Introduction
The International Society for Seed Science's J. Derek Bewley Career Lecture is a retrospective presentation by a ‘late-career seed scientist’ on their area of expertise and career aimed at providing early-career seed scientists with an insight into lessons learned and implications for the future to support their development as researchers, teachers, technologists and communicators. Care is required with such retrospection, however. As Hartley (Reference Hartley1953) wrote ‘The past is a foreign country; they do things differently there.’
In this lecture, I follow in the large reputational footsteps of Derek Bewley and Kent Bradford whose careers were largely forged in Canada and the USA, respectively. Mine developed in the UK. The inevitable but simple advice on career development to ‘think hard, think clever’ and then to ‘work hard, work clever’ is relevant, but insufficient. Careers do not develop in a vacuum. Science is global, but the environment for career development is influenced strongly by local and national factors – and people.
I organized the 1-week-long Fifth International Workshop on Seeds at the University of Reading in 1995, the last time this event, which predated the founding of the ISSS, was held in the UK. That conference was attended by some 350 seed scientists from all over the world, with proceedings that ran to almost 1000 pages with 90 research papers (Ellis et al., Reference Ellis, Black, Murdoch and Hong1997). The 13th Triennial Conference of the International Seed Science Society was to have been held in 2020 at Brighton, UK, but due to COVID-19 the meeting was delayed to 2021 and held virtually. To extend the somewhat retrospective brief for this career lecture, I also apply the theme of my title to consider temporal aspects of the environmental regulation of seed quality development. The environment has long been known to be important during seed production but is now much more so (and will become yet more important) with the challenge of climate change (IPCC, 2014).
Mentors and colleagues
Ambition, ability, inspiration, foresight, hard work and opportunity are key to success, but mentoring and teamwork are also important. The 1995 Workshop at the University of Reading recognized the career and contributions to seed science, genetic resources conservation and crop improvement globally of Eric Roberts OBE (https://seedscisoc.org/obituary-prof-eric-roberts/). Eric was my PhD supervisor, but much more: a great mentor and humourist from whose exemplary conduct I learnt a great deal. I have begun at this point to highlight the importance of people – the social and especially inter-generational interactions in pure and applied science and in tertiary education that are vital to exchange knowledge and to its generation and understanding (including testing for integrity and robustness and recognizing what is not yet understood). Throughout my career, I have been most fortunate in the network of those who have mentored me, my peers and just as important my alumni (now my peers, whom in turn I hope I mentored well).
I embarked on a PhD studentship in seed science and technology due to Eric Robert's infectious enthusiasm and support for the topic. We developed an immediate rapport during my interview, a first meeting which entailed mutual, almost competitive, use of a whiteboard (yes, involving many drawings) long into the evening. Beyond the core science, his artistic inspiration is evident in his reflective end-of-career paper on his ‘search for pattern and form’ (Roberts, Reference Roberts1999). His influence is evident in my career and this paper.
My second mentor was Stan Matthews who appointed me to my first post-doctoral position at the University of Stirling. Stan also combined good humour with a strong intellect and an ability to work hard alongside a strong family ethos. I also gained from him a much clearer understanding of the role science can play to support the seed industry and growers, and that industry can be strong partners in research, not merely recipients of knowledge from research through dissemination.
The first advice, therefore, is to choose your mentor(s) well (or be lucky). Liénard et al. (Reference Liénard, Achakulvisut, Acuna and David2018) highlight the value of mentorship to success in academia. They concluded that:
(a) successful mentors tend to train successful students;
(b) post-doctoral mentors are more instrumental to trainees’ success than doctoral mentors;
(c) progress is better when there is an intellectual synthesis between doctoral and post-doctoral mentors and
(d) combining mentors with disparate expertise is better, but only if trainees can integrate contrasting expertise within their subsequent research.
Supportive colleagues are also vital in science. Roger Smith OBE (initially at Reading, later at RBG Kew, Wakehurst Place, where he founded and led the successful Millennium Seed Bank Project) is one to whom I am especially grateful, not only for his intellect and wise, instructive counsel (combining logic, loquaciousness and appropriate cynicism of the world at large), but also for designing and realizing the University of Reading's Seed Science Laboratory. Similarly, I thank Alistair Murdoch for his many insights, not least prudence and for probing my part-formed ideas, and our work together for many decades across the ‘crops-weeds boundary’; and Tran Dong Hong for our considerable long-term professional collaboration, evident from our many publications together, and for sharing his comprehensive insights into agriculture and crop improvement in SouthEast Asia, not least rice (Oryza sativa L.). I am also indebted to my alumni, not just for the partnerships evident from our publications, but also for the wealth of different insights provided to me from many diverse cultures across the world.
Hence, choose your teams well. The media downplays the role of teams in research, with individuals lauded for their achievements in science; but field and laboratory experimental science is a team game. Moreover, it is possible (though demanding) to be a member of several teams simultaneously, which can be highly advantageous if research funding is capricious (as it is in many countries, including the UK).
The corollary of the above is to support others in turn. I have gained much pleasure and satisfaction in my leadership roles at the University of Reading (as an academic teaching, supervising and tutoring student, and more widely as Head of the School of Agriculture, Policy and Development and then Dean of the Faculty of Life Sciences) from the successes of those I have supported and worked with.
Pure and applied research
Much debate in UK research circles concerns the balance, especially funding, between pure (‘blue skies’) research and applied research (aimed at solving specific problems). There are two long-standing UK principles on research funding. First, decisions about the allocation of funds to research topics are better made by researchers, not politicians (the so-called Haldane principle). Second, social good (determined by government for the research it funds) and commercial opportunity (determined by industry for its research funds) are more important than researchers’ freedom in deciding research direction. These principles are not mutually exclusive: the former relates more to blue skies research; the latter more to applied research. They can become muddled, however, if industry seeks to influence government research spending, or government seeks to coerce industry to fund national strategies.
Opening the Fourth International Workshop on Seeds, Karssen (Reference Karssen, Côme and Corbineau1993) pointed out two main categories of seed scientists: those primarily interested in plant physiology, biochemistry and/or molecular biology for whom seeds are simply a convenient material for their research, and those who wish to learn more about seeds per se. Roberts (Reference Roberts, Ellis, Black, Murdoch and Hong1997), opening the Fifth International Workshop on Seeds, pointed out that the latter group could be further divided into pure and applied seed researchers. My interests tend towards the applied, but I concur with the view that the separation of basic and applied science is synthetic – a judgement of funders as much as scientists: new commercial products and procedures can emanate from ‘blue skies’ research, whilst applied research may reveal considerable new knowledge and understanding. My advice to early-career seed researchers is to cross and recross the apparent divide between pure and applied research whenever feasible.
Role of seed science, seed technology and seed ecology
Seed science and technology underpin agriculture, horticulture, forestry and conservation (both ex situ and in situ). The UK's National Institute of Agricultural Botany (NIAB) was founded in 1919 with the motto ‘Better Seeds: Better Crops’ and the International Seed Testing Association (ISTA) in 1924, following Nobbe's Handbook of Seed Science (Nobbe, Reference Nobbe1876). Thus, this scientific basis for our modern agriculture, horticulture and forestry was established a century ago. Yet, the need for understanding and expertise in seed science and technology is greater than ever before. The global human population has trebled from 2.6 to 7.8 billion to date during my lifetime and is expected to reach 9.7 billion in 2050 and 10.9 billion in 2100 (United Nations, Department of Economic and Social Affairs, Population Division, 2019). The percentage increase in food demand by 2050 will exceed that for population growth. Moreover, the seed industry is global but there remain large gaps where, for example, farmers in Africa have limited access to high-quality seed and more than 80% of all seed is obtained through informal seed systems (Kamga et al., Reference Kamga, Afari-Sefa, Tenkouano, Fleissner and Ndoye2016). At the same time, however, plant conservation is a considerable challenge to maintain global biodiversity (Sharrock, Reference Sharrock2020), with ex situ and in situ crop and wild plant conservation vital to safeguard the future. Seed science, seed technology and seed ecology are vital complementary disciplines to apply to meet these challenges.
Agriculture and horticulture face a loss of productive land for crop production due to climate change, soil erosion, salinity, inundation (flooding and sea-level rise), pollution, deforestation, urbanization and mining. Beyond crop production, not only is biodiversity susceptible to damage from the above but also the greater demand for agricultural products (food, feed, fuel, fibre and other raw products for industries as diverse as construction and pharmaceuticals) has damaged this biodiversity through loss or modification of habitats – with (beyond ethical and aesthetic considerations) negative consequences for agriculture potentially. The market for the seed of wild species to support the restoration of habitats for conservation has burgeoned during my career. In the UK, for example, the recreation of species-rich pastures from intensively managed grassland has relied upon reintroduced seed (Walker et al., Reference Walker, Stevens, Stevens, Mountford, Manchester and Pywell2004). The soil seed bank can also provide a local, free source of seeds for regeneration. Seeds can remain dormant and viable for years, sometimes many decades, beneath the soil surface as studies such as Beal's classic long-term seed burial investigation (Telewski and Zeevaart, Reference Telewski and Zeevaart2002) have shown. Regeneration by seed dispersal is also possible. Two sites in arable cultivation for centuries previously at Rothamsted, UK, were fenced off in the 1880s and reverted naturally to woodland dominated by seed-propagated species: by ash (Fraxinus excelsior L.), sycamore (Acer pseudoplatanus L.) and hawthorn (Craetagus monogyna Jacq.) on a neutral soil; and oak (Quercus robur L.) on an acidic one (Rothamsted Research, 2015). Excluding grazing animals by fencing is a simple tool in woodland regeneration. If, however, the demand is for high-quality timber, or the area is isolated from another woodland, then the seed technology approach (certified seed from registered stands to produce tree seedlings in nurseries for transplanting) is more appropriate. In other words, seed ecology and seed technology both provide potential routes to applying seed science to solve real-world problems.
Much of my research has concerned ex situ plant genetic resources conservation by long-term seed storage, with dissemination of the research to support genebank management (e.g. Cromarty et al., Reference Cromarty, Ellis and Roberts1982; Ellis et al., Reference Ellis, Hong and Roberts1985a,Reference Ellis, Hong and Robertsb; Hong et al., Reference Hong, Linington and Ellis1996). The genebanks of the CGIAR (Consultative Group on International Agricultural Research) have succeeded in maintaining accessions of (orthodox) crop seeds at high viability in cool, dry storage over several decades now (Ellis et al., Reference Ellis, Nasehzadeh, Hanson and Woldemariam2018, Reference Ellis, Nasehzadeh, Hanson, Ndiwa and Woldemariam2019; Hay et al., Reference Hay, Whitehouse, Ellis, Sackville Hamilton, Lusty, Ndjiondjop, Tia, Wenzl, Santos, Yazbek, Azevedo, Peerzada, Abberton, Oyatomi, Guzman, Capilit, Muchugi and Kinyanjui2021). Nonetheless, genebanks have faced many constraints (Hay et al., Reference Hay, Whitehouse, Ellis, Sackville Hamilton, Lusty, Ndjiondjop, Tia, Wenzl, Santos, Yazbek, Azevedo, Peerzada, Abberton, Oyatomi, Guzman, Capilit, Muchugi and Kinyanjui2021). One is a heavy workload of accession regeneration because of their poor quality when received. The profound effect of the production environment on seed quality is considered later.
Illustrating understanding and ideas
I learnt early in my career that a Rőtring pen, black ink, graph and tracing papers, a ruler, French curves and a scalpel blade (vital to eliminate my many mistakes) were important tools that provided me with an additional dimension to not only express myself but to also think and explore ideas. Table 1 lists figures selected from my research that illustrate how they can achieve several different aims:
1. Summarize results, perhaps because the number of observations is too large to tabulate (and in effect archive data).
2. Show the precise relation, whether or not linear, between the results and an independent variable and how this is quantified.
3. Provide greater context to, and understanding of, the results. One example would be where there are two or more independent variables; a figure can show if the effects are additive or interact. A second example would be where effects vary with the range of the independent variable (with perhaps critical, minimum, maximum or ceiling values identified).
Table 1. A list of published figures illustrating different aims in research and research communication (see text for further details)
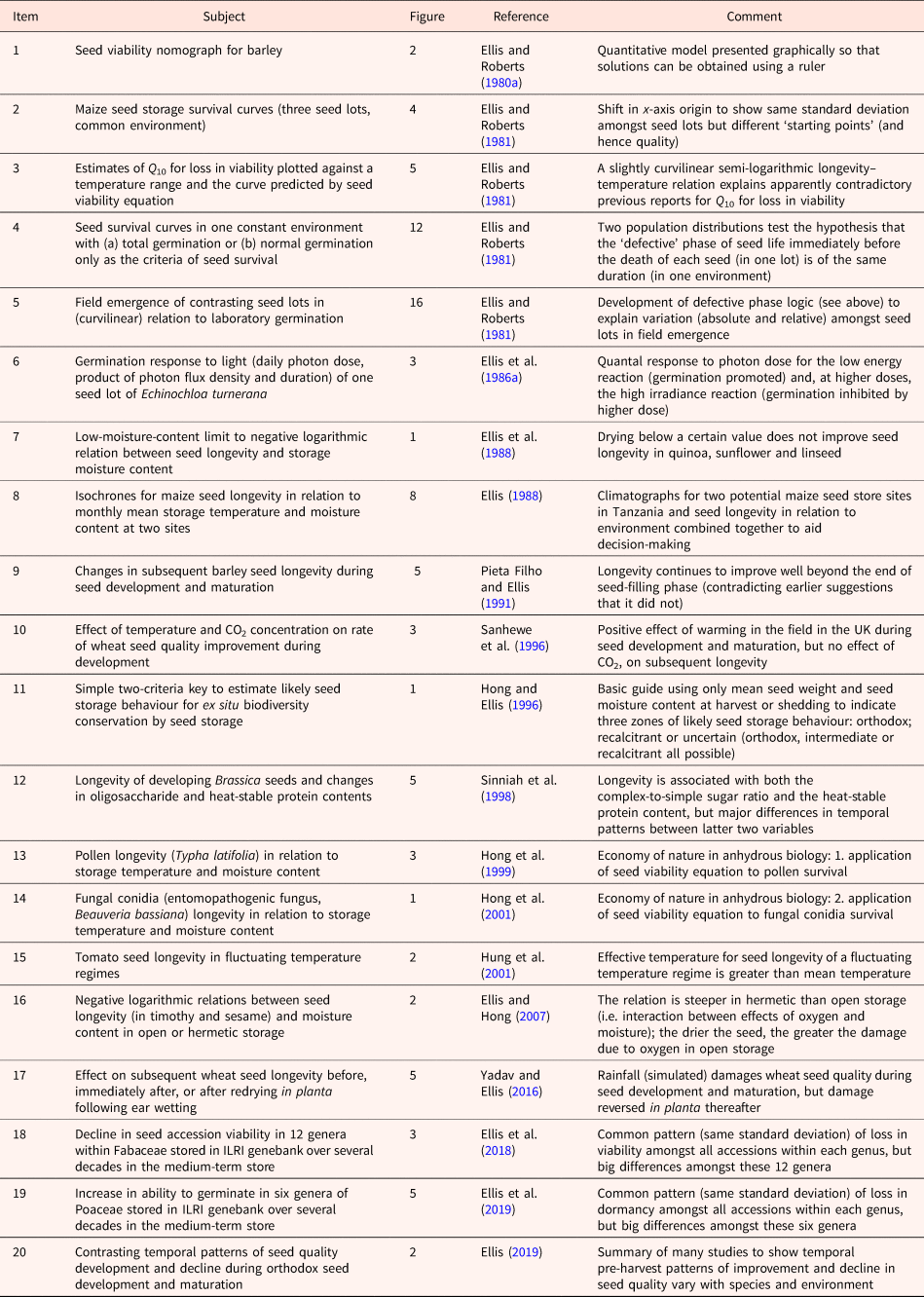
In addition, diagrammatic figures can be applied to achieve the following:
4. Provide tools for practitioners to apply, particularly where users require quantitative information.
5. Develop, explain and validate or counter hypotheses.
6. Summarize complex discussions and indicate future research directions.
The use of figures to present large amounts of data (point 1 above) has grown in importance with the automation of laboratory analytical procedures and environmental recording. For example, meta-analytic data have been presented in more than 200 different styles of graphs and graph variants (Kossmeier et al., Reference Kossmeier, Tran and Voracek2020). In addition to data visualization, however, much more can be achieved with figures beyond presenting results (points 2–6 above). The aim of Table 1 is to encourage the creativity of early-career seed scientists to communicate visually in figures as well as in writing. The list is self-explanatory, but examples are provided below of the links with the points above beginning with two examples that provide research information to practitioners.
The seed viability nomograph for barley (Hordeum vulgare L.), Item 1 in Table 1, is an example of point 4 above. It quantifies the relationship between barley seed survival (initial and final viability), the environment (seed storage temperature and moisture content) and the duration of hermetic storage in a format in which it is possible for users to solve for any one variable (or provide matching combinations of two variables, such as the many different combinations of temperature and moisture content that would provide a common period of longevity) using only a ruler. Its development and publication preceded the era of the personal computer and the World Wide Web. Nonetheless, the ‘low-tech’ nomograph retains some useful features, for example, to hint at likely errors in estimating longevity: using the nomograph and moving a ruler only very slightly from desired values on the temperature and/or moisture content axes has a big effect on the estimate of longevity. This emphasizes a consequence of the exponential relation of longevity with each variable: a small error, or variation, in either component of the environment can affect the estimate of longevity considerably.
Item 8 in Table 1 was an attempt to answer an explicit question from a seed company, stimulated by the seed viability nomograph: which of two potential new seed store sites (in Tanzania) would provide the most suitable ambient environment for the short- to medium-term storage of seed lots of maize (Zea mays L.)? In response, I combined long-term weather data at each site and, assuming rapid equilibrium of seed moisture and temperature with ambient relative humidity and temperature, plotted each site month by month in the annual cycle superimposed on isochrones (different periods of longevity) (Ellis, Reference Ellis1988). One site was far better for longevity. The company decided to build the new store at the harsher seed storage site, however, because other factors (particularly logistical and economic) were more important commercially. Nonetheless, and in response to the climatograph, alterations were made to reduce both seed storage moisture and temperature: the building design was altered to slow warming from solar gain; seed drying and packaging were instituted upon receipt of seed.
The benefit from drying (orthodox) seeds to improve subsequent seed survival was known to our ancestors (an example of technology preceding science) and provides a suitable topic to illuminate several further points about the use of illustration in the progression of research. The negative logarithmic relation between seed longevity and seed moisture content in hermetic storage (Ellis and Roberts, Reference Ellis and Roberts1980a,Reference Ellis and Robertsb; Ellis et al., Reference Ellis, Hong and Roberts1986b), points 1 and 2 above, shows that the relative benefit to longevity from a 1% seed moisture content reduction is greater the lower the moisture content. This relation invoked the obvious question ‘how low can you go?’ and a series of linked hypotheses, points 3 and 5 above: is there a low-moisture-content limit to that negative logarithmic relation; if so, what is its value; and what is the consequence of crossing this limit?
There is a low-moisture-content limit to the negative logarithmic relation between seed longevity and seed moisture content in hermetic storage below which further drying generally leads to no further change in longevity but is not damaging (Ellis et al., Reference Ellis, Hong and Roberts1988, Reference Ellis, Hong and Roberts1989, Reference Ellis, Hong, Roberts and Tao1990), points 2, 3 and 5 above. That begged a further question (point 5 above): is the low-moisture-content limit affected by the storage temperature? The answer, requiring long-term research, was yes: the cooler the storage temperature, the slightly higher the low-moisture-content limit (Ellis and Hong, Reference Ellis and Hong2006).
The negative logarithmic relationship between seed longevity and moisture content also provided a successful experimental and analytical approach to study the effect of the gaseous environment on seed longevity – and a further example of points 2, 3 and 5 above. Item 16 in Table 1 highlights a dramatic interaction between the effects of oxygen and moisture content on seed storage longevity, in which longevity in hermetic storage is increased by drying far more than it is in open storage, and highlights one of the reasons for hermetic storage of dry seed.
But what about the quality of seed lots or seed accessions when they enter a store? It has long been debated when seeds first attain maximum quality in planta during their development and maturation and when seed quality begins to be damaged if harvest is delayed. A series of stylized figures (Item 20, Table 1) compared contrasting temporal patterns of results for changes in seed quality (assessed by seed survival in subsequent air-dry storage, a sensitive indicator for this purpose) during their development and maturation detected with hypothesized patterns (points 5 and 6 above). These temporal patterns can be altered by the effects of genotype, environment and their interaction (Ellis, Reference Ellis2019).
Effect of environment during seed development and maturation on subsequent seed quality
The weather in some years is better for good-quality seed production than others. Austen (Reference Austen and Roberts1972) noted that ‘hot, dry conditions before and at harvest give good seed’ in the UK, whilst some regions of the world produce better-quality seed than others consistently. Climate change (IPCC, 2014, Reference Shukla, Skea, Calvo Buendia, Masson-Delmotte, Pörtner, Roberts, Zhai, Slade, Connors, van Diemen, Ferrat, Haughey, Luz, Neogi, Pathak, Petzold, Portugal Pereira, Vyas, Huntley, Kissick, Belkacemi and Malley2019), therefore, has the potential to affect high-quality seed production. A difference in seed production temperature regime may alter both seed quality and when maximum seed quality is attained during seed development and maturation (Ellis et al., Reference Ellis, Hong and Jackson1993). How can we anticipate such effects and adapt seed production and harvesting in the current as well as future environments?
Historically, there was a tendency to describe the effect of environment on seed quality development as if it were a constant throughout. A positive effect of mean temperature, calculated throughout seed development and maturation, was shown in the UK on the relation between the subsequent air-dry seed longevity in wheat (Triticum aestivum L.) and the duration from anthesis (Item 10, Table 1). This implies that warmer temperatures throughout are always beneficial to seed quality. In addition to the obvious point that such a response would be limited to a specific temperature range, evidence is emerging to suggest that the effect of temperature, and perhaps moisture status, is not consistent from anthesis to harvest maturity.
Figure 1 synthesizes conclusions from several studies of the effect of environment at different stages of seed development and maturation on seed quality in wheat and rice. This summary shows that the effect of seed production environment in planta on subsequent seed quality can vary temporally, principally between early and late seed development and maturation. At the beginning of the seed-filling phase in wheat, cooler temperatures result in better subsequent seed quality and warmer temperatures poorer quality; whereas in late-maturation drying, warmer temperatures provide better subsequent seed quality and cooler temperatures poorer. In rice also, high temperatures during seed development provide poorer subsequent seed quality, whereas high temperatures during seed maturation result in better seed quality. Cool temperatures during early seed development in rice are also problematic. This is not a contradiction: it implies a comparatively narrow range of temperatures is required at this vulnerable developmental stage for subsequent good seed quality.
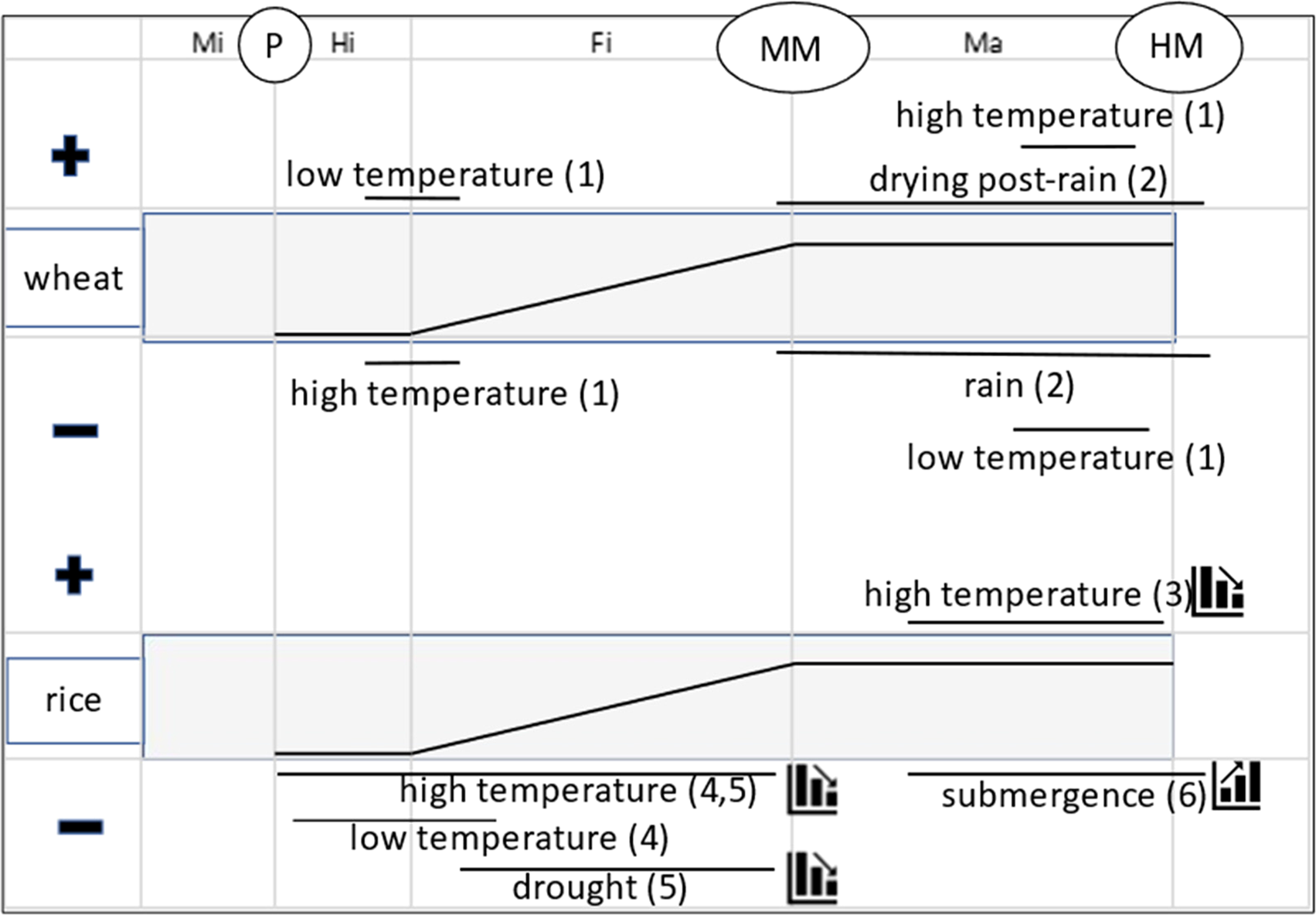
Fig. 1. Summary of effect of extreme environments imposed at different phases (Mi = microsporogenesis; Hi = histodifferentiation; Fi = seed filling; Ma = maturation drying) and stages (P = pollination; MM = mass maturity; HM = harvest maturity) of seed development and maturation in rice (Oryza sativa L.) and wheat (Triticum aestivum L.) on subsequent seed quality (+ benefit; − detrimental), principally seed storage longevity at harvest maturity. The central broken-stick relations provide a stylized representation of changes in seed dry weight during these phases. The solid horizontal lines represent approximately when the effects were detected. The step symbols (for rice only) indicate whether the effect increases or diminishes over time (e.g. steps down for a negative effect means damage to subsequent quality is less the later it is imposed). Sources: (1) Nasehzadeh and Ellis (Reference Nasehzadeh and Ellis2017); (2) Ellis and Yadav (Reference Ellis and Yadav2016), Yadav and Ellis (Reference Yadav and Ellis2016); (3) Whitehouse et al. (Reference Whitehouse, Hay and Ellis2015, Reference Whitehouse, Hay and Ellis2017, Reference Whitehouse, Hay and Ellis2018); (4) Martínez-Eixarch and Ellis (Reference Martínez-Eixarch and Ellis2015); (5) Abdul Rahman and Ellis (Reference Abdul Rahman and Ellis2019) and (6) Tejakhod and Ellis (Reference Tejakhod and Ellis2018). Low and high are relative terms: see original papers for temperature ranges.
Figure 1 identifies causes and outcomes, not underlying mechanisms. Several different regulatory pathways underlie the acquisition of seed longevity in this period (Leprince et al., Reference Leprince, Pellizzaro, Berriri and Buitink2017); Item 12 in Table 1 provides an example of a major difference during seed development and maturation in the temporal patterns of accumulation between soluble carbohydrates and heat-stable proteins, both associated positively with seed longevity. Given that different biosynthetic pathways show peak activity at different times, it is not surprising that the response of seed quality development to environment contrasts amongst different phases of seed development and maturation (Fig. 1).
This summary, an example of point 6 above, indicates that understanding and adapting to climate change (IPCC, 2014, Reference Shukla, Skea, Calvo Buendia, Masson-Delmotte, Pörtner, Roberts, Zhai, Slade, Connors, van Diemen, Ferrat, Haughey, Luz, Neogi, Pathak, Petzold, Portugal Pereira, Vyas, Huntley, Kissick, Belkacemi and Malley2019) in the diverse regions in which crop and forest tree seeds are produced globally will need to consider environmental conditions during several different phases during reproductive development. Extreme temperatures at vulnerable stages of plant development damage pollen (e.g. Coast et al., Reference Coast, Murdoch, Ellis, Hay and Jagadish2016) and reduce seed set (e.g. Wheeler et al., Reference Wheeler, Hong, Ellis, Batts, Morison and Hadley1996), and hence seed number per plant. But it is important to further recognize that the timing of extreme temperature events (and other stresses) can also affect subsequent seed quality (Fig. 1). Hence, climate change has the potential to alter the regions best suited to future crop and forest tree seed production and when and how to harvest sufficient volumes of high-quality seed; in wild plant species, the success of natural regeneration by seed may be affected.
Concluding remarks
Two related transformations have long fascinated me and provided a focus for my research: (a) from the dry, viable but quiescent, seed (of orthodox species) to an autotrophic seedling showing vigorous growth; and (b) from the immature, recently fertilized, seed growing and maturing on the mother plant to an independent, dispersed, dry plant propagule (again, viable but quiescent) able to survive considerable spatial and temporal distribution. Seeds are not the only plant propagule or organ capable of withstanding desiccation when dispersed and whose survival benefits from this (e.g. Items 13 and 14, Table 1). Much remains to be learnt about anhydrous biology, and then applied. The nexus between human population growth, loss in biological diversity and rapid environmental change is a major challenge for all in the 21st Century. Research in seed science will be vital in meeting ensuing complex challenges, but only if it can be, and then is, applied.
Acknowledgements
I thank all those I have worked and networked with and all those who have supported me during my career.
Financial support
The author is employed by the University of Reading.
Conflicts of interest
The author declares none.