This concluding article of a three-part series summarises current knowledge on the primary common pathways thought to contribute to psychosis risk. A range of risk factors have been identified in parts 1 and 2 of this series (Romain Reference Romain, Eriksson and Onyon2019a, Reference Romain, Eriksson and Onyon2019b) and these now come together with a focus on three final common pathways, overlapping and interacting with each other in a complex system. The three key mechanisms involved are: alterations in neurology, neurochemistry and inflammatory pathways.
It is not possible to identify one pathway as the only cause, as often risk factors have significant effects on multiple key paths and these three primary pathways themselves interact. In this article we summarise each pathway and, from our review of recent data in this field, offer suggestions for primary, secondary and tertiary preventive measures. Given the complexity of this topic, there is significant ongoing research to further clarify these and other mechanisms.
Pathway 1: neurological structure and function
Neurostructure, function and development are frequently implicated as pathways by which psychosis risk factors lead to significant biological changes and symptom expression. For example, in part 2 of this series (Romain Reference Romain, Eriksson and Onyon2019b) we discussed evidence that childhood maltreatment could alter neurostructure, particularly hippocampal development (Teicher Reference Teicher, Anderson and Polcari2012). Other factors discussed in this series that might increase risk for psychosis via neurodevelopment, plasticity and structure include, among others, cannabis use, sleep difficulty, genetic factors and perinatal difficulties.
Development and plasticity
The orbitofrontal cortex has been identified as an area in which early neurodevelopmental abnormalities can be detected. It is thought to be involved in sensory integration and decision-making, and it fixes in a folding pattern shortly after birth (Lavoie Reference Lavoie, Bartholomeuz and Nelson2014). There are four types of orbitofrontal cortex folding patterns, and a reduced incidence of type 1 has been demonstrated to be present more often in high-risk individuals who later transition to psychosis (Lavoie Reference Lavoie, Bartholomeuz and Nelson2014; Isomura Reference Isomura, Hashimoto and Nakamura2017). Further markers of abnormal neurodevelopment include decreased cerebral asymmetry, in the form of reduced one-sided dominance (Dean Reference Dean, Orr and Newberry2016).
Alterations in neuroplasticity have been linked to symptoms of psychosis. Neuroplasticity is thought to occur in an early critical period, where developmental experiences and the resulting neuronal activity shape neural responses. Later, further changes in neuronal activity due to experiences throughout development lead to lasting neural representations. The brain can be thus altered in adaptive or maladaptive ways. Changes in the structure of key brain circuits can lead to cognitive deficits, affective dysregulation and psychosis (Keshavan Reference Keshavan, Mehta and Padmanabhan2015). Current knowledge on this topic has produced information on many different mechanisms by which neuroplasticity is disrupted, key examples of which are demonstrated in Fig. 1.
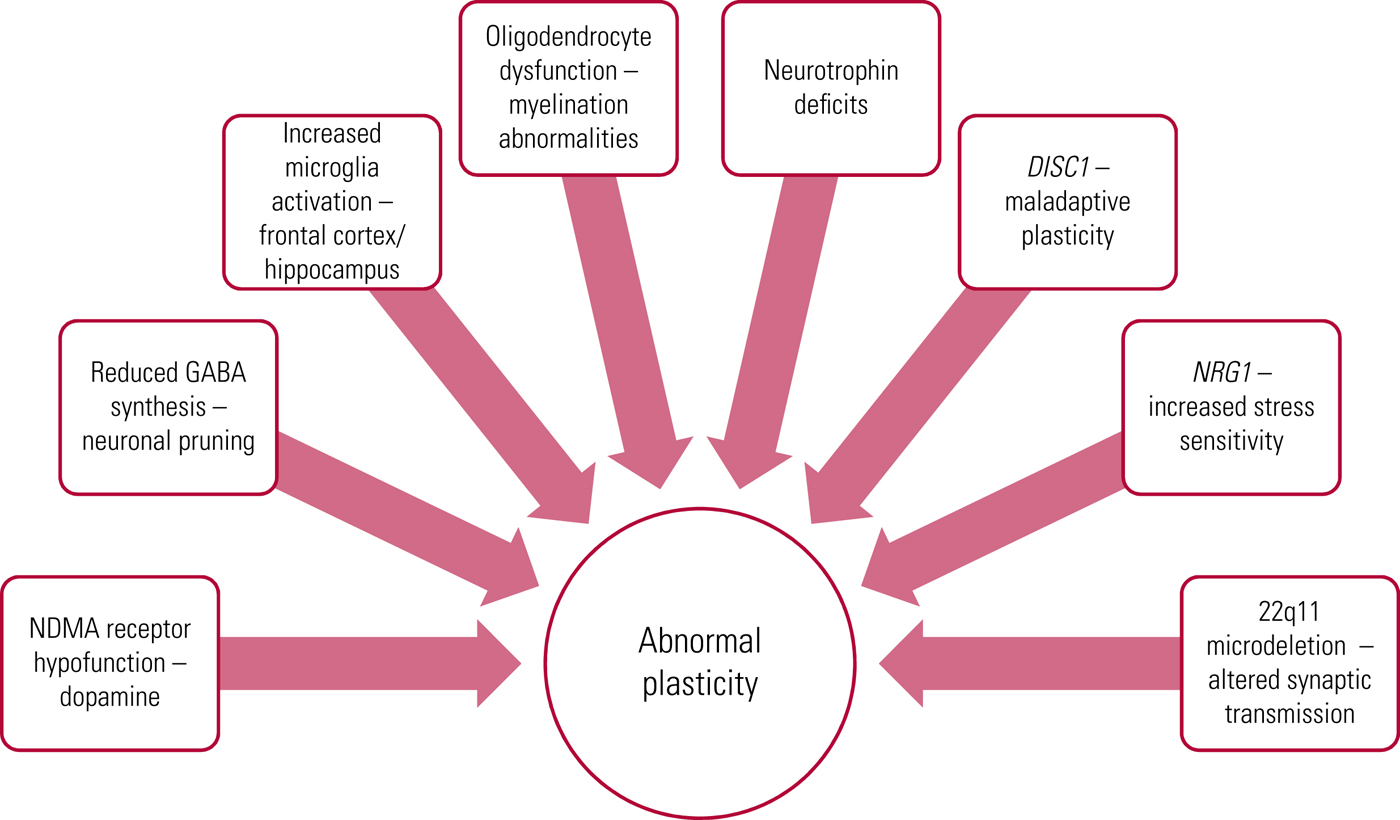
FIG 1 Mechanisms known to disrupt neuroplasticity (Keshavan Reference Keshavan, Mehta and Padmanabhan2015). GABA, γ-aminobutyric acid; NMDA, N-methyl-d-aspartate.
Positive symptoms of schizophrenia are linked to aberrant excessive synaptic plasticity due to dysregulation in the pathways in Fig. 1. This is thought to be a result of the genetic and environmental factors discussed previously (Romain Reference Romain, Eriksson and Onyon2019a, Reference Romain, Eriksson and Onyon2019b), including stress and substance misuse, on a background of decreased cortical plasticity. This abnormal connectivity has been linked to aberrant processing of language, auditory and visual stimuli, thus suggesting an association between abnormal plasticity and clinical psychopathology (Keshavan Reference Keshavan, Mehta and Padmanabhan2015). Neuronal synchronisation can be altered by oxidative stress, which is itself linked to genetic and environmental risk factors for psychosis. This is particularly true of parvalbumin inhibitory interneurons, which are thought to have a role in social behaviour and cognitive processing (Steullet Reference Steullet, Cabungcal and Coyle2017).
Neurostructure
A large body of evidence examines the role of neurostructural changes in psychosis development; some key papers, which consider a variety of psychosis-risk populations, are summarised in Table 1. They include, for example, a meta-analysis by Fusar-Poli et al (Reference Fusar-Poli, Borgwardt and Crescini2011), who demonstrated the key finding of grey matter reductions in regions of interest in psychosis (a finding that has been further corroborated by more recent evidence).
TABLE 1 Key papers that consider risk factors for psychosis
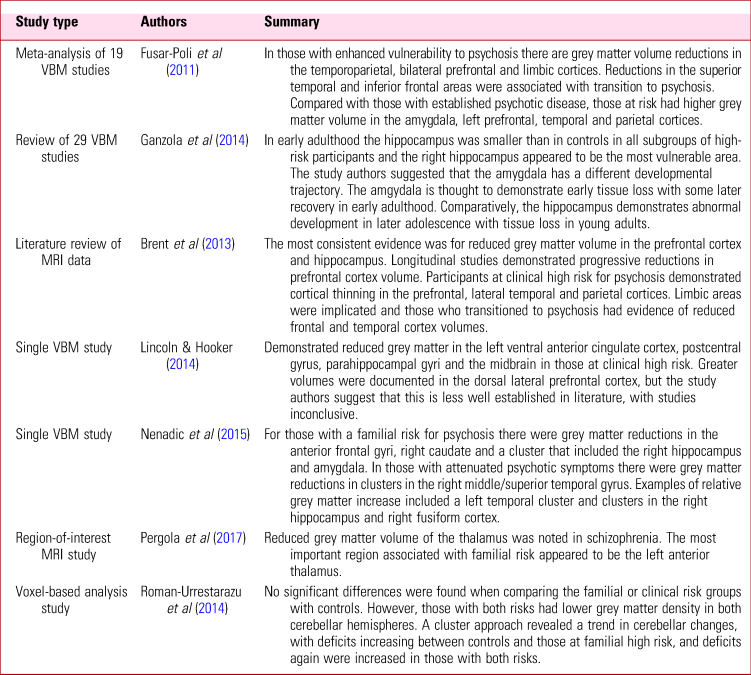
MRI, magnetic resonance imaging; VBM, voxel-based morphometry: automated whole-brain imaging that allows investigation of focal differences in brain areas using statistical mapping (Fusar-Poli Reference Fusar-Poli, Borgwardt and Crescini2011).
When studied in adulthood, participants with childhood or early-onset schizophrenia have consistently smaller whole-brain volume, with enlargement of the lateral ventricles and a smaller prefrontal cortex (Brent Reference Brent, Thermenos and Keshavan2013). Selvaraj et al (Reference Selvaraj, Bloomfield and Cao2018) investigated the relationship between volume changes in cortical regions and microglial activation using positron emission tomography (PET) and magnetic resonance imaging (MRI). Findings demonstrated that individuals with schizophrenia showed microglial activation inversely related to total cortical grey matter volume. Although this was not evident in their ‘high risk for psychosis’ group, their data begin to suggest that microglial activity could be related to these cortical volume changes and demonstrate a need for future research to examine the nature of this relationship further (Selvaraj Reference Selvaraj, Bloomfield and Cao2018).
The limbic areas of the hippocampus and amygdala were considered in work by Ganzola et al (Reference Ganzola, Maziade and Duchesne2014) because of the role of the hippocampus in memory and that of the amygdala in emotional expression and regulation. Their work suggested a critical period in which the hippocampus begins to develop differently in those at high risk and therefore concluded that hippocampal changes could represent a prodromal marker of psychosis (Ganzola Reference Ganzola, Maziade and Duchesne2014).
Functional neuroimaging data
Electroencephalogram (EEG) alterations such as higher levels of frontal and parietal delta power are linked with transition to psychosis in those at clinical high risk. Furthermore, alpha peak frequencies, a marker of thalamocortical processing speed, have been demonstrated to be slower in those who later transitioned (van Tricht Reference van Tricht, Ruhrmann and Arns2014). Andreou et al (Reference Andreou, Faber and Leicht2014) found that specific EEG microstates, defined as momentary local states associated with different mental processes, were similar in those at high risk and those with a schizophrenia diagnosis. This evidence collectively demonstrates that not only structural but also electrical alterations may be evident in those at risk for psychosis.
Winton-Brown et al (Reference Winton-Brown, Kumari and Windler2015) found that clinical high risk for psychosis was associated with both early and late deficits in sensorimotor gating when assessed with acoustic stimuli and the eye-blink startle response. This process has a role in how we allocate our cognitive resources, and therefore alters how we respond to a stimulus. Early changes appeared to be modulated by, for example, cannabis use and so could demonstrate a possible mechanism for how this alters neurological function (Winton-Brown Reference Winton-Brown, Kumari and Windler2015).
Further functional data were considered in a meta-analysis by Mothersill & Donohoe (Reference Mothersill and Donohoe2016), who investigated whether environmental stress was associated with blood oxygen level-dependent (BOLD) response in different brain regions. They demonstrated altered responses to stress in specific areas. The most significant findings were in the right amygdala, and responses were greater in individuals with a history of environmental stress. It is therefore plausible, as discussed in part 2 (Romain Reference Romain, Eriksson and Onyon2019b), that exposure to stressful social environments may have important influences on these neurological pathways (Mothersill Reference Mothersill and Donohoe2016).
The central executive network (CEN), investigated by Jukuri et al (Reference Jukuri, Kiviniemi and Nikkinen2015a), is located in the frontoparietal region and is responsible for managing high-level cognitive functions such as attention, decision-making and working memory. It was shown that in those at familial high risk for psychosis, activity was reduced in the right inferior frontal gyrus. Activation of the default mode network (DMN) (which is utilised during resting) was lower in the posterior cingulate cortex in familial high-risk participants. Vulnerability to psychosis is further associated with increased activity in the right anterior lobe of the cerebellum and these functional changes again link to altered DMN and CEN functioning (Jukuri Reference Jukuri, Kiviniemi and Nikkinen2015b).
Impaired working memory is a feature of schizophrenia related to prefrontal cortex dysfunction. A decrease in memory-task-related deactivation in the medial prefrontal cortex is present in those at clinical high risk for psychosis. Familial high-risk patients were shown to have an intermediate level of deactivation between those at clinical high risk and controls. Increased activation in the precuneus during completion of a memory task was particularly associated with altered levels of negative symptoms (Falkenberg Reference Falkenberg, Chaddock and Murray2015). Valli et al (Reference Valli, Marquand and Mechelli2016) investigated responses to a verbal memory task, using support vector machine (SVM) technology. The SVM method helps identify a set of areas that distinguish a group, rather than a specific individual region. In those at high risk for psychosis their results suggested that focal functional changes were present on a background of diffuse structural alterations.
Link to symptomatology
Many risk factors, as described previously (Romain Reference Romain, Eriksson and Onyon2019a, Reference Romain, Eriksson and Onyon2019b), are linked to neurological alterations. Evidence suggests that many of these altered areas can be to varying extents associated with psychosis symptoms. For example, reduced grey matter volume in the postcentral gyrus and anterior cingulate cortex have been associated with decreased social functioning. Abnormalities in the anterior cingulate gyrus have been proposed as a neurobiological mechanism for social dysfunction in schizophrenia, on the basis of its role in social and emotional processing. Similarly, as the postcentral gyrus has been linked to social cognition and, in particular, emotion recognition, this has also been put forward as a candidate area (Lincoln Reference Lincoln and Hooker2014).
Pathway 2: neurochemical
Dopamine
Schizophrenia, and more broadly psychosis, research has often focused on the role of dopamine. Many of the risk factors discussed in parts 1 and 2 of this series (Romain Reference Romain, Eriksson and Onyon2019a, Reference Romain, Eriksson and Onyon2019b), for example, amphetamines, caffeine, isolation and childhood maltreatment, have been found to result in a variety of different alterations within the dopamine system.
Investigating 47 individuals at ultra-high risk for psychosis and 20 healthy matched volunteers, Egerton et al (Reference Egerton, Valmaggia and Howes2016) assessed striatal dopamine function using PET. Within the sample as a whole, both severe abuse and unstable family arrangements in childhood were associated with elevated striatal dopamine function in adulthood. However, they were not able to demonstrate a significant difference between high-risk participants and controls (Egerton Reference Egerton, Valmaggia and Howes2016). Higher levels of dopamine release follow psychological stress in those at clinical high risk compared with controls. Animal studies suggest that chronic inescapable stressors may downregulate the dopamine system, whereas intermittent or escapable stressors may have a sensitising effect; these findings demonstrate that the type and duration of stress seem to be associated with differences in dopamine response (Howes Reference Howes, McCutcheon and Owen2016).
Meta-analysis of imaging studies by Howes et al (Reference Howes, Kambeitz and Kim2012) found primarily presynaptic dopaminergic abnormalities in schizophrenia, alongside abnormal dopamine synthesis capacity. Box 1 summarises further primary dopamine alterations discussed by Howes et al in a subsequent article (Howes Reference Howes, Kambeitz and Kim2016).
BOX 1 Primary dopamine alterations in schizophrenia
• Increased striatal presynaptic dopamine synthesis and release
• Significant elevation in dopamine following a challenge designed to stimulate release
• Baseline occupancy of D2 and D3 receptors is elevated, indicating higher resting dopamine levels
• Striatal dopamine release and baseline dopamine levels are closely correlated (suggesting that the same abnormality possibly underlies both)
• Dopaminergic hypofunction in the dorsolateral prefrontal cortex is associated with negative symptoms
(Howes et al, Reference Howes, Kambeitz and Kim2012)
Increased striatal dopamine synthesis capacity has been demonstrated using PET in those at ultra-high risk for psychosis (Egerton Reference Egerton, Chaddock and Winton-Brown2013; Jauhar Reference Jauhar, Nour and Veronese2017). It appears to precede psychosis and encompasses a further progressive increase in striatal dopamine synthesis capacity with psychosis onset. This may be a valuable neurobiological marker in predicting transition risk (Egerton Reference Egerton, Chaddock and Winton-Brown2013). Changes in dopamine synthesis capacity have been associated not only with the pathogenesis of schizophrenia but also with psychotic symptoms in bipolar disorder (Jauhar Reference Jauhar, Nour and Veronese2017).
Overall, it is clear from these collective data that there is a substantial link between many risk factors for psychosis and dopamine dysregulation as well as between dopamine dysregulation and later symptoms.
Glutamate
Treen et al (Reference Treen, Battle and Mollà2016) systematically reviewed studies that used proton magnetic resonance spectroscopy, a technique that can be used to assess glutamate function. The focus was investigation of N-methyl-d-aspartate (NMDA) receptor hypofunction as a hypothesis for the pathophysiology underlying schizophrenia. Glutamate receptors include NMDA and metabotropic forms, and NMDA receptor dysfunction has been implicated in dopamine dysregulation resulting in higher mesolimbic and lower mesocortical dopamine, showing that these two systems are interlinked. Treen et al’s review of 11 studies found that 6 of them demonstrated significant alterations in glutamate metabolites in the frontal lobes, thalamus and associative striatum in individuals with an at-risk mental state for psychosis. However, the other 5 studies did not report a significant difference in these same areas. One of these studies was conducted by Egerton et al (Reference Egerton, Stone and Chaddock2014), who focused on the thalamus and anterior cingulate cortex regions. Their main finding was that low levels of thalamic glutamate at presentation were associated with poor clinical outcomes in high-risk individuals. This included the worsening of positive symptoms and abnormal thought content. Therefore, they suggest that measures of neurological glutamate function may be useful in predicting clinical outcomes.
NMDA receptors have been linked to processing of information and prediction errors. The proposed theory is that perception is conditional on our individual expectations of a situation. When these are not aligned with reality a prediction error is said to have occurred (Treen Reference Treen, Battle and Mollà2016). Alpha-amino-3-hydroxy-5-methyl-4-isoxazolepropionic acid (AMPA) is involved with processing perceptions and in removing irrelevant information. Alongside this, NMDA is involved in using previously established models to explain stimuli. Dysregulation here is proposed as a pathway underlying psychotic symptom development (Treen Reference Treen, Battle and Mollà2016). Ermakova et al (Reference Ermakova, Knolle and Justicia2018) further investigated prediction errors, finding disrupted reward prediction error signalling in the dopaminergic midbrain in those at risk for psychosis, present at an intermediate level between controls and individuals with first-episode psychosis.
Prediction-error signalling can be assessed by looking at mismatch negativity (MMN) and event-related potential through EEG measurements (Treen Reference Treen, Battle and Mollà2016). Auditory MMN, for example, is elicited automatically when a heard sound is different in a series of repeated standard sounds. MMN amplitude is higher the more the sound deviates from the standard sound and this is thought to reflect echoic memory, as detection of the deviant sound relies on short-term memory of the preceeding sounds (Perez Reference Perez, Woods and Roach2014). In the studies by both Treen et al (Reference Treen, Battle and Mollà2016) and Perez et al (Reference Perez, Woods and Roach2014) reduced amplitude was found in those with schizophrenia. Furthermore, reduced MMN has been observed in those at high risk for psychosis, and at an even higher rate in those who subsequently develop psychotic illness. Perez et al (Reference Perez, Woods and Roach2014) quantified this by calculating that a 1 unit decrease in a specific type of MMN (double deviant MMN) was associated with a 1.63-fold increase in risk of conversion to psychosis. It is therefore possible that subsets of these measurements may be of use in the future to help predict psychosis development (Perez Reference Perez, Woods and Roach2014; Pantlin Reference Pantlin and Davalos2016).
Cortisol
Stress has been repeatedly implicated across our three-part series of articles as a factor that increases vulnerability to, or acts as a precipitating factor for, psychosis. Increased cortisol levels have been linked with social and environmental factors and psychosis via hypothalamic–pituitary–adrenal (HPA) axis dysregulation (Chaumette Reference Chaumette, Kebir and Man-Lam-Fook2016). Prolonged exposure to high cortisol levels is further associated with dysregulation in neurotransmission (Chaumette Reference Chaumette, Kebir and Man-Lam-Fook2016). Elevated levels of cortisol impair explicit memory as well as affect hippocampal volume. This is paralleled in post-traumatic stress disorder, where deficits in hippocampal-dependent memory can be observed. Changes in memory and in the hippocampus provide a link to the cognitive deficits often observed in clinical schizophrenia (Clarke Reference Clarke, Tanskanen and Huttunen2013). Stress further increases the long-term depression of neurons in the hippocampus via NMDA receptor activation (Keshavan Reference Keshavan, Mehta and Padmanabhan2015).
Meta-analysis by Chaumette et al (Reference Chaumette, Kebir and Man-Lam-Fook2016) demonstrated that levels of basal salivary cortisol were increased in ultra-high-risk individuals compared with controls. Similarly, Labad et al (Reference Labad, Stojanovic-Pérez and Montalvo2015) showed differences in the baseline cortisol secretion pattern after waking in those who later transitioned to psychosis. Debate continues regarding cortisol and whether it is a marker of anxiety and distress associated with emerging psychosis or has a causative role. Chaumette et al (Reference Chaumette, Kebir and Man-Lam-Fook2016) suggest that confounding factors, particularly medication intake, could be having an effect on these results and that cortisol levels themselves may therefore not be a reliable biomarker for psychosis risk at this stage.
Labad et al (Reference Labad, Stojanovic-Pérez and Montalvo2015) considered prolactin and albumin alongside cortisol in those with an at-risk mental state. Prolactin is primarily regulated by inhibition from dopamine D2 receptors and is known to increase in response to psychosocial stress. Prolactin has been shown to be raised in those with either first-episode psychosis or an at-risk mental state. If prolactin is raised, dopamine response increases in an attempt to inhibit further prolactin release and restore balance (Labad Reference Labad, Stojanovic-Pérez and Montalvo2015; Ittig Reference Ittig, Erich and Heitz2017). Trying to quantify this, Ittig et al (Reference Ittig, Erich and Heitz2017) demonstrated that hyperprolactinemia was present in 32% of their at-risk mental state participants and 35% of their first-episode psychosis participants, compared with 2.5% in the baseline population. Female participants had higher prolactin levels than their male counterparts (Labad Reference Labad, Stojanovic-Pérez and Montalvo2015; Ittig Reference Ittig, Erich and Heitz2017). Albumin, on the other hand, has a positive influence on regulation of inflammation. Reduced levels have been linked to multiple psychiatric diagnoses, including psychotic disorders. Overall, Labad et al (Reference Labad, Stojanovic-Pérez and Montalvo2015) demonstrated that, although increased prolactin was a predictor of psychosis transition, albumin had a statistically significant protective effect.
Pathway 3: inflammation
The last pathway considered in our three articles is the inflammatory pathway and we have focused on how risk factors influence it. For example, in parts 1 and 2 (Romain Reference Romain, Eriksson and Onyon2019a, Reference Romain, Eriksson and Onyon2019b) we considered the role of infection and pollution, and throughout have considered the role of stress. This is the most recently developed of the three primary pathways considered and is the focus of a large body of ongoing research.
Metcalf et al (Reference Metcalf, Jones and Nordstrom2017) investigated the role of inflammation in their study of C-reactive protein (CRP). Raised levels of CRP in adolescents are associated with subsequent development of schizophrenia and this appears to be in a dose–response relationship. In a comparison of high (>3mg/L) and low (<1mg/L) baseline CRP levels, individuals with high levels had an odds ratio of developing schizophrenia of 4.25 (95% CI 1.30–13.93); evidence also suggested that higher CRP might be associated with an earlier onset (Metcalf Reference Metcalf, Jones and Nordstrom2017).
Zeni-Graiff et al (Reference Zeni-Graiff, Rizzo and Mansur2016) discovered higher levels of interleukin 6 (IL-6) and decreased interleukin 17 (IL-17) in their study of an ultra-high-risk group, suggesting a proinflammatory profile and immunological imbalance. This was present in both early psychosis and at-risk stages. IL-6 has been shown to be involved in active inflammatory processes and crosses the blood–brain barrier. IL-17 is linked with inflammation, autoimmunity and some neurological disorders, and IL-17 alterations potentially modify brain structures, increasing psychosis vulnerability. This work linked higher levels of IL-17 with lower symptom burden. These and some of the other markers discussed here are summarised in Fig. 2.

FIG 2 Inflammatory markers associated with increased risk for psychosis. IL, interleukin; sFlt-1, soluble FMS-like tyrosine kinase.
Karanikas et al (Reference Karanikas, Manganris and Ntouros2017) demonstrated variation in cytokine profiles between controls, ultra-high-risk and first-episode psychosis groups. In the first-episode psychosis group there were higher pro-inflammatory cytokines (tumour necrosis factors TNF-α and TNF-β, and interferon-gamma IFN-γ) and an increase in IL-4, an anti-inflammatory cytokine. In their ultra-high-risk sample they noted only an increase in IL-4 compared with controls. These findings suggest that there may be activation in different areas of the immune system once transition to psychosis has occurred (Karanikas Reference Karanikas, Manganris and Ntouros2017). Contrary to the work by Karanikas et al (Reference Karanikas, Manganris and Ntouros2017), in which a raised IFN-γ was identified, a reduced level was found in the first-episode psychosis group in the work of Lizano et al (Reference Lizano, Keshavan and Tandon2016)). This emphasises that work is still needed to clarify the cytokine profiles more conclusively.
Lizano et al (Reference Lizano, Keshavan and Tandon2016) found that an anti-angiogenesis factor (soluble FMS-like tyrosine kinase, sFlt-1) was increased in the familial high-risk group. This factor can sensitise endothelial cells to inflammatory signals, making cells more susceptible to damage. It is also linked with vascular endothelial growth factor (VEGF), a factor involved in neurogenesis regulation. There have been links between sFlt-1 and obstetric factors such as low birthweight and pre-eclampsia (discussed in Romain Reference Romain, Eriksson and Onyon2019a). Overall, Lizano et al suggest an activation of the angiogenic cascade in familial high-risk individuals that persists in first-episode psychosis. This demonstrates that there is a link between immunological dysfunction, angiogenesis dysfunction and increased psychosis risk.
The complement system, a mediator of innate and acquired immunity, has been implicated in both the inflammatory and neurodevelopmental theories of schizophrenia development. This system has a role not only in the immune system but also in synaptic pruning. Genome-wide studies have demonstrated polymorphisms in the complement 4A and 4B genes, suggesting these as risk factors for psychosis via dysregulation in the complement pathways (Nimgaonkar Reference Nimgaonkar, Prasad and Chowdari2017). Another factor that has more recently been implicated is γ-glutamyl transferase (GGT), usually used as a marker of liver damage, but now linked to oxidative stress and the inflammatory pathways thought to underlie psychosis. However, it is currently unclear whether this is involved in pathogenesis or is simply a marker of inflammatory pathology (Kunutsor Reference Kunutsor and Laukkanen2017).
The role of autoimmune diseases as a cause of psychosis is a topic of current interest. A subgroup of patients have been found to have autoantibodies against neurotransmitter receptors and ion channels, for example autoimmune NMDA receptor antibodies, resulting in psychotic symptoms and neurological deterioration. Identifying this subgroup presents a challenge, but these autoantibodies are another potentially causative factor contributing to psychosis; many more autoantibodies are now under investigation (Ellul Reference Ellul, Groc and Tamouza2017). Further supporting this link is the increase in autoimmune disease found in the relatives of those with schizophrenia (Kočovskà Reference Kočovskà, Gaughran and Krivoy2017).
Models of psychosis
Our series of articles has provided a narrative chronological overview of potential and known risk factors for psychosis from which we have produced the psychosis risk timeline shown in Fig. 3. Other models of psychosis exist alongside this and psychosis can be viewed from many different perspectives. Howes & Murray (Reference Howes and Murray2014), for example, proposed a sociodevelopmental–cognitive model, positing that developmental changes occur as a result of genetic and childhood factors that sensitise the dopamine system. Social adversity links into this, with altered cognitive schemas resulting in, and further causing, stress. A cycle is established, with stress increasing dopamine changes, leading to altered perceptions and so more stress, and resulting in the development of increasingly psychotic interpretations. This model best fits with our present discussion on risk factors as well as our risk timeline structure, since it further accounts for the progressive changes seen throughout the prodromal period, fluctuations in symptoms and links with other disorders (Howes Reference Howes and Murray2014).

FIG 3 The psychosis risk timeline: factors over the lifespan that can affect the risk for psychosis.
Prevention
To ensure a more comprehensive review, we have included in this three-part narrative review recent research studies which have a variety of outcome measures. We have included not only work that demonstrates a direct link between a risk factor for psychosis and development of psychosis itself, but also studies in which the outcome was an increased likelihood of being described as ‘at high risk of developing psychosis’. Every effort has been made to make this distinction clear. Psychosis is a broad concept and, although our overall timeline helps structure this series and provide a simplified overview, it would not be possible to include all of the many overlaps and interactions within this model.
It is hoped that by including such a range of research, with a focus on the most up-to-date studies, we enable the reader to gain an overview of psychosis and the many factors contributing to risk, forming a basis for further exploration of this topic.
Bringing together the evidence considered throughout this series we will now suggest some of many possible preventive strategies based on these factors, as prevention has been recognised as a key factor for the ongoing care of patients (Schizophrenia Commission 2012). Although this section is organised into a primary, secondary and tertiary structure, some suggestions will apply to multiple categories.
Primary prevention
Primary prevention focuses on avoiding the development of psychosis. Proposed strategies based on the evidence discussed in this series are summarised in Box 2. Of these strategies we primarily suggest an increase in interventions to reduce environmental factors conferring a higher risk of psychosis: for example, improving access to healthcare, good nutrition, and psychological and social support structures.
BOX 2 Primary preventive strategies
• Adequate childhood nutrition
• Good access to obstetric care
• Interventions to reduce childhood infection
• Greater resources to support those experiencing stress
• Greater resources to support good social networks and social support
• Substance misuse education
• Improved access to specialist substance misuse treatment, including psychological therapy
• Improving genetic testing utility to support targeting of primary prevention
A recurring theme identified in our review is the effect that stressors have on risk for psychosis. Therefore, we propose that overall there is a good argument to be made for improved access to support services for problems such as child abuse and bullying and general societal improvements to socioeconomic environments to reduce other stressors, such as poverty. Furthermore, supporting communities in developing good social networks, particularly for those in high-risk groups (such as refugees), will be highly important. These interventions would likely be beneficial not only for reducing psychosis risk but also more widely for communities and public health as a whole.
We advocate ongoing targeted interventions to reduce substance misuse, in light of our review of this evidence in part 2 (Romain Reference Romain, Eriksson and Onyon2019b). Although research in this area is difficult, owing to the nature of the substances and ethical considerations, overall there is significant evidence to link many substances with increased psychosis risk. Continuing to educate the public and provide improved access to substance misuse treatment, including psychological therapy, is likely to be of benefit.
Finally, although it may not be possible to alter underlying genetics, as the clinical utility of genetic testing and polygenic risk scoring improves we may be able to better target interventions and preventive strategies to avoid compounding existing risk factors. However, this is an area in which significant further research is needed and there are many ethical considerations that will need careful navigation.
Secondary prevention
Once symptoms manifest, the focus changes to trying to prevent transition and reduce symptom burden. Some strategies that could be targeted are summarised in Box 3.
BOX 3 Secondary preventive strategies
• Support with social interactions
• Developing coping strategies
• Stress-reduction strategies
• Early psychosocial support
• Comprehensive medical assessment
As with the primary preventive strategies, there is a focus on improving social and psychological support, including working to improve self-perceptions and coping strategies. However, here we further suggest that, once symptoms manifest, improved physical health assessments will help identify organic causes and decide treatment plans. Work by Giannitelli et al (Reference Giannitelli, Consoli and Raffin2018) and Ellul et al (Reference Ellul, Groc and Tamouza2017) suggests that these assessments should consider screening for autoantibodies and inborn errors of metabolism. Although this is unlikely to be necessary in every case, we believe that more frequent testing for these could identify patients who need alternative treatment plans.
Tertiary prevention
Tertiary prevention considers what can be done once psychosis is diagnosed, to minimise symptom burden as well as the frequency and severity of relapse. Suggested strategies are outlined in Box 4.
BOX 4 Tertiary preventive strategies
• Improved screening for psychiatric comorbidity
• Improved assessment of auditory and visual impairment
• Further assessment and treatment of sleep dysfunction
• Screening for comorbid physical illness
• Psychoeducation
• Improved social and family support
• Focus on improving community environments
• Treatment of comorbid substance misuse
• Further interventions to reduce stigma and discrimination
Alongside conventional psychosis treatment we advocate a move towards an improved system for screening for comorbidities as part of both secondary and tertiary prevention. It is important to consider psychiatric comorbidities. However, we also suggest assessing for auditory and visual impairment and sleep dysfunction. This may open up new avenues of treatment: for example, Lunsford-Avery et al (Reference Lunsford-Avery, LeBourgeois and Gupta2015) reported that cognitive–behavioural therapy with an insomnia focus could be considered. More data are needed on the utility of this method, and longitudinal outcomes and the effect of treatment on psychosis need further assessment.
Alongside this, psychoeducation and support not only for the patient but their family is important. Targeting, for example, emotion recognition skills may support psychological treatment strategies. It may also be possible to work towards improving neuroplasticity via cognitive training, non-invasive brain stimulation, medication and exercise (Keshavan Reference Keshavan, Mehta and Padmanabhan2015). However, not all of these strategies are commonly available at present.
To further support patients with a psychosis diagnosis, early symptoms or as part of primary prevention, interventions to reduce stress remain highly important. Previously (Romain Reference Romain, Eriksson and Onyon2019b) we discussed the role of stigma, and large-scale work to reduce both stigma and discrimination regarding mental health problems and psychosis will reduce distress in this population.
Current preventive work
Some of our suggestions are already accessible to patients in the UK through wider public health measures or services that target secondary and tertiary prevention, such as the early intervention and recovery or rehabilitation psychosis services. Therefore, we would suggest that ensuring these teams are well resourced to help them to meet these targets could be beneficial. Although it is not within the remit of this article to provide a full review of the effectiveness of these services we will give brief summary here.
The National Institute for Health and Care Excellence reports that early intervention services are evidence based, cost-saving, and preferred by patients and carers (National Collaborating Centre for Mental Health 2016). Similarly, the Department of Health (2011) states that intervening at an earlier point can improve long-term mental health health and well-being and that early intervention services should be prioritised. For psychosis it states that early intervention promotes employment and education and reduces relapse risk. Importantly, it makes an economic case for early intervention in both the first-episode and at-risk periods (Department of Health 2011).
Neale & Kinnair (Reference Neale and Kinnair2017) considered some key studies of early intervention services and felt overall that outcomes were better for patients receiving early intervention service care, in part owing to factors such as lower caseloads and so more intensive support. However, they call for evidence on longer-term outcomes. Although it appears logical that early intervention services would produce improved outcomes, recent research findings, such as the systematic review by Aceituno et al (Reference Aceituno, Vera and Prina2019), were not able to clearly support or oppose such services as a cost-effective strategy. Davies et al (Reference Davies, Radua and Cipriani2018) in their meta-analysis suggest that sample heterogeneity makes analysis of research in this area difficult and that more personalised interventions and research into factors predictive of treatment response are needed, as there is no clearly superior strategy emerging for early treatment.
Further to this, some studies have demonstrated underwhelming results. One such is an analysis of an ‘at-risk mental state’ clinic (Ajnakina Reference Ajnakina, David and Murray2019). Although in this specific study a significant limitation was noted to be the clinic's ability to reach its target population. This team, contrary to Davies et al (Reference Davies, Radua and Cipriani2018), who suggest more personalised work, instead suggest broader public health strategies targeting environmental factor exposure. Overall, therefore, the best way forward remains controversial.
Clinical staging
Clinical staging of the psychosis prodromal period has become more promising and may help in the future to reduce sample heterogeneity in research studies and allow for more personalised treatment planning. Carrión et al (Reference Carrión, Correll and Auther2017), for example, divided the psychosis prodromal period into four stages with a focus on symptom severity. Importantly, they were able to demonstrate differing transition rates for their subgroups. Contrasting this is the clinical staging model for psychosis in the Australian clinical guidelines for early psychosis (Early Psychosis Guidelines Writing Group 2016). In this model the authors describe stages of both prodromal and later psychosis, in this case with four stages consisting of eight subcategories, ranging from premorbid function to severe and persistent psychotic phenomena. They also consider factors such as duration of symptoms. Although both models require further consolidation, it may prove beneficial to consider the prodrome not as a single entity, and instead to consider risk factors and preventive strategies at different clinical stages.
Conclusions
Across this series of three articles, we have identified significant risk factors for psychosis in a chronological format, producing the psychosis risk timeline model in Fig. 3. This provides a simplified overview structure to a highly complex and interlinked topic, supporting the reader to navigate the wider field of psychosis. This proposed model includes a wide range of risk factors, both for schizophrenia and more broadly for psychosis and the associated at-risk states. Furthermore, the model includes the three primary pathways through which risk factors are thought to influence psychosis development, including newer knowledge of inflammatory components.
This series is limited in its application as it is a largely narrative review. Given the scale and complexity of the topic we have focused on recent publications to ensure up-to-date data. The simplified model proposed intends to provide a structure on which clinicians and future clinicians practising in this area can develop their understanding and begin to access the wide range of ongoing research. The best strategy for future work remains controversial, and experts in this field are continuing to conduct work and review how best to implement prevention in wider healthcare policy.
In summary, on the basis of current knowledge, we suggest that preventive work is possible. In particular, a focus on social and environmental interventions is likely not only to reduce the impact of risk factors on psychosis but to have broader implications for public health. However, we suggest that our preventive strategy suggestions be subject to further formal systematic work focused on individual strategies and assessment of utility, acceptability and cost-effectiveness to support effective implementation of the best strategies. Overall, in advocating for further development of these strategies, it is hoped that in the future psychosis risk factors can be targeted and successfully reduced.
MCQs
Select the single best option for each question stem
1 A reduced incidence of which type of orbitofrontal cortex pattern has been associated with transition to psychosis?
a type 1
b type 2
c type 3
d type 4
e type 5.
2 When considering EEG data, which of the following has been noted in those at high risk for psychosis?
a slower alpha peak frequencies with reduced mismatch negativity amplitude
b slower alpha peak frequencies with increased mismatch negativity amplitude
c higher alpha peak frequencies with reduced mismatch negativity amplitude
d higher alpha peak frequencies with increased mismatch negativity amplitude
e absence of alpha peak frequencies altogether.
3 Which of the following is not an alteration in dopamine thought to be linked with psychosis?
a higher resting dopamine levels
b increased dopamine synthesis capacity
c primarily postsynaptic alterations
d higher dopamine release following psychological stress
e reduced baseline occupancy of D2 and D3 receptors.
4 The proportion of individuals with an at-risk mental state for psychosis shown to have raised prolactin levels is approximately:
a 10%
b 18%
c 27%
d 32%
e 43%.
5 The levels of all of the following inflammatory markers is elevated in psychosis, except:
a IL-4
b CRP
c IL-6
d IL-17
e sFlT-1.
MCQ answers
1 a 2 a 3 c 4 d 5 d
eLetters
No eLetters have been published for this article.