tunnel valleys below Norderney
A unit of Middle Pleistocene sediments (F1) was found at a depth of −17.9 m (core N24b) and −16.8 m NHN (core N72). In addition, these deposits were present in transect 3 (Fig. 5). This facies is interpreted as lacustrine-deltaic deposits, where coarser-grained sediments represent either a distributary channel infill or a sandy mouthbar deposit, while the fine-grained deposits may result from deposition within an interdistributary bay (i.e. swampy delta-plain; subaerial conditions). Based on their potential stratigraphic position (see below), these sediments are considered to be indicators for the presence of tunnel valleys, since they have been used by various authors (e.g. Ehlers & Linke, Reference Ehlers and Linke1989; Streif, Reference Streif1990; Lutz et al., Reference Lutz, Kalka, Gaedicke, Reinhardt and Winsemann2009; Stackebrandt, Reference Stackebrandt2009; Kehew et al., Reference Kehew, Piotrowski and Jørgensen2012) to mark the uppermost unit of the infill of Elsterian tunnel valleys in Northern Germany. These tunnel valleys developed subglacially, i.e. beneath ice masses (Kehew et al., Reference Kehew, Piotrowski and Jørgensen2012).
The Elsterian tunnel valleys of Northern Germany have been mapped by Stackebrandt (Reference Stackebrandt2009) who noted that their NS orientation is influenced by the so-called NW German ice stream, i.e. the broad orientation of ice movement during the Pleistocene (cf. Stackebrandt et al., Reference Stackebrandt, Ludwig and Ostaficzuk2001). Stackebrandt’s (Reference Stackebrandt2009) map indicates the presence of Elsterian tunnel valleys to the south of Norderney, the closest approx. 30 km to the south. However, the map does not indicate the presence of any tunnel valley in the Wadden Sea area. An alternative map of the Elsterian tunnel valleys in the southern North Sea has been published by Lutz et al. (Reference Lutz, Kalka, Gaedicke, Reinhardt and Winsemann2009). This map is more detailed with regard to the German North Sea sector than that of Stackebrandt (Reference Stackebrandt2009). It also indicates the presence of four to five tunnel valleys located approx. 5 km north of Norderney. Both of these results are in agreement with the large number of Pleistocene tunnel valleys recorded by Huuse (Reference Huuse2000) in his comprehensive study of the tunnel valleys of Northern Europe. Thus, it can be reasonably assumed that the valleys located offshore were originally part of a broader system of subglacial valleys which extended onshore, connecting with the ones on the mainland. The broad NS orientation which has been noted from the onshore subglacial tunnel valleys would thus have continued into the offshore areas.
Subsequent to the retreat of the Elsterian ice masses, tunnel valleys functioned as locations of sediment preservation during phases of glacial and interglacial climate conditions throughout the Middle Pleistocene. According to Lutz et al. (Reference Lutz, Kalka, Gaedicke, Reinhardt and Winsemann2009), the sedimentary infill of the tunnel valleys is dependent on their size, with smaller valleys containing mainly homogeneous infill, while the infill within the larger valleys is mainly heterogeneous, documenting multiple depositional and erosional events. The uppermost facies present within the tunnel valley successions is interpreted as comprising stacked lacustrine delta deposits (e.g. Lang et al., Reference Lang, Winsemann, Steinmetz, Polom, Pollok, Böhner, Serangeli, Brandes, Hampel and Winghart2012, Reference Lang, Böhner, Polom, Serangeli and Winsemann2015), which is supported by our interpretation of F1. The deposits of F1 may be stratigraphically correlated with either the Holsteinian (a) or the Dömnitz temperate stage (b), which are described in the following:
-
a) Holsteinian lacustrine facies. The Holsteinian transgression did not extend as far as the area of Norderney (Knudsen, Reference Knudsen1988; Streif, Reference Streif1990, Reference Streif2004). Coeval deposits located to the south of Norderney have been described by Litt et al. (Reference Litt, Behre, Meyer, Stephan and Wansa2007) as fluvial and lacustrine. The sediments are described as mainly silt and clay, and may contain diatoms (Unger et al., Reference Unger, Hübner and Escher1995). Both Unger et al. (Reference Unger, Hübner and Escher1995) and Litt et al. (Reference Litt, Behre, Meyer, Stephan and Wansa2007) have also suggested that the deposition of the Holsteinian lacustrine facies occurred across the broader East Frisian area and was preserved in the older Elsterian tunnel valleys. Hepp et al. (Reference Hepp, Hebbeln, Kreiter, Keil, Bathmann, Ehlers and Mörz2012) recently described very similar deposits, also of Holsteinian age, preserved within an Elsterian tunnel valley in the southern North Sea.
-
b) Dömnitz temperate stage (also Wacken/Schöningen temperate stage). A succession of lacustrine deposits present across Northern Germany have been interpreted as representing the Dömnitz temperate stage (e.g. Litt et al., Reference Litt, Behre, Meyer, Stephan and Wansa2007; Urban, Reference Urban2007; Urban et al., Reference Urban, Sierralta and Frechen2011; Stephan et al., Reference Stephan, Urban, Lüttig, Menke and Sierralta2012). Urban (Reference Urban2007) describes a unit of mud and peat, which developed under swampy conditions at the location of the Elsterian-age Schöningen tunnel valley (Lower Saxony, Germany). Lang et al. (Reference Lang, Winsemann, Steinmetz, Polom, Pollok, Böhner, Serangeli, Brandes, Hampel and Winghart2012, Reference Lang, Böhner, Polom, Serangeli and Winsemann2015) examined this succession and reinterpreted the sediments as shallow lacustrine delta deposits, based on extensive investigation of seismic and core data. Urban et al. (Reference Urban, Sierralta and Frechen2011) and Stephan et al. (Reference Stephan, Urban, Lüttig, Menke and Sierralta2012) described a similar sedimentary succession (which is also similar to that described in this study) from the area of Leck (North Frisian area).
Based on the overall similarity of the sediments (vfS), the facies architecture (i.e. a stacked lacustrine delta) and the depth (−17.9 to −16.8 m NHN) of the deposits, it is concluded that F1 correlates more likely to the Dömnitz temperate stage.
In conclusion, it cannot be proven with any certainty that tunnel valleys exist below the island of Norderney, based on the data set from this study. However, Middle Pleistocene deposits considered to be indicators for the presence of tunnel valleys were found below the western part of Norderney. Transect 3 shows the wide distribution of these Middle Pleistocene deposits over some hundreds of metres (Fig. 5).
The Pleistocene–Holocene boundary
The landscape relief at the end of the last glacial period (Weichselian) was reshaped during the Holocene. New channels cut into the Pleistocene deposits and existing channel systems were reactivated, deepened and shifted during the Holocene sea-level rise. The precise morphology and depth of the Pleistocene–Holocene boundary and the composition of the overlying Holocene deposits are of particular relevance not only for scientific purposes, but also for planning and managing conservation and development in the coastal region of NW Germany (e.g. offshore wind parks cables and energy/water pipelines from the mainland to the Frisian Islands).
In order to define the morphology of the Pleistocene–Holocene boundary and to reconstruct the related palaeogeographical maps, information about the geological preconditions of the pre-existing landscape (mainly Pleistocene) can be useful. This is especially interesting with regard to resistance to erosion or reworking of previously deposited sediments. Based on both transects and cross-sections from our study, it would appear that the deposits of the Saalian moraine (i.e. Drenthe Main Till) limited the depth of the Riffgat channel. The channel does not appear to cut into the Saalian moraine, but only partially erodes the top of it. However, this situation can only be determined for the 3.5 km2 of the study area. Further research is needed to analyse the limiting impact of the Saalian moraine (at varying depths) on lateral changes in the main channel (or channel system). Such information will be important for prediction of channel shift with regard to ongoing sea-level rise and predicted rises of the tidal range.
Reconstruction of the local palaeolandscape evolution
Based on our analysis of the geometries of the acoustic reflectors, interpreted as deltaic channels and swampy delta plain deposits (F1), a WE-oriented prograding lacustrine delta environment is suggested for the Middle Pleistocene of the Nordeney area (Fig. 7A). This interpretation is supported by detailed analysis of core N72 (i.e. western to core N24b), which contains deposits indicative of a swampy delta plain. In addition, sediments within core N24b have been interpreted as representing a deltaic channel.

Fig. 7. Schematic palaeoenvironmental reconstruction of the Norderney area (figure panels are not to scale; roughly 5 × 5 km). From (A) = oldest to (E) = youngest: (A) Formation of a lacustrine delta in a former Elsterian tunnel valley during the Middle Pleistocene (Holsteinian? or Dömnitz temperate stage). (B) Formation of a sandur plain in front of the glacier, during the Saalian (Main Drenthe). (C) Formation of a transgressional tidal-flat system during the Eemian. (D) Formation of a periglacial meandering river system, with crevasse splays during the Weichselian. (E) Formation of bogs and lagoons during the early Holocene. See Figure 2 for the stratigraphy of Lower Saxony.
Within the channels of the Saalian sandur plain (F2), downstream-migrating structures, e.g. cross-stratification, are present as oblique planar reflectors in transect 2, indicating that the sediment transport direction (cf. Scherer et al., Reference Scherer, Goldberg and Bardola2015) was from NW to SE. This would confirm the results of several authors such as Ehlers et al. (Reference Ehlers, Grube, Stephan, Wansa, Ehlers, Gibbard and Hughes2011) (Fig. 7B).
The base of the Eemian deposits in the study area documents a transgression, with the presence of a mixed tidal-flat system (F4), overlain by a sand tidal-flat system (F5) (Fig. 7C). This configuration is interpreted as representing a southward shift of the Eemian coastline, thus confirming the results of Dechend (Reference Dechend1954) and Dechend & Sindowski (Reference Dechend and Sindowski1956).
It is difficult to find precise, or relevant, palaeoenvironmental information for the trace of the fluvial channel(s) during the Weichselian. Based on the geometries of the acoustic reflectors (i.e. westward-dipping orientation of individual reflectors) from F6, an EW-oriented sediment transport direction within the channel was identified, thus suggesting a possible drainage direction towards the Palaeo-Ems (cf. Hepp et al., Reference Hepp, Romero, Mörz, de Pol-Holz and Hebbeln2019) (Fig. 7D).
With the onset of the Holocene, large bogs developed in the East Frisian area (Fig. 7E) (Grohne, Reference Grohne1957, Reference Grohne1958; Schlütz et al., Reference Schlütz, Enters and Bittmann2021). The individual peat beds (F8) contain occasionally sand grains most likely of aeolian origin. Localised brackish-lagoonal (F9) deposits were also noted, and these appear to thicken from W to E, suggesting that the depositional centre was to the E.
Conclusions
The combination of hydroacoustic methods and sedimentological investigation allowed a series of high-resolution stratigraphic profiles for the western part of the Riffgat channel, at the transition from the tidal inlet to the island of Norderney (Central Wadden Sea, southern North Sea) to be produced. Sediment facies information, derived from eight cores from the WASA project, was correlated with three sub-bottom transects. As a result, acoustic facies could be described and correlated with the lithological and sedimentological facies, resulting in the definition of 12 facies. This integrated approach allowed the palaeoenvironment of a series of Middle Pleistocene lacustrine deposits (Holsteinian? – Dömnitz temperate stage?), the last two interglacials (Eemian-early Holocene), and the last two glacials (Saalian–Weichselian), to be reconstructed, thus illustrating the evolution of the East Frisian area in great detail. Furthermore, eight additional new cores (16 WASA cores in total) and 14 older cores from the LBEG were correlated to generate three short cross-sections at the transition from the tidal inlet (Riffgat channel) to the island of Norderney.
-
1. The Middle Pleistocene lacustrine deltaic deposits are described for the western part of Norderney for the first time. Sedimentary facies descriptions in combination with the acoustic data suggest that these sediments belong either to the Holsteinian limnic facies or to the Dömnitz temperate stage, which are prominent deposits inside Elsterian tunnel valleys. Such Elsterian tunnel valleys may be present below Norderney.
-
2. The Saalian moraine (Drenthe Main Till) was documented over large parts of the recent sea floor. As it is an erosionally resistant layer it appears to be a limiting constraint on the vertical development of tidal inlets and the main tidal channels.
-
3. The core database allowed three new cross-sections across the Riffgat channel and the western head of Norderney to be produced. These cross-sections clearly demonstrate the presence of both Eemian and Middle Pleistocene deposits in the area, in contrast to previously published works.
-
4. Due to stratigraphic contextualisation, it was possible to recognise a series of Eemian shallow marine deposits between the Saalian moraine (Drenthe Main Till) and the Weichselian fluvial deposits. At the western head of Norderney island, an Eemian mixed tidal-flat system is overlain by Eemian sand tidal-flat deposits.
Acknowledgements
This study was performed within the joint research project WASA (The Wadden Sea archive of landscape evolution, climate change and settlement history: exploration – analysis – predictive modelling) funded by the Ministry for Science and Culture of Lower Saxony under ZV 3197. We are grateful to the Wadden Sea National Park administration for fieldwork permission. The EasyCopy company is acknowledged for providing the core-sketching software (EasyCore). We thank the Landesamt für Bergbau, Energie und Geologie (LBEG) for providing the archive core data. Thanks to A. Wurpts, F. Mascioli, T. Kunde (NLWKN), the crews of RV Burchana and RV Senckenberg and to all of the students who helped during the fieldwork. E. Schindler (Senckenberg Research Institute, Frankfurt) is thanked for determination of the crinoid element found in the fine-gravel fraction. Special thanks to E. Drews for her help in creating the figures. Thanks also to T. Scheidl and L. Schenk-Schlautmann for their support. Furthermore, the authors would like to thank the two anonymous reviewers whose comments greatly helped to improve the manuscript.
Supplementary material
Supplementary material is available online at https://doi.org/10.1017/njg.2021.3.