Introduction
Despite advances in treatments, many obsessive–compulsive disorder (OCD) patients have reported poor treatment responses mainly due to heterogeneity of the disease (Lack, Reference Lack2012; Rosa-Alcazar, Sanchez-Meca, Gomez-Conesa, & Marin-Martinez, Reference Rosa-Alcazar, Sanchez-Meca, Gomez-Conesa and Marin-Martinez2008). OCD is considered clinically heterogeneous in that many OC symptoms, such as washing, checking, or ordering, may meet the diagnostic criteria for the disorder, and various other phenotypic differences are also evident in OCD patients (Taylor, Reference Taylor2011), making it difficult to identify pure characteristics of the disorder. The Research Domain Criteria project also addresses the need for the identification of more homogeneous subgroups of patients with psychiatric disorders using biological evidence (Insel, Reference Insel2014; Wardenaar & de Jonge, Reference Wardenaar and de Jonge2013). To date, several useful subtyping methods have been proposed to identify more homogeneous OCD subgroups to better understand complexities of the disorder (Leckman et al., Reference Leckman, Denys, Simpson, Mataix-Cols, Hollander, Saxena and Stein2010), and evidence suggests that age of onset serves as an important subtyping scheme for reliably distinguishing OCD populations (Taylor, Reference Taylor2011).
The onset age of OCD is bimodally distributed, with an early peak in childhood or early adolescence before puberty and a late peak in early adulthood, suggesting distinct subtypes between early- and late-onset OCD (Geller et al., Reference Geller, Biederman, Jones, Park, Schwartz, Shapiro and Coffey1998; Swedo, Rapoport, Leonard, Lenane, & Cheslow, Reference Swedo, Rapoport, Leonard, Lenane and Cheslow1989). Early-onset OCD patients are known to have onset of subclinical symptoms before puberty, while late-onset OCD patients are likely to show obsessive and compulsive symptoms after puberty (Sobin, Blundell, & Karayiorgou, Reference Sobin, Blundell and Karayiorgou2000). Using this criterion, 17 years has been suggested as a cutoff onset age for classifying two OCD patient subgroups approximately at puberty and has been used in many studies including our previous work (Butwicka & Gmitrowicz, Reference Butwicka and Gmitrowicz2010; Fontenelle, Mendlowicz, Marques, & Versiani, Reference Fontenelle, Mendlowicz, Marques and Versiani2003; Henin et al., Reference Henin, Savage, Rauch, Deckersbach, Wilhelm, Baer and Jenike2001; Hesse et al., Reference Hesse, Stengler, Regenthal, Patt, Becker, Franke and Sabri2011; Hwang et al., Reference Hwang, Kwon, Shin, Lee, Kim and Kim2007; Kang, Kim, Kim, Hwang, & Kim, Reference Kang, Kim, Kim, Hwang and Kim2017; Kim et al., Reference Kim, Kwak, Hur, Lee, Shin, Lee and Kwon2020; Wang et al., Reference Wang, Cui, Wang, Fan, Xu, Qiu and Xiao2012). Previous systematic reviews and meta-analyses have reported that patients with early-onset OCD are reliably differentiated from those with late-onset OCD in terms of multiple etiologic and phenotypic factors, with early-onset OCD patients showing a higher prevalence in males, higher levels of genetic loading and heritability, higher comorbidity rates with other neurodevelopmental diseases such as tics and Tourette's syndrome, poorer treatment responses, and a more gradual appearance of symptoms than patients with late-onset OCD, suggesting that early-onset OCD patients have more neurodevelopmental loading than late-onset OCD patients (Geller et al., Reference Geller, Biederman, Jones, Park, Schwartz, Shapiro and Coffey1998; Taylor, Reference Taylor2011). In addition to these well-known clinical comparisons, neurobiological differences have also been evidenced in subtypes of OCD based on onset age (Boedhoe et al., Reference Boedhoe, Schmaal, Abe, Ameis, Arnold, Batistuzzo and van den Heuvel2017; Jurng et al., Reference Jurng, Park, Kim, Park, Moon, Lho and Kwon2021; Kim et al., Reference Kim, Kwak, Hur, Lee, Shin, Lee and Kwon2020), suggesting that there are distinct pathophysiological mechanisms in early- and late-onset OCD groups. However, the underlying neural markers to establish neurodevelopmental differences between patients with early- and late-onset OCD have rarely been investigated, although they need to be identified to subtype OCD patients into more biological evidence-based homogeneous subgroups to appropriately understand the neurobiological underpinnings of different etiologies.
Brain gyrification is mostly determined in the last two trimesters of pregnancy, and its pattern remains relatively stable throughout life, making it a reliable marker for early neurodevelopmental deficits in psychiatric illnesses (Armstrong, Schleicher, Omran, Curtis, & Zilles, Reference Armstrong, Schleicher, Omran, Curtis and Zilles1995; Zilles, Palomero-Gallagher, & Amunts, Reference Zilles, Palomero-Gallagher and Amunts2013). Based on the concept that neurodevelopmental risk factors may play key roles in OCD pathophysiology (Huyser, Veltman, de Haan, & Boer, Reference Huyser, Veltman, de Haan and Boer2009; Rosenberg & Keshavan, Reference Rosenberg and Keshavan1998), gyrification has been previously investigated in OCD patients to determine whether early developmental alterations exist. However, previous findings on gyral patterns have been inconsistent, with some studies showing increased gyrification and others showing decreased gyrification, although the regions with abnormal gyrification were somewhat consistent in OCD patients. These conflicting findings were mainly due to differences in the methods used to measure gyrification. The first two studies reported reduced gyrification in the anterior cingulate gyrus (ACC) (Shim et al., Reference Shim, Jung, Choi, Jung, Jang, Park and Kwon2009) and prefrontal cortex (Wobrock et al., Reference Wobrock, Gruber, McIntosh, Kraft, Klinghardt, Scherk and Moorhead2010) in OCD patients compared to healthy controls (HCs) using a two-dimensional (2D) approach of assessing gyrification in regions of interest (ROIs). However, this previously adapted method has several limitations in that the surface perimeter is biased by slice orientation and inclusion of buried sulci, and it is impossible to precisely localize gyral abnormalities in sublobar regions, which raises questions of accuracy and reproducibility (Schaer et al., Reference Schaer, Cuadra, Tamarit, Lazeyras, Eliez and Thiran2008).
Subsequent studies used three-dimensional (3D) vertexwise analyses of the local gyrification index (lGI), which enables a non-biased estimation of whole-brain vertexwise morphometric changes across all of the sulcogyral regions in the cerebral cortex. However, conflicting results continued to be reported. While Venkatasubramanian et al. (Reference Venkatasubramanian, Zutshi, Jindal, Srikanth, Kovoor, Kumar and Reddy2012) found no significant differences using an ROI-based lGI approach, both Fan et al. (Reference Fan, Palaniyappan, Tan, Wang, Wang, Li and Liddle2013) and Rus et al. (Reference Rus, Reess, Wagner, Zaudig, Zimmer and Koch2017) found hyper- and hypogyrification, respectively, in similar regions of the frontoparietal cortex using whole-brain lGI analysis. These heterogeneous results among lGI studies could be partly explained by several confounding factors, such as illness severity and medication status, because these factors could possibly affect cortical morphology (Fan et al., Reference Fan, Palaniyappan, Tan, Wang, Wang, Li and Liddle2013). In these two previous studies using lGI in the whole-brain, which was the same method we used, only unmedicated or drug-naive OCD patients showed increased gyrification. Importantly, such inconsistent results raise the likelihood that there are neurodevelopmentally distinct subtypes in OCD, highlighting the significance of investigating gyrification differences in separate subgroups rather than investigating gyrification in a whole OCD population compared to HCs. Since abnormal gyrification reflects early neurodevelopmental cortical deficits (Armstrong et al., Reference Armstrong, Schleicher, Omran, Curtis and Zilles1995; Zilles et al., Reference Zilles, Palomero-Gallagher and Amunts2013), investigating gyrification differences between the early- and late-onset OCD subgroups may help establish more biological evidence-based homogeneous subtypes in OCD that aligns with neurodevelopmental perspectives.
Furthermore, among the various neurocognitive function impairments reported in OCD (Shin, Lee, Kim, & Kwon, Reference Shin, Lee, Kim and Kwon2014), visual memory and verbal fluency deficits are persistently observed during pharmacological treatment (Kim, Park, Shin, & Kwon, Reference Kim, Park, Shin and Kwon2002; Roh et al., Reference Roh, Shin, Kim, Ha, Shin, Lee and Kwon2005) and cognitive behavioral therapy (Vandborg, Hartmann, Bennedsen, Pedersen, & Thomsen, Reference Vandborg, Hartmann, Bennedsen, Pedersen and Thomsen2015) despite significant improvements in OC symptoms. That these neuropsychological deficits are still apparent in fully recovered OCD patients and their unaffected relatives (Rao, Reddy, Kumar, Kandavel, & Chandrashekar, Reference Rao, Reddy, Kumar, Kandavel and Chandrashekar2008; Zartaloudi, Laws, & Bramon, Reference Zartaloudi, Laws and Bramon2019) further supports the hypothesis that these impairments are trait-related markers for OCD rather than state-dependent features of the disease. In fact, neuropsychological differences in visual memory and verbal fluency have been consistently reported between the early- and late-onset OCD subtypes (Hwang et al., Reference Hwang, Kwon, Shin, Lee, Kim and Kim2007; Kim et al., Reference Kim, Kwak, Hur, Lee, Shin, Lee and Kwon2020; Roth, Milovan, Baribeau, & O'Connor, Reference Roth, Milovan, Baribeau and O'Connor2005), suggesting that these deficits are prominent neurocognitive features reflecting effects of neurodevelopmental abnormalities on cortical gyrification that distinguish OCD into more homogenous subgroups.
In this study, we investigated cortical gyrification differences in OCD subgroups based on age of onset compared to HCs to identify potential neurobiological markers that can be used to subdivide neurodevelopmentally homogeneous subtypes in OCD. Since our OCD patients were all drug-naïve or unmedicated, and early-onset OCD patients are likely to have higher neurodevelopmental loading according to previous studies, it was hypothesized that patients with early-onset OCD would exhibit hypergyrification in frontoparietal and cingulate regions compared to late-onset OCD patients and HCs. Furthermore, to strengthen the evidence regarding the effects of neurodevelopmental deficits on abnormal gyrification, neurocognitive functions, such as visuospatial memory and verbal fluency, which are suggested to be trait-related impairments in OCD and prominent neurocognitive features that show differences between neurodevelopmental subtypes of OCD, were compared among OCD subgroups and the HC group, and the associations between these neurocognitive impairments and clusters with significant differences in gyrification were explored in exploratory analyses.
Methods
Participants
A total of 177 OCD patients and 152 HCs participated in this study, and their T1 magnetic resonance imaging (MRI) data were used in our previous studies (Jurng et al., Reference Jurng, Park, Kim, Park, Moon, Lho and Kwon2021; Kim et al., Reference Kim, Kwak, Hur, Lee, Shin, Lee and Kwon2020). Patients with OCD were recruited from the OCD clinic of the Department of Psychiatry at Seoul National University Hospital (SNUH, Seoul, South Korea). Patients were diagnosed with OCD based on the Diagnostic and Statistical Manual of Mental Disorders, fourth edition (DSM-IV) by experienced psychiatrists. The severity of obsessive–compulsive symptoms was evaluated in patients using the Yale–Brown Obsessive Compulsive Scale (Y-BOCS) (Goodman et al., Reference Goodman, Price, Rasmussen, Mazure, Fleischmann, Hill and Charney1989). OCD patients were also administered with the Hamilton Depression Rating Scale (HAM-D) (Hamilton, Reference Hamilton1960) and the Hamilton Anxiety Rating Scale (HAM-A) (Hamilton, Reference Hamilton1959) to assess the severity of depression and anxiety, respectively. All patients were either drug-naïve or unmedicated for at least 1 month at the time of inclusion (78 drug-naïve and 99 unmedicated). We designated the cutoff onset age to be 17 years, classifying the two OCD subgroups approximately at puberty to be consistent with previous studies: early-onset OCD (age at onset <17 years; n = 84) and late-onset OCD (age at onset >17 years; n = 84) (de Mathis et al., Reference de Mathis, do Rosario, Diniz, Torres, Shavitt, Ferrao and Miguel2008). Data from nine OCD patients with an onset age of 17 years were excluded from further analysis. Psychiatric comorbidities were assessed according to the International Classification of Diseases, 10th edition (ICD-10): 6 OCD patients had a diagnosed comorbidity of major depressive disorder; 2 patients had dysthymic disorder; 39 patients had depressive disorder, not otherwise specified (NOS); 3 patients had bipolar II disorder; 1 patient had bipolar disorder, NOS; 4 patients had tic disorder; and 10 patients had personality disorders. HCs were recruited via internet advertisements and screened for the presence of psychiatric disorders or symptoms using the Structured Clinical Interview for DSM-IV–Non-Patient Version (SCID-NP). Potential HCs were excluded if they reported past or current axis-I diagnoses. The intelligence quotient (IQ) of all participants was evaluated with the Korean version of the Wechsler Adult Intelligence Scale (K-WAIS) to estimate intelligence. The exclusion criteria for both the OCD and HC groups included a lifetime history of any major psychotic disorders, substance abuse or dependence (except nicotine), neurological disease or clinically important head injury, and intellectual disability (IQ < 70).
Written informed consent was obtained from all participants and from the parents of subjects under 18 years of age after the procedures had been fully explained (IRB nos. H-1201-008-392, H-1110-009-380, and H-1503-045-655). The present study was also approved by the Institutional Review Board at Seoul National University Hospital (IRB no. H-2101-053-1188).
Neurocognitive function tests
Visuospatial memory and verbal fluency, among the neurocognitive functions that were previously reported to reveal abnormalities in OCD patients compared to HCs (Shin et al., Reference Shin, Lee, Kim and Kwon2014), were examined in 71 early-onset OCD patients, 71 late-onset OCD patients, and 98 HCs.
Visuospatial memory was evaluated using the following Rey–Osterrieth Complex Figure Test (RCFT) (Meyers & Meyers, Reference Meyers and Meyers1995) subsets: immediate recall (IR; 3 min after the copy condition) and delayed recall (DR; 30 min after the copy condition) conditions. The method developed by Meyers and Meyers was used to rate response times and accuracy in each condition (Meyers & Meyers, Reference Meyers and Meyers1995).
To assess verbal fluency, the Korean version of the Controlled Oral Word Association Test (COWA) (Kang, Chin, Na, Lee, & Park, Reference Kang, Chin, Na, Lee and Park2000) was administered. Within 1 min, subjects were required to remember as many words as possible starting with a given letter and belonging to a specific category. The total number of responses for each letter and category was counted and scored.
Image acquisition
High-resolution anatomical T1-weighted (T1) scans were acquired using a Siemens 3T MAGNETOM Trio MRI scanner (Siemens, Erlangen, Germany) from all subjects. A 12-channel head coil and a 3D magnetization-prepared rapid acquisition gradient-echo (MPRAGE) sequence were used for the T1 images with the following imaging acquisition parameters: repetition time (TR), 1670 ms; echo time (TE), 1.89 ms; flip angle, 9°; field of view, 250 mm; voxel size, 1.0 × 0.98 × 0.98 mm3; and 208 slices. For quality control, the obtained MRI images were visually inspected for any artifacts or malformation of brain structures.
Image processing
The preprocessing of the structural T1 images was performed using the FreeSurfer software package (version 5.3.0, http://surfer.nmr.mgh.harvard.edu) in accordance with the standard and automatic reconstruction algorithm (Fischl & Dale, Reference Fischl and Dale2000). This processing stream consists of removal of non-brain tissue, automated transformation to Talairach space, intensity normalization, and segmentation of gray/white matter tissue resulting in a white mesh and a pial mesh. The meshes consist of approximately 150 000 vertices for each hemisphere.
Next, to measure gyrification, the lGI for the entire cortex was computed at each vertex with the FreeSurfer pipeline using the method of Schaer et al. (Reference Schaer, Cuadra, Tamarit, Lazeyras, Eliez and Thiran2008). This method is a vertexwise extension of the classic 2D GI approach, which is the ratio of the inner pial surface to the outer perimeter on coronal sections (Zilles, Armstrong, Schleicher, & Kretschmann, Reference Zilles, Armstrong, Schleicher and Kretschmann1988). The 3D lGI method takes into account the inherent nature of the cortex, which includes buried sulci, and is not restricted by sulcal walls or biased by the orientation or thickness of the slices. This method calculates the amount of cortex buried within the sulcal folds compared with the amount of visible cortex across the whole brain, allowing for the region-specific localization of gyral deficits (Schaer et al., Reference Schaer, Cuadra, Tamarit, Lazeyras, Eliez and Thiran2008).
In brief, the automatic lGI computation creates an outer smoothed surface tightly wrapping the pial surface and estimates 800 overlapping 25 mm spherical 3D ROIs on the smoothed outer surface and their corresponding paired circular ROIs on the pial surface. Then, the lGI at each vertex of the reconstructed cortical surface was measured as the ratio between the smoothed outer perimeter and inner buried contour of the cortex resulting in individual gyrification maps.
Statistical analysis
Demographic variables between the early-onset OCD, late-onset OCD, and HC groups were compared using χ2 tests or one-way analysis of variance, and the neurocognitive variables were compared using analysis of covariance (ANCOVA) with age as a covariate, followed by post hoc Bonferroni tests, in Statistical Package for Social Sciences (IBM SPSS Version 22). Clinical variables were compared between the early- and late-onset OCD groups with χ2 tests or independent t tests.
A whole-brain lGI analysis for T1 images was conducted with FreeSurfer. The vertexwise lGI values were mapped on the average template subject (fsaverage) for each participant to generate the contrasts of the analysis in the Query Design Estimate Contrast (QDEC) tool in FreeSurfer. A general linear model for surface-based group analyses controlling for the effect of age as a covariate was conducted to analyze regional differences in gyrification between groups (discrete factors) at each vertex in both hemispheres. The lGI maps were then smoothed with a full-width at half-maximum Gaussian kernel of 5 mm. The cluster-forming threshold of significance was set to p < 0.05, and statistical corrections of clusters for multiple comparisons were conducted by Monte Carlo simulation with 10 000 permutations implemented in FreeSurfer. Sensitivity analyses were performed to control for the effect of duration of illness (DOI) by adding it as an additional covariate. Detailed information on these analyses is included in the Supplementary Methods in the online Supplementary materials.
Pearson's correlation analyses between mean lGI values of clusters showing significant group differences and neurocognitive function test scores were performed in early-onset OCD patients in an exploratory manner.
Results
Participant characteristics
The demographic and clinical characteristics and neurocognitive function test results of the participants are presented in Table 1. There were no significant group differences between groups except for age (F = 26.945, p < 0.001). Based on post hoc results, the early-onset OCD group was younger than both the late-onset OCD group (p < 0.001) and HCs (p = 0.021), and the late-onset OCD group was older than the HCs (p < 0.001). Early-onset OCD patients had a significantly lower onset age (t = −16.188, p < 0.001) (online Supplementary Fig. S1) and longer DOI (t = 5.076, p < 0.001) than late-onset OCD patients. No significant differences in clinical variables, such as symptom severity for obsession and compulsion, depression, and anxiety, and in comorbidities were found between the early- and late-onset OCD groups. Regarding the neurocognitive function test results, there were significant group differences in the RCFT IR (F = 4.615, p = 0.011) and DR (F = 3.631, p = 0.028) measures and the COWA letter (F = 5.473, p = 0.005) and category (F = 6.845, p = 0.001) measures based on ANCOVA with age as a covariate. Based on Bonferroni post hoc analysis results, the two OCD subgroups performed significantly worse than the HC group in the following neurocognitive tests: RCFT IR (p = 0.014), RCFT DR (p = 0.032), COWA letter (p = 0.010), and COWA category (p = 0.005) in the early-onset OCD group; COWA letter (p = 0.043) and COWA category (p = 0.012) in the late-onset OCD group.
Table 1. Characteristics of the participants

OCD, obsessive–compulsive disorder; IQ, intelligence quotient; Y-BOCS, Yale–Brown Obsessive Compulsive Scale; HAM-A, Hamilton Anxiety Rating Scale; HAM-D, Hamilton Depression Rating Scale; NOS, not otherwise specified; ICV, intracranial volume; RCFT, Rey–Osterrieth Complex Figure Test; COWA, Controlled Oral Word Association Test; EO, early-onset OCD; LO, late-onset OCD; HCs, healthy controls.
Data are presented as the mean ± standard deviation.
*p < 0.05; **p < 0.01; ***p < 0.001.
Group comparisons in gyrification
The results of the whole-brain lGI analysis are summarized in Tables 2 and 3. Significantly increased gyrification was observed in patients with early-onset OCD compared to HCs across several regions of the cortex with age as a covariate, including one cluster in the left hemisphere and one cluster in the right hemisphere. The left cluster with a significantly increased lGI (p < 0.001) had a peak vertex located within the precuneus gyrus and covered portions of the precentral, postcentral, precuneus, paracentral, posterior cingulate, superior frontal, and caudal anterior cingulate gyri. The right cluster with a significantly increased lGI (p < 0.001) had a peak vertex located within the paracentral gyrus and comprised of portions of the precentral, postcentral, precuneus, paracentral, posterior cingulate, superior frontal, and caudal anterior cingulate gyri. No region had a significantly reduced lGI in the early-onset OCD group compared to the HC group (Fig. 1a).
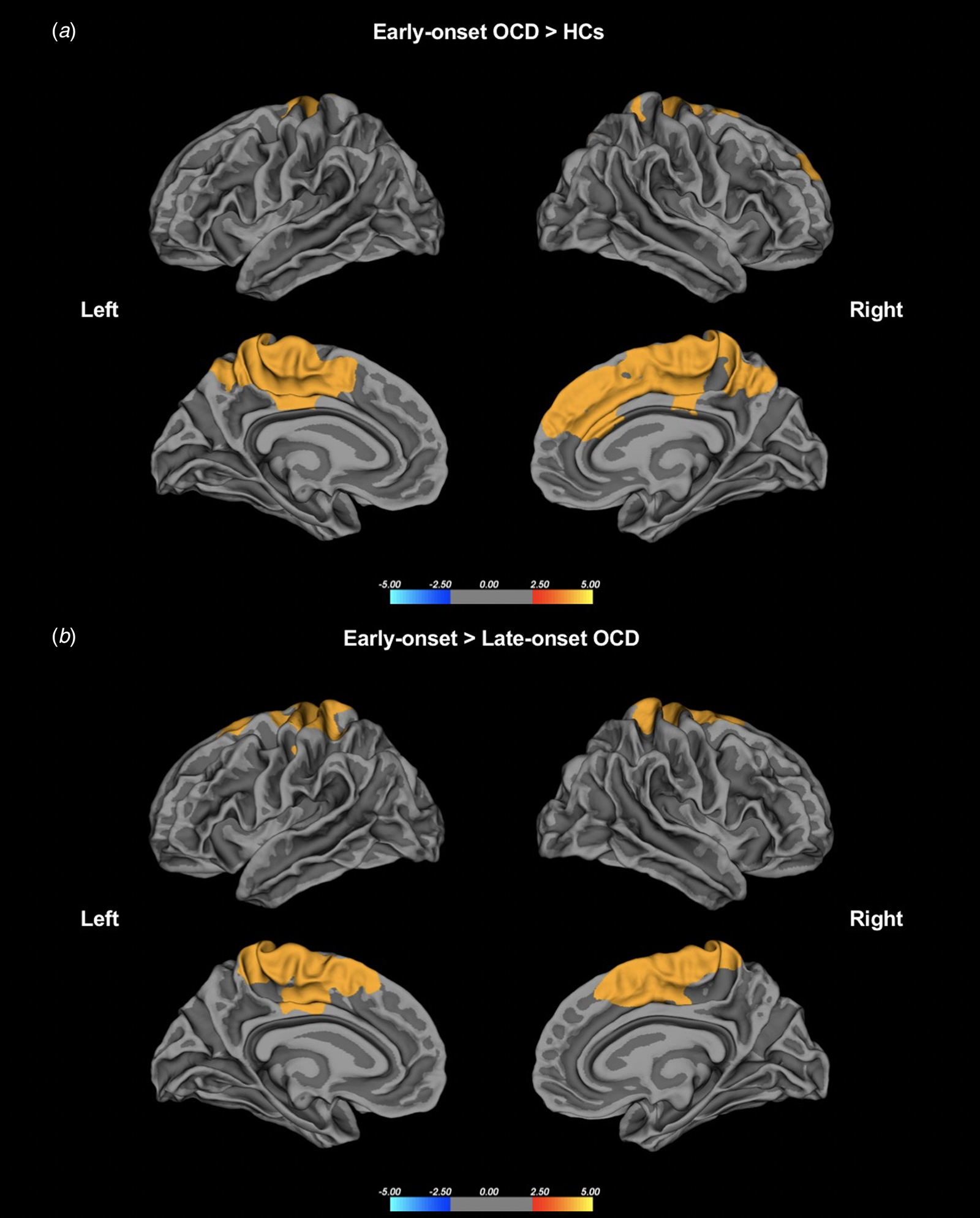
Fig. 1. (a) Group differences in the whole-brain lGI between the patients with early-onset OCD and HCs. (b) Group differences in lGI between the patients with early-onset OCD and those with late-onset OCD. Statistical maps of the left and right hemispheres are shown in the lateral and medial views, respectively. The maps are shown for the clusters with significantly increased lGI in the patients with early-onset OCD after clusterwise correction for multiple comparisons (p < 0.05).
Table 2. Description of clusters with a significantly increased lGI in patients with early-onset OCD compared to HCs in the left and right hemispheres after clusterwise correction for multiple comparisons using Monte Carlo simulation (p < 0.05)

L, left hemisphere; R, right hemisphere; VtxMax, number of peak vertices in the significant cluster; MNI, Montreal Neurological Institute (coordinate system); CWP, clusterwise probability and the nominal p value.
***p < 0.001.
Table 3. Description of clusters with a significantly increased lGI in patients with early-onset OCD compared to patients with late-onset OCD in the left and right hemispheres after clusterwise correction for multiple comparisons using Monte Carlo simulation (p < 0.05)

L, left hemisphere; R, right hemisphere; VtxMax, number of peak vertices in the significant cluster; MNI, Montreal Neurological Institute (coordinate system); CWP, clusterwise probability and the nominal p value.
***p < 0.001.
Significantly increased gyrification was also observed in patients with early-onset OCD compared to patients with late-onset OCD in similar regions, and these clusters had a peak vertex within the left precuneus and right precentral gyri and covered the precentral, postcentral, precuneus, paracentral, posterior cingulate, superior frontal, and caudal anterior cingulate gyri in both hemispheres. No region had a significantly reduced lGI in early-onset OCD patients compared to late-onset OCD patients (Fig. 1b). There were no significant differences between patients with late-onset OCD and HCs. Sensitivity analyses controlling for age and DOI as potential confounding effects showed similar regions of significance in early-onset OCD as the effects found in the main analyses, indicating that DOI did not confound the main results. Details on the regions that remained significant are included in the Supplementary Results, Fig. S2, and Table S1 in the online Supplementary materials.
Associations between lGI and neurocognitive variables
The significantly increased mean lGI values showed correlations with poor neurocognitive function test scores in the early-onset OCD group (Fig. 2). Although these correlations did not survive after multiple comparisons, uncorrected p values are presented as an exploratory analysis. Patients with early-onset OCD showed significant negative correlations between the mean lGI in the left precuneus cluster and both RCFT IR (r = −0.244, p = 0.040) and RCFT DR (r = −0.273, p = 0.021) measures. A negative correlation between the significantly increased mean lGI in the right paracentral cluster and COWA letter scores (r = −0.240, p = 0.044) was also observed in the early-onset OCD group.

Fig. 2. Correlations between the mean lGI values in clusters with significant differences between patients with early-onset OCD and HCs and neurocognitive function test results in the early-onset OCD patients. RCFT, Rey–Osterrieth Complex Figure Test; IR, immediate recall; DR, delayed recall; COWA, Controlled Oral Word Association Test.
Discussion
To identify underlying neurobiological markers that reflect the neurodevelopmental distinctions between OCD subgroups based on age of onset, we investigated gyrification differences between patients with early- and late-onset OCD who were drug-naïve or unmedicated. In this first study reporting gyrification differences in subtypes of OCD using whole-brain lGI, patients with early-onset OCD showed significantly increased gyrification patterns in medial and lateral frontoparietal and cingulate regions bilaterally when compared to those with late-onset OCD and HCs, while the late-onset OCD and HC groups showed no gyrification differences, which was consistent with the notion of greater neurodevelopmental deficits in early-onset OCD patients than those with late-onset OCD. Furthermore, impaired visuospatial memory and verbal fluency were related to higher lGI in both the left precuneus and right paracentral clusters in patients with early-onset OCD, supporting the idea that hypergyrification in frontoparietal and cingulate regions as a potential neurobiological marker may expand upon previous evidence of the neurodevelopmental model of early-onset OCD.
Cortical gyrification is regarded as a marker for early neurodevelopmental defects because its pattern is mainly formed and determined in the last two trimesters of pregnancy and remains relatively stable after birth (Armstrong et al., Reference Armstrong, Schleicher, Omran, Curtis and Zilles1995; Zilles et al., Reference Zilles, Armstrong, Schleicher and Kretschmann1988, Reference Zilles, Palomero-Gallagher and Amunts2013). In fact, gyrification is known to be more resistant to longitudinal changes and state-dependent effects of illness or medication than other structural parameters (Zilles et al., Reference Zilles, Palomero-Gallagher and Amunts2013). In the present study, the early-onset OCD group showed increased gyrification in similar regions when compared to the HC and late-onset OCD groups, while no differences were observed when the entire OCD group was compared to the HC group. Our results are in line with previous findings that reported hypergyrification in the middle frontal cortex extending to the precuneus region in drug-naïve or unmedicated OCD patients (Fan et al., Reference Fan, Palaniyappan, Tan, Wang, Wang, Li and Liddle2013), and it can be inferred that the areas with significant neurodevelopmental differences in this previous study became more evident when OCD patients were subgrouped into neurodevelopmental subtypes and compared to HCs in our study. Our results of hypergyrification in early-onset OCD patients compared to that in late-onset OCD patients in the absence of symptom differences between the two subgroups further support the existence of distinct neurodevelopmental risk factors in the pathogenesis of early-onset OCD that may be predisposing to symptoms of the disorder and may serve as a potential structural marker for the neural correlates of OCD subtypes reflecting neurodevelopmental changes.
In this study, early-onset OCD patients had significantly increased gyrification in the frontoparietal and cingulate cortex, which are known as major component regions in the cortico–striato–thalamo–cortical (CSTC) model of OCD pathophysiology (Milad & Rauch, Reference Milad and Rauch2012). Although not directly comparable due to methodological differences, the regions of hypergyrification observed in our study are consistent with other neuroimaging findings, as earlier onset age in OCD was reported to be associated with larger precentral and middle frontal volumes (Kim et al., Reference Kim, Kwak, Hur, Lee, Shin, Lee and Kwon2020) as well as medial frontal volumes (Koprivova et al., Reference Koprivova, Horacek, Tintera, Prasko, Raszka, Ibrahim and Hoschl2009). In addition, abnormal volume in the ACC was observed in an early illness state, and its lack of association with illness duration further suggested a neurodevelopmental basis for ACC abnormalities in OCD (Rosenberg & Keshavan, Reference Rosenberg and Keshavan1998). The alterations in gyrification are believed to reflect disruptions in underlying white matter connectivity of closely related cortical regions according to a tension-based model (Toro & Burnod, Reference Toro and Burnod2005; Van Essen, Reference Van Essen1997; White, Su, Schmidt, Kao, & Sapiro, Reference White, Su, Schmidt, Kao and Sapiro2010). In the CSTC neuronal circuit, frontoparietal and cingulate regions are connected to the striatum and thalamus (Jung et al., Reference Jung, Jang, Park, Kim, Goo, Im and Kwon2014), and ventral prefrontal–striatal deficits in the CSTC have been proposed as neurodevelopmentally mediated network abnormalities in pediatric OCD patients (Huyser et al., Reference Huyser, Veltman, de Haan and Boer2009; Rosenberg & Keshavan, Reference Rosenberg and Keshavan1998). Reduced white matter integrity in arcuate fibers near the superior parietal lobule and decreased fiber quantities and thickness in highly connected cortical areas, including the postcentral, precuneus, posterior cingulate, paracentral, and several frontal regions, were reported as potential endophenotypes for OCD (Peng et al., Reference Peng, Shi, Shi, Miao, Yang, Gao and Shen2014). Additionally, reduced myelin-related growth in both gray and white matter of the dorsolateral and medial frontal cortex centered around the superior frontal gyrus and ACC was further reported to be associated with psychiatric traits of compulsivity during cortical development in healthy subjects, suggesting alterations in cortical gyrification and structurally connected white matter in the frontoparietal and cingulate regions represent trait characteristics of OCD (Ziegler et al., Reference Ziegler, Hauser, Moutoussis, Bullmore, Goodyer, Fonagy and Dolan2019).
In the correlation analyses performed with an exploratory purpose, visuospatial memory and verbal fluency deficits were significantly associated with a higher mean lGI in early-onset OCD patients, supporting the notion that increased gyrification is a reliable marker for distinguishing neurodevelopmentally homogenous subtypes of OCD. Among the impairments in various neurocognitive functions observed in OCD, visual memory has been reported to show the largest effect size, with the most consistent reports of deficits in immediate recall in the RCFT (Shin et al., Reference Shin, Lee, Kim and Kwon2014). OCD patients have also shown persistent impairments in the RCFT and COWA during pharmacological treatments (Kim et al., Reference Kim, Park, Shin and Kwon2002; Roh et al., Reference Roh, Shin, Kim, Ha, Shin, Lee and Kwon2005) and cognitive behavioral therapy (Vandborg et al., Reference Vandborg, Hartmann, Bennedsen, Pedersen and Thomsen2015) despite significant improvements in their OC symptoms. These neuropsychological deficits evidenced in fully recovered OCD patients and their unaffected relatives (Rao et al., Reference Rao, Reddy, Kumar, Kandavel and Chandrashekar2008; Zartaloudi et al., Reference Zartaloudi, Laws and Bramon2019) further supported the hypothesis that impairments in visual memory and verbal fluency are trait markers for OCD rather than state-dependent features of the disease. In fact, neuropsychological differences in visual memory and verbal fluency have been consistently reported between early- and late-onset OCD subtypes. Hwang et al. (Reference Hwang, Kwon, Shin, Lee, Kim and Kim2007) observed worse visual memory and verbal fluency in late-onset OCD patients than in HCs, while Roth et al. (Reference Roth, Milovan, Baribeau and O'Connor2005) reported worse visual memory and verbal fluency in late- and early-onset OCD patients, respectively, than in HCs. These inconsistent findings may have resulted from low general intelligence, medication effects, or different criteria for onset age (Hwang et al., Reference Hwang, Kwon, Shin, Lee, Kim and Kim2007; Kim et al., Reference Kim, Kwak, Hur, Lee, Shin, Lee and Kwon2020). However, in our previous volumetric study, with 17 years as the cutoff age of onset, unmedicated or drug-naïve early-onset OCD patients exhibited worse visuospatial memory than HCs (Kim et al., Reference Kim, Kwak, Hur, Lee, Shin, Lee and Kwon2020). Using a larger sample, early-onset OCD patients in the current study demonstrated significant impairments in both RCFT and COWA performance in relationship with gyrification abnormalities, suggesting that visuospatial memory and verbal fluency deficits are prominent neurocognitive features for the neurodevelopmental subtyping of OCD.
Limitations
There are several limitations that should be considered in this study. First, there is presently no clear standard for the cutoff onset age to distinguish between early- and late-onset OCD, and it is possible that the results would differ if the specific cutoff used by researchers to create such classification varies; thus, assumptions regarding onset age should be considered with caution before accepting the generality of the results. Second, the use of cross-sectional samples precludes investigation of the causal relationships between gyrification abnormalities as a vulnerability marker and the development of OCD. Thus, longitudinal MRI analyses in both early- and late-onset OCD patients are required to further clarify the neurodevelopmental origin and identify the longitudinal trajectories of these alterations in cortical folding. Third, the results of correlations between lGI and neurocognitive impairments were not corrected by multiple comparison and should be considered exploratory. In future studies, larger sample sizes would help to improve effect size and reproducibility of brain structure and cognitive phenotype correlations, and more pronounced effects of neurodevelopmental abnormalities may be seen with larger sample sizes (Marek et al., Reference Marek, Tervo-Clemmens, Calabro, Montez, Kay, Hatoum and Dosenbach2022).
Conclusion
Our findings demonstrated that the early-onset OCD group had increased gyrification in the frontoparietal and cingulate gyri, key regions involved in the major pathophysiology of OCD, compared to the late-onset OCD and HC groups that was associated with impaired trait-related neurocognitive functions, which is consistent with the notion of greater neurodevelopmental deficits in early-onset OCD than in late-onset OCD. Taken together with previous findings of accumulated phenotypic and clinical evidence suggesting that early-onset OCD is a neurodevelopmental subtype of OCD, our findings provide biological evidence to distinguish the OCD population into more neurodevelopmentally homogeneous subtypes that may provide structural markers for understanding neurobiological underpinnings of different etiologies and helping to find appropriate treatment options for OCD subtypes.
Supplementary material
The supplementary material for this article can be found at https://doi.org/10.1017/S0033291722003129
Financial support
This research was supported by the Basic Science Research Program and the Bio & Medical Technology Development Program through the National Research Foundation of Korea (NRF) and the KBRI basic research program through Korea Brain Research Institute, funded by the Ministry of Science & ICT (grant nos. 2019R1C1C1002457, 2020M3E5D9079910, 2021M3A9E4080784, and 21-BR-03-01).
Conflict of interest
None.