Diet plays an important role in the aetiology and prevention of the risk factors of the metabolic syndrome(Reference O'Keefe, Gheewala and O'Keefe1). Many epidemiological studies have shown a strong inverse association between an increased consumption of fruits and vegetables and a decreased incidence of CVD(Reference Bazzano, He and Ogden2–Reference Appel, Moore and Obarzanek5), especially stroke(Reference Joshipura, Ascherio and Manson3), IHD(Reference Bazzano, He and Ogden2, Reference Joshipura, Ascherio and Manson3), CHD(Reference Joshipura, Hu and Manson4) and blood pressure(Reference Appel, Moore and Obarzanek5). Although the risk of type 2 diabetes is not related to the consumption of fruit or vegetables, the intake of antioxidant phytochemicals has been associated with reduction in the risk of type 2 diabetes(Reference Hamer and Chida6, Reference Dembinska-Kiec, Mykkanen and Kiec-Wilk7). Close relationships between obesity, the metabolic syndrome and the development of non-alcoholic fatty liver disease (NAFLD) have been described, with most NAFLD patients displaying multiple components of the metabolic syndrome(Reference Marchesini, Brizi and Bianchi8). However, the effects of increased intakes of fruit and vegetables on NAFLD patients have not been studied.
Glucosinolates, flavonoids, tannins, carotenoids, phytates and phyto-oestrogens represent the major classes of phytochemicals present in fruits and vegetables(Reference van Dokkum, Frølich and Saltmarsh9). These nutrients may improve health through many mechanisms, such as reducing oxidative stress and inflammation, improving lipid profiles, lowering blood pressure and enhancing glucose metabolism(Reference van Dokkum, Frølich and Saltmarsh9). Anthocyanins, a subclass of flavonoids, are pigments of red fruits such as cherries, plums, strawberries, raspberries, blackberries, grapes, red and black currants and, together with the phenolic acids, are the major phytochemicals in the human diet(Reference Scalbert and Williamson10). Although research on the therapeutic uses of the phytochemicals in carrots started with the demonstration of pro-vitamin A activity by Moore in 1929(Reference Moore11), few data exist on possible therapeutic benefits from carrot varieties other than orange carrots where β-carotene is the predominant phytochemical component.
Carrot varieties differ in colour from white to orange, yellow, red and purple and contain different combinations and quantities of macronutrients, fibre, vitamins, minerals and phytochemicals including carotenoids, phenolic acids, anthocyanins, isocoumarins, terpenes and sesquiterpenes(Reference Metzger, Barnes and Reed12, Reference Sun, Simon and Tanumihardjo13). The colour of orange carrots is due to higher concentrations of α- and β-carotene; red carrots contain increased lycopene and yellow carrots contain increased lutein concentrations while white carrots have lower concentrations of all carotenoids(Reference Sun, Simon and Tanumihardjo13). The anthocyanidins and chlorogenic acid are the major antioxidants in purple carrots(Reference Sun, Simon and Tanumihardjo13, Reference Grassmann, Schnitzler and Habegger14). The colour of purple carrots (Daucus carota L. ssp. sativus var. atrorubens Alef.) comes from the presence of anthocyanins such as cyanidin-3-(2″-xylose-6″-sinapoyl-glucose-galactoside)(Reference Sun, Simon and Tanumihardjo13, Reference Charron, Kurilich and Clevidence15). Additionally, at least forty phenolic acids are present in purple carrots including chlorogenic and caffeic acids as the predominant compounds(Reference Sun, Simon and Tanumihardjo13). Purple carrots also contain polyacetylene compounds such as falcarindiol, falcarindiol 3-acetate and falcarinol(Reference Christensen and Kreutzmann16). Purple carrots remain a sparsely studied potential dietary component that are rich in anthocyanins, phenolic acids and carotenoids(Reference Sun, Simon and Tanumihardjo13).
β-Carotene, the best known of the large carotenoid family of phytochemicals, is the major dietary source of vitamin A (retinol). Epidemiological studies have inversely associated circulating β-carotene concentrations with the risk of hypertension(Reference Hozawa, Jacobs and Steffes17, Reference Yanagisawa, Suzuki and Kimura18) but not with type 2 diabetes(Reference Liu, Ajani and Chae19, Reference Kataja-Tuomola, Sundell and Mannisto20). The antioxidant as well as pro-oxidant actions of β-carotene are widely known(Reference Paiva and Russell21, Reference El-Agamey, Lowe and McGarvey22). Carotenoids, particularly β-carotene and lycopene, protect animals and humans against oxidative processes, especially in lipophilic environments such as cell membranes by acting as free radical scavengers(Reference Tapiero, Townsend and Tew23). Carotenoids have been proposed as a dietary strategy to prevent cancers, possibly by antioxidant actions and the stimulation of intercellular communication via gap junctions(Reference Tapiero, Townsend and Tew23). In vivo, β-carotene prevented ethanol-induced liver damage and improved hepatic antioxidant enzyme status in rats(Reference Lin, Huang and Lin24). In streptozotocin-induced diabetic rats, β-carotene treatment increased hepatic and cardiac antioxidant enzymes(Reference Maritim, Dene and Sanders25), improved circulatory insulin and glucose concentrations(Reference Furusho, Kataoka and Yasuhara26) but increased lipid peroxidation(Reference Maritim, Dene and Sanders25).
Epidemiological studies have shown that plant-based diets protect against CVD and cancer; flavonoids, including the anthocyanins, occur widely in plants and may improve human health(Reference Kris-Etherton, Hecker and Bonanome27, Reference Galvano, La Fauci and Lazzarino28). In particular, the anthocyanins may improve the symptoms of the metabolic syndrome such as dyslipidaemia, impaired glucose tolerance and hypertension(Reference Kris-Etherton, Hecker and Bonanome27, Reference Galvano, La Fauci and Lazzarino28). Anthocyanin-rich plant extracts lowered plasma lipid concentrations in rat models of hyperlipidaemia(Reference Cignarella, Nastasi and Cavalli29, Reference Valcheva-Kuzmanova, Kuzmanov and Mihova30) and an extract from black rice (Oryza sativa L. indica) lowered plasma lipid concentrations and improved insulin resistance in fructose-fed rats(Reference Guo, Ling and Wang31). Spontaneously hypertensive rats fed on anthocyanin-rich purple maize, purple sweet potato and red radish showed reduced blood pressure and heart rate(Reference Shindo, Kasai and Abe32). Other constituents of carrots such as the polyacetylenes may suppress inflammation in the metabolic syndrome by decreasing lipopolysaccharide-induced expression of inflammatory proteins in macrophages and endothelial cells(Reference Metzger, Barnes and Reed12).
Although the phytochemical profile of purple carrots has been reported, sparse information exists on the pharmacological activity of purple carrot juice. The aims of the present study were to determine whether purple carrot juice attenuates or reverses the cardiovascular, liver and metabolic changes produced in a high-carbohydrate, high-fat diet-fed rat model of the metabolic syndrome and whether these responses are comparable with that of β-carotene.
Diets with increased carbohydrates, mainly as fructose(Reference Stanhope and Havel33), increased fats(Reference Buettner, Scholmerich and Bollheimer34) or a combination of both(Reference Poudyal, Campbell and Brown35) in rats have been studied to mimic the human metabolic syndrome. Maize starch is a slowly digestible carbohydrate(Reference Zhang and Hamaker36) and served as a control for the high-carbohydrate, high-fat diet in the present study where the primary carbohydrate is fructose. Unlike fructose, maize starch does not increase blood glucose, plasma insulin or NEFA concentrations(Reference Poudyal, Campbell and Brown35, Reference Zhang and Hamaker36).
The present study characterised structural changes in the rat heart by histology and echocardiography, while heart function was assessed in vivo using echocardiography and ex vivo in isolated perfused hearts. Systolic blood pressure was measured and isolated thoracic rings were used to measure vascular reactivity. Biochemical parameters (enzyme activities of alanine transaminase, aspartate transaminase and alkaline phosphatase as well as total plasma bilirubin concentrations) were assessed, and along with histology, were used to define structural and functional changes in the liver. Circulatory markers of impaired metabolism (lactate dehydrogenase, urea and oral glucose tolerance test), oxidative stress (malondialdehyde and uric acid) and inflammation (C-reactive protein; CRP) were measured.
Materials and methods
Purple carrot juice
Purple carrot juice was prepared by SDS Beverages Pty Ltd (Irymple, VIC, Australia). Purple carrots were crushed in a hammer mill with water acidified with citric acid. Following separation of solids, pectinases were added to the juice and mixed at 50–55°C for clarification. The juice was then subjected to pasteurisation, chilling and filtering through a 200 μm mesh before evaporation was carried out to concentrate the juice to 60 brix. This 60 brix purple carrot juice was processed through another 200 μm mesh and a metal analyser and stored at 4°C. This product was added as a 5 % supplement to either the maize starch or high-carbohydrate, high-fat diet described below (Table 1).
Table 1 Diet composition and energy contents of diets
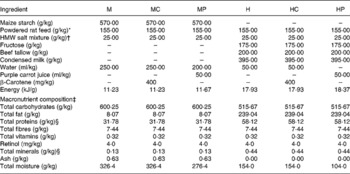
M, maize starch diet; MC, maize starch+β-carotene diet; MP, maize starch+purple carrot juice diet; H, high-carbohydrate, high-fat diet; HC, high-carbohydrate, high-fat+β-carotene diet; HP, high-carbohydrate, high-fat+purple carrot juice diet.
* Meat-free rat and mouse feed (Specialty Feeds) contained (g/kg feed): carbohydrate, 707·07; protein, 194·00; fat, 48·00; fibre, 48·00; total vitamins, 2·08; total minerals, 0·85.
† Hubble, Mendel and Wakeman salt mixture(Reference Hubbell, Mendel and Wakeman54) (MP Biochemicals, Seven Hills, NSW, Australia).
‡ Derived from powdered rat feed.
§ H, HC and HP diets additionally derive these micronutrients from condensed milk.
Total monomeric anthocyanins were determined using the pH differential method as previously described(Reference Lee, Durst and Wrolstad37). Absorbance was measured at 520 and 700 nm. Total monomeric anthocyanins were expressed as cyanidin-3-glucoside (molar extinction coefficient of 26 900 litres/cm per mol and molecular weight of 449·2 g/mol).
Carotenoid concentrations in purple carrot juice were analysed on an Agilent 1100 series HPLC system equipped with a photodiode array UV-visible detector (Agilent Technologies, Waldbronn, Germany). Separations were achieved on a 150 × 3·0 mm internal diameter, 3 μm particle size, analytical scale YMC C30 reversed-phase column (YMC, Wilmington, MA, USA). Elution was performed at a solvent flow rate of 1·0 ml/min and detection at 450 nm. The mobile phases consisted of acetone and Milli-Q water. For routine analysis, a 10–95 % linear gradient elution of acetonitrile and Milli-Q water was pumped through the column at 1·0 ml/min over 30 min. All solvents were HPLC grade and contained 0·005 % trifluoroacetic acid (Sigma-Aldrich Australia, Sydney, NSW, Australia) to enhance peak shape. Purple carrot juice was dissolved in 100 % analytical grade methanol and filtered through a Whatman polytetrafluoroethylene (PTFE) membrane filter (0·45 μm; Whatman PLC, Maidstone, Kent, UK) before loading the sample (10 μl injection volume). The UV spectra of the different carotenoid (all-trans-β-carotene, zeaxanthin and lutein) compounds were recorded with a diode array detector. Carotenoids were quantified using calibration curves of the corresponding standard compounds at the specific absorption maximum.
Rats
The experimental groups consisted of eighty-four male Wistar rats (aged 8–9 weeks; weight 337 ± 5 g) supplied by The University of Queensland Biological Resources unit and individually housed at the School of Biomedical Sciences Animal House Facility. All experimental procedures were approved by the Animal Experimentation Ethics Committee of The University of Queensland under the guidelines of the National Health and Medical Research Council of Australia. All experimental groups were housed in a temperature-controlled, 12 h light–dark cycle environment with ad libitum access to water and the group-specific rat diet. Daily body weight, feed and water measurements were taken to monitor the day-to-day health of the rats. The rats were randomly divided into six separate groups based on their diet: maize starch (n 12); maize starch+β-carotene (n 12); maize starch+purple carrot juice (n 12); high-carbohydrate, high-fat (H; n 24); high-carbohydrate, high-fat+β-carotene (HC; n 12); high-carbohydrate, high-fat+purple carrot juice (HP; n 12). The full description of the diets is given in Table 1. In addition, the drinking water of all high-carbohydrate, high-fat diet-fed rats contained 25 % fructose. Both purple carrot juice and β-carotene (Sigma-Aldrich Australia) were administered for 8 weeks starting 8 weeks after the initiation of the maize starch or high-carbohydrate, high-fat diets. Twelve rats were killed from the high-carbohydrate, high-fat diet group at 8 weeks to assess the pathophysiological state before purple carrot or β-carotene intervention (H 8 weeks). Two rats per group were taken for histological analysis. Two slides were prepared per tissue specimen and two random, non-overlapping fields pictured for analysis. Organs were also collected from rats used for perfusion studies.
Systolic blood pressure was measured under light sedation with intraperitoneal injection of Zoletil (tiletamine 15 mg/kg, zolazepam 15 mg/kg; Virbac, Peakhurst, NSW, Australia). Measurements were taken using an MLT1010 Piezo-Electric Pulse Transducer (ADInstruments, Sydney, NSW, Australia) and inflatable tail-cuff connected to a MLT844 Physiological Pressure Transducer (ADInstruments) and PowerLab data acquisition unit (ADInstruments).
For the oral glucose tolerance test, basal blood glucose concentrations were measured in tail-vein blood using a Medisense Precision Q.I.D glucose meter (Abbott Laboratories, Bedford, MA, USA) after an overnight (10–12 h) feed deprivation. Fructose-supplemented drinking water in H, HC and HP groups was replaced with normal water for the overnight feed-deprivation period for the measurement of basal blood glucose concentrations. The rats were given glucose at 2 g/kg body weight as a 40 % solution via oral administration. Tail-vein blood samples were taken at 0, 30, 60, 90 and 120 min following glucose administration.
Echocardiography
Echocardiographic examination (Philips iE33 system, 12 MHz transducer; Philips Medical System, Andover, MA, USA) was performed at 8 and 16 weeks as previously described(Reference Poudyal, Campbell and Brown35, Reference Brown, Fenning and Chan38). Briefly, rats were anaesthetised using intraperitoneal Zoletil (toletamine 15 mg/kg and zolazepam 15 mg/kg intraperitoneally; Virbac, Peakhurst, NSW, Australia) combined with xylazine (10 mg/kg) (Ilium Xylazil; Troy Laboratories, Sydney, NSW, Australia) and positioned in dorsal recumbency. Electrodes attached to the skin overlying the elbows and right stifle facilitated the simultaneous recording of a lead II electrocardiogram.
A short axis view of the left ventricle at the level of the papillary muscles was obtained and used for direct acquisition of M-mode images of the left ventricle for measurement of diastolic posterior wall thickness, left ventricular internal systolic dimension and left ventricular end-diastolic diameter. For these parameters, diastole was defined by the beginning of the QRS complex on the simultaneously recorded electrocardiogram and systole identified as the nadir of systolic anterior wall motion independent of the electrocardiogram complex.
Pulsed-wave Doppler velocity profiles of mitral inflow was obtained from the left apical four-chamber view for measurement of early (EM) and late (AM) mitral inflow velocity, mitral inflow E-wave deceleration time, and time from mitral valve closure to opening (MCMO). A pulsed-wave Doppler velocity profile of the ascending aorta was obtained from a suprasternal view for measurement of aortic (Ao) velocity, ejection time (ET) and the diameter of the ascending aorta at the point of transition to the transverse aorta.
Derived indices of left ventricular systolic function (fractional shortening (FS%), ejection fraction (EF%)) were calculated using well-established formulae(Reference Brown, Fenning and Chan38). Left ventricular mass was estimated using the standard cube equation as previously described(Reference Poudyal, Campbell and Brown35, Reference Brown, Fenning and Chan38).
Isolated heart preparation
The left ventricular function of the rats in all treatment groups was assessed using the Langendorff heart preparation. Terminal anaesthesia was induced via intraperitoneal injection of pentobarbitone sodium (Lethabarb®, 100 mg/kg). After heparin (Sigma-Aldrich Australia) administration (100 IU) through the right femoral vein, blood (about 5 ml) was taken from the abdominal aorta. Isovolumetric ventricular function was measured by inserting a latex balloon catheter into the left ventricle connected to a Capto SP844 MLT844 physiological pressure transducer and Chart software on a Maclab system (ADInstruments Australia and Pacific Islands, Bella Vista, NSW, Australia). All left ventricular end-diastolic pressure values were measured while pacing the heart at 250 beats per min using an electrical stimulator. End-diastolic pressures were obtained starting from 0 mmHg up to 30 mmHg. The diastolic stiffness constant (κ, dimensionless) was calculated as in previous studies(Reference Poudyal, Campbell and Brown35, Reference Brown, Duce and Miric39).
Organ bath studies
Thoracic aortic rings (4 mm in length) were suspended in an organ bath chamber with a resting tension of 10 mN. Cumulative concentration–response (contraction) curves were measured for noradrenaline (Sigma-Aldrich Australia); concentration–response (relaxation) curves were measured for acetylcholine (Sigma-Aldrich Australia) and sodium nitroprusside (Sigma-Aldrich Australia) in the presence of a submaximal (70 %) contraction to noradrenaline(Reference Poudyal, Campbell and Brown35).
Organ weights
The right and left ventricles were separated after perfusion experiments and weighed. Liver and abdominal fat were removed following heart removal and blotted dry for weighing. Perirenal, epididymal and omental fat were together weighed as abdominal fat. Organ weights were normalised relative to the tibial length at the time of their removal (in mg/mm).
Histology
Immediately after removal, heart and liver tissues were fixed in 10 % buffered formalin for 3 d with change of formalin every day to remove traces of blood from the tissue. The samples were then dehydrated and embedded in paraffin wax. Thin sections (10 μm) of left ventricle and the liver were cut and stained with haematoxylin and eosin stain for determination of inflammatory cell infiltration. Liver sections were also stained with Milligan's Trichrome stain to determine fibrosis. Collagen distribution was measured in the left ventricle with picrosirius red stain. Laser confocal microscopy (Zeiss LSM 510 upright Confocal Microscope)(Reference Poudyal, Campbell and Brown35) with colour intensity quantitatively analysed using NIH-imageJ software (National Institutes of Health, Bethesda, MD, USA) was used to determine the extent of collagen deposition in selected tissue sections.
Plasma analysis
Briefly, blood was centrifuged at 5000 g for 15 min within 30 min after collection into heparinised tubes. Plasma samples were separated and transferred to Eppendorf tubes for analysis. Plasma enzymic activities and analyte concentrations were determined using kits and controls supplied by Olympus using an Olympus analyser (AU 400; Olympus, Tokyo, Japan): cholesterol, Olympus OSR6516 enzymic colour test; TAG, Olympus OSR 6133 enzymic colour test; lactate dehydrogenase, Olympus OSR6127 kinetic UV test; urea, Olympus OSR 6134 kinetic UV test; uric acid, Olympus OSR 6098 enzymic colour test; alanine transaminase, Olympus OSR 6107 kinetic UV test; aspartate transaminase, Olympus OSR 6109 kinetic UV test; alkaline phosphatase, Olympus OSR 6004 knetic UV test; albumin, Olympus OSR 6101 photometric colour test; total bilirubin, Olympus OSR 6111 photometric colour test. Globulin was calculated as total protein-albumin. Plasma malondialdehyde concentrations were measured by HPLC (Shimadzu®) as previously described(Reference Sim, Salonikas and Naidoo40). Plasma CRP was estimated using a commercial kit (Kamiya Biomedical, CA, USA) according to manufacturer-provided standards and protocols using a Cobas-Mira automated analyzer (Roche Diagnostics, Basel, Switzerland).
Statistical analysis
All data are presented as mean values with their standard errors. Results were tested for variance using Bartlett's test and data that were not normally distributed were transformed (using log 10 function) before statistical analyses. Differences between the groups were determined by one-way ANOVA with the Newman–Keuls multiple comparison post test. For non-parametric data (total plasma cholesterol, plasma NEFA, lactate dehydrogenase, malondialdehyde and plasma urea concentrations and feed intake), Kruskal–Wallis tests were performed. All statistical analysis was performed using GraphPad Prism version 5.00 for Windows (San Diego, CA, USA). P < 0·05 was considered as statistically significant.
Results
Diet and supplement intake, body weight and metabolic parameters
Purple carrot juice contained anthocyanins (total 5·53 mg/ml) and traces of β-carotene (0·75 μg/ml), lutein (0·54 μg/ml) and zeaxanthin (4·4 μg/ml). The mean daily intakes of total anthocyanins and carotenoids were higher in the maize starch+purple carrot juice and maize starch+β-carotene groups than in the HP and HC groups, respectively, as the feed intake was higher in all maize starch diet-fed rats (Table 2). However, the carotenoid intakes in the maize starch+purple carrot juice and HP rats were extremely low due to the low carotenoid content of the juice (Table 2).
Table 2 Changes in dietary intakes, body anthropometrics, blood and plasma biochemistry
(Mean values with their standard errors for ten animals per group)

M, maize starch diet; MC, maize starch+β-carotene diet; MP, maize starch+purple carrot juice diet; H, high-carbohydrate, high-fat diet; HC, high-carbohydrate, high-fat+β-carotene diet; HP, high-carbohydrate, high-fat+purple carrot juice diet; AUC, area under the curve; CRP, C-reactive protein.
a–q Mean values within a row with unlike superscript letters were significantly different (P < 0·05).
* Body-weight gain calculated as percentage of body weight increase from 8 weeks to 16 weeks for all groups and week 1 to week 8 for the high-carbohydrate, high-fat diet-fed rats (8 weeks).
The high-carbohydrate, high-fat diet induced an increased body weight, especially with increased abdominal fat pads and abdominal circumference, compared with maize starch diet-fed rats (Table 2). Although high-carbohydrate, high-fat diet feeding reduced feed and water intake, rats on this diet had higher daily energy intake compared with maize starch diet-fed rats (Table 2). Neither purple carrot juice nor β-carotene supplementation altered daily feed, water or energy intake. However, purple carrot juice supplementation reduced percentage gain in body weight, abdominal fat pads and abdominal circumference (Table 2). The high-carbohydrate, high-fat diet produced impaired glucose tolerance, increased plasma concentrations of total cholesterol, TAG, NEFA, malondialdehyde, CRP and uric acid, increased lactate dehydrogenase activity and decreased plasma urea concentration (Table 2). Purple carrot juice supplementation improved oral glucose tolerance (Table 2) and reduced plasma total cholesterol, TAG, NEFA and CRP concentrations (Table 2). Although β-carotene improved oral glucose tolerance and reduced plasma CRP concentrations (Table 2), it further increased plasma TAG and NEFA concentrations (Table 2). Further, purple carrot juice supplementation attenuated the changes in lactate dehydrogenase activity, plasma uric acid, malondialdehyde and urea concentrations in high-carbohydrate, high-fat diet-fed rats; these parameters were unaltered by β-carotene supplementation (Table 2).
Cardiovascular structure and function
High-carbohydrate, high-fat diet-fed rats showed increased left ventricular diastolic internal diameter and relative wall thickness with increased estimated left ventricular mass and wet weight in comparison with the maize starch diet-fed rats (Table 3). Moreover, the high-carbohydrate, high-fat diet reduced fractional shortening and ejection fraction and increased ejection time (Table 3). Both β-carotene and purple carrot juice supplementation reduced estimated left ventricular mass and wet weight. In addition, β-carotene reduced left ventricular diastolic internal diameter, increased fractional shortening and ejection fraction and decreased ejection time (Table 3). High-carbohydrate, high-fat diet-fed rats showed sustained elevation in systolic blood pressure after 4 weeks of diet administration as compared with maize starch diet-fed rats (Table 3). Purple carrot juice as well as β-carotene supplementation normalised blood pressure as early as 4 weeks post-intervention (Table 3). Following high-carbohydrate, high-fat diet feeding for 16 weeks, diminished vascular responses were observed in isolated thoracic aortic rings to noradrenaline, sodium nitroprusside and acetylcholine when compared with maize starch diet-fed rats (Fig. 1). However, this reduced thoracic aortic reactivity was not observed after 8 weeks of high-carbohydrate, high-fat diet feeding. Purple carrot juice and β-carotene supplementation prevented any further decrease in sodium nitroprusside- and acetylcholine-dependent vasorelaxation (Fig. 1(B) and (C)) as compared with high-carbohydrate, high-fat diet-fed rats at 8 weeks but failed to attenuate the reduced contractility with noradrenaline (Fig. 1(A)).
Table 3 Changes in cardiovascular structure and function
(Mean values with their standard errors for ten animals per group)

M, maize starch diet; MC, maize starch+β-carotene diet; MP, maize starch+purple carrot juice diet; H, high-carbohydrate, high-fat diet; HC, high-carbohydrate, high-fat+β-carotene diet; HP, high-carbohydrate, high-fat+purple carrot juice diet; LVIDd, left ventricular internal diameter in diastole; LVIDs, left ventricular internal diameter in systole; LVPWd, left ventricular posterior wall thickness in diastole; EM, early mitral inflow velocity; AM, late mitral inflow velocity; MCMO, mitral closing-mitral opening time.
a–p Mean values within a row with unlike superscript letters were significantly different (P < 0·05).
* Four animals per group.

Fig. 1 Cumulative concentration–response curves for noradrenaline (A), sodium nitroprusside (B) and acetylcholine (C) in thoracic aortic rings from maize starch diet (○), maize starch diet+β-carotene (△), maize starch diet+purple carrot juice (⋄), high-carbohydrate, high-fat diet for 8 weeks (▿), high-carbohydrate, high-fat diet for 16 weeks (□), high-carbohydrate, high-fat diet+β-carotene (●) and high-carbohydrate, high-fat diet+purple carrot juice (▾)-fed rats. Values are means for eight to ten rats per group, with standard errors represented by vertical bars. a,b Mean values with an unlike letter were significantly different (P < 0·05).
Ex vivo cardiac function as measured in the Langendorff isolated heart showed an increased diastolic stiffness (Table 3) in the high-carbohydrate, high-fat diet-fed rats as compared with the maize starch diet-fed group (Table 3). Reduction in diastolic stiffness was only observed in purple carrot juice-supplemented rats. Histological analysis revealed that high-carbohydrate, high-fat diet-fed rats showed increased infiltration by inflammatory cells into the left ventricle (Fig. 2(E)) and increased interstitial collagen deposition and hypertrophy (Fig. 2(I); Table 3) when compared with the maize starch diet-fed rats (Fig. 2(A) and (H); Table 3). Both purple carrot juice- and β-carotene-supplemented high-carbohydrate, high-fat diet-fed rats showed normalised infiltration of inflammatory cells (Fig. 2(G); Fig. 2(F)) and reduced collagen deposition with normalised hypertrophy (Fig. 2(N); Fig. 2(M); Table 3). No major changes were observed in purple carrot juice- and β-carotene-supplemented maize starch diet-fed rats (Fig. 2(B), (C), (I) and (J); Table 3).

Fig. 2 Haematoxylin and eosin staining of left ventricle ( × 20) showing inflammatory cells (marked as ‘in’) as dark spots outside the myocytes in maize starch diet (A), maize starch diet+β-carotene (B), maize starch diet+purple carrot juice (C), high-carbohydrate, high-fat diet for 8 weeks (D), high-carbohydrate, high-fat diet for 16 weeks (E), high-carbohydrate, high-fat diet+β-carotene (F) and high-carbohydrate, high-fat diet+purple carrot juice (G)-fed rats. Picrosirius red staining of left ventricular interstitial collagen deposition (40 × ) in maize starch diet (H), maize starch diet+β-carotene (I), maize starch diet+purple carrot juice (J), high-carbohydrate, high-fat diet for 8 weeks (K), high-carbohydrate, high-fat diet for 16 weeks (L), high-carbohydrate, high-fat diet+β-carotene (M) and high-carbohydrate, high-fat diet+purple carrot juice (N)-fed rats; fibrosis is marked as ‘fi’ and hypertrophied cardiomyocytes are marked as ‘hy’.
Hepatic structure and function
In comparison with maize starch diet-fed rats, high-carbohydrate, high-fat diet-fed rats had elevated plasma alanine transaminase, aspartate transaminase and alkaline phosphatase activities although the albumin:globulin ratio and plasma bilirubin concentrations remained unaffected (Table 4). Purple carrot juice supplementation reversed liver function abnormalities indicated by normalised activity of these enzymes (Table 4). Although β-carotene supplementation normalised transaminases activities in high-carbohydrate, high-fat diet-fed rats, alkaline phosphatase activity remained elevated (Table 4).
Table 4 Changes in hepatic structure and function
(Mean values with their standard errors for ten animals per group)

M, maize starch diet; MC, maize starch+β-carotene diet; MP, maize starch+purple carrot juice diet; H, high-carbohydrate, high-fat diet; HC, high-carbohydrate, high-fat+β-carotene diet; HP, high-carbohydrate, high-fat+purple carrot juice diet; ALT, alanine transaminase; AST, aspartate transaminase; ALP, alkaline phosphatase.
a–k Mean values within a row with unlike superscript letters were significantly different (P < 0·05).
* Four animals per group.
By histological examination, high-carbohydrate, high-fat diet feeding to rats caused macrovesicular steatosis (Fig. 3(E)) resulting from accumulation of lipids in large and small droplets within hepatocytes with markedly high portal inflammation (Fig. 3(M)) and portal fibrosis (Fig. 3(T); Table 4) as compared with the maize starch diet-fed rats (Fig. 3(A), (H) and (P); Table 4). The purple carrot juice-supplemented high-carbohydrate, high-fat diet-fed rats displayed minimal signs of macrovesicular steatosis (Fig. 3(G)), portal inflammation (Fig. 3(O)) or fibrosis (Fig. 3(V); Table 4). The β-carotene-supplemented high-carbohydrate, high-fat diet-fed rats displayed normalised portal inflammation (Fig. 3(N)) and fibrosis (Fig. 3(P); Table 4) but showed obvious hepatocyte ballooning and increased fat vacuole size and number (Fig. 3(F)). No major hepatic changes were observed in purple carrot juice- and β-carotene-supplemented maize starch diet-fed rats (Fig. 3(C), (K) and (R) and 3(B), (J) and (Q); Table 4).

Fig. 3 Haematoxylin and eosin staining of hepatocytes ( × 40) showing hepatocytes with enlarged fat vacuoles (marked as ‘fv’) and inflammatory cells (marked as ‘in’) (20 × ) from maize starch diet (A, H), maize starch diet+β-carotene (B, I), maize starch diet+purple carrot juice (C, J), high-carbohydrate, high-fat diet for 8 weeks (D, K), high-carbohydrate, high-fat diet for 16 weeks (E, L), high-carbohydrate, high-fat diet+β-carotene (F, M) and high-carbohydrate, high-fat diet+purple carrot juice (G, N)-fed rats. Milligan's trichrome staining of the hepatic portal regions showing collagen (marked as ‘pf’) (20 × ) in maize starch diet (O), maize starch diet+β-carotene (P), maize starch diet+purple carrot juice (Q), high-carbohydrate, high-fat diet for 8 weeks (R), high-carbohydrate, high-fat diet for 16 weeks (S), high-carbohydrate, high-fat diet+β-carotene (T) and high-carbohydrate, high-fat diet+purple carrot juice (U)-fed rats. bl, Hepatocyte ballooning.
Discussion
A combination of high carbohydrate and high fat in the diet was used in the present study to mimic human diets that have been associated with the development of the metabolic syndrome. This diet produced changes in metabolic, cardiovascular and hepatic structure and functions such as excessive abdominal fat deposition, impaired glucose tolerance, elevated blood pressure and increased plasma lipid concentrations together with increased plasma oxidative stress markers and liver enzymes compared with maize starch diet-fed rats. Further, histological evaluation of heart and liver showed increased infiltration of inflammatory cells and increased collagen deposition in addition to increased fat vacuoles in the liver. This was accompanied by increased left ventricular stiffness and decreased aortic reactivity.
The present study compares therapeutic responses from anthocyanin-rich purple carrot juice with responses to β-carotene in high-carbohydrate, high-fat diet-fed rats. Since carotenoids are sparsely soluble in water, unlike anthocyanins, the purple carrot juice was very low in carotenoids but high in total anthocyanins. The present results suggest that many responses to purple carrot juice differ from those following β-carotene supplementation. β-Carotene and purple carrot juice produced different responses on blood lipid profiles, lipid deposition in the liver, plasma alkaline phosphatase and lactate dehydrogenase activities, left ventricular stiffness and plasma markers of oxidative stress such as uric acid and malondialdehyde. The responses to β-carotene may be produced by vitamin A and its major metabolites, retinoic acid and retinaldehyde. Rats fed with vitamin A and retinoic acid showed a marked increase in hepatic and plasma concentrations of glycogen, cholesterol and acylglycerols with elevated plasma NEFA and increased glucose incorporation into liver lipids(Reference Singh, Singh and Venkitasubramanian41, Reference Gerber and Erdman42). Vitamin A stimulated fatty acid mobilisation from adipose tissue and enhanced formation of glycerophosphate through glycolysis, with consequent increases in the acylglycerol synthesis in the liver(Reference Singh, Singh and Venkitasubramanian41). Additionally, both retinoic acid and retinaldehyde inhibited adipogenesis(Reference Ziouzenkova, Orasanu and Sharlach43). Here we show that β-carotene prevented further increases in abdominal fat weight after treatment. It is therefore likely that β-carotene and its major metabolites suppress adipogenesis, thereby allowing excess accumulation of lipids in the liver and the plasma.
In the heart, retinoic acid inhibited G protein-coupled receptor-mediated hypertrophy as well as angiotensin II-induced increases in intracellular Ca2+ in neonatal cardiomyocytes(Reference Pan and Baker44). In vivo, retinoic acid suppressed myocardial cell hypertrophy(Reference Pan and Baker44) and prevented ventricular fibrosis and remodelling in spontaneously hypertensive rats(Reference Lu, Yao and Zhu45). β-Carotene and carotenoid breakdown products induced apoptosis in Jurkat E6.1 malignant T-lymphoblast cells(Reference Muller, Carpenter and Challis46) and human neutrophils(Reference Siems, Capuozzo and Crifo47) but impaired mitochondrial respiration and elevated accumulation of malondialdehyde(Reference Siems, Sommerburg and Schild48). Thus, β-carotene as a precursor of vitamin A and retinoic acid may increase lipid peroxidation, decrease cardiac hypertrophy, and prevent cardiac fibrosis and inflammation.
In contrast, purple carrot juice supplementation normalised plasma TAG, total cholesterol and NEFA concentrations, consistent with the hypolipidaemic responses to other anthocyanin-rich plant extracts such as blueberry leaf(Reference Cignarella, Nastasi and Cavalli29). NEFA is involved both in insulin resistance and hypertension by inhibiting aortic endothelial NO synthase activity through an oxidative mechanism(Reference Du, Edelstein and Obici49, Reference Steinberg, Tarshoby and Monestel50). The present results demonstrated enhanced vascular relaxant responses, consistent with improved NO production and lowered blood pressure.
Left ventricular dysfunction has been correlated with metabolic disturbances, oxidative stress and the appearance of inflammatory cells preceding left ventricular fibrosis and left ventricular stiffness(Reference van Heerebeek, Somsen and Paulus51). Purple carrot juice supplementation reduced left ventricular stiffness, the number of inflammatory cells and left ventricular collagen deposition. Echocardiographic assessment showed improved systolic function and decreased left ventricular dimensions following purple carrot treatment. Along with other results, reduction in lactate dehydrogenase activity and improved N homeostasis with purple carrot juice supplementation could be indicative of normalised metabolism leading to cardioprotective effects. Our data also showed that uric acid concentrations decreased in the purple carrot juice-treated rats. Uric acid, the endproduct of purine metabolism and a major endogenous water-soluble antioxidant(Reference Mene and Punzo52), is increased in fructose-fed rats following increased production of reactive oxygen species(Reference Stanhope and Havel33). Additionally, the reduction in plasma malondialdehyde concentrations, a major product of PUFA peroxidation, suggests in vivo antioxidant activity of purple carrot juice.
In contrast to β-carotene, purple carrot juice supplementation reduced liver wet weight, portal inflammation, fat deposition and portal fibrosis in the liver and showed total restoration of liver function. We have shown that purple carrot juice reversed the pathological features of NAFLD in the high-carbohydrate, high-fat diet-fed rats. Increased activities of the serum enzymes including aspartate transaminase, alanine transaminase and alkaline phosphatase reflect active liver damage. Inflammatory hepatocellular disorders result in extremely elevated transaminase levels(Reference Hultcrantz, Mengarelli and Strandvik53). Thus, purple carrot juice treatment has a significant role in the decrease or reversal of liver dysfunction since the activity of these plasma enzymes was lower than in high-carbohydrate-high-fat diet-fed rats and similar to maize starch diet-fed rats. Both β-carotene and purple carrot juice reduced plasma CRP concentrations, suggesting their possible in vivo anti-inflammatory effects.
The present study demonstrates for the first time that treatment of the metabolic syndrome induced by diet in rats with purple carrot juice attenuates or reverses the changes in cardiovascular and liver structure and functions as well as in metabolic parameters, especially abdominal fat deposition and plasma lipid profiles. As the juice itself contained low concentrations of carotenoids, it is likely that the anthocyanins are responsible for the antioxidant and anti-inflammatory properties of purple carrot juice. Furthermore, β-carotene alone produces limited and sometimes contradictory responses compared with purple carrot juice in this rat model of the metabolic syndrome.
Acknowledgements
We thank Mr Greg Jardine (Dr Red Nutraceuticals, Mt Nebo, QLD, Australia) for financial support to allow this project to be undertaken. The purple carrot juice was supplied by SDS Beverages Pty Ltd (Irymple, VIC, Australia). We thank Dr Fiona Campbell, School of Veterinary Science, The University of Queensland, for the acquisition of echocardiographic images.
H. P. and L. B. developed the original study aims; H. P. and S. P. conducted the experiments and carried out the data analyses; data were interpreted by all authors. The preparation of the manuscript drafts has involved all authors and L. B. has been the corresponding author throughout the writing process.
There are no conflicts of interest.