Introduction
According to the World Health Organisation (WHO), vector-borne diseases (VBDs) cause more than 700,000 deaths annually, representing 17% of all infectious diseases around the world. During the last two decades, the emergence, re-emergence and prevalence of some arthropod-borne viruses (arboviruses) have increased worldwide, constituting a major threat to public health (Grubaugh et al. Reference Grubaugh, Ladner, Lemey, Pybus, Rambaut, Holmes and Andersen2019) (Figure 1). Arboviruses are naturally maintained in transmission cycles through the arthropod vector–virus–vertebrate host triad, in which haematophagous arthropods biologically participate in virus transmission between susceptible hosts (Figure 2). Arboviruses, such as Chikungunya virus (CHIKV) and Zika virus (ZIKV), historically restricted to sylvatic regions in Africa are now widely distributed in the tropical zone, causing mild-to-severe disease in humans due to well-established urban transmission cycles (Gould et al. Reference Gould, Pettersson, Higgs, Charrel and de Lamballerie2017). Although there are several determinants of arbovirus emergence and transmission to humans, the close contact between sylvatic vector species from several genera (e.g. Aedes (Ae.), Anopheles, Culex, Haemagogus, Sabethes, Ochlerotatus, Psorophora, Coquillettidia, Ixodes, Amblyomma, Dermacentor, Lutzomyia, Phlebotomus, Culicoides, etc.) and humans in the tropical zone affected by ecological changes associated with the environment-modifying human activity could be of major importance (Echeverry et al. Reference Echeverry, Giraldo and Castaño2012). A more mobile population and migration caused by political and economic instability facilitate the spread of VBDs. New infectious disease agents are introduced into areas where vector populations are not controlled, while massive influxes of susceptible people are coming to unplanned urban and deforested areas. Strengthening current control programmes is therefore fundamental to protect against these risks (Parra-Henao et al. Reference Parra-Henao, Coelho, Escobar, Gonzalvez and Bezerra2021).

Figure 1. Emergence and re-emergence of major arboviral diseases in humans. (a) Emergence and major arbovirus outbreaks in the human population linked to the enzootic cycles. (b) Re-emergence and establishment of relevant arboviruses in urban cycles.

Figure 2. Determinants for arbovirus establishment and dynamics in different transmission settings. The establishment of a virus in a transmission cycle depends on several vector, virus and vertebrate host determinants, including the presence and abundance of the species and the ability of the virus to productively infect every vector and host species.
The vectors become infected by ingesting blood from a viremic vertebrate host or through transovarial or even venereal transmission (Campos et al. Reference Campos, Fernandes, dos Santos, de Miranda, Telleria, Ferreira-de-Brito, de Castro, Failloux, Bonaldo and Lourenço-de-Oliveira2017; Heath et al. Reference Heath, Grossi-Soyster, Ndenga, Mutuku, Sahoo, Ngugi, Mbakaya, Siema, Kitron, Zahiri, Hortion, Waggoner, King, Pinsky and LaBeaud2020). Commonly, despite changes in the transcriptomic profile of vectors associated with viral infection (Bonizzoni et al. Reference Bonizzoni, Dunn, Campbell, Olson, Marinotti and James2012), arboviruses do not exert a deleterious effect on its vector, which could be crucial for long-term virus persistence. In contrast, the same virus could be from mild to extremely pathogenic in vertebrate hosts, where it is usually associated with acute infections (Weaver & Reisen Reference Weaver and Reisen2010).
From an evolutionary ecological perspective, the resulting interactions, if sustained through time, can allow sylvatic arboviruses to explore the fitness landscape and adapt several features including the virus tropism to a new host cell environment, leading to the host range expansion (Coffey et al. Reference Coffey, Forrester, Tsetsarkin, Vasilakis and Weaver2013).
If the virus is successfully amplified in the new host and blood viral loads are high enough for a second round of arthropod infection, a new transmission cycle can be potentially established with unpredictable clinical and epidemiologic consequences (Go et al. Reference Go, Balasuriya and Lee2014). Arbovirus emergence is not a unidirectional spillover process; indeed, we have witnessed the spillback of Yellow fever virus (YFV) and serious concerns exist about the potential establishment of enzootic cycles of urban arboviruses, involving new vectors and hosts/reservoirs (Guth et al. Reference Guth, Hanley, Althouse and Boots2020) (Figure 3).
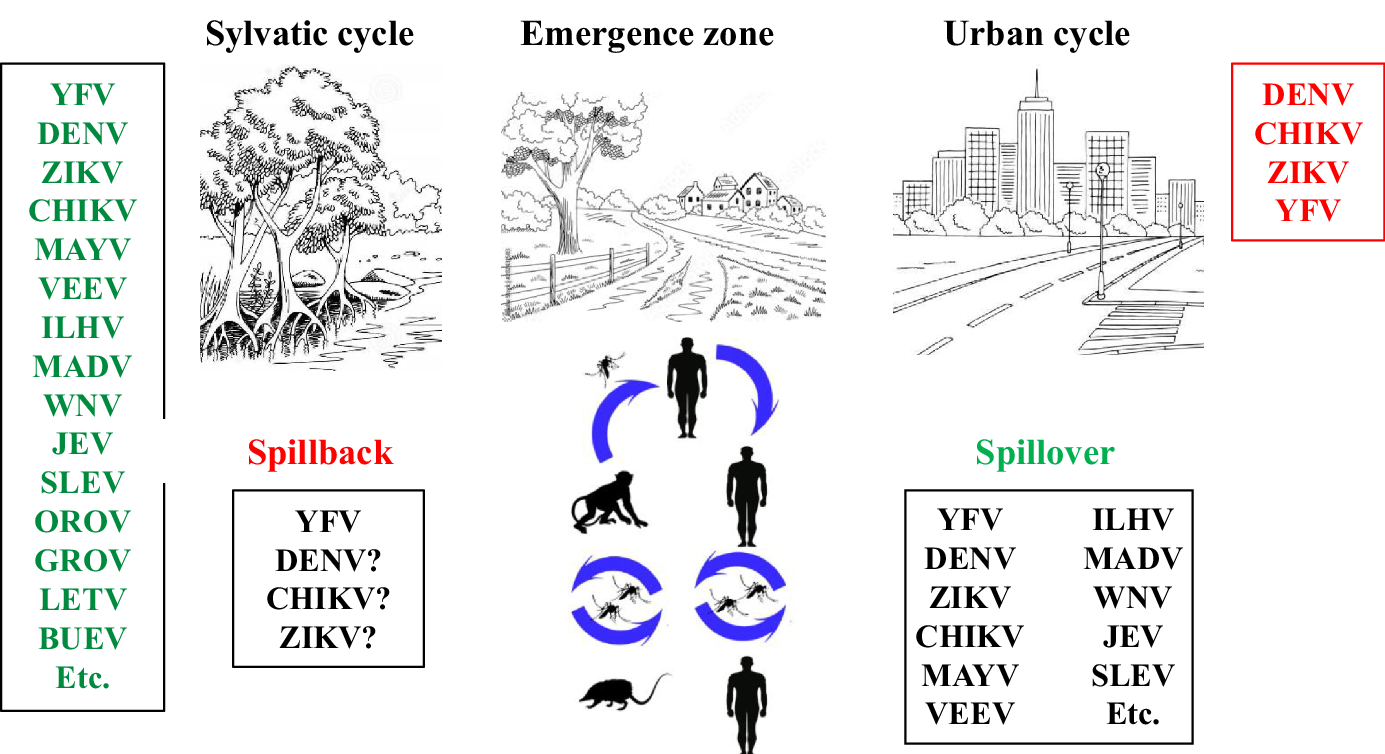
Figure 3. Arbovirus dynamics in different transmission cycles. Arboviruses have been tracked to natural environments where they have evolved to efficiently replicate and transmit from the available vectors and hosts. Ecological factors are involved in the contact between enzootic viruses and humans (spillover), sometimes leading to virus emergence. Eventually, an arbovirus from the urban cycle can again reach the species from natural environments (spillback) and become established in new enzootic cycles.
Several mosquito-borne viruses with a potential impact in public health have been historically identified in enzootic cycles, mainly in the tropical zone (Vasilakis et al. Reference Vasilakis, Tesh, Popov, Widen, Wood, Forrester, Gonzalez, Saluzzo, Alkhovsky, Lam, Mackenzie and Walker2019). They represent different phylogenetic origins and are the source for present and future viral emergence threats.
The implementation of vector surveillance and control programmes and massive vaccination, when possible, have helped to mitigate the impact of arbovirus in public health; however, with the increase in vector resistance to insecticides, the extensive tropical urbanisation and the expansion of the habitat of invasive and highly anthropophilic mosquito vectors, such as Ae. aegypti and Ae. albopictus (Weaver & Reisen Reference Weaver and Reisen2010), including rural and temperate areas, the elimination and potential eradication of arboviral diseases become challenging. For these reasons, the application of robust tools that allow the identification and discovery of viruses with emergence potential, the surveillance of circulating arboviruses and the real-time monitoring are essential to identify, mitigate and predict future outbreaks and epidemics. With the development of next-generation sequencing (NGS) technologies and the metagenomic approach for the unbiased characterisation of nucleic acid sources in complex biological samples (Shi et al. Reference Shi, Lin, Tian, Chen, Chen, Li, Qin, Li, Cao, Eden, Buchmann, Wang, Xu, Holmes and Zhang2016; Zhang et al. Reference Zhang, Shi and Holmes2018), a new early-warning system for virus discovery and surveillance was reached, indeed imposing an enormous challenge for the current virus taxonomy assignments (Simmonds et al. Reference Simmonds, Adams, Benkő, Breitbart, Brister, Carstens, Davison, Delwart, Gorbalenya, Harrach, Hull, King, Koonin, Krupovic, Kuhn, Lefkowitz, Nibert, Orton, Roossinck, Sabanadzovic, Sullivan, Suttle, Tesh, van der Vlugt, Varsani and Zerbini2017). One of the main tasks to reduce the public health impact of arboviral diseases is to expand the tools and methods for virus identification and discovery, which is also fundamental to unravel transmission dynamics and to an adequate global genomic surveillance strategy and response to eventual emergence and re-emergence episodes, as it has been recently encouraged by the WHO (WHO 2022).
Sylvatic origin and emergence of mosquito-borne viruses
In general, mosquito-borne viruses are confined to sylvatic areas and naturally circulate between vertebrate reservoirs or hosts and permissive and competent arthropod vectors. Most of the public health relevant arboviruses originally circulated in non-human primates (NHPs) in sylvatic areas (Braack et al. Reference Braack, Gouveia De Almeida, Cornel, Swanepoel and De Jager2018), where several mosquito species or other haematophagous arthropods would have allowed the transmission from viremic to susceptible vertebrates, enabling a transmission dynamic known as the enzootic, jungle or sylvatic cycle (Valentine et al. Reference Valentine, Murdock and Kelly2019). NHPs rarely show signs of disease despite their important role in virus amplification and maintenance in nature (Valentine et al. Reference Valentine, Murdock and Kelly2019), which could be interpreted as an evolutionarily stable strategy warranting low virulence and host survival. Phylogenetic analysis of most mosquito-borne viruses suggests an ancestral origin in the sylvatic area of the old world (Gould et al. Reference Gould, de Lamballerie, Zanotto and Holmes2003) with several emergence attempts and a few successful establishments in the human population. A notorious example of a successful emergence as a common process is the establishment of Dengue virus (DENV) in the urban cycle, with phylogenetic evidence (closely related sequences obtained from strains circulating in mosquitoes and NHPs in forests in Asia and Africa) of independent origin from sylvatic ancestors for at least three of the four DENV serotypes (Vasilakis et al. Reference Vasilakis, Cardosa, Hanley, Holmes and Weaver2011; Wang et al. Reference Wang, Ni, Xu, Barrett, Watowich, Gubler and Weaver2000).
As a spillover event, humans could be exposed to an arbovirus by contact with vectors entering areas of human settlements (e.g. rural or peri-urban areas of populated centres and indigenous communities living far from the cities) in search of blood meals, mainly through bridge vectors that could move and feed on several vertebrate hosts in transition zones (Hoyos et al. Reference Hoyos, Carrasquilla, León, Montgomery, Salyer, Komar and González2021); by the direct incursion of people in sylvatic settings for several activities, such as hunting, deforestation and mining (Valentine et al. Reference Valentine, Murdock and Kelly2019); by the contact of a vertebrate host or reservoir from the enzootic cycle with vectors from the urban area that could lead to virus adaptation to a new potential vector and host; and finally, by the initial adaptation to domestic vertebrates; the virus could reach the human host. The result of the interaction of a new viral entity with humans could be unpredictable as the introducing virus might not find a suitable or permissive host to replicate and reach enough viral titers to establish a second round of infection (Weaver et al. Reference Weaver, Charlier, Vasilakis and Lecuit2018); therefore, humans act as a dead-end host, impeding new rounds of transmission from this source (Ellwanger & Chies Reference Ellwanger and Chies2021). For instance, it has been reported that Japanese encephalitis virus (JEV) infects swine, Rift Valley fever virus (RVFV) infects cattle and sheep, West Nile virus (WNV) infects birds and Venezuelan equine encephalitis virus (VEEV) infects equids, with eventual or sometimes frequent spillover events to humans (Guzmán-Terán et al. Reference Guzmán-Terán, Calderón-Rangel, Rodriguez-Morales and Mattar2020).
Under certain conditions, arboviruses might find an appropriate environment to establish a transmission cycle in a new environment for a prolonged time (Vasilakis et al. Reference Vasilakis, Cardosa, Hanley, Holmes and Weaver2011). First, vector competence is crucial for the success in the process. Several features are required for a productive viral infection of the vector, including the presence of appropriate receptors and cell factors in the cells at the midgut and salivary glands to allow virus entry and successful replication and to overcome the different mosquito barriers to become competent for transmission (Kuno & Chang Reference Kuno and Chang2005). In addition, the infection should not negatively affect the vector’s life cycle. Second, the arbovirus should reach a naïve or significantly susceptible host population without history of previous immunological challenges by the infecting arbovirus or other viruses belonging to the same serocomplexes. Third, in the fact of virus inoculation through haematophagous arthropod bite, viraemia levels should be high enough to sustain secondary rounds of vector–host transmission (Pereira dos Santos et al. Reference Pereira dos Santos, Roiz, Santos de Abreu, Luz, Santalucia, Jiolle, Santos Neves, Simard, Lourenço-de-Oliveira and Paupy2018; Valentine et al. Reference Valentine, Murdock and Kelly2019).
Mosquito-borne viruses with major public health impact
During the last decades, different arboviruses have successfully emerged or re-emerged in urban settlements, such as YFV, DENV, CHIKV, ZIKV, WNV, VEEV and JEV, causing small outbreaks or even epidemics of major importance (Weaver & Reisen Reference Weaver and Reisen2010).
YFV is the representative species of the family Flaviviridae and genus Flavivirus. Sailing from Africa to the Americas during the colonisation period, YFV became established in urban transmission cycle during the 15th and 16th centuries, involving Ae. aegypti (Barrett & Monath Reference Barrett and Monath2003; Reed et al. Reference Reed, Carroll, Agramonte and Lazear2001). In 1937, the use of the 17D vaccine was implemented for the first time by attenuating an isolated strain of an asymptomatic individual. This vaccine was used in population older than 9 years and is still administered in endemic countries (Frierson Reference Frierson2010; Theiler & Smith Reference Theiler and Smith1937). In 1961, most of the American countries were considered free of Ae. aegypti, by the intense and efficient vector control and eradication campaigns led by WHO, and the efforts of immunisation through administration of the vaccine achieved the successful interruption of the urban cycle (Soper Reference Soper1963). However, YFV managed to infect sylvatic mosquitoes, such as Sabethes spp. and Haemagogus spp., and new world NHPs, establishing a successful sylvatic transmission cycle in different countries in the Americas. While only sporadic epizootics and human cases were since annually reported from endemic countries in the Americas, a major outbreak was registered in the municipalities of Minas Gerais, Sao Paulo and Rio de Janeiro, Brazil during 2016–2019, with at least 2,240 human cases and 760 deaths (Sacchetto et al. Reference Sacchetto, Silva, Rezende de, Arruda, Costa, de Mello, Oliveira, Alves, de Mendonça, Stumpp, Prado, Paglia, Perini, Lacerda Nogueira, Kroon, de Thoisy, Trindade de and Drumond2020).
DENV is the flavivirus of major epidemiologic impact, with at least 390 million infections worldwide each year, including 96 million symptomatic cases and around 50,000 deaths (Bhatt et al. Reference Bhatt, Gething, Brady, Messina, Farlow, Moyes, Drake, Brownstein, Hoen, Sankoh, Myers, George, Jaenisch, William Wint, Simmons, Scott, Farrar and Hay2013). Four serotypes comprise the DENV serocomplex, all of them currently circulating in the urban cycle involving the human host and Aedes spp. as vectors, mainly Ae. aegypti. There is phylogenetic evidence for the independent emergence of at least three of the four DENV serotypes, from forest areas in Africa and Asia (Wang et al. Reference Wang, Ni, Xu, Barrett, Watowich, Gubler and Weaver2000). Despite efforts to develop a vaccine, its use is currently limited to people with previous DENV exposure, and the intensified control of the mosquito vector has shown to be unfruitful, leading to virus maintenance with DENV epidemic periods happening every 3–5 years in hyperendemic countries where different serotypes are co-circulating (Laiton-Donato et al. Reference Laiton-Donato, Alvarez, Peláez-Carvajal, Mercado, Ajami, Bosch and Usme-Ciro2019).
CHIKV belongs to the family Togaviridae and genus Alphavirus. It was first isolated in Tanzania in 1952 (Robinson Reference Robinson1955) and was confined to Asia and Africa until the 1960s–1970s, with esporadic reports (De Ranitz et al. Reference De Ranitz, Myers, Varkey, Isaac and Carey1965). The virus re-emerged in epidemic form in East Africa in 2004 and quickly spread to tropical and subtropical countries in Africa, Asia and Oceania. In 2013, CHIKV reached the Caribbean through Saint Martin island and in short time it spread to several continental countries of the Americas (Morrison Reference Morrison2014; Mowatt & Jackson Reference Mowatt and Jackson2014; Tsetsarkin et al. Reference Tsetsarkin, Vanlandingham, McGee and Higgs2007; Weaver Reference Weaver2014). CHIKV Asian genotype was successfully disseminated through the Americas owing to the widespread and high densities of Ae. Aegypti, especially in urban areas, while the ECSA (East-Central-South African) genotype spread mainly in Europe where the A226V amino acid substitution in the E1 protein conferred the virus the ability to successfully replicate in the vector, Ae. albopictus (Tsetsarkin et al. Reference Tsetsarkin, Vanlandingham, McGee and Higgs2007)
ZIKV belongs to the family Flaviviridae, genus Flavivirus. It was isolated for the first time in Uganda in 1947 (Dick Reference Dick1952). The first human cases were reported in Nigeria during 1954 (MacNamara Reference MacNamara1954) and ZIKV distribution was limited to Africa and Asia until 2007, when it spread to different Pacific Islands (Attar Reference Attar2016; Hayes Reference Hayes2009). In 2015, ZIKV was introduced to Brazil, presumably from French Polynesia (Musso Reference Musso2015; Zanluca et al. Reference Zanluca, Melo de, Mosimann, Santos dos, Santos dos and Luz2015) and subsequently dispersed to several countries of the Americas (European Centre for Disease Prevention and Control 2018; Fauci & Morens Reference Fauci and Morens2016). ZIKV has been involved in economic and social burden for public health systems for the atypical disease manifestations, among them, microcephaly in neonates (Pan American Health Organisation 2015; Ventura et al. Reference Ventura, Maia, Bravo-Filho, Góis and Belfort2016) and Guillain Barré syndrome (Oehler et al. Reference Oehler, Watrin, Larre, Leparc-Goffart, Lastãre, Valour, Baudouin, Mallet, Musso and Ghawche2014). Moreover, it has been described the possibility of sexual transmission of ZIKV (Moreira et al. Reference Moreira, Peixoto, Siqueira and Lamas2017; Musso et al. Reference Musso, Roche, Robin, Nhan, Teissier and Cao-Lormeau2015). The high density of Ae. aegypti in urban settings and ineffective vector control measures have sustained endemic/epidemic DENV transmission from 1970s and have been determinant for the rapid dissemination of CHIKV and ZIKV in the Americas.
WNV is a member of the genus Flavivirus, family Flaviviridae. It was first isolated in Uganda in 1937 and since then, it has spread extensively to the Middle East, Europe, South Asia and Australia. The first urban epidemic was reported in 1996 in Bucharest, Romania, and the first report in America was in 1999 after an arboviral encephalitis outbreak in New York City (Nash et al. Reference Nash, Mostashari, Fine, Miller, O’Leary, Murray, Huang, Rosenberg, Greenberg, Sherman, Wong, Campbell, Roehrig, Gubler, Shieh, Zaki, Smith and Layton2001). WNV has migratory birds as the main amplifying hosts that occasionally transmit the virus to domestic birds and mammals, including horses and humans through the bite of mosquitoes from the genus Culex. Mammals act as dead-end hosts as they are accidental hosts that do not play an essential role in maintaining the transmission cycle (Johnston & Conly Reference Johnston and Conly2000). Just before WNV was detected in human population in the Americas, an unusual increase in deaths of crows was observed in New York city and the virus responsible for the outbreak was demonstrated to be closely related to the virus that caused an outbreak of disease in geese in Israel in 1998 (Weaver & Reisen Reference Weaver and Reisen2010). This zoonosis underscores the importance of the One Health approach to establish stronger links between animal and human disease and the potential use of animals as sentinels for outbreak prediction and surveillance.
VEEV belongs to the genus Alphavirus, family Togaviridae. The virus was first isolated in 1935 after equine outbreaks in Venezuela (Lundberg et al. Reference Lundberg, Carey and Kehn-Hall2017). It is mainly distributed in South and Central America. The principal host of the epizootic cycle is horses and main vectors are Ae. taeniorhynchus and Culex species, while the human is an accidental host (Zacks & Paessler Reference Zacks and Paessler2010). During the epizootic cycle, the equine fatality rate was 19–83% and 14% of encephalitis incidence in humans has been estimated (Zacks & Paessler Reference Zacks and Paessler2010). There are six antigenic subtypes (I–VI), and only the subtype I strains have been associated with human disease (Forrester et al. Reference Forrester, Wertheim, Dugan, Auguste, Lin, Adams, Chen, Gorchakov, Leal, Estrada-Franco, Pandya, Halpin, Hari, Jain, Stockwell, Das, Wentworth, Smith, Kosakovsky Pond and Weaver2017; Kumar et al. Reference Kumar, Manuja, Gulati, Virmani and Tripathi2018).
JEV belongs to the family Flaviviridae, genus Flavivirus, and the virus was isolated from a human brain in the 1930s (Solomon Reference Solomon2006). There is an estimation of 30,000–50,000 cases of Japanese encephalitis each year with a fatality rate as high as 30% and peaks each 2–15 years (WHO 2019). Japanese encephalitis is found especially in Asia, Western Pacific and Australia (Erlanger et al. Reference Erlanger, Weiss, Keiser, Utzinger and Wiedenmayer2009). JEV has been isolated from humans and farm animals, and the principal vectors are Culex tritaeniorhynchus and Culex vishnui (Solomon Reference Solomon2006). At the phylogenetic level, JEV has been classified into four genotypes, the genotype 1 being the more frequent during the last 30 years (Gao et al. Reference Gao, Liu, Wang, Fu, Guo and Liang2013).
Worldwide, there is a plethora of arboviruses that could have the potential to emerge, but our knowledge of their ecological dynamics and adaptive potential is limited. Among them, Mayaro virus (MAYV), Usutu virus (USUV), Oropouche virus (OROV), RVFV and Saint Louis encephalitis virus (SLEV) have reached some interest (Esposito & Fonseca Reference Esposito and Fonseca da2017; Gould et al. Reference Gould, Pettersson, Higgs, Charrel and de Lamballerie2017) and justify further surveillance.
Arbovirus spillback from the urban cycle
While spillover events have been widely reported, evidence of the establishment of sylvatic cycles by arboviruses circulating in urban areas, known as spillback, is scarce and only represented by the new word sylvatic YFV cycle, despite being plausible for other arboviruses due to the proximity of peri-urban, rural and sylvatic areas in several scenarios of the tropical world that allow the interaction between arboviruses and potential new vectors and vertebrate hosts (Figure 3).
Despite YFV being officially eradicated from the urban cycle in the Americas, epizootics and human cases have been periodically reported ever since (Cunha et al. Reference Cunha dos, Duarte-Neto, Pour, Ortiz-Baez, Černý, Pereira de, Braconi, Ho, Perondi, Sztajnbok, Alves, Dolhnikoff, Holmes, Saldiva and Zanotto de2019), involving new world NHPs from the genera Aotus, Alouatta, Cebus, Ateles, Callithrix and Saimiri and mosquito vectors from the genera Haemagogus and Sabethes (Domingo et al. Reference Domingo, Charrel, Schmidt-Chanasit, Zeller and Reusken2018). While it is uncertain if the circulating sylvatic YFV in the Americas has conserved its ancestral biological ability to infect the urban vector, Ae. aegypti, vaccination coverages in areas of demonstrated natural transmission have been the major measure to avoid its re-emergence in the urban cycle, being used massively during the 2016–2017 outbreak in Brazil (WHO/PAHO 2017).
Ecological factors, and the viral, vector and vertebrate features discussed above as determinants for the spillover event and viral emergence could be critical for the success of the arbovirus spillback, mainly those related to the close and sustained contact between original and potentially new hosts leading to the gradual and successful virus adaptation (Dennehy et al. Reference Dennehy, Friedenberg, Holt and Turner2006), and to the ability of the virus to reach virus loads enough to warrant transmission through the mosquito bite.
The historical circulation of DENV, and the recent re-emergence and establishment of CHIKV and ZIKV in the urban cycle in the Americas represent a potential risk for a new sylvatic cycle to be established (Althouse et al. Reference Althouse, Vasilakis, Sall, Diallo, Weaver and Hanley2016; Figueiredo Reference Figueiredo2019). Human outbreaks, as well as the natural infection of urban and sylvatic mosquitos in peri-urban and rural settings have been documented (Alencar et al. Reference Alencar, Ferreira de Mello, Brisola Marcondes, Érico Guimarães, Toma, Queiroz Bastos, Olsson Freitas Silva and Lisboa Machado2021; Catenacci et al. Reference Catenacci, Ferreira, Fernandes, Padda, Travassos-da-Rosa, Deem, Vasconcelos and Martins2021; Hoyos-López et al. Reference Hoyos-López, Atencia-Pineda and Gallego-Gómez2019; Lee et al. Reference Lee, Vasco, Márquez, Zuniga-Moya, Van Engen, Uruchima, Ponce, Cevallos, Trueba, Trostle, Berrocal, Morrison, Cevallos, Mena, Coloma and Eisenberg2021).
Recently, vector competence of the sylvatic mosquitoes Haemagogus leucocelaenus and Ae. terrens for CHIKV transmission was experimentally demonstrated (Lourenço-de-Oliveira & Failloux Reference Lourenço-de-Oliveira and Failloux2017), and although these species are mainly confined to the tropical rainforest, their populations can establish in human-modified rural areas (Cardoso et al. Reference Cardoso da, de Almeida, dos Santos, da Fonseca, Sallum, Noll, Monteiro, Cruz, Carvalho, Pinto, Castro, Neto, Segura and Vasconcelos2010). Seroprevalence studies have failed to show evidence of CHIKV circulation in NHPs in the Americas (Moreira-Soto et al. Reference Moreira-Soto, Carneiro de, Fischer, Feldmann, Kümmerer, Silva, Santos, Souza de, Liborio de, Valença-Montenegro, Laroque de, da Fontoura, Oliveira, Drosten, de Lamballerie, Franke and Drexler2018), while in Africa, it was determined that NHPs are amplifiers (Althouse et al. Reference Althouse, Guerbois, Cummings, Diop, Faye, Faye, Diallo, Sadio, Sow, Faye, Sall, Diallo, Benefit, Simons, Watts, Weaver and Hanley2018), and in Asia, a low seroprevalence of CHIKV in NHPs was described (Rougeron et al. Reference Rougeron, Sam, Caron, Nkoghe, Leroy and Roques2015). Mathematical models have predicted that ZIKV could establish sylvatic transmission cycles in South America (Althouse et al. Reference Althouse, Vasilakis, Sall, Diallo, Weaver and Hanley2016) near to urban regions where it is endemic (Vorou Reference Vorou2016), due to the presence of the YFV vectors, and abundant NHP species suitable for acting as sylvatic hosts, as it has been experimentally supported (Li et al. Reference Li, Acosta, Hill, Brady, de Almeida, Cardoso da, Hamlet, Mucci, Telles de Deus, Iani, Alexander, Wint, Pybus, Kraemer, Faria and Messina2022).
Metagenomic NGS for the identification and discovery of new mosquito-borne viruses with potential public health implications
Conventional methods have been used to detect the viral agent through viral isolation in permissive cell cultures and virus characterisation via serologic and sequence-based molecular methods, and several arboviruses were detected or discovered, and associated with animal and human health through this classic virologic approach during the last seven decades (Kauffman et al. Reference Kauffman, Franke, Wong and Kramer2010). However, only a fraction of viruses can be detected in this way due to the low sensitivity of cell culture to allow replication of all possible species of arboviruses that can be present in a biological sample, and the fact that conventional nucleic acid amplification techniques require a priori knowledge of the virus genetic background, limiting the whole description of the virodiversity and the discovery of new arbovirus species with emerging potential (Zhang et al. Reference Zhang, Shi and Holmes2018).
The enormous diversity of viruses, as an essential component for the equilibrium in natural ecosystems, is yet waiting to be unraveled (French & Holmes Reference French and Holmes2020), as it can be inferred from recent metagenomic studies (Roux et al. Reference Roux, Matthijnssens and Dutilh2021; Shi et al. Reference Shi, Lin, Tian, Chen, Chen, Li, Qin, Li, Cao, Eden, Buchmann, Wang, Xu, Holmes and Zhang2016). In the new wave of the genomics era, high-throughput sequencing technologies have been made available for genomic surveillance and monitoring of known mosquito-borne viruses (Andrade et al. Reference Andrade de, Campos, Campos, Abreu, Melo, Sevá da, Cardoso da, Dos Santos, Born, Silva da, Müller, Oliveira de, Silva da, Simonini-Teixeira, Bernal-Valle, Mares-Guia, Albuquerque, Romano, Franco, Ribeiro, Roehe and Almeida de2021; Edgerton et al. Reference Edgerton, Thongsripong, Wang, Montaya, Balmaseda, Harris and Bennett2021; Giovanetti et al. Reference Giovanetti, Faria, Lourenço, Goes de Jesus, Xavier, Claro, Kraemer, Fonseca, Dellicour, Thézé, da Silva Salles, Gräf, Silveira, do Nascimento, Costa de Souza, de Melo Iani, Castilho-Martins, Cruz, Wallau, Fabri, Levy, Quick, de Azevedo, Aguiar, de Oliveira, Bôtto de Menezes, da Costa Castilho, Terra, Souza da Silva, Bispo de Filippis, Luiz de Abreu, Oliveira, Croda, Campelo de Albuquerque, Nunes, Sabino, Loman, Naveca, Pybus and Alcantara2020). When combined to robust unbiased molecular approaches for experimental data collection (Conceição-Neto et al. Reference Conceição-Neto, Yinda, Van Ranst and Matthijnssens2018; Lewandowska et al. Reference Lewandowska, Zagordi, Geissberger, Kufner, Schmutz, Böni, Metzner, Trkola and Huber2017; López-Labrador et al. Reference López-Labrador, Brown, Fischer, Harvala, Van Boheemen, Cinek, Sayiner, Madsen, Auvinen, Kufner, Huber, Rodriguez, Jonges, Hönemann, Susi, Sousa, Klapper, Pérez-Cataluňa, Hernandez, Molenkamp, der Hoek van, Schuurman, Couto, Leuzinger, Simmonds, Beer, Höper, Kamminga, Feltkamp, Rodríguez-Díaz, Keyaerts, Nielsen, Puchhammer-Stöckl, Kroes, Buesa, Breuer, Claas and de Vries2021) and computational analysis (Ajami et al. Reference Ajami, Wong, Ross, Lloyd and Petrosino2018; de Vries et al. Reference de Vries, Brown, Couto, Beer, Le Mercier, Sidorov, Papa, Fischer, Oude Munnink, Rodriquez, Zaheri, Sayiner, Hönemann, Pérez-Cataluña, Carbo, Bachofen, Kubacki, Schmitz, Tsioka, Matamoros, Höper, Hernandez, Puchhammer-Stöckl, Lebrand, Huber, Simmonds, Claas and López-Labrador2021; Kalantar et al. Reference Kalantar, Carvalho, de Bourcy, Dimitrov, Dingle, Egger, Han, Holmes, Juan, King, Kislyuk, Lin, Mariano, Morse, Reynoso, Cruz, Sheu, Tang, Wang, Zhang, Zhong, Ahyong, Lay, Chea, Bohl, Manning, Tato and DeRisi2020; Vilsker et al. Reference Vilsker, Moosa, Nooij, Fonseca, Ghysens, Dumon, Pauwels, Alcantara, Vanden Eynden, Vandamme, Deforche and de Oliveira2019; Ye et al. Reference Ye, Siddle, Park and Sabeti2019), the metagenomic NGS (mNGS) emerges as a powerful approach for molecular characterisation of the ‘infectome’ of cases of clinical relevance (Holmes et al. Reference Holmes, Rambaut and Andersen2018).
Through the mNGS approach, it is now possible to obtain millions of sequence reads from RNA or DNA molecules present in a biological sample, which constitutes an unprecedented amount of information for a full characterisation of the presence of biological entities, without previous knowledge of the suspected agents (Vasilakis et al. Reference Vasilakis, Tesh, Popov, Widen, Wood, Forrester, Gonzalez, Saluzzo, Alkhovsky, Lam, Mackenzie and Walker2019). By using robust bioinformatic tools and directed pipelines, sequence reads are submitted to quality control, and then, rationally trimmed to eliminate nucleotide sequences at the ends, corresponding to the indexes, adapters or primers that were used during the Polymerase Chain Reaction (PCR)-based enrichment and library preparation for NGS (Hess et al. Reference Hess, Kohl, Kotrová, Rönsch, Paprotka, Mohr, Hutzenlaub, Brüggemann, Zengerle, Niemann and Paust2020). There are two main strategies for metagenomic assembly: first, with previous knowledge of the infectious agent, single reads are mapped to a reference genome. On the other hand, a robust and unbiased approach for de novo assembly of single reads into contigs or scaffolds generates a high number of assembled sequences that can be confronted to genome databases in the search for sequence homology and identity (Bahassi & Stambrook Reference Bahassi and Stambrook2014) (Figure 4).

Figure 4. Genomic characterisation through next-generation sequencing (NGS). Bioinformatic tools allow the successful assembly of a nearly complete genome of an arbovirus based on a reference sequence. The coverage is represented horizontally by the regions for which genetic information was obtained, in this case only excluding the 5’ and 3’ ends of the viral genome. The depth is represented vertically by the number of reads that contribute genetic information to every nucleotide position.
Several viruses (including arboviruses) have been identified as aetiological agents of human disease, by using the mNGS approach (Grard et al. Reference Grard, Fair, Lee, Slikas, Steffen, Muyembe, Sittler, Veeraraghavan, Ruby, Wang, Makuwa, Mulembakani, Tesh, Mazet, Rimoin, Taylor, Schneider, Simmons, Delwart, Wolfe, Chiu and Leroy2012; Huang et al. Reference Huang, Wang, Gao, Lü, Cao, Deng and Yue2021; Phan et al. Reference Phan, del Valle Mendoza, Sadeghi, Altan, Deng and Delwart2018; Piantadosi et al. Reference Piantadosi, Mukerji, Ye, Leone, Freimark, Park, Adams, Lemieux, Kanjilal, Solomon, Ahmed, Goldstein, Ganesh, Ostrem, Cummins, Thon, Kinsella, Rosenberg, Frosch, Goldberg, Cho and Sabeti2021; Quintão et al. Reference Quintão de, Slavov, de Oliveira, Bezerra dos, Cassemiro, Alves de, Gontijo, Martins dos, Gurgel da, Noronha, Ramalho, de Araújo, Pereira and Haddad2022; Reyes et al. Reference Reyes, Carbo, Harinxma thoe Slooten van, Kraakman, Sidorov, Claas, Kroes, Visser and De2021; Tang & Chiu Reference Tang and Chiu2010; Xie & Zhu Reference Xie and Zhu2021; Yozwiak et al. Reference Yozwiak, Skewes-Cox, Stenglein, Balmaseda, Harris and DeRisi2012), including the pandemic SARS-CoV-2 (Wu et al. Reference Wu, Zhao, Yu, Chen, Wang, Song, Hu, Tao, Tian, Pei, Yuan, Zhang, Dai, Liu, Wang, Zheng, Xu, Holmes and Zhang2020). This approach can also be used for the rapid identification of new vector species, the feeding sources and the screening for arboviruses, allowing the surveillance of potential emerging viruses and the prevention and control of VBDs (Batovska et al. Reference Batovska, Lynch, Cogan, Brown, Darbro, Kho and Blacket2018). When applied to the whole body, midgut, salivary glands or expectorated saliva samples of haematophagous arthropods that feed on the blood of several vertebrate hosts, including mammals and birds, this strategy contributes to vector incrimination in enzootic, endemic and epidemic transmission cycles and to the assessment of the risk for the human population (Batson et al. Reference Batson, Dudas, Haas-Stapleton, Kistler, Li, Logan, Ratnasiri and Retallack2021; Birnberg et al. Reference Birnberg, Temmam, Aranda, Correa-Fiz, Talavera, Bigot, Eloit and Busquets2020; Brinkmann et al. Reference Brinkmann, Nitsche and Kohl2016; Charles et al. Reference Charles, Tangudu, Hurt, Tumescheit, Firth, Garcia-Rejon, Machain-Williams and Blitvich2018; Grubaugh et al. Reference Grubaugh, Sharma, Krajacich, Fakoli, Bolay, Diclaro, Johnson, Ebel, Foy and Brackney2015; Hameed et al. Reference Hameed, Khan, Xu, Zhang, Wang, Di, Chen, Naveed Anwar, Wahaab, Ma, Nawaz, Liu, Li, Shao, Qiu, Wei and Ma2021; Russell et al. Reference Russell, Campos, Stone, Blosser, Burkett-Cadena and Jacobs2018; Xiao et al. Reference Xiao, Li, Zhang, Han, Guo, Xie, Tian, Li, Wang, Liu, Ren, Zhou, Lu and Jin2018; Yang et al. Reference Yang, Garver, Bingham, Hang, Jochim, Davidson, Richardson and Jarman2015).
More recently, the whole genome characterisation of viruses has been proposed as a powerful tool for the identification of changes that contribute to viral fitness, virulence of strains, transmission capacity and epidemic potential. This characterisation also provides information for other research approaches, including the detection of epidemiological changes through genotype surveillance, phylogeographic analysis, interaction with host receptors and vector competence (Geoghegan & Holmes Reference Geoghegan and Holmes2018). In the clinical and veterinary fields, mNGS is a current and future trend, as it allows the rapid identification of viruses and microbial pathogens causing diseases of unknown aetiology and the surveillance of new potential zoonotic threats. Perhaps the most important application of this technology is the possibility of integrating the mNGS approach to the clinical, epidemiological and ecological information from humans, domestic animals, wild vertebrates and vectors, as a One Health framework to establish early-warning public health systems for a rapid response (Bohl et al. Reference Bohl, Lay, Chea, Ahyong, Parker, Gallagher, Fintzi, Man, Ponce, Sreng, Kong, Oliveira, Kalantar, Tan, Fahsbender, Sheu, Neff, Detweiler, Yek, Ly, Sath, Huch, Kry, Leang, Huy, Lon, Tato, DeRisi and Manning2022; Botti-Lodovico et al. Reference Botti-Lodovico, Nair, Nosamiefan, Stremlau, Schaffner, Agignoae, Aiyepada, Ajogbasile, Akpede, Alhasan, Andersen, Asogun, Ayodeji, Badiane, Barnes, Bauer, Bell-Kareem, Benard, Benevolence, Blessing, Boehm, Boisen, Bond, Branco, Butts, Carter, Colubri, Deme, DeRuff, Diédhiou, Edamhande, Elhamoumi, Engel, Eromon, Fallah, Folarin, Fry, Garry, Gaye, Gbakie, Gevao, Gionet, Gladden-Young, Goba, Gomis, Happi, Houghton, Ihekwuazu, Iruolagbe, Jackson, Jalloh, Johnson, Kanneh, Kayode, Kemball, Kingsley, Koroma, Kotliar, Mehta, Metsky, Michael, Mirhashemi, Modjarrad, Momoh, Myhrvold, Naregose, Ndiaye, Ndiaye, Ndiaye, Normandin, Odia, Oguzie, Okogbenin, Okokhere, Okolie, Olawoye, Olumade, Oluniyi, Omoregie, Park, Paye, Petros, Philippakis, Priscilla, Ricks, Rimoin, Sandi, Schieffelin, Schreiber, Seck, Siddiqui, Siddle, Smither, Sy, Sy, Tomkins-Tinch, Tomori, Ugwu, Uwanibe, Uyigue, Victoria, Vinzé, Vodzak, Welch, Wurie, Zoumarou, Grant, Ndiaye, MacInnis, Sabeti and Happi2021; Gardy & Loman Reference Gardy and Loman2018; Vasilakis et al. Reference Vasilakis, Tesh, Popov, Widen, Wood, Forrester, Gonzalez, Saluzzo, Alkhovsky, Lam, Mackenzie and Walker2019; WHO 2022).
Conclusions
Determination of the spatial and temporal distribution of emerging and re-emerging arboviruses of public health interest has been a challenge historically. The biological characteristics of arboviruses and their intrinsic ability to infect different vector and host species leading to dramatic changes in their transmission cycles have forced the health systems to establish a multidisciplinary approach to prevent and mitigate their impact in animal and human health. The mNGS approach has demonstrated its robustness for arbovirus discovery and monitoring in a wide spectrum of biological samples and ecological settings. Its integration to the recently proposed global genomic surveillance strategy with a holistic One Health approach will warrant the early-warning, preparedness and public health response to future biological threats.
Acknowledgements
The authors want to thank the vector-borne diseases intervention group (Eric Perdomo, Adalberto Duica, Juan Domínguez and Juan Osorio) at the health secretary of the Santa Marta district, Colombia for their assistance in the fieldwork and community activities of the research project from which this review is derived.
Financial support
This work was supported by MINCIENCIAS (grant number 210477757671 to JAU-C and DP-C).
Conflicts of interest
The authors declare none.
Ethical statement
None.