Introduction
The insect cuticle is mainly composed of chitin, linear polysaccharides, and proteins, resists mechanical damage, prevents dehydration, responds adeptly to fluctuations in the external environment, and acts as an impediment to the penetration of insecticides (Andersen, Reference Andersen2010; Noh et al., Reference Noh, Muthukrishnan, Kramer and Arakane2016b; Wan et al., Reference Wan, Hao and Feng2016). Pigmentation of the cuticle can also impact a number of insect physiological functions and behaviours including visual communication, camouflage, reproductive behaviours, and cuticle sclerotisation (Carroll, Reference Carroll2005; True et al., Reference True, Yeh, Hovemann, Kemme, Meinertzhagen, Edwards, Liou, Han and Li2005; Wittkopp and Beldade, Reference Wittkopp and Beldade2009). For most insects, the pigmentation process is driven by cuticular deposition of melanins, ommochromes, pteridines, tetrapyrroles, carotenoids, and/or flavonoids/anthocyanins (Fukuda and Onishi, Reference Fukuda and Onishi1971; Gorman and Arakane, Reference Gorman and Arakane2010).
Melanisation and the associated melanin biosynthetic pathway were first described in Drosophila melanogaster and have since been shown to be the biochemical basis for the phenotypic plasticity observed in body coloration across insects (Suderman et al., Reference Suderman, Dittmer, Kanost and Kramer2006; Gibert et al., Reference Gibert, Mouchel-Vielh, De Castro and Peronnet2016). Although most natural melanin pigments consist of varying ratios of eumelanin and pheomelanin (Thody et al., Reference Thody, Higgins, Wakamatsu, Ito, Burchill and Marks1991; Ito and Wakamatsu, Reference Ito and Wakamatsu2003; Naysmith et al., Reference Naysmith, Waterston, Ha, Flanagan, Bisset, Ray, Wakamatsu, Ito and Rees2004), insect melanins are derived from aromatic amino acids and related phenolic compounds (Prota, Reference Prota1995; Ito Reference Ito2003). These pigments are synthesised by melanocytes and subsequently conveyed through epidermal cells, culminating in their deposition within the cuticle (Wright, Reference Wright1987; Ito et al., Reference Ito, Wakamatsu and Ozeki2000; Ito and Wakamatsu, Reference Ito and Wakamatsu2008). The melanin biosynthetic pathway that regulates insect body colour patterning consists of an enzymatic cascade built on derivatisation of tyrosine (Shamim et al., Reference Shamim, Ranjan, Pandey and Ramani2014; Sugumaran and Barek, Reference Sugumaran and Barek2016). Tyrosine hydrogenase (TH) converts tyrosine into dihydroxyphenylalanine (DOPA), which can then be converted into DOPA melanin by a DOPA conversion enzyme (presumably a yellow family gene) or into dopamine via DOPA decarboxylase (DDC). Dopamine is then converted into one of three products: dopamine melanin by a dopamine conversion enzyme (presumably another yellow family gene), N-acetyl dopamine (NADA) by an arylalkylamine-N-acetyltransferase (aaNAT), or N-β-alanyldopamine (NBAD) by an NBAD synthetase encoded by ebony. NBAD can also be generated from L-aspartic acid by sequential enzymatic action of aspartate decarboxylase (black), which yields β-alanine, and the ebony NBAD synthetase. Both NADA and NBAD can then undergo enzymatic conversion to yield NADA sclerotin and NBAD sclerotin. Conversion of NBAD into dopamine is catalysed by the hydrolytic enzyme encoded by tan (Czapla et al., Reference Czapla, Hopkins and Kramer1990; Richardt et al., Reference Richardt, Kemme, Wagner, Schwarzer, Marahiel and Hovemann2003; Phillips et al., Reference Phillips, Smart, Strauss, Brembs and Kelly2005; True et al., Reference True, Yeh, Hovemann, Kemme, Meinertzhagen, Edwards, Liou, Han and Li2005; Aust et al., Reference Aust, Brüsselbach, Pütz and Hovemann2010; Mehere et al., Reference Mehere, Han, Christensen and Li2011; Hiragaki et al., Reference Hiragaki, Suzuki, Mohamed and Takeda2015; Chen et al., Reference Chen, Hou, Wei, Dou, Liu, Yang, Smagghe and Wang2018). The synthesis of yellow sclerotin pigments is thus dependent on the actions of ebony, whereas brown and black coloration requires tan (Wittkopp and Beldade, Reference Wittkopp and Beldade2009). Given the role of the pathway in insect pigmentation, disruption of different points in the enzymatic cascade can have significant impacts on cuticular coloration. For example, silencing TH and DDC severely reduced black coloration in Oncopeltus fasciatus (Liu et al., Reference Liu, Lemonds and Popadić2014). Similarly, Bombyx mori larval coloration changed from white to reddish-brown following functional deletion of yellow-e in (Ito et al., Reference Ito, Katsuma, Yamamoto, Kadono-Okuda, Mita and Shimada2010), and yellow-y knockdown caused Aedes albopictus eggs to turn yellowish-brown or reddish-brown (Noh et al., Reference Noh, Mun, Kramer, Muthukrishnan and Arakane2021).
Although disruptions of the melanin cascade manifest most clearly in atypical cuticular pigmentation, genes in the pathway also impact cuticle hardening, ecdysis, UV protection, thermoregulation, camouflage, mimicry, reproduction, immune defence reaction, and wound healing (Hill, Reference Hill1992; Söderhäll and Cerenius, Reference Söderhäll and Cerenius1998; Nappi and Christensen, Reference Nappi and Christensen2005; Sugumaran, Reference Sugumaran2016; Whitten and Coates, Reference Whitten and Coates2017; Sterkel et al., Reference Sterkel, Ons and Oliveira2019; Bai et al., Reference Bai, Pei, Liu, Fan and Zhang2022). Consequently, knocking down black, DDC, TH, or yellow-y genes can have diverse biological and physioloical impacts, including reduced survival in Sogatella furcifera (Guo et al., Reference Guo, Long, Liu, Ma, Zhang, Gong, Dewer, Hull, Wang, Wang, He and He2023), impaired vision in D. melanogaster (Phillips et al., Reference Phillips, Smart, Strauss, Brembs and Kelly2005), incomplete moulting in T. castaneum (Arakane et al., Reference Arakane, Lomakin, Beeman, Muthukrishnan, Gehrke, Kanost and Kramer2009) and Blattella germanica (Bai et al., Reference Bai, Pei, Liu, Fan and Zhang2022), and impaired mating success and reduced oviposition in D. melanogaster (Drapeau et al., Reference Drapeau, Cyran, Viering, Geyer and Long2006) and A. gambiae (Fuchs et al., Reference Fuchs, Behrends, Bundy, Crisanti and Nolan2014).
The German cockroach (Blattella germanica) is a major indoor pest of urban and rural areas that pollutes food and poses a health threat as both an allergenic source and as a vector of various parasites, viruses, and bacteria (Zhang et al., Reference Zhang, Sun, Zhang, Zhang, Lu, Xia, Huang and Wang2018). Further, as an intermediate host for many parasites, it also poses a risk to livestock. Currently, control of B. germanica is largely dependent on chemical pesticides, however, the persistence of pesticide residues and the potential for resistance to develop have become increasingly problematic (Wu and Appel, Reference Wu and Appel2017; Li et al., Reference Li, Zhu, Li, Qian, Yao and Guo2021; Mei et al., Reference Mei, Chen, Dong, Huang, Liu, Zhang, Wang, Hao and Yang2022). Thus, there is an urgent need for novel, environmentally friendly biological control methods and pest management strategies (Pan and Zhang, Reference Pan and Zhang2019). One potential strategy involves targeted disruption of insect reproduction, which is dependent on coordinated sexual behaviours that are frequently influenced by the emission and detection of sexual cues (Le Moëne and Ågmo, Reference Le Moëne and Ågmo2018; Ryan Reference Ryan2021). These cues include volatile sex pheromones that facilitate mate-finding and non-volatile contact sex pheromones (CSP) that mediate sex-recognition and mate selection (Auer and Benton, Reference Auer and Benton2016). In B. germanica, courtship and mating selection are mainly impacted by two homologous cuticular hydrocarbons, 3,11-dimethylheptacosan-2-one (C27 methyl ketone) and 3,11-dimethylnonacosan-2-one (C29 methyl ketone), as well as alcohols and aldehydes with each component capable of independently eliciting courtship (Eliyahu et al., Reference Eliyahu, Mori, Takikawa, Leal and Schal2004, Reference Eliyahu, Nojima, Capracotta, Comins and Schal2008; Wada-Katsumata and Schal, Reference Wada-Katsumata and Schal2019). Sexual communication in mature B. germanica is mediated through antennal contacts in which female-released CSPs trigger male-specific courtship behaviours that include reorientation around the female, wing lifts, and secretion from the male tergal gland (Shimomura et al., Reference Shimomura, Ishii and Nojima2019; McPherson et al., Reference McPherson, Wada-Katsumata, Hatano, Silverman and Schal2022). In response, female feeding on the secretion promotes male abdominal extension, grasping of female genitalia, and concludes in copulation (Hamilton et al., Reference Hamilton, Wada-Katsumata, Ko and Schal2021). The role of melanin-based cuticular pigmentation in CSP-mediated courtship processes is unclear. Here, we identify and functionally characterise the B. germanica black gene product and provide evidence for its function in cuticular coloration as well as wing development and male courtship behaviour.
Materials and methods
Insects
Blattella germanica were collected in Guilin, Guangxi, China in 2022 and subsequently raised in the laboratory. The colony was maintained in glass jars that were housed in an insect incubator set at 27 ± 1℃, 30–40% relative humidity, and a 12:12 h light/dark photoperiod. The colony was reared on commercial rat chow (Huizhou, China), the main guinea pig food source, and tap water.
Gene identification
To identify a potential B. germanica homologue of the D. melanogaster black gene (GenBank: NP_001246025.1) (Wright, Reference Wright1987), the fly protein sequence was queried against the B. germanica genome (Harrison et al., Reference Harrison, Jongepier, Robertson, Arning, Bitard-Feildel, Chao, Childers, Dinh, Doddapaneni, Dugan, Gowin, Greiner, Han, Hu, Hughes, Huylmans, Kemena, Kremer, Lee, Lopez-Ezquerra, Mallet, Monroy-Kuhn, Moser, Murali, Muzny, Otani, Piulachs, Poelchau, Qu, Schaub, Wada-Katsumata, Worley, Xie, Ylla, Poulsen, Gibbs, Schal, Richards, Belles, Korb and Bornberg-Bauer2018) using default BLASTp parameters.
RNA extraction and cDNA synthesis
Total RNA was isolated from 20 mg whole-body, 5-day-old B. germanica adults (mixed sex) using a FastPure cell / tissue total RNA isolation kit according to the manufacturer's instructions (Vazyme, Nanjing, China). RNA was quantified with an UV–Vis spectrophotometer SMA-4000 (Merinton, Beijing, China). First-strand complementary DNAs (cDNAs) were synthesised from 1 μg total RNA using a TransScript One-Step gDNA Removal and cDNA Synthesis SuperMix according to the manufacturer's instructions (Transgen Biotech, Beijing, China).
Phylogenetic tree
Protein sequences for Bgblack and black homologues (complete opening reading frames when available) from 55 species representing six insect orders (Blattaria, Orthoptera, Hemiptera, Coleoptera, Diptera, and Lepidoptera) were aligned with MAFFT v7.505 (Katoh et al., Reference Katoh, Rozewicki and Yamada2019) and a maximum-likelihood tree was constructed based on 1000 bootstrap values using IQ-TREE 1.6.12. The phylogenetic tree was annotated using ITOL version 6.5.8 (https://itol.embl.de). Protein sequences and GenBank accession numbers are listed in Table S1.
dsRNA synthesis and injection
Gene specific primers containing a 5’ T7 sequence (Table S2) were designed to amplify a 450-bp fragment of Bgblack and a 413-bp fragment of the negative control gene green fluorescence protein (GFP). Note, the regions targeted for knockdown were randomly selected. Bgblack amplification was performed in 25 μl reaction containing 12.5 μl of 2 × Taq Plus Master Mix (Vazyme),10 μl of ddH2O, 0.5 μl (50 ng μl−1) of cDNA template, and 1 μl of each primer (10 mmol l−1). The PCR programme consisted of: 95 °C for 2 min, followed by 40 cycles at 95 °C for 30 s, 62 °C for 20 s ( − 1 °C/cycle), 72 °C for 40 s, and another 30 cycles followed by 95 °C for 30 s, 52 °C for 20 s, and 72 °C for 40 s, with a final extension step at 72 °C for 10 min. PCR products were detected on a 1% agarose gel and the target bands were purified using a FastPure Gel DNA Extraction Mini Kit (Vazyme). Purified PCR products were subcloned into pMD19-T (Takara, Dalian, China) and transformed into Trans-T1 competent cells (Transgen Biotech). Single clones were sequenced by General Biology Co, LTD (Chuzhou, Anhui, China). The sequenced plasmids were used as templates to generate T7-containing PCR products. The purified products were then used to synthesise the corresponding dsRNAs with a T7 RNAi Transcription Kit (Vazyme). The dsRNA quality was checked using agarose gels and concentration was determined using a SMA-4000 UV–Vis spectrophotometer (Merinton). dsRNAs were stored at −80 °C until needed.
Fifth instar B. germanica nymphs were anesthetised with carbon dioxide for approximately 30 s and intrathoracically injected with 2 μl dsRNA (1500 ng μl−1) using a 10 μl glass syringe (Hamilton, Reno, NV, USA) as described previously (Wang et al., Reference Wang, Ma, Wang, Chen, Dewer, He, Zhang, Yang, Liu and He2020; Zhu et al., Reference Zhu, Liu, Liao, Yang, Bai, Li, Li, Luan and Chen2021). Three biological replicates were assayed with 10 nymphs per replicate. Survival rate and the percentage of adults exhibiting atypical phenotypes (e.g., cuticular coloration, wing development, etc.) were recorded daily for 20 days post-injection (dpi).
Quantitative real-time PCR (qPCR)
To examine the relative transcript level of Bgblack in B. germanica, total RNA was extracted from adults at 8 dpi. Five mixed sex dsBgblack- and dsGFP-injected adults comprised one biological replicate and transcript levels were assessed across three replicates. Glyceraldehyde-3-phosphate dehydrogenase (GAPDH, WCD39345.1) and elongation factor-1-alpha (EF1a, PSN34991.1) were used as reference genes (Zhu et al., Reference Zhu, Liu, Liao, Yang, Bai, Li, Li, Luan and Chen2021; Ma et al., Reference Ma, Gong, Zhang, Liu, Guo, Hull, Long, Wang, Dewer, Zhang, He and He2023). cDNAs were synthesised from 1 μg total RNA using a cDNA Synthesis SuperMix with gDNA removal (Transgen Biotech, Beijing, China). qPCR was performed on an iQ5 real-time PCR instrument (Bio-Rad, USA) using PerfectStar Green qPCR SuperMix (Transgen Biotech, Beijing, China) and gene specific primers (Table S2). All reactions were carried out in a 20 μl volume under the following conditions: one cycle at 94 ℃ for 30 s, then 50 cycles at 94 ℃ for 5 s, 60 ℃ for 30 s, 55 °C for 60 s followed by a melt curve analysis programme. The qPCR products were sequenced and the relative expression levels of the target genes were calculated using the 2−ΔΔCT method (Livak and Schmittgen, Reference Livak and Schmittgen2001). Primer efficiencies were all approximately 100% as determined from standard curves generated using five concentrations (1:10 serial dilutions) of template.
Colour intensity measurement
Experimental and control insects (ten 7-day-old whole body male and female adults) were digitally imaged using a JC-1002F stereo microscope (Jing Chang-48 million pixels, Shenzhen, China). Colour intensity in equivalent regions of the whole-body images was determined as mean greyscale values (average luminance) via ImageJ (version 1.53e). The colour intensities of each sex were measured separately. A higher colour intensity indicates darker cuticle pigmentation (Noh et al., Reference Noh, Koo, Kramer, Muthukrishnan and Arakane2016a). All of the samples were imaged under the same conditions.
Courtship bioassay
Newly emerged adults were separated by sex and maintained in groups. Control (dsGFP) and experimental (dsBgblack) adult males at 7 dpi (D7) were introduced during hours 4–10 of the dark cycle into a black plastic box (23 × 6 × 10 cm) and allowed to equilibrate to the surrounding conditions for 20–30 mins. The courtship bioassay was generally followed by previous studies. The antennae of wild-type (WT) D10 virgin female cockroaches are cut off from the base using medical tweezers and then attached to a 20 cm long metal rod with solid paraffin to create an ‘antenna on a stick’ as a stimulus source. Courtship behaviour assays were conducted in a dark room during mid-scotophase (i.e., the first and last 2 hrs of scotophase were not assessed). The males were then exposed to females from the control and experimental groups and antennation between the sexes was recorded on an HD camera (Sony, FDR-AX60, Shanghai, China) under red light. The distance between the camera and the insects was ~20 cm. Recording began at male wing extension (i.e., indicator of active courtship) following male antennal contact with female antennae and ended when male wings returned to their normal positions. The total behaviour test period evaluated was limited to 300 s. Females were discarded after every two male encounters. A courtship index [CI = total courtship time/total behaviour test time (300 s)] was determined with the number and duration of wing-raising events determined over the evaluation period. Assays consisted of 20 males and all adults used in the courtship assay were 7-days-old. Statistical significance was determined using unpaired t-tests.
Results
Gene identification and phylogenetic tree
Bgblack (GenBank accession number: PSN32645.1) encodes a 1053 amino acid protein. The sequence similarity for the highest BLAST hit is the Diploptera punctata black gene (KAJ9589117.1), which has 70.61% sequence identity with Bgblack. Phylogenetic analysis of the protein with black proteins from species representing six insect orders revealed order-specific clustering of the sequences with the Bgblack protein sorting to the Blattodea clade along with proteins from Coptotermes formosanus, Cryptotermes secundus, and Zootermopsis nevadensis (fig. 1). Further, the tree topology suggests an evolutionarily conserved ancestor of black that is common to the hemimetabolous Blattodea, Hemiptera, and Orthoptera.
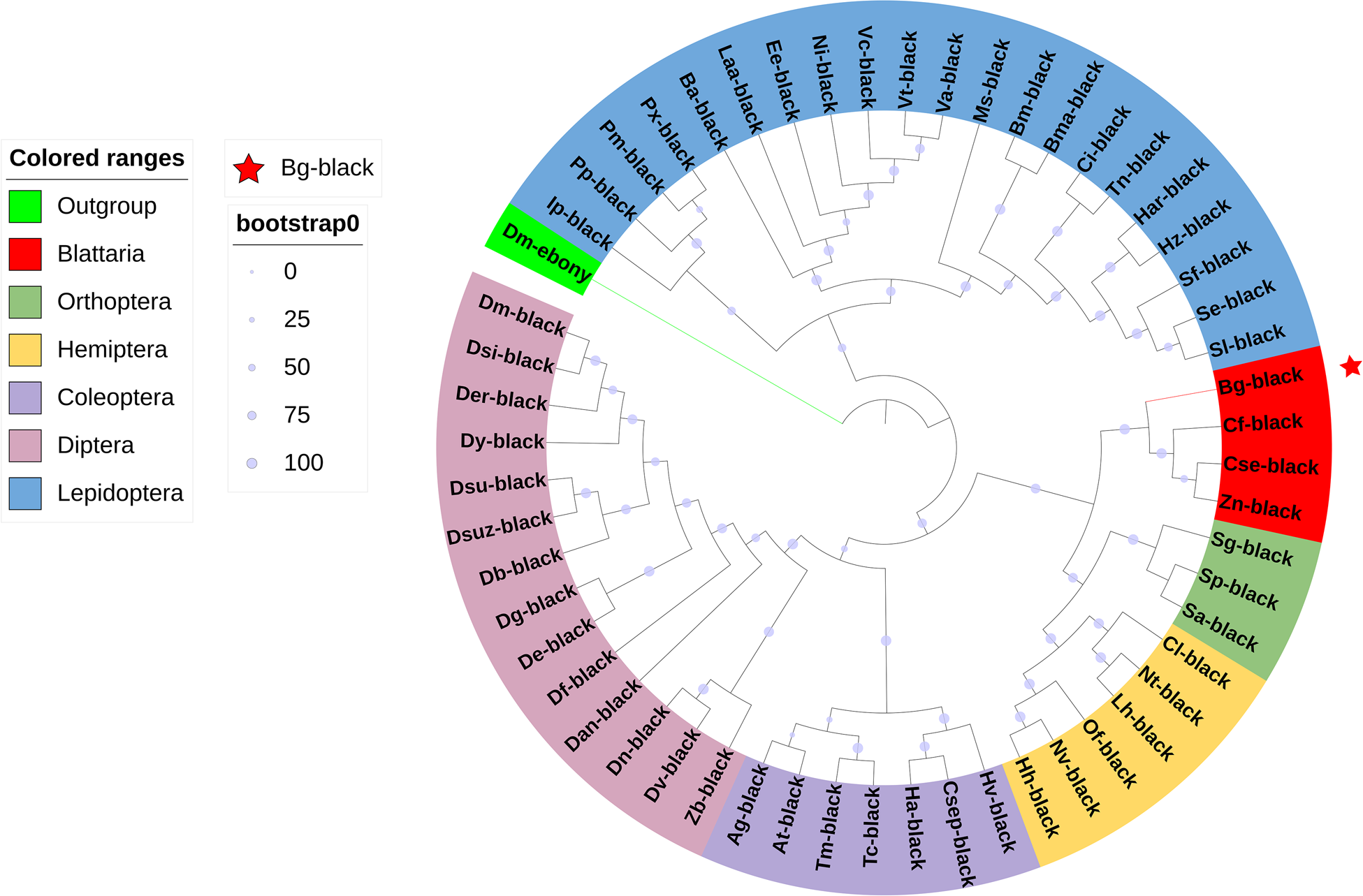
Figure 1. Phylogeny of black proteins from diverse insects.
Phylogenetic analysis of Bgblack in relation to black homologues identified in insects from six orders (Blattodea, Orthoptera, Hemiptera, Coleoptera, Diptera, and Lepidoptera). Bgblack is labelled with red solid five-pointed stars in the figure. The phylogenetic tree was rooted on the Drosophila melanogaster ebony protein. Accession numbers of proteins used in the analysis are listed in Table S1.
Phenotypic analysis of Bgblack
At 8 dpi, target transcript levels were significantly reduced compared with the dsGFP control group (fig. 2). Perturbations in cuticular coloration was observed in 95.83% of dsBgblack adults, whereas no phenotypic abnormalities were observed in the dsGFP group (fig. 3). The dsGFP group was characterised by semi-transparent tannish-brown coloration throughout the adult body regardless of sex (fig. 4A-B). In contrast, males and females in the dsBgblack group were opaque black (fig. 4C-D). Based on pixel intensity, melanin-associated pigmentation in males and females of the dsGFP group was lower than those of the dsBgblack group (fig. 4E-F). Compared to the dsGFP group (fig. 5A-G), atypical coloration was apparent throughout the body and appendages (e.g., antennae, wings, legs, cerci) of the dsBgblack group (fig. 5H-N). Although dsBgblack adults were significantly darker than the control group, both sexes exhibited incomplete melanisation of wings, abdomens, thoraxes, and legs as some yellow coloration persisted and the main body segments (abdomen/thorax and dorsum) retained a degree of transparency (figs 5J-K). In contrast, the heads, antennae, and cerci were opaque black (figs 5H, 5I and 5N). Moreover, the abdominal and thoracic regions of dsBgblack females were darker in appearance than that of males (fig. 5J).

Figure 2. RNAi-mediated knockdown of Bgblack transcripts.
Bgblack transcript levels were assessed using adults at 8 days post-dsRNA injection in relation to GAPDH and EF1a. Controls were injected with dsGFP. Statistical differences were determined using an unpaired t-test (*, P < 0.1; **, P < 0.01).

Figure 3. Percentage of B. germanica adults exhibiting atypical cuticular coloration.
Cuticular coloration was assessed in adults at 8 days post-dsRNA injection. The control group was injected with dsGFP. Statistical differences were determined using an unpaired t-test (*, P < 0. 1).

Figure 4. Effects of RNAi-mediated knockdown on cuticular coloration.
(A-D) Cuticular coloration phenotypes in adults at 8 days post-dsRNA injection. (A) dsGFP knockdown male. (B) dsGFP knockdown female. (C) dsBgblack knockdown male. (D) dsBgblack knockdown female. Images are representative of phenotypes observed in 10 replicates, including 10 female and 10 male per replicate. Scale bar is 500 μm. The whole-body colour (sum of dorsal and ventral) intensity was determined as mean grey values (average luminance) with ImageJ. (E) Male colour intensity. (F) Female colour intensity. The control group was injected with dsGFP. Values are the mean of 10 males and females from each treatment group. Statistical differences were determined using an unpaired t-test (*, P < 0.1; **, P < 0.01, ***, P < 0.001), ‘n.s.’ indicates no significant differences.

Figure 5. Bgblack knockdown phenotypic effects on appendage and body segment pigmentation.
Cuticular coloration phenotypes exhibited by various appendages (antennae, wings, legs, and cerci) and body segments (head, ventral thorax and abdomen, and dorsum). (A–G) dsGFP. Adults exhibited typical coloration and morphology. (H–N) dsBgblack. Adults were characterised by significantly darkened body colour and opaque cuticle. Phenotypes were assessed using adults 8 days post-dsRNA injection. Scale bar is 500 μm.
Unexpectedly, impaired wing expansion was observed in a significant percentage (37.50%; 9/24) of the dsBgblack group (fig. 6A). Unlike the control group wings, that rested seamlessly along the length of the dorsal abdomen (fig. 6B-C), both the forewing and the hindwing in the dsBgblack group were severely stunted and protruded from the abdomen (fig. 6D-E). The wing defects were not sex-specific as they occurred with comparable frequency in males (44.44%) and females (55.56%).

Figure 6. Bgblack knockdown effects on adult wing development.
(A) Percentage of Blattella germanica exhibiting atypical wing expansion. (B) Effects of RNAi-mediated Bgblack knockdown on wing expansion. Bars indicate means ± SEM. The asterisks (**) indicate significant differences (P < 0.01) relative to dsGFP injected adults. Statistical differences were determined using an unpaired t-test. (B-E) Wing phenotypes in adults 8 days post-dsRNA injection. (B) dsGFP male. (C) dsGFP female. (D) dsBgblack male. (E) dsBgblack female. Insets are a focused zoom on the wings. Scale bar is 500 μm.
Impacts on male courtship ability
Males in the dsGFP control group exhibited stereotypical mating behaviours. When a male antennae touched a female antennae, the male executed a stereotypical courtship response, which involved rotation of the male body relative to the stimulus (i.e., female antennae), male wing lift, and presentation of the male dorsal abdomen in response to antennation by females from the dsGFP group (Video S1). The mean calculated courtship index (CI) was 0.53 (fig. 7A) and the mean number of male wing extensions was 17.96 (fig. 7B). In contrast, reductions in the CI (0.23; Fig. 7A) and increased latency in wing extension (11.17; Fig. 7B) were observed in dsGFP males following female dsBgblack antennation (Video S2). For dsBgblack males, courtship responses (fig. 7A-B) to dsGFP female antennae were severely inhibited (CI of 0.10; mean wing extension = 4.14) with most males (16/22) unresponsive throughout the 300 s evaluation period (Video S3). For the few responsive males (6 of 22), the latency between wing extensions was longer and the duration of each event was shorter. The most attenuated behaviours (CI of 0.05; mean wing extension = 2.43) were observed in the double dsBgblack group (i.e., Bgblack knockdown in both males and females, Video S4). Notably, males with wing deformities (4/22) were unable to raise their wings and as a result had a failed courtship (CI = 0).

Figure 7. Effect of Bgblack knockdown on male courtship.
(A) Male courtship index (CI = total courtship time/total behavioural test time). (B) The number of males exhibiting wing extension. Controls were injected with dsGFP. In total 23 dsGFP and 21 dsBgblack males were tested. The evaluation period was 300 s. Error bars represent ± SEM. Statistical differences were determined using an unpaired t-test (*, P < 0.1, **, P < 0.01; ***, P < 0.001, ****, P < 0.0001). ‘n.s.’ denotes ‘not significant’ (P > 0.05). See supplementary Video 1–4 for representative depictions of behaviour for each group.
Discussion
Unlike vertebrates, dopamine-derived compounds dominate the highly conserved insect melanin biosynthesis pathway (Wittkopp and Beldade, Reference Wittkopp and Beldade2009; Barek et al., Reference Barek, Sugumaran, Ito and Wakamatsu2018a), (Lemonds et al., Reference Lemonds, Liu and Popadić2016; Mun et al., Reference Mun, Mi, Kramer, Muthukrishnan, Arakane and Biology2019). With tyrosine as a precursor, TH and DDC catalyse the synthesis of DOPA and dopamine, respectively, which are subsequently converted to melanin forms via DOPA and dopamine conversion enzymes (Gorman et al., Reference Gorman, An and Kanost2007; Arakane et al., Reference Arakane, Lomakin, Beeman, Muthukrishnan, Gehrke, Kanost and Kramer2009). NBAD melanin, in contrast, is derived from a separate pathway that uses ebony to generate NBAD from β-alanine, which is generated by black-mediated catalysis of L-aspartic acid. In this study, the B. germanica homologue of black was identified and functionally characterised. Consistent with previous studies (Popadić and Tsitlakidou, Reference Popadić and Tsitlakidou2021; Brent et al., Reference Brent, Heu, Gross, Fan, Langhorst and Hull2022), phylogenetic analyses revealed that black is highly conserved across insect orders. Similarly, the phenotypic effects of Bgblack knockdown on cuticular coloration (i.e., darker opaque melanin pigmentation across body segments and appendages, with more pronounced effects in female abdomens and thoraces) are consistent with previous reports. For example, RNAi-mediated knockdown of black in O. fasciatus adults resulted in expanded black pigmentation in heads, thoraces and forewings, but not in the hindwings or abdomen (Liu et al., Reference Liu, Lemonds, Marden and Popadić2016), and its knockdown in B. mori resulted in pupae with darker-coloured heads and thoraces (Dai et al., Reference Dai, Qiao, Cao, Liu, Tong, He, Hu, Zhang, Wu, Tan, Xiang and Lu2015). In Lygus hesperus, RNAi knockdown of tan, black, and ebony had differential effects on appendage and body coloration (Brent et al., Reference Brent, Heu, Gross, Fan, Langhorst and Hull2022). In Henosepilachna vigintioctopunctata, silencing black selectively deepened the colour of the larval heads, antennae, mouthparts, and legs in third instar larvae and further darkened the coloration of adult bodies (Ze et al., Reference Ze, Jin and Li2022).
In addition to affecting body coloration, Bgblack also impacts wing development as knockdown impaired expansion of both the forewing and hindwing. Similar defects were reported following targeted knockdown of melanin biosynthetic pathway genes in S. furcifera (Guo et al., Reference Guo, Long, Liu, Ma, Zhang, Gong, Dewer, Hull, Wang, Wang, He and He2023). Insect wing development is typically regulated by hormonal factors, as well as Hox genes such as antennapedia (Chen et al., Reference Chen, Tong, Li, Fu, He, Hu, Xiang, Lu and Dai2013; Fang et al., Reference Fang, Xin, Sun, Monteiro, Ye, Dai, Lu and Tong2022). Although Hox genes have been reported to act upstream of cuticular pigmentation pathways in some species(Angelini et al., Reference Angelini, Liu, Hughes and Kaufman2005; Jeong et al., Reference Jeong, Rokas and Carroll2006; Liu et al., Reference Liu, Ramos-Womack, Han, Reilly, Brackett, Rogers, Williams, Andolfatto, Stern and Rebeiz2019), their connection with Bgblack remains to be elucidated as does the linkage between the melanin biosynthetic pathway and wing development.
Courtship behaviours are critical prerequisites for insect reproduction. Adult male B. germanica sexual cues are communicated through female antennation, which promotes wing extension and presentation of the abdominal dorsum by the male (Eliyahu et al., Reference Eliyahu, Mori, Takikawa, Leal and Schal2004). We found that male responses to female antennation were inhibited following Bgblack knockdown and that knockdown males rarely displayed stereotypical courtship behaviour regardless of the status (control or experimental) of the female partner. The few instances in which knockdown males did respond, their behaviour was lethargic, with increased latency in wing extension and reduced periods of wing lifts. Bgblack knockdown also negatively impacted females, which manifested as a decrease in female antennae attractiveness, suggesting impaired CSP production and/or detection. Incredibly, black male individuals with damaged wings are unable to exhibited normal courtship behaviour indicating that wing/wing-raising is a key factor in German cockroach courtship. The impact of melanin biosynthesis genes on insect sexual behaviour either indirectly through neural circuit influences or directly via impaired development of sex-associated tissues and structures is not without precedent. Silencing yellow-y reduces expression of the D. melanogaster mating-success regulatory sequence, which is positively correlated with mating success rate (Drapeau et al., Reference Drapeau, Cyran, Viering, Geyer and Long2006). Furthermore, fruitless, which plays an important role in D. melanogaster sex differentiation, is upstream of yellow and inhibition of yellow reduces male courtship ability (Drapeau et al., Reference Drapeau, Radovic, Wittkopp and Long2003). Direct effects of melanin biosynthesis genes on reproduction have also been reported. TH disruption results in smaller D. melanogaster ovaries and reduced female reproductive capability (Fuchs et al., Reference Fuchs, Behrends, Bundy, Crisanti and Nolan2014).
As a potent neurotransmitter that is active in dopaminergic neurons, dopamine plays a critical role in insect development and reproduction, it is also a key precursor in the epidermal melanin biosynthetic pathway (Saedi et al., Reference Saedi, Khoradmehr, Mohammad Reza and Tamadon2018). In insect brains, some of the dopamine is converted in glial cells into NBAD, which is then transported to the presynaptic neurons and converted back to dopamine by NBAD hydrolase (Abebe et al., Reference Abebe, Zheng, Evans and Sugumaran2010). Despite their presence, neither NBAD nor NADA are involved in sclerotisation of the insect brain (Barek et al., Reference Barek, Veraksa and Sugumaran2018b). However, both are key substrates in the epidermal melanin biosynthetic pathway and play key roles in sclerotisation of the insect cuticle. Thus, the reduced NBAD biosynthesis associated with Bgblack knockdown may impede the B. germanica neural circuit. Dopamine impacts multiple behaviours in Apis mellifera (Huang et al., Reference Huang, Zhang, Feng, Zhao, Aldanondo, de Brito Sanchez, Paoli, Rolland, Li, Nie, Lin, Zhang, Giurfa and Su2022), and dopaminergic signalling regulates the persistence and duration of mating in male Drosophila (Zhang et al., Reference Zhang, Rogulja and Crickmore2016). Moreover, in addition to cuticular pigmentation effects, behavioural defects are associated with mutations to tan and black in Drosophila (Yamamoto and Seto, Reference Yamamoto and Seto2014). For tan mutants, the abnormally low dopamine levels that negatively impact the visual system may also lead to behavioural impairments (Konopka, Reference Konopka1972). Conversely, functional loss of black, which converts dopamine to NBAD, can lead to excess dopamine. Tong et al. (Liu et al., Reference Liu, Dartevelle, Yuan, Wei, Wang, Ferveur and Guo2008) found that increased dopamine levels promoted courtship behaviour between Drosophila males but did not change behaviours towards virgin females, general olfactory responses, general gustatory responses, or locomotor activity. In addition to inducing perturbations in normal male courtship behaviours, excessive dopamine levels can also lead to sleep and visual behavioural abnormalities (Andretic et al., Reference Andretic, van Swinderen and Greenspan2005). Recently, black knockdown was reported to lead to a significant decrease in dopamine levels in the head of D. melanogaster (Deal et al., Reference Deal, Bei, Gibson, Delgado-Seo, Fujita, Wilwayco, Seto and Yamamoto2023). Based on these findings, the atypical mating behaviours observed following Bgblack knockdown might be attributable to lower dopamine levels in the head or brain of affected males. Alternatively, the lower sensitivity of Bgblack knockdown male cockroaches to female antennation might indicate a role for Bgblack in regulating CSP levels. Correspondingly, dopamine has been shown to modulate female sexual receptivity in D. melanogaster (Neckameyer, Reference Neckameyer1998).
Conclusion
In conclusion, we utilised RNAi to functionally characterise the role of black in B. germanica. Consistent with the hypothesised role in melanin biosynthesis, Bgblack is essential for body colour patterning; however, it also plays a critical role in wing development. Furthermore, Bgblack knockdown also significantly disrupted stereotypical adult mating behaviours, and black male individuals with damaged wings were unable to exhibit courtship behaviour. Taken together, these findings provide further insights into insect pigmentation pathways and open new avenues of research to explore new ecologically friendly pest control strategies.
Supplementary material
The supplementary material for this article can be found at https://doi.org/10.1017/S0007485324000166.
Acknowledgements
We thank Dr Nan Chen (Huanan Normal University) for assistance on the CSP contact assay. This research was supported by the National Natural Science Foundation of China (No. 32370527 for PH and Grant No. 32260671 for MH), the Guizhou Provincial Science and Technology Projects (QKHJ-ZK[2024]-ZD-007 for PH), the Scientific Research Foundation of Guizhou University of China (2017-33 for MH), the Program of Talent Cultivation of Guizhou University ([2019]05 for PH); the Science and Technology Support of Guizhou province (QKH[2017]2956 for MH); the Program of Introducing Talents of Discipline to Universities of China (111 Program, D20023), and the Frontiers Science Center for Asymmetric Synthesis and Medicinal Molecules, Department of Education, Guizhou Province [Qianjiaohe KY number (2020) 004]. Mention of trade names or commercial products in this article is solely for the purpose of providing specific information and does not imply recommendation or endorsement by the U.S. Department of Agriculture. USDA is an equal opportunity provider and employer.