INTRODUCTION
In Poland, the dendrochronological method is often used for the precise dating of building structures, historic wooden monuments and artifacts. This is particularly important considering the period covering the beginnings of statehood in Poland, which was born in the region of today’s Wielkopolska (Kara and Krąpiec Reference Kara and Krąpiec2005; Kara Reference Kara2009). Since the 1990s, dating oak wood samples from Wielkopolska (Greater Poland) has been relatively easy because the long master chronology for the region has already been constructed, covering the period 449-1998 AD (Krąpiec, Reference Krąpiec1998). However, the possibility of dating pine wood looks, quite different. Combining the efforts of several years, a pine chronology for Wielkopolska has not been constructed due to the lack of suitable wood samples. This leaves a gap for this very important region between master pine chronologies in the neighboring areas which range 1091–2004 AD for Małopolska (Szychowska-Krąpiec Reference Szychowska-Krąpiec2010) and 1106–1994 AD for Kujawy and Gdańsk Pomerania (Zielski Reference Zielski1997).
This situation changed in 2008 due to extensive rescue archaeological excavations due to construction of a new sewage network required by the municipality in Ujście, covering the sub-stronghold zone (Rybacka Street) and the Piast stronghold castle (Old Market) (Figure 1).
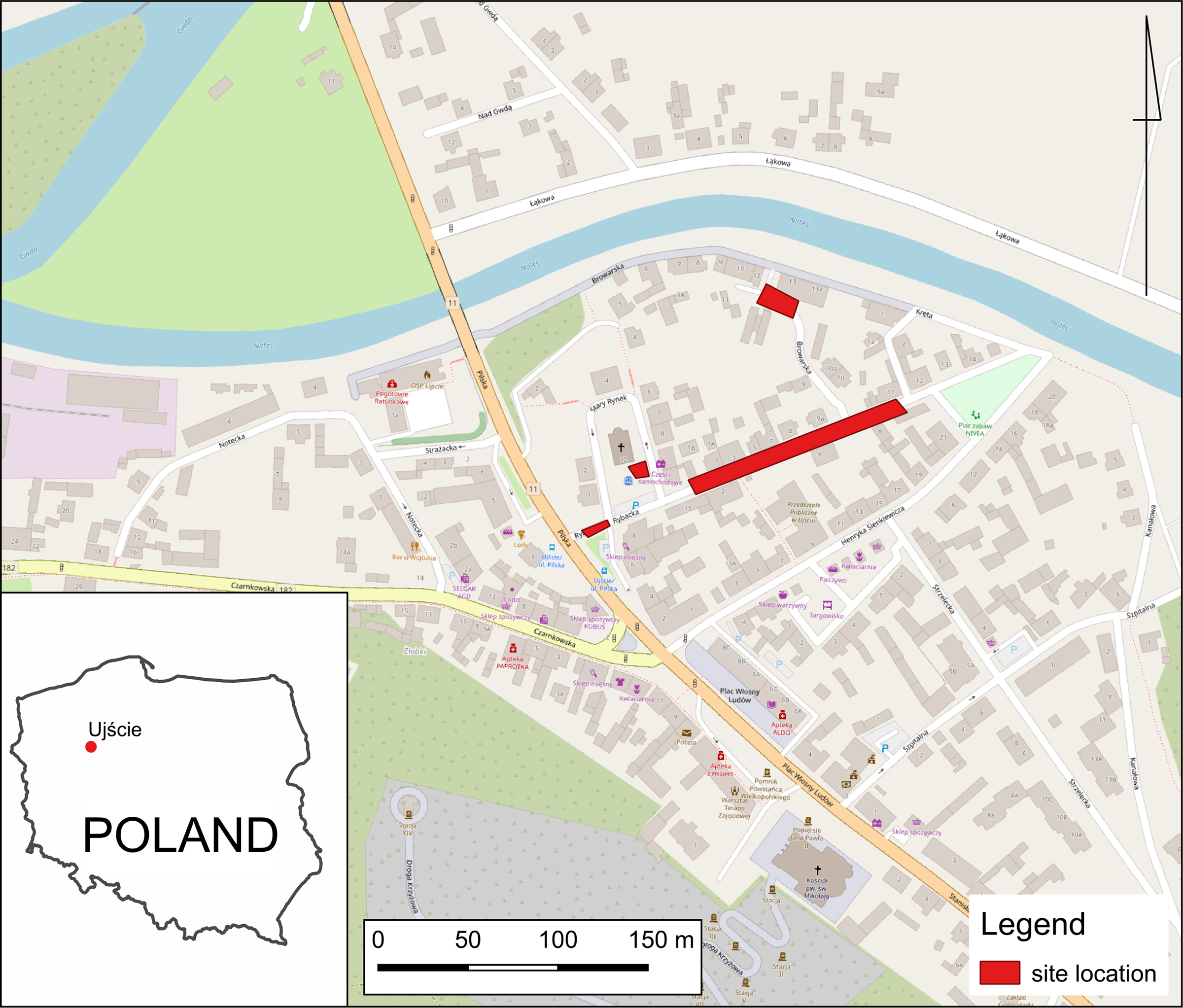
Figure 1 Location of the site Ujście and archaeological trenches in which pine wood was encountered and sampled for analyses.
Altogether, approximately 800 wood samples from a wide spectrum of species were gathered from all excavations, of which 300 fulfilled the requirements of the dendrochronological method, i.e. an adequate number of increments and a highly conserved wood structure. Among them, the elements made of pine wood predominated and should be considered as a special case, since generally, wooden structures from that time were made of oak wood. Presumably, the less durable pinewood was used in earthen structures because of a deficit of oak wood. The area around Ujście is characterized by poor sandy soils, predominated by pine forests and with the occasional mixed forests with oak.
Preliminary results, based on archaeological artefacts and dendrochronological dating of the oak wood, indicated that the pine wood found in the structures examined should allow to extend the existing pine master chronologies back in time.
Based on the most converging dendrochronological sequences, a floating pine chronology 2U_02A, covering 227 years, was compiled (Bolka and Krąpiec Reference Bolka and Krąpiec2012). The comparison of the 2U_02A chronology with the master chronologies developed for pine from the neighbouring areas, i.e. the regional chronology from northern Poland covering the period 1106–1991 AD (Zielski Reference Zielski1997) and northern Germany 924–1995 AD (Heussner Reference Heussner and Moździoch1996), did not allow the identification of synchronous positions. In such a situation, the most suitable dating method was the wiggle-matching method, with which the period represented by the curve2U_02 was determined for the years 860–1080 (±10) cal. AD (Bolka and Krąpiec Reference Bolka and Krąpiec2012).
Precise dating, with a 1-year accuracy, of the chronology w 2U_02A, produced from almost 100 individual tree-ring sequences, would be of substantial importance for Polish regional archaeology. The chronology covers an important transition to state organization in the early Middle Ages and is urgently needed to refine a construction sequence from a series of defensive pine-constructions which could be dated to the year with a regional master pine chronology.
This purpose could be achieved by taking advantage of the sudden increase in the radiocarbon (14C) concentration in annual tree rings, described by Miyake et al. (Reference Miyake, Nagaya, Masuda and Nakamura2012, Reference Miyake, Masuda and Nakamura2013, Reference Miyake, Masuda, Hakozaki and Nakamura2014, Reference Miyake, Jull, Panyushkina, Wacker, Salzer, Baisan, Lange, Cruz, Masuda and Nakamura2017) and Park et al. (Reference Park, Southon, Fahrni, Creasman and Mewaldt2017). Studies on the radiocarbon content in the annual growth of different tree species growing in both northern and southern hemispheres have demonstrated that the so-called “Miyake effects” dated to 661/660 BC, 774/775 AD and 993/994 AD are of a global extent (Usoskin et al. Reference Usoskin, Kromer, Ludlow, Beer, Friedrich, Kovaltsov, Solanki and Wacker2013; Jull et al. Reference Jull, Panyushkina, Lange, Kukarskih, Myglan, Clark, Salzer, Burr and Leavitt2014; Güttler et al. Reference Güttler, Adolphi, Beer, Bleicher, Boswijk, Christl, Hogg, Palmer, Vockenhuber, Wacker and Wunder2015; Büntgen et al. Reference Büntgen, Myglan, Ljungqvist, McCormick, Di Cosmo, Sigl, Jungclaus, Wagner, Krusic, Esper, Kaplan, de Vaan, Luterbacher, Wacker, Tegel and Kirdyanovet2016, Reference Büntgen, Wacker, Galván, Arnold, Arseneault, Baillie, Beer, Bernabei, Bleicher and Boswijk2018; Fogtmann-Schulz et al. Reference Fogtmann-Schulz, Ostbo, Nielsen, Olsen, Karoff and Knudsen2017). Similar results have also been determined in annual increments of oaks growing around Krakow (S Poland) (Rakowski et al. Reference Rakowski, Krapiec, Huels, Pawlyta, Dreves and Meadows2015, Reference Rakowski, Krąpiec, Huels, Pawlyta and Boudin2018, Reference Rakowski, Krąpiec, Huels, Pawlyta, Hamann and Wiktorowski2019). The Miyake 775 AD peak was successfully used for dating of the period of building the Holy Cross chapel in Val Müstair in Switzerland (Wacker Reference Wacker, Güttler, Goll, Hurni, Synal and Walti2014) as well as the Baitoushan Volcano eruption in China (Hakozaki et al. Reference Hakozaki, Miyake, Nakamura, Kimura, Masuda and Okuno2018).
The sudden increase of 1.5% in atmospheric radiocarbon concentration between AD 774 and 775 is the strongest observed Miyake effect. The increase in radiocarbon concentration between AD 993 and 994 was less intense and amounted to 0.9% (Miyake et al. Reference Miyake, Masuda and Nakamura2013, Reference Miyake, Masuda, Hakozaki and Nakamura2014). Both of the events have global character and show the same characteristic shape of increase in radiocarbon concentration, which made possible to use them for high-resolution wiggle-matching (HR wiggle-matching).
As the chronology 2U_02A does not cover the effect 774/775 AD, it was possible to date the sequence using the weaker increase of the 14C contents in 993/994 AD (Δ14C = 6.8‰), which was detected in annual rings of subfossil oaks from Kujawy near Kraków (Rakowski et al. Reference Rakowski, Krąpiec, Huels, Pawlyta and Boudin2018).
MATERIAL AND METHODS
The wood sample selected for the analyses had the form of a slice, was labeled with the laboratory code 2UJC141 and obtained from a beam, which was a structural element of a timber trackway.
Measurements of annual growth rings were made with 0.01-mm accuracy using a DENDROLAB 1.0 apparatus, and subsequently, the ring-width sequences were processed using the software packages TREE-RINGS (Krawczyk and Krąpiec Reference Krawczyk and Krąpiec1995), TSAP (Rinn Reference Rinn2005), and DPL (Holmes Reference Holmes1999). The sample contained 101 annual rings, the width of which allowed the separation of wood samples of the appropriate mass to obtain several mg of alpha cellulose. The dendrogram (annual increment width graph) of this sample has a clear resemblance to the 2U_2A chronology between 108 and 208 relative years, with t = 5.5, GL = 70%, where the t-value represents the significance of the correlation of two series in relations to their overlap and should not be below 3.5 (Baillie and Pilcher Reference Baillie and Pilcher1973). The GL (Gleichlaufigkeit) was developed as a special tool for the cross-dating of tree ring series. The degree of similarity based on the positive or negative trend of each width is expressed as a percentage of the number of intervals (Eckstein and Bauch Reference Eckstein and Bauch1969), as shown in Figure 2.
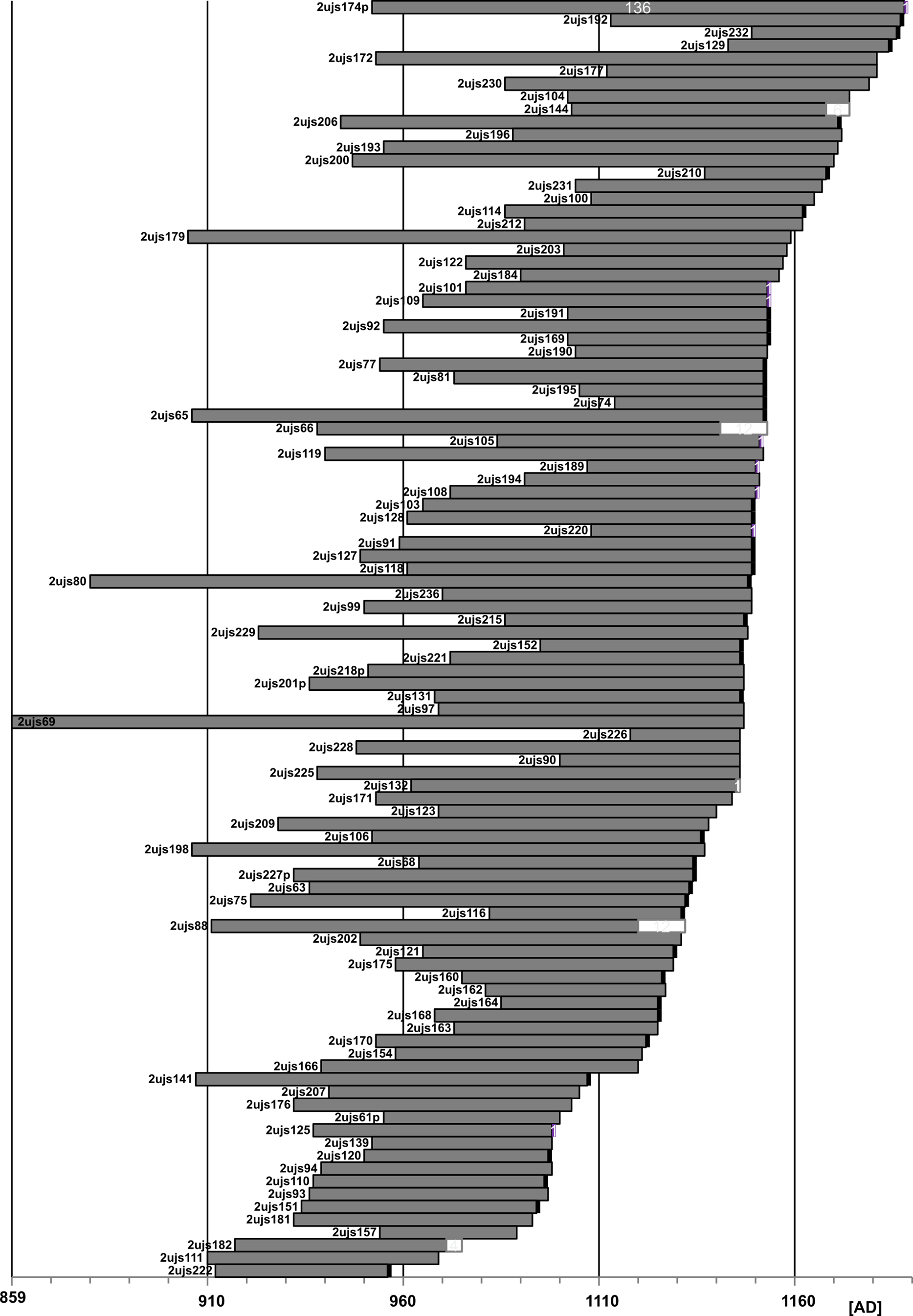
Figure 2 Diagram of the time extent of growth sequences forming the pine chronology 2U_02A from Ujście.
From the sample 2UC141, 36 annual rings were separated; their nos. 22–58 were equivalent to 125–165 relative years of the chronology. Of the selected rings, we extracted α-cellulose, a preferred component of wood for high-precision analysis of radiocarbon. Cellulose represents the most stable part of the wood, since carbon in the cellulose fraction does not cross ring boundaries, whereas other carbon fractions, such as lignin and hemicelluloses, may cross ring boundaries or be exchanged with the atmosphere after formation (Loader et al. Reference Loader, Switsur and Field1995; Santos et al. Reference Santos, Bird, Pillans, Fifield, Alloway, Chappell, Hausladen and Arneth2001; Nemec et al. Reference Nemec, Wacker, Hajdas and Gaggeler2010).
For cleaning and α-cellulose extraction, we used a method described previously (Green Reference Green and Whistler1963), with some modifications. To expedite the separation of single cellulose fibres from wood samples and thus to increase the penetration of reagents, an ultrasonic bath was used (Pazdur et al. Reference Pazdur, Korput, Fogtman, Szczepanek, Hałas, Krąpiec and Szychowska-Krąpiec2005). Wood samples were dried, cut into shavings, and subsequently weighed and placed into glass tubes. Each sample was placed into a glass tube with deionized water, sodium chlorite, and 1% HCl. The tube was then placed in an ultrasonic bath at 70°C for 1 hr, and subsequently, sodium chlorite and 1% HCl were added to each sample. This procedure was repeated five times, and the samples were then rinsed with hot deionized water up to a neutral pH. This process removes most of the lignin, and the remaining sample consists mostly of holocellulose. For the next step, 10% NaOH solution was added to the samples, which were then placed into an ultrasonic bath at 70°C for 45 min. After the solution was removed, the sample was rinsed with cold deionized water. In the following step, 17% NaOH solution was added, and the samples were placed into an ultrasonic bath at room temperature for 45 min. Subsequently, deionized water was added; this step completely removes the remaining lignin and holocellulose. The samples were then rinsed with hot deionized water up to a neutral pH. Finally, 1% HCl was added to the samples, and the samples were again rinsed to a neutral pH. The obtained α-cellulose was dried overnight at 70°C (Michczyńska et al. Reference Michczyńska, Krapiec, Michczyński, Pawlyta, Goslar, Nawrocka, Piotrowska, Szychowska-Krąpiec, Waliszewska and Zborowska2018).
After the pretreatment, around 4 mg of α-cellulose, extracted from each sample, was combusted to CO2 and subsequently reduced to graphite (Krąpiec et al. Reference Krąpiec, Rakowski, Huels, Wiktorowski and Hamann2018). The resulting mixture of graphite and Fe powder was pressed into a target holder for AMS 14C measurements. All prepared targets contained approximately 1 mg of carbon and were measured at the Center for Applied Isotope Studies at the University of Georgia, USA (Labcode UGAMS; Cherkinsky et al. Reference Cherkinsky, Culp, Dvoracek and Noakes2010). The 14C contents are reported as Δ14C in per mil (‰) deviations from the standard sample, 0.7459 activity of NBS oxalic acid (SRM-4990C). Age correction and isotopic composition correction were calculated following formulas presented elsewhere (Stuiver and Polach Reference Stuiver and Polach1977). The correction for isotopic composition was made based on δ13C, measured with an AMS system.
RESULTS AND DISCUSSION
In the first stage, the measurements were made for six α-cellulose samples, separated from the increments representing 33, 37, 42, 47, 52, and 57 relative years. Dating results indicated that the older increments selected contained the diagnostic signal from 994/5 AD. In the two following sessions, α-cellulose from rings 21–41 relative years was measured (Table 1).
Table 1 14C concentrations of measured tree rings from Ujście (northwest Poland). The calendar ages of the annual tree rings were obtained using comparison with the radiocarbon concentration during the Miyake effect around AD 993/994.

To fix the floating chronology, we analysed the normalized sum of squares of offsets of the sequence 2U_2A from Ujście D14C and a combination of other available D14C data sets; we used data from the Kujawy tree ring sequence (Rakowski et al. Reference Rakowski, Krąpiec, Huels, Pawlyta and Boudin2018) and two sequences from Japan (Miyake et al. Reference Miyake, Masuda and Nakamura2013, Reference Miyake, Masuda, Hakozaki and Nakamura2014).). Normalisation was obtained by dividing sum of squares by number of offsets used for the calculation of the sum. We tried three different approaches. In the first approach, for each year, starting from 981 AD to 1013 AD, the average D14C of the three sequences was calculated. When no data were available for a particular year, the final “average” sequence was calculated using two or one data points (Figure 3). The Ujście sequence starting point was then shifted year by year, and for each shift, the sum of squared offsets of Ujście and the “average” data points was calculated. The sum of squared offsets was then normalized by the number of offsets used in the calculation of the sum (Figure 4, x – symbols in the graph). In the second approach, we made similar calculations, but only the offsetsfor the Kujawy and Ujście data sets were analysed (Figure 4, stars). In the third approach, we separately calculated the offsets for each available data point for the Ujście data set and for any other data set. Subsequently, we calculated the squared sum of all the differences and normalized it (Figure 3, triangles). For each approach, the minimum of the normalized sum was obtained for the Ujście sequence starting in the year 986 AD (Figure 4). On this basis, it can be concluded that the pine chronology 2U-02A from Ujście was accurately dated to the period 859–1085 AD. Its value for absolute dating is difficult to overestimate; it serves as a tool for precise dating, but also as a potential record to be used in dendroclimatic studies both in traditional terms, using tree ring width variability as well as the contents of stable isotopes.
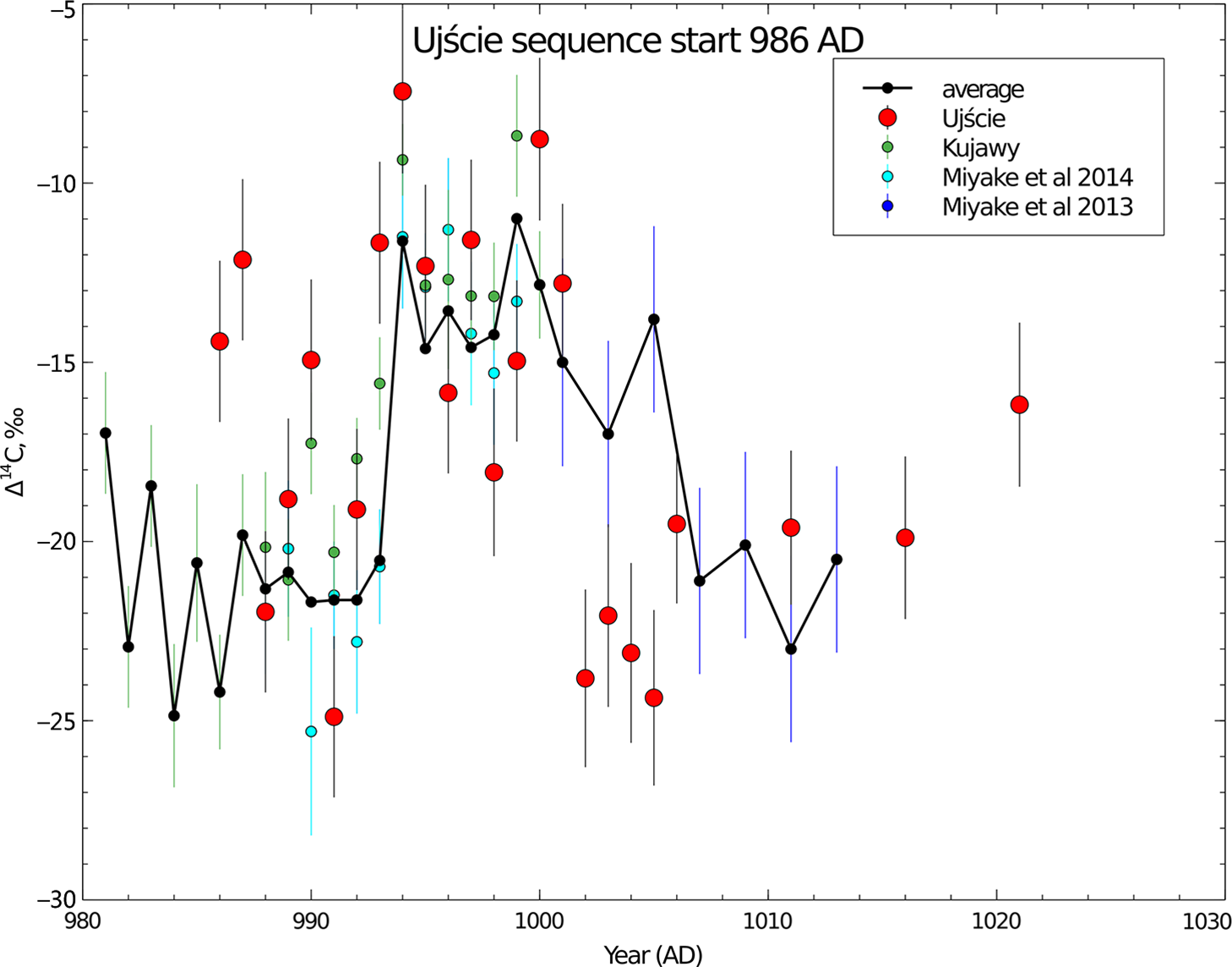
Figure 3 Δ14C in Japan (cedar, Miyake et al. Reference Miyake, Masuda and Nakamura2013, Reference Miyake, Masuda, Hakozaki and Nakamura2014) and Kujawy, Poland (Quercus sp., Rakowski et al. Reference Rakowski, Krąpiec, Huels, Pawlyta and Boudin2018) plus average values with results of measurements from this study (Pinus sylvestris, Ujście site).

Figure 4 Normalized sum of squared distances between data sets for a given starting position. Kujawy data after Rakowski et al. (Reference Rakowski, Krąpiec, Huels, Pawlyta and Boudin2018), Japan data sets after Miyake et al. (Reference Miyake, Masuda and Nakamura2013, Reference Miyake, Masuda, Hakozaki and Nakamura2014).
Dating using the Miyake effect (Reference Miyake, Masuda and Nakamura2013) allowed us to assess the quality of the earlier dating of the Ujście chronology with the wiggle-matching method, based on bulk wood samples containing several increments, with the LSC technique using the Hidex 3000 SL low-background spectrometer (Krąpiec and Walanus Reference Krąpiec and Walanus2011). The result of the WM match fits perfectly and deviates only by 1 year from the date obtained using the Miyake approach. Although such high convergence is probably an exceptional case, it still demonstrates that the LSC date of wood prepared with the AAA method does not deviate from the result obtained with the AMS technique and can be considered as a cheaper alternative to the pre-placement of sequences on the timescale.
We demonstrate that dating of a floating chronology containing annual rings from the period of abrupt changes in the radiocarbon content (so-called “Miyake effects”) allows precise dating, but identification of the correct position requires the analysis of about a dozen annual increments. In cases where a hypothetical dendrological date was confirmed, the number of dated tree rings could be reduced to perhaps 5 to 10, as demonstrated by the study of Wacker et al. (Reference Wacker, Güttler, Goll, Hurni, Synal and Walti2014). Due to the characteristic shape of the increase in radiocarbon concentration, and its global character (Büntgen et al. Reference Büntgen, Wacker, Galván, Arnold, Arseneault, Baillie, Beer, Bernabei, Bleicher and Boswijk2018), those events could be used for high-resolution wiggle-matching, resulting in 1-year resolution.
One has to keep in mind that the 14C excursion event occurring in 993/994 AD has an amplitude of less than 10‰ (Miyake et al. Reference Miyake, Masuda and Nakamura2013, Reference Miyake, Masuda, Hakozaki and Nakamura2014), which means that uncertainty of radiocarbon measurements for each sample in the analysed tree-ring sequence should be better than 3‰.
ACKNOWLEDGMENTS
This work was supported by the National Science Centre, Poland, grant UMO-2017/25/B/ST10/02329, and partly by the Silesian University of Technology, grant 14/990/BK_190119.