Implications
Broiler production is known to produce large amounts of ammonia, contributing to eutrophication and soil acidification. One efficient way to limit ammonia emission from manure is to decrease nitrogen excretion by broilers by lowering the dietary CP content. We investigated the consequences of such a decrease associated with a supplementation of amino acids (AA) on broiler performance, meat quality and nitrogen utilization during the growing-finishing phase. Using the ideal protein concept, reducing dietary CP content from 19% to 17% decreased nitrogen excretion and volatilization without negative consequences on animal performance or meat quality.
Introduction
The environmental effects of livestock production are well described in the literature (Steinfeld et al., Reference Steinfeld, Gerber, Wassenaar, Castel, Rosales and de Haan2006; de Vries and de Boer, Reference de Vries and de Boer2010; Gerber et al., Reference Gerber, Steinfeld, Henderson, Mottet, Opio, Dijkman, Falcucci and Tempio2013). The main environmental burden caused by broiler production is ammonia emission, responsible for water pollution (eutrophication) and soil acidification (Bouwman et al., Reference Bouwman, Vuuren, Derwent and Posch2002; Méda et al., Reference Méda, Hassouna, Aubert, Robin and Dourmad2011). Ammonia is emitted from the manure by the breakdown of undigested protein and uric acid. As reported by Méda et al. (Reference Méda, Hassouna, Aubert, Robin and Dourmad2011), a possible way to decrease ammonia emission is to decrease nitrogen excretion by lowering the dietary CP content. Indeed, several studies have shown a reduction in nitrogen excretion by about 10% with a 1 percentage point decrease in the dietary CP content in broilers (Aletor et al., Reference Aletor, Hamid, Nieß and Pfeffer2000; Bregendahl et al., Reference Bregendahl, Sell and Zimmerman2002; Gomide et al., Reference Gomide, Rodrigues, Zangeronimo, Bertechini, dos Santos and Alvarenga2011).
Formulation of low CP diets requires controlling the provision of indispensable AA, that is, Lys, Met (+ Cys), Thr, Val, Ile, Leu, Trp, Arg, Phe (+Tyr) and His. In particular, the need for the first limiting indispensable AA can be satisfied by incorporating feed-grade AA. To balance the AA profile of the diet, practical formulation is generally based on an ‘ideal AA profile’ or ‘ideal protein concept’, for instance to maximize growth performance. The indispensable AA requirements in ideal protein are usually expressed relative to the requirement of Lys (National Research Council, 1994; Mack et al., Reference Mack, Bercovici, De Groote, Leclercq, Lippens, Pack, Schutte and van Cauwenberghe1999; Wu, Reference Wu2014). However, contrary to what is classically observed in pigs (Gloaguen et al., Reference Gloaguen, Le Floc’h, Corrent, Primot and van Milgen2014), broiler performance is not maintained systematically when the CP content in the diet is reduced, even when the indispensable AA requirements are apparently met (Aletor et al., Reference Aletor, Hamid, Nieß and Pfeffer2000; Bregendahl et al., Reference Bregendahl, Sell and Zimmerman2002; Dean et al., Reference Dean, Bidner and Southern2006). These findings raise the question of the adequacy of AA provision, and also whether a requirement for indispensable AA or non-indispensable AA such as glycine and its precursors should be met (Dean et al., Reference Dean, Bidner and Southern2006, Siegert et al., Reference Siegert, Wild, Schollenberger, Helmbrecht and Rodenhutscord2015 and Reference Siegert, Ahmadi, Helmbrecht and Rodehutscord2016). Moreover, few studies have been performed during the growing-finishing period (from 21 days of age) whereas this stage of production corresponds to >75% of total feed intake and is the most important in terms of economic (feed cost) and environmental impact (e.g. nitrogen excretion), and the consequences of a reduction in dietary CP content on meat quality are rarely documented. Therefore, the aim of the experiments presented here was to evaluate the effects of reducing the dietary CP content in growing-finishing broilers on animal performance, carcass composition, meat quality and nitrogen utilization (i.e. body retention, excretion and volatilization).
Material and methods
Two successive dose response studies were performed. Experimental procedures and animal care were carried out according to current French legislation and under Authorizations 004601 and 006865 granted to S. T. and H. J., respectively, by the French Ministry of Agriculture, Agrifood and Forestry.
Animals, experimental design and diets
A total of 3000 (experiment 1) and 2000 (experiment 2) 1-day-old Ross PM3 male chicks were reared together on sawdust in an experimental poultry unit from days 1 to 20 (INRA EASM, Le Magneraud, France for experiment 1 and INRA PEAT, Nouzilly, France for experiment 2). Broilers were fed the same starter and grower diets (Supplementary Table S1). They were wing-tagged individually at day 7. At day 21, before the experimental period, they were weighed. Among broilers with similar BW (i.e. within 1 SD), 1520 and 912 were randomly distributed in 40 and 24 pens of 3 m² (experiments 1 and 2, respectively), provided with sawdust (38 broilers/pen; 8 pens/treatment; 12 kg sawdust/pen). The average BW of the selected broilers was the same in both experiments (945±90 g). The ambient temperature programme applied was 31°C from days 0 to 3, 29°C from days 4 to 6, 28°C from days 7 to 13, 26°C from days 14 to 20, 24°C from days 21 to 24, 22°C from days 25 to 27 and 20°C from day 28 until the end of the experiment. The lighting schedule was of 23 h of light from days 0 to 3 and of 18 h of light from days 4 to 31. During the whole experiment, broilers had free access to water and feed. From days 21 to 35 (i.e. the experimental period), broilers were offered one of the experimental pelleted diets shown in Table 1. In both experiments, the experimental diets (Table 1) provided 13.2 MJ ME/kg and were sub-limiting in digestible Lys (dLys) at 0.9% (to be certain that the variations in measured responses are only due to the changes in dietary CP content). In each diet, the actual digestible AA (dAA) to dLys ratios were equal or above the ratios proposed by Mack et al. (Reference Mack, Bercovici, De Groote, Leclercq, Lippens, Pack, Schutte and van Cauwenberghe1999), except for Arg and Thr. The minimum dArg:dLys ratio was 108% (instead of 112%) and the minimum dThr:dLys ratio was 68% (instead of 63%) based on recent literature data (Rostagno et al., Reference Rostagno, Teixeira Albino, Donzele, Gomes, de Oliveira, Lopes, Ferreira, de Toledo Barreto and Euclides2011; Wu, Reference Wu2014) and on results from a previous experiment showing that feed conversion ratio (FCR) could possibly be altered when decreasing the CP content (Supplementary Tables S2 and S3). Indeed, the dThr:dLys ratio proposed by Mack et al. (Reference Mack, Bercovici, De Groote, Leclercq, Lippens, Pack, Schutte and van Cauwenberghe1999) was low in comparison with the recent recommendations. This is particularly important in low CP diets because the concentration of the dispensable AA Gly is reduced and, without Gly supplementation, additional Thr as a precursor of Gly may be required (Dean et al., Reference Dean, Bidner and Southern2006; Siegert et al., Reference Siegert, Wild, Schollenberger, Helmbrecht and Rodenhutscord2015 and Reference Siegert, Ahmadi, Helmbrecht and Rodehutscord2016). The actual ratios of dArg:dLys and dThr:dLys in the experimental diets were therefore equal or above these new ratios (Table 1).
Table 1 Feedstuffs and chemical composition (%) of the diets differing in CP contents fed to broilers between 21 and 35 days of age

AA, amino acids.
1 Supplied per kilogram of diet: NaCl=3 g; Co=0.6 mg; Cu=20 mg; Fe=58 mg; I=2 mg; Mn=80 mg; Se=0.2 mg; Zn=90 mg; retinyl acetate=15 000 IU; cholecalciferol=4300 IU; dl-α tocopheryl acetate=100 mg; thiamine mononitrate=5 mg; riboflavin=8 mg; calcium pantothenate=25 mg; cyanocobalamin=0.02 mg; menadione=5 mg; pyridoxine hydrochloride=7 mg; folic acid=3 mg; biotin=0.3 mg; niacin=100 mg; choline chloride=550 mg; antioxidant (buthylhydroxyanisole, propyl gallat, ethoxyquin)=50 mg.
2 Available phosphorus was calculated from total phosphorus in feedstuffs and availability coefficients from Sauvant et al. (Reference Sauvant, Perez and Tran2004).
3 Digestible AA content was calculated from the total AA feedstuff content (chemical analyses) using digestibility coefficients from Sauvant et al. (Reference Sauvant, Perez and Tran2004).
4 In all diets, AA:Lys ratios were equal or above the ratios proposed by Mack et al. (Reference Mack, Bercovici, De Groote, Leclercq, Lippens, Pack, Schutte and van Cauwenberghe1999): dMet+Cys:Lys=75%, dVal:dLys=81%, dIle:dLys =71% and dTrp:dLys=112%. For Thr and Arg, the minimum ratios were dThr:dLys=68% and dArg:dLys=108%.
For both experiments, the same feedstuff batches were used and analysed before formulation by Ajinomoto Eurolysine S.A.S for total AA contents (Supplementary Table S4). From these analyses, and using digestibility values of AA taken from Sauvant et al. (Reference Sauvant, Perez and Tran2004), dAA content of feedstuffs were calculated, and were used to formulate the experimental diets. In experiment 1, only the two extreme diets were formulated using linear programming to obtain 19% and 15% CP. The three intermediary diets (i.e. 18%, 17% and 16% CP) were obtained by blending these diets at different proportions (75:25, 50:50 and 25:75 for the 18%, 17% and 16% CP diets, respectively; Table 1). In experiment 2, the three experimental diets were formulated using linear programming to reach 19%, 17.5% and 16% CP, respectively (Table 1). Due to these differences in formulation strategy, the intermediary diets in experiment 1 contained the whole range of added feed-grade AA and a higher inclusion of these AA compared with experiment 2. As a result, some dAA concentrations were lower in the 17.5% and 16% CP diets of experiment 2 compared with the 17% and 16% CP diets of experiment 1. Moreover, Arg, Trp and Ile ratios to Lys in the intermediate diets of experiment 1 were above the levels recommended by the adjusted ideal AA profile of Mack et al. (Reference Mack, Bercovici, De Groote, Leclercq, Lippens, Pack, Schutte and van Cauwenberghe1999).
Animal performance, carcass characteristics and meat quality traits
Total feed intake (g) over the experimental period was measured per pen. At the end of the experiment, all broilers were weighed individually after 6 h of fasting. Average BW (g) and BW gain (g) were calculated for each pen. Feed conversion ratio was calculated for the experimental period from feed intake and BW gain. Four broilers per pen, representative of the average BW in the pen, were selected and slaughtered (32 broilers/treatment) in an experimental slaughter house. Broilers were electrically stunned in a water bath and then killed by ventral neck cutting. After partial evisceration (only the gut was removed), whole carcasses were air-chilled and stored at 2°C until the next day. Carcasses, abdominal fat and the left Pectoralis muscles (P. major and P. minor) were weighed and total breast meat weight was calculated ((P. major+P. minor)×2). Abdominal fat and breast meat yield were expressed as a percentage of BW.
In experiment 2, the ultimate pH of the P. major muscle was measured at 24 h postmortem with a portable pH meter (model 506; Crison Instruments SA, Alella, Barcelona, Spain) by inserting a glass electrode directly into the thickest part of the muscle. Breast colour was measured on the cranial ventral side of the muscle using a Miniscan spectrocolorimeter (HunterLab, Reston, VA, USA), and using the CIE LAB (international convention defined by CIE, Vienna, Austria) trichromatic system for lightness (L*) values. After being weighed at 24 h postmortem, the P. major muscle was placed in a hanging plastic bag and stored at 2°C for 96 h. After hanging, the sample was wiped with absorbent paper and weighed again. The difference in weight corresponded to the drip loss and was expressed as the percentage of the initial muscle weight.
Nitrogen utilization
For each pen (n=8/treatment), total nitrogen intake (Nintake, g) was calculated by multiplying total feed intake (FI) of the pen by CP content of the diet and divided by 6.25 (equation (1)). Whole-body nitrogen retention (Nret, g) was estimated according to equation (2) by multiplying the total BW gain of the pen by a constant value for whole-body nitrogen content (Nbody=29 g/kg; ITAVI, 2013) in agreement with previous studies (Aletor et al., Reference Aletor, Hamid, Nieß and Pfeffer2000; Bregendahl et al., Reference Bregendahl, Sell and Zimmerman2002) which showed that whole-body nitrogen content was not affected by a reduction in dietary CP content, even when dietary CP is limiting. The efficiency of nitrogen retention (Neffi, %) was calculated using equation (3). Total excretion of nitrogen (Nexc, g) was calculated according to the difference between Nintake and Nret (equation (4)) and was also expressed per kg of BW gain using equation (5) (Nexc_BWG, g/kg BW gain) to allow comparison between treatments and experiments:





A sample of sawdust was collected before the experimental period, frozen and stored at −20°C. At day 35, four pens per treatment in each experiment were randomly selected. For those pens, total manure was removed and weighed (after broiler withdrawal), from which a sample of 1500 g was taken by mixing all the manure. During the experiments, no extra sawdust was added and no manure was removed. To stop all gaseous emissions from the manure, manure samples were frozen and stored at −20°C. Total nitrogen loss (Nloss, g) through gas emissions was estimated for these pens using equation (6), Wmanure being the total weight of the manure produced in the pen (kg), Nmanure the nitrogen content of the manure (g/kg), Wsawdust the weight of sawdust used as bedding material (Wsawdust=12 kg/pen) and Nsawdust the nitrogen content of the sawdust (g/kg). The nitrogen volatilization rate (Nvol, %) was calculated using equation (7) and expressed as a percentage of total N excretion:


Chemical analyses
Samples of the main feed ingredients and diets, sawdust and manure were analysed for dry matter (DM) (AFNOR method V18-109), nitrogen (Kjedahl method, ISO 1871) and AA content (only for the feed ingredients and diets, ISO 13903) by Ajinomoto Eurolysine S.A.S. Customers Laboratory (Amiens, France). The analysed values for nitrogen and AA content of the experimental diets (Supplementary Table S4) were consistent with expected values.
Statistical Analyses
One-way ANOVA was performed using the GLM procedure of SAS (SAS Institute Inc., Cary, NC, USA) for BW, feed intake, BW gain, FCR, breast meat yield, abdominal fat (both experiments) and ultimate pH, lightness and drip loss (experiment 2). For BW, BW gain, feed intake and FCR the statistical unit was the pen (n=8/treatment). For breast meat yield, abdominal fat, ultimate pH, lightness and drip loss, the statistical unit was the animal (n=32/treatment). Normality of data was assessed using the Shapiro–Wilk test and the hypothesis for a normal distribution of the data was not rejected. Differences between treatments were tested and significance was accepted at P<0.05.
The relationships between Neffi, Nexc_BW, Nvol, nitrogen and moisture (i.e. 100 – DM) contents in the manure content v. CP content of the experimental diet were evaluated using linear regressions (R software v. 3.2.3; R Core Team, Vienna, Austria). In experiment 1, regression methods (i.e. linear, quadratic, linear plateau and curvilinear plateau models) were also used to model the effects of CP on animal performance and carcass characteristics using the REG procedures of SAS.
Results
Animal responses and consequences on carcass and meat quality traits
In experiment 1, reducing the dietary CP content from 19% to 15% did not alter BW, BW gain or feed intake, in contrast to FCR (P<0.01; Table 2). The FCR was significantly higher in the 16% and 15% CP diets compared with the other three diets. The breast meat yield was not affected by the dietary CP. The percentage of abdominal fat was increased by the reduction in dietary CP content (P<0.01), the highest values being observed for the 16% and 15% CP diets (Table 2). Only a linear relation between FCR and CP was found to be significant (FCR=1.95−0.017×CP; R 2=0.53; P<0.001).
Table 2 Performance and carcass characteristics of Ross PM3 male broilers fed with diets differing in CP between 21 and 35 days of age (experiment 1)
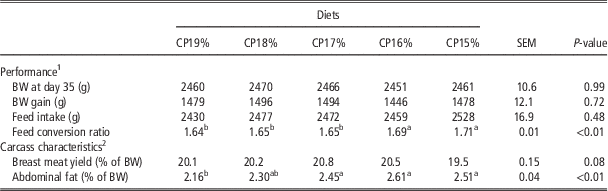
1 n=8/treatment.
2 n=32/treatment.
a,bValues within a row with different superscripts differ significantly at P<0.05.
As shown in Table 3, no significant effect of dietary CP on animal performance (i.e. BW, BW gain, feed intake and FCR) was observed in experiment 2, even though a tendency was observed for feed intake (P=0.053). The percentage of abdominal fat was significantly increased (P=0.02) when decreasing CP content but with no effect on breast meat yield (P=0.06). The dietary CP decrease also had significant effects on meat quality traits with an increase in ultimate pH and a decrease of meat lightness and drip loss (P<0.001 for both ultimate pH and L*, and P=0.045 for drip loss).
Table 3 Performance, carcass characteristics and meat quality traits of Ross PM3 male broilers fed with diets differing in CP contents between 21 and 35 days of age (experiment 2)
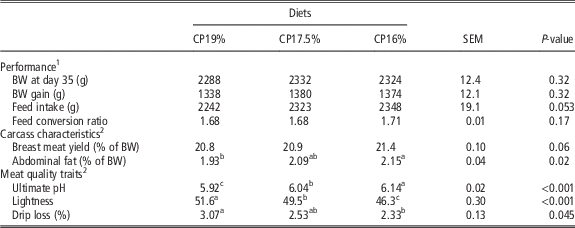
1 n=8/treatment.
2 n=32/treatment.
a,b,cValues within a row with different superscripts differ significantly at P<0.05.
Nitrogen utilization and manure composition
The evolution of estimate efficiency of nitrogen retention, nitrogen excretion, nitrogen and moisture contents in the manure, and nitrogen volatilization with dietary CP content are given in Figure 1a, b, 2a and b, respectively. The efficiency of nitrogen retention increased from +3.2% to +3.6%/CP percentage point when dietary CP content was reduced (Figure 1a). Nitrogen excretion per kg of BW gain decreased with reduced CP content (Figure 1b), from 2.1 to 2.9 g/kg BW gain/CP percentage point (Figure 1b). The analytical results of manure composition are presented in Figure 2a. Nitrogen and moisture content decreased slightly when the dietary CP content was reduced. The calculated nitrogen volatilization also decreased when dietary CP content was reduced (−3.9 to −6.4 points/CP percentage point; Figure 2b).
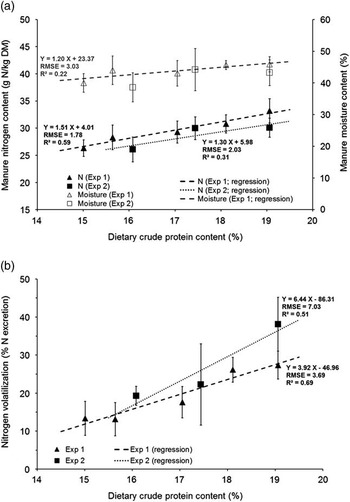
Figure 2 Manure composition and calculated nitrogen volatilization according to the dietary CP content. Average (±SD) values of (a) nitrogen and moisture content of manure and (b) total nitrogen volatilization (Nvol, equation (7)). Linear regressions were fitted with pen values (n=4/treatment). For manure moisture in experiment 2, coefficients of the linear regression were not significantly different from 0.
Discussion
To investigate the effects of reducing the dietary CP content in broiler feed, two successive experiments were performed. Using an adjusted AA profile to test the response to the decrease in dietary CP, no significant effect was found between treatments for BW, BW gain and feed intake in experiment 1, while FCR was maintained until 17% CP and increased below this level. In experiment 2, reducing the dietary CP content did not affect FCR, even with 16% CP. This means that implementing the adjusted ideal AA profile of Mack et al. (Reference Mack, Bercovici, De Groote, Leclercq, Lippens, Pack, Schutte and van Cauwenberghe1999) with changes in dArg:dLys and dThr:dLys ratios allows to decrease the CP content by 2 to 3 percentage points in broiler diets without impairing performance. These findings contradict the decrease in BW gain and feed efficiency frequently described in the literature (Alleman and Leclercq, Reference Alleman and Leclercq1997; Bregendahl et al., Reference Bregendahl, Sell and Zimmerman2002; Dean et al., Reference Dean, Bidner and Southern2006), even though the indispensable AA requirements, in these studies, were apparently met. This negative effect on performance was also reported in a meta-analysis modelling the responses of BW gain and feed efficiency on dietary CP content (Pesti, Reference Pesti2009). In the present study, BW gain was unchanged and the negative impact of the reduction in CP content on FCR was found to be lower than in this meta-analysis (−0.017 v. −0.033 per 1% CP). The improvement in feed efficiency by genetic selection of broilers during this last decade could be an explanation to this difference, as Pesti’s study was based on trials performed before 2007. The reduction in dietary CP content tended to result in an increase in feed intake in experiment 2 (P=0.053). One hypothesis might be that the reduction of dietary CP is associated with a reduction of some indispensable AA such as Leu that, when provided in excess, are known to limit ingestion in various species including pigs (Gloaguen et al., Reference Gloaguen, Le Floc’h, Corrent, Primot and van Milgen2012). Another explanation might be that broilers consumed more feed because they needed more protein to attain their genetic potential according to the theory of feed intake and growth proposed by Emmans (Reference Emmans1987). Nevertheless, more recent findings reported by Gous (Reference Gous2007) did not fully confirm this theory since, instead of increasing feed intake as dietary CP content was reduced, some broiler genotypes reduced their feed intake. The possibility of broilers to adjust feed intake according to their genotype has implications for the optimization of feeding strategies. An increase in feed intake (without an increase in weight gain) would also partly explain the increase in abdominal fat such as the one we observed with low CP diets, the excess of energy being stored as fat.
This significant increase in abdominal fat with reduced CP content is in agreement with the literature, whereas the findings are more controversial for breast meat yield that remained unchanged (with a tendency for an increase) but decreased according to other studies (Alleman and Leclercq, 1997; Berres et al., Reference Berres, Vieira, Dozier, Cortês, de Barros, Nogueira and Kutschenko2010; Namroud et al., Reference Namroud, Shivazad and Zaghari2010). The increase in abdominal fat could here be explained by the increase of the dietary ME:CP ratio when reducing CP (Table 1). This suggests that the use of an adapted AA profile allows maximization of breast meat yield, at least until 16% CP. In addition, meat quality traits measured in experiment 2 were affected by the reduction in dietary CP content. It resulted in an increase in ultimate pH of breast meat. According to several authors, the ultimate pH is negatively correlated to the glycogen content of the muscle (Berri et al., Reference Berri, Besnard and Relandeau2008, Le Bihan-Duval et al., Reference Le Bihan-Duval, Debut, Berri, Sellier, Santé-Lhoutellier, Jégo and Beaumont2008). We assumed that the provision of a more balanced AA profile (associated with the reduction in CP content) reduced the excess of AA, decreasing the amount of nutrients available for glycogen production/storage through AA catabolism. Several studies have indeed shown that the ultimate pH responds to dietary treatments and in particular to variation in the Lys, Met or protein content (Berri et al., Reference Berri, Besnard and Relandeau2008; Jlali et al., Reference Jlali, Gigaud, Metayer-Coustard, Sellier, Tesseraud, Le Bihan-Duval and Berri2012; Conde-Aguilera et al., Reference Conde-Aguilera, Cholet, Lessire, Mercier, Tesseraud and van Milgen2016). Moreover, we showed that the increase in ultimate pH of breast meat was associated with a lower lightness and drip loss, in agreement with results from others (Berri et al., Reference Berri, Besnard and Relandeau2008; Le Bihan-Duval et al., Reference Le Bihan-Duval, Debut, Berri, Sellier, Santé-Lhoutellier, Jégo and Beaumont2008). Moreover, as the ultimate pH increased with decreasing CP content, one can expect an improvement in the processing ability of breast meat, in line poultry processors’ expectations (Alnahhas et al., Reference Alnahhas, Berri, Boulay, Baéza, Jégo, Baumard, Chabault and Le Bihan-Duval2014).
The estimated nitrogen efficiency in the two experiments was improved by 3.2 to 3.6 percentage points/percentage point CP reduction. As shown in Table 4, these values are higher than those reported in the literature (up to +2 percentage points/percentage point CP reduction; Aletor et al., Reference Aletor, Hamid, Nieß and Pfeffer2000; Bregendahl et al., Reference Bregendahl, Sell and Zimmerman2002; Gomide et al., Reference Gomide, Rodrigues, Zangeronimo, Bertechini, dos Santos and Alvarenga2011). Moreover, the efficiency of nitrogen retention for broilers that received diets with <16% CP were above 70%. Even though these values are extremely high and could be questioned, efficiencies >70% have been reported in growing broilers (Siegert et al., Reference Siegert, Ahmadi, Helmbrecht and Rodehutscord2016). Further investigations are required to evaluate the physiological limit to the increase in the efficiency of nitrogen utilization in relation to the maximum metabolic utilization of absorbed AA. Two hypotheses can be formulated to explain the higher nitrogen retention found in our study. First, due to an adequate AA profile in our experimental diets, BW gain was maintained whereas growth and FCR were negatively impacted in other studies. Second, the broilers used in our study may have been more efficient, due to the genetic selection for feed efficiency for over more than a decade.
Table 4 Linear regressions of nitrogen retention efficiency (Neffi) and nitrogen excretion (Nexc_BWG) v. CP content from data published in the literature
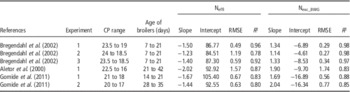
The main consequence of the improvement of the efficiency of nitrogen utilization was the reduction in nitrogen excretion as shown in Figure 1b. As for Neffi, the reduction in excretion (−2.1 to −2.9 g/kg BW gain) was higher in the present experiments than in previous studies (Table 4), which can be explained by a higher efficiency of nitrogen utilization found in our experiment. Between 19% and 16% CP, the reduction of one CP percentage point decreased nitrogen excretion by 13% (Supplementary Table S5 for details on this calculation), while in the literature the decrease was closer to 10% (Aletor et al., Reference Aletor, Hamid, Nieß and Pfeffer2000; Bregendahl et al., Reference Bregendahl, Sell and Zimmerman2002; Gomide et al., Reference Gomide, Rodrigues, Zangeronimo, Bertechini, dos Santos and Alvarenga2011). Moreover, reducing the dietary CP content decreased the nitrogen and moisture content of the manure, which also confirms previous reports on manure and fresh droppings (Ferguson et al., Reference Ferguson, Gates, Taraba, Cantor, Pescatore, Ford and Burnham1998; Si et al., Reference Si, Fritts, Burnham and Waldroup2004; Hernández et al., Reference Hernández, López, Martínez, Megías, Catalá and Madrid2012). This reduction in moisture content may be due to a lower water intake and water excretion by broilers fed the low CP diets (Alleman and Leclercq, 1997; Hernández et al., 2012). In our experiments, the decrease in dietary CP was associated with a lower use of soyabean meal, which is very rich in potassium. A decreased potassium content in the diet can lead to a lower water intake (Alleman and Leclercq, 1997; Francesch and Brufau, Reference Francesch and Brufau2004).
The observed decrease in manure moisture content could also explain the lower volatilization rate of excreted nitrogen in pens with broilers fed low CP diets (−5 points/CP percentage point on average for the two experiments). The moisture content of manure is one of the main factors contributing to the microbial activity in manure, and thus in the transformation of excreted nitrogen into ammonia (Méda et al., Reference Méda, Hassouna, Aubert, Robin and Dourmad2011). This is in agreement with the work of Ferguson et al. (Reference Ferguson, Gates, Taraba, Cantor, Pescatore, Ford and Burnham1998), who reported a decrease of moisture content and nitrogen volatilization in manure when the dietary CP content was reduced. As a consequence, the simultaneous decrease of nitrogen excretion and nitrogen volatilization percentage leads to strong and synergistic decrease of nitrogen amounts lost through volatilization by about −30%/CP percentage point (Supplementary Table S6 for details on this calculation).
Conclusions
In conclusion, the present findings clearly show that a reduction of the dietary CP content by several CP percentage points is possible in growing-finishing broilers with positive implications for the sustainability of broiler production. By using the ideal AA profile of Mack et al. (Reference Mack, Bercovici, De Groote, Leclercq, Lippens, Pack, Schutte and van Cauwenberghe1999) modified for Arg and Thr, growth performance of modern growing-finishing broilers was not affected when the diets provided between 19% and 17% CP. Such a nutritional strategy also had a positive impact on meat quality traits with potential better processing abilities for breast meat, and on the environment with reduced nitrogen excretion and volatilization rates. In the future, other nutritional strategies have to be identified to achieve an even greater decrease in dietary CP (i.e. below 17%). Also, the requirements for the next limiting AA (e.g. Val and Ile, and also non-indispensable AA such as Gly) should be assessed.
Acknowledgements
The authors thank Fréderic Mercerand and Nicolas Besne (INRA PEAT, Nouzilly, France), Philippe Rousseau (INRA EASM, Le Magneraud, France), Michel Couty, Jean-Marc Hallouis, Sabine Crochet, Thierry Bordeau and Estelle Cailleau-Audouin (INRA URA, Nouzilly, France) and Marcelle Eudaimon (Ajinomoto Eurolysine S.A.S. Customers Laboratory, Amiens, France) for their collaboration in this study. They are also grateful to Doreen Raine (Surrey, UK) for editing the English language. This study received financial support from INRA and Ajinomoto Eurolysine S.A.S.
Supplementary materials
To view supplementary material for this article, please visit https://doi.org/10.1017/S1751731117000660