The early weaning process of piglets is always followed by weaning stress, and diarrhoea is one of the main symptoms of stress. It has been reported that 10 million piglets die of diarrhoea annually worldwide, resulting in 11 % mortality in post-weaning piglets( Reference Owusu-Asiedu, Nyachoti and Marquardt 1 ). Newly weaned piglets always encouter psychosocial and physical stressors, such as maternal and littermate separation, and changes of diet and environment( Reference Moeser, Klok and Ryan 2 ). As nutrition is one of the major factors causing stress, nutritional regulation plays an important role in alleviating post-weaning diarrhoea( Reference Funderburke and Seerley 3 ). Zn, one of the essential trace elements in the animal body, takes part in the synthesis of over 300 enzymes, and is intimately associated with gut development and immune function. Pharmacological concentration of inorganic Zn, especially ZnO, is widely used in the diets of piglets in the swine industry, due to its effects on enhancing growth performance and alleviating the incidence of diarrhoea( Reference Carlson, Hill and Link 4 , Reference Hill, Mahan and Carter 5 ). However, the addition of a high dose of ZnO to the diets of weaned piglets has been criticised worldwide, and many countries have limited or banned the use of such high dose of ZnO in weaned piglets owing to severe environmental pollution caused by a large amount of Zn excretion( Reference Hahn and Baker 6 ).
ZnO is an amphoteric molecule and almost insoluble in water, but has a higher solubility at acid pH. When ZnO in the diet reaches the stomach of piglets where the pH value is about 3·5–4·5, most insoluble ZnO is transformed into Zn ions, thus just a small amount of ZnO can work in the intestine, which is the important site of its action. In addition, compared with other inorganic forms of Zn, ZnO has been found to have a better effect on the resistance to intestinal diseases( Reference Roselli, Finamore and Garaguso 7 ), which probably means that besides Zn ions, the ZnO molecule itself also plays a crucial role in alleviating the incidence of diarrhoea. This is the reason why a high dose of ZnO is added into the diets of piglets. If ZnO is processed to make its dissociation percentage to reduce in the stomach, a larger amount of ZnO can reach the intestine, thereby a lower dose of ZnO could be used in the diet. Some studies have proved that this is entirely feasible. As clay mineral carriers, such as diosmectite and zeolite, have high ion-exchange and adsorption capacities, they can be used as a means of controlling the release of ZnO in the gastrointestinal tract; therefore, the addition levels of ZnO can be reduced to 500–900 mg Zn/kg( Reference Hu, Song and Li 8 , Reference Hu, Xiao and Song 9 ). However, the amount of ZnO supported by these carriers is still obviously supra-physiological for piglets. A better method should be sought to further decrease the addition levels of ZnO. Enteric coating is a common technology to protect oral medications against the effects of stomach juices in the field of pharmacy. This technology has been used in the field of feed additives for a long time. Under the protection of outer enteric coating, the inner core component can safely pass through the stomach. We have conducted an in vitro experiment on the dissociation percentage of coated ZnO and ZnO (uncoated) in the stomach digesta of weaned piglets. The results showed that the dissociation percentage of coated ZnO in stomach is 25·03 %, while that of ZnO (uncoated) is 85·26 %, which indicated that coating can well protect ZnO from being dissociated in the stomach. At alkaline pH (i.e. in the gastrointestinal tract), the coating would be gradually degraded and the inner ZnO would be released slowly in the entire intestine. Kim et al. ( Reference Kim, Lee and Park 10 ) reported that a low dose of coated ZnO (100 mg Zn/kg) achieved the same effect as with a high dose of ZnO (3000 mg Zn/kg) in alleviating post-weaning diarrhoea( Reference Kim, Hansen and Mullan 11 ). This observation demonstrated that the use of enteric coating is an ideal method to reduce the addition levels of ZnO. It is known that post-weaning diarrhoea is closely associated with the damage of intestinal immunity function and microbiota composition; however, little study has been carried out to investigate the effects of coated ZnO on these two aspects. Therefore, in the present study, we hypothesised that supplementation of a low dose of coated ZnO, as a potential substitute for a high dose of ZnO, could alleviate the incidence of diarrhoea in weaned piglets by improving intestinal immunity function and regulating microbiota composition. To test this hypothesis, we evaluated the effects of coated ZnO on growth performance and diarrhoea index in piglets. Furthermore, intestinal morphology and mRNA expression levels of insulin-like growth factor 1 (IGF-1), tight junction (TJ; zonula occludens protein-1 (ZO-1) and occludin) and inflammatory cytokines (TNF-α, IL-6, transforming growth factor β1 (TGF-β1) and IL-10), and secretory IgA (SIgA) concentration in the jejunal mucosa were determined to investigate the effects of coated ZnO on intestinal development and immune status in piglets. Microbiota richness, the Shannon diversity index, clustering of the denaturing gradient gel electrophoresis (DGGE) profiles and the relative abundances of Lactobacillus and Escherichia coli were also determined to assess the effects of coated ZnO on the intestinal microbiota composition of piglets. In addition, Zn concentrations in the serum, liver, kidney and faeces of weaned piglets were analysed to assess Zn residues in animals and environmental pollution of Zn.
Materials and methods
The animal use and care protocol was approved by the Animal Care and Use Committee of Sichuan Agricultural University.
Experimental animals and design
A total of eighty-four cross-bred piglets (Duroc × Landrace × Yorkshire), weaned at an age of 28 (sem 1) d, were allocated randomly, on the basis of average initial body weight (7·72 (sem 0·65) kg), to one of seven dietary treatments with four replicates each. There were three pigs in each replicate placed together in an individual pen equipped with woven-wire floors, stainless-steel nipple drinkers and feeders. There were four rooms and each room contained seven pens. The room temperature was maintained at 28°C.
Seven treatments consisted of a 250 mg Zn (ZnO)/kg diet (low Zn (LZ); basal diet+250 mg Zn (ZnO)/kg), a 2250 mg Zn (ZnO)/kg diet (high Zn (HZ); basal diet+2250 mg Zn (ZnO)/kg) and five experimental diets in which coated ZnO (CZ) (Guangzhou Wisdom Bio-Tech Company Limited) was added at 250 (CZ250), 380 (CZ380), 570 (CZ570), 760 (CZ760) and 1140 (CZ1140) mg Zn/kg of basal diet, respectively. No other Zn source was added into the basal diet.
Diets and management
The basal diet was formulated on the basis of nutrient requirements for weaned piglets( 12 ) (Table 1) . The ZnO used in the study was a feed-grade source.
Table 1 Compositions and nutrient levels of the basal diet (air-dried basis)

DE, digestible energy; CP, crude protein.
* Zn concentration was the measured value and others were calculated values.
† Compound vitamin provided the following per kg of diet: vitamin A, 10·56 mg; cholecalciferol, 0·2 mg; vitamin E, 200 mg; menadione, 40 mg; vitamin B1, 16 mg; vitamin B2, 128 mg; vitamin B6, 4·8 mg; vitamin B12, 0·24 mg; d-calcium pantothenate, 200 mg; nicotinic acid, 280 mg; folic acid, 4 mg.
‡ Minerals provided the following per kg of diet: Fe, 100 mg/kg; Cu, 10 mg/kg; Mn, 10 mg/kg; Se, 0·3 mg/kg; I, 0·3 mg/kg.
The trial was carried out at the Research Base of the Institute of Animal Nutrition in Sichuan Agricultural University. It included an adaptation period of 5 d adaptation and an experimental period of 14 d during which piglets were allowed for ad libitum access to diets and water. The diets were added to the feeders four times daily (08.00, 12.00, 16.00 and 20.00 hours). Individual feed consumption was determined daily throughout the duration of the trial. Initial (day 0) and final (day 14) body weights were measured after 12 h fasting. Response variables measured included average daily feed intake, average daily gain and feed:gain ratio. The diarrhoea index was monitored according to the previous faecal scoring system from 0 to 3 as follows: 0, normally shaped faeces; 1, shapeless faeces; 2, soft faeces; 3, liquid faeces( Reference Castillo, Martin-Orue and Taylor-Pickard 13 ).
Analysis of small-intestinal histology
On day 14, twenty-eight piglets (n 4 per treatment group) were randomly selected, and then held under general anaesthesia by an intravenous injection of 4 % sodium pentobarbital solution (40 mg/kg body weight) and killed. After a midline laparotomy, segments of the duodenum, the middle section of the jejunum and the ileum (20 mm) were taken quickly, and then fixed in formalin (formaldehyde 3·8 %). For histological analysis, the different intestinal segments (20 mm) were embedded in paraffin. Sections (5 μm) were cut and stained with periodic acid–Schiff reagent. The stained sections were subsequently used to determine villus height (μm) and crypt depth (μm). For each piglet, one slide was used and the average values were taken for a minimum of four villi and crypts.
Analysis of zinc concentrations in serum, liver, kidney and faeces
On day 14, four piglets from each treatment group were randomly selected for blood sampling via the jugular vein. The blood samples were collected into 20 ml tubes and allowed to clot at an ambient temperature (about half an hour). Thereafter, the samples were centrifuged at 3000 rpm for 15 min to obtain serum and then kept frozen until further analysis.
After twenty-eight piglets (one per replicate) were killed, liver and kidney were collected and washed with normal saline, and then stored at − 20°C for the determination of Zn concentrations. Faeces of each replication were also collected on day 14, dried using a forced-air oven (65°C) for 24 h, and then ground to pass a 2 mm screen for analysis of Zn concentrations.
Liver, kidney and faecal samples were prepared for Zn analysis using nitric–perchloric acid wet digestion( 14 ). The analysis of Zn concentrations was carried out with a nov-AA400 flame atomic absorption spectrometer (Analytik Jena AG).
Analysis of mucosal secretory IgA
Jejunal mucosa samples were collected, washed with normal saline and then stored at − 80°C for further analysis. The samples were mixed with PBS buffer through tissue homogenate, resulting in the formation of a 10 % solution. Then, SIgA concentrations were determined using the sandwich ELISA kit (Nanjing Jiancheng Biochemical Reagent Company), according to the manufacturer's instructions.
RNA isolation and real-time quantitative PCR
Total RNA was extracted from jejunal mucosa samples, which were triturated in liquid N2, using RNAiso Plus (Takara), according to the manufacturer's instructions. The integrity of the extracted RNA was checked by 1 % agarose gel electrophoresis and visualisation of intact 18S and 28S ribosomal RNA bands under UV light. Reverse transcription was performed using the PrimeScript™ RT Reagent Kit (Takara) with a 1 μg RNA sample, according to the manufacturer's instructions. Expression levels of IGF-1, ZO-1, occludin, IL-6, TNF-α, IL-10 and TGF-β1 in the jejunal mucosa were analysed by real-time quantitative PCR with SYBR Premix Ex Taq™ II (Takara), which was performed on complementary DNA samples in ninety-six-well optical plates on a CFX96™ Real-Time System (Bio-Rad). All of the primers (all the target genes and the reference gene TBP (TATA box-binding protein)) used for real-time PCR were designed using Primer Premier 5.0 software, synthesised by BGI. The primer sequences are presented in Table 2. The 25 μl PCR mixtures contained 12·5 μl of 2 × SYBR® premix Ex Taq™ II, 10 μmol/l of each forward and reverse primer and 2 μl of complementary DNA template. The PCR cycle parameters were as follows: an initial denaturation step at 95°C for 10 s, forty cycles of 95°C for 5 s and 58–63°C for 30 s (Table 2). A melt curve analysis was generated following each real-time quantitative PCR assay to verify the specificity and purity of the resulting PCR products. Relative quantification of the target gene transcript with a chosen reference gene transcript (TBP) was performed using the ΔΔC T method. All of the samples were analysed in triplicate. The mean threshold cycle (C T) of triplicates of each sample was used for calculations.
Table 2 Primers used for real-time PCR analyses

T m, melting temperature; E, PCR efficiency; IGF-1, insulin-like growth factor 1; ZO-1, zonula occludens protein-1; TGF-β1, transforming growth factor β 1; TBP, TATA box-binding protein (reference gene).
Analysis of PCR-denaturing gradient gel electrophoresis
Jejunal digesta and faecal samples (approximately 5 g) were aseptically obtained from the jejunum (middle section) and rectum of the twenty-eight piglets immediately post-slaughter, stored in sterile microtubes on ice, and transported immediately to the laboratory.
Total DNA was extracted from jejunal digesta and faecal samples using the QIAamp DNA Stool Mini Kit (Qiagen), according to the manufacturer's instructions( Reference Li, Gong and Cottrill 15 ). The universal bacterial primers HDAI-GC (5′-CGCCCGGGGCGCGCCCCGGGCGGGGCGGGG GCACGGGGGGACTCCTACGGGAGGCAGCAGT-3′) and HDA2 (5′-GTATTACCGCGGCTGCTGGCAC-3′) were used to amplify the V3 regions of 16S ribosomal DNA( Reference Fang, Yan and Kong 16 ). The reaction mixture (50 μl) contained 5 μl of 10-fold PCR buffer, 4 μl of dNTP mixture (10 mmol/μl), 2 μl of DNA template, 2 μl of each primer (2 μmol/l), 1 μl of Taq polymerase and 34 μl of distilled deionised water. The PCR was carried out on a S1000™ Thermal Cycler System (Bio-Rad) with a programme set at 94°C for 4 min, thirty cycles at 94°C for 30 s, 58°C for 30 s and 72°C for 2 min. Subsequently, V3 amplicons of 16S ribosomal DNA were used for sequence-specific separation by DGGE, which were performed on 10 % polyacrylamide gels with 35–65 % linear gradients of denaturant (100 % denaturant corresponds to 7 m-urea and 40 % deionised formamide) in 1-fold Tris acetate–EDTA buffer using a Bio-Rad D-Code System™ (Bio-Rad) at 60°C for 16 h at 100 V. The gel was developed after completion of electrophoresis and stained with AgNO3 according to the method described previously( Reference Wang, Zhu and Yao 17 ). Microbiota richness and the Shannon diversity index were calculated by the number of DGGE bands and the amount in each band, both of which were obtained by the image analysis of DGGE gels performed with Gel-Pro Analyzer 4.0 (Media Cybernetics). For the cluster of DGGE profiles, bands in each DGGE gel were scored as 1 (present) or 0 (absent)( Reference Kim, Lee and Park 10 ), and these DGGE data were used to compile a binary matrix for cluster analysis with a NTSYS-pc 2.10 package (Exeter software). Similarity among the DGGE profiles was calculated according to Jaccard's similarity coefficients, using the SIMQUAL (Similarity for Qualitative Data) routine. Then, the similarity coefficients were used to construct a dendrogram using the UPGMA (unweighted pair group method with arithmetic average) through the SHAN (sequential, hierarchical, agglomerative and nested clustering) routine of the NTSYS-pc package.
Analysis of the relative abundances of Lactobacillus and Escherichia coli
Genomic DNA was extracted from faecal samples (0·2 g each) using a TIANamp Stool DNA Kit (Tiangen Biotech), according to the manufacturer's instructions.
The Lactobacillus primers FQ-RSF (GAGGCAGCAGTAGGGAATCTTC) and FQ-RSR (CAACAGTTA CTCTGACACCCGTTCTTC) and the E. coli primers FQ-DCF (CATGCCGCGTGTATGAAGAA) and FQ-DCR (CGGGTAACGTCAATGAGCAAA)( Reference Qi, Xiang and Han 18 ) were used to perform real-time quantitative PCR by the ΔΔC T method for determining the relative abundances of the two types of bacteria with universal bacteria in the intestine of weaned piglets( Reference Livak and Schmittgen 19 ). The universal bacterial primers were Eub338F (ACTCCTACGGGAGGCAGCAG) and Eub518R (ATTACCGCGGCTG CTGG)( Reference Fierer, Jackson and Vilgalys 20 ). The PCR efficiencies of Lactobacillus, E. coli and universal bacteria were 95·7, 93·5 and 93·2 %, respectively.
Statistical analyses
Data were analysed by a one-way ANOVA procedure using SPSS 17.0 software (SPSS, Inc.). Differences between means were tested using Duncan's range test. Significance was reported at P< 0·05. Planned contrast was used to compare the LZ and HZ treatments. Polynomial (linear, quadratic and cubic) contrasts were used to determine the effect of the coated ZnO dose.
Results
Growth performance and diarrhoea index
No significant difference was observed in the growth performance of weaned piglets in all the groups, such as average daily gain, average daily feed intake and feed:gain ratio (Table 3). There was no incidence of diarrhoea in the present study as the diarrhoea index of all the groups remained low ( < 1). Nonetheless, the HZ-fed group had a lower diarrhoea index than the LZ-fed group (P< 0·01). The diarrhoea index decreased steadily as the level of coated ZnO was increased from 250 to 570 mg Zn/kg, and then increased with further addition (quadratic and cubic; P< 0·01). Compared with the LZ-fed group, the CZ380- and CZ570-fed groups had lower diarrhoea indices (P< 0·05), but were not significantly different from that of the HZ-fed group. Among the five groups supplemented with the coated ZnO diet, the CZ570-fed group, having the minimum value of the diarrhoea index, had 40·63 % lower diarrhoea index than the LZ-fed group (P< 0·05).
Table 3 Growth performance and diarrhoea index of weaned piglets fed different levels of coated zinc oxide (Mean values with their standard errors)

LZ, piglets fed at a dose of 250 mg Zn (ZnO)/kg; HZ, piglets fed at a dose of 2250 mg Zn (ZnO)/kg; CZ250, piglets fed at a dose of 250 mg Zn (coated ZnO)/kg of basal diet; CZ380, piglets fed at a dose of 380 mg Zn (coated ZnO)/kg of basal diet; CZ570, piglets fed at a dose of 570 mg Zn (coated ZnO)/kg of basal diet; CZ760, piglets fed at a dose of 760 mg Zn (coated ZnO)/kg of basal diet; CZ1140, piglets fed at a dose of 1140 mg Zn (coated ZnO)/kg of basal diet; L, linear; Q, quadratic; C, cubic; BW, body weight; ADG, average daily gain; ADFI, average daily feed intake; F:G, feed:gain ratio.
a,bMean values within a row with unlike superscript letters were significantly different (P< 0·05).
Intestinal morphology of weaned piglets
The effects of coated ZnO supplementation on the small-intestinal morphology of weaned piglets are displayed in Table 4. The villus heights of the duodenum and ileum increased with the increasing amounts of coated ZnO supplementation. Moreover, duodenal villus height was greater for the groups supplemented with the coated ZnO diet (except the CZ250-fed group) than for the LZ-fed group (P< 0·05); furthermore, the CZ760-fed group had a greater duodenal villus height than the HZ-fed group (P< 0·05). Additionally, the groups supplemented with the coated ZnO diet (except the CZ380-fed group) had decreased jejunal and ileal crypt depths (P< 0·05) when compared with the LZ-fed group, and especially, the ileal crypt depths of these groups were not different from that of the HZ-fed group. In the duodenum, the ratio of villus height:crypt depth (V:C) significantly increased with the increasing levels of coated ZnO up to 760 mg Zn/kg, and decreased thereafter (P< 0·05). The V:C values were higher for the coated ZnO-supplemented groups than for the LZ-fed group (except the CZ250-fed group), but none of the groups had a higher value than the HZ-fed group (P< 0·05). In the jejunum and ileum, the V:C values for the CZ250-, CZ570- and CZ1140-fed groups were not significantly different from that for the HZ-fed group (P>0·05). There were cubic (P< 0·01) effects of the coated ZnO dose on all small-intestinal morphology indices, except for the duodenal and ileal crypt depths, and jejunal V:C values. Duodenal crypt depth showed a quadratic (P< 0·01) response to the coated ZnO dose, while ileal crypt depth had a cubic (P< 0·05) response.
Table 4 Small-intestinal morphology of weaned piglets fed different levels of coated zinc oxide (Mean values with their standard errors)

LZ, piglets fed at a dose of 250 mg Zn (ZnO)/kg; HZ, piglets fed at a dose of 2250 mg Zn (ZnO)/kg; CZ250, piglets fed at a dose of 250 mg Zn (coated ZnO)/kg of basal diet; CZ380, piglets fed at a dose of 380 mg Zn (coated ZnO)/kg of basal diet; CZ570, piglets fed at a dose of 570 mg Zn (coated ZnO)/kg of basal diet; CZ760, piglets fed at a dose of 760 mg Zn (coated ZnO)/kg of basal diet; CZ1140, piglets fed at a dose of 1140 mg Zn (coated ZnO)/kg of basal diet; L, linear; Q, quadratic; C, cubic.
a,b,c,d,e,fMean values within a row with unlike superscript letters were significantly different (P< 0·05).
Zinc concentrations of liver, kidney, serum and faeces
Zn concentrations in the serum, liver and kidney of weaned piglets fed different levels of coated ZnO were not significantly different from that of the LZ-fed group (Table 5; P>0·05), but the HZ-fed group had higher zinc concentrations (P< 0·05) than all the coated ZnO-supplemented groups except the CZ1140-fed group, as Zn concentration in the serum of the CZ1140-fed group was not significantly different when compared with the HZ-fed group. Zn concentrations in the faeces of weaned piglets increased linearly with the increasing levels of coated ZnO (P< 0·01). When coated ZnO was added at the level of 1140 mg Zn/kg, it reached the highest value (5020 mg Zn/kg), which was 321 % higher than that of the LZ-fed group and 47 % lower than that of the HZ-fed group. Nevertheless, Zn concentration in the faeces of the CZ380-fed group was 79 % lower than that of the HZ-fed group.
Table 5 Zinc concentrations in the serum, liver, kidney and faeces of weaned piglets fed different levels of coated zinc oxide (Mean values with their standard errors)

LZ, piglets fed at a dose of 250 mg Zn (ZnO)/kg; HZ, piglets fed at a dose of 2250 mg Zn (ZnO)/kg; CZ250, piglets fed at a dose of 250 mg Zn (coated ZnO)/kg of basal diet; CZ380, piglets fed at a dose of 380 mg Zn (coated ZnO)/kg of basal diet; CZ570, piglets fed at a dose of 570 mg Zn (coated ZnO)/kg of basal diet; CZ760, piglets fed at a dose of 760 mg Zn (coated ZnO)/kg of basal diet; CZ1140, piglets fed at a dose of 1140 mg Zn (coated ZnO)/kg of basal diet; L, linear; Q, quadratic; C, cubic.
a,b,c,d,e,fMean values within a row with unlike superscript letters were significantly different (P< 0·05).
Secretory IgA concentrations in the jejunal mucosa of weaned piglets
The concentrations of SIgA in the jejunal mucosa of weaned piglets fed different levels of coated ZnO are shown in Fig. 1. Compared with the LZ-fed group, the CZ380-, CZ570-, CZ760- and CZ1140-fed groups had higher SIgA concentrations in the jejunal mucosa (P< 0·05); of these, the CZ570-fed group had the highest concentration, but was not significantly difference from that of the HZ-fed group.
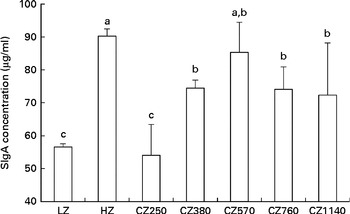
Fig. 1 Secretory IgA (SIgA) concentrations in the jejunal mucosa of weaned piglets fed different levels of coated zinc oxide (ZnO). Values are means, with their standard errors represented by vertical bars. a,b,cMean values with unlike letters were significantly different (P< 0·05). LZ, piglets fed at a dose of 250 mg Zn (ZnO)/kg; HZ, piglets fed at a dose of 2250 mg Zn (ZnO)/kg; CZ250, piglets fed at a dose of 250 mg Zn (coated ZnO)/kg of basal diet; CZ380, piglets fed at a dose of 380 mg Zn (coated ZnO)/kg of basal diet; CZ570, piglets fed at a dose of 570 mg Zn (coated ZnO)/kg of basal diet; CZ760, piglets fed at a dose of 760 mg Zn (coated ZnO)/kg of basal diet; CZ1140, piglets fed at a dose of 1140 mg Zn (coated ZnO)/kg of basal diet.
Gene expressions of insulin-like growth factor 1, tight junctions and inflammatory cytokines in the jejunal mucosa of weaned piglets fed different levels of coated zinc oxide
As shown in Table 6, mRNA level of IGF-1 in the jejunal mucosa increased significantly (P< 0·05) as coated ZnO was added up to the level of 380 mg Zn/kg, at which the IGF-1 mRNA level was the highest, and then kept decreasing consistently until it reached the lowest value (1140 mg Zn/kg). Compared with the LZ- and HZ-fed groups, the CZ250-, CZ380- and CZ570-fed groups expressed higher IGF-1 mRNA levels (P< 0·05), and the mRNA levels of IGF-1 for the CZ380-fed group were approximately 345 and 189 % higher than those for the LZ- and HZ-fed groups, respectively (P< 0·05). Additionally, the HZ-fed group had 54 % higher IGF-1 mRNA level than the LZ-fed group (P< 0·05). ZO-1 mRNA levels were up-regulated as coated ZnO was added at the level of 380 mg Zn/kg, compared with that of the LZ-fed group (P< 0·05), but other levels of coated ZnO did not regulate the gene expression levels of ZO-1. Occludin mRNA levels were up-regulated in the coated ZnO-fed groups, except the CZ1140-fed group, compared with that of the LZ-fed group (P< 0·05). Moreover, occludin mRNA levels for the CZ380- and CZ570-fed groups were not significantly different compared with that of the HZ-fed group. TNF-α mRNA levels significantly decreased with the increasing levels of coated ZnO up to 570 mg Zn/kg (P< 0·05), and plateaued subsequently. IL-6 mRNA levels showed a similar trend to the gene expression levels of TNF-α, but plateaued later when the level of coated ZnO was increased up to 760 mg Zn/kg. A similar change in trends was also observed in the gene expression levels of TGF-β1 and IL-10: TGF-β1 mRNA level reached the peak when the level of coated ZnO was increased up to 380 mg Zn/kg, while IL-10 mRNA level did so when increased up to the level of 570 mg Zn/kg. Compared with the LZ-fed group, piglets fed the 250 and 380 mg Zn (coated ZnO)/kg diets had significantly higher TGF-β1 mRNA levels (P< 0·05), but were not significantly different from the other groups. IL-10 mRNA levels of all the coated ZnO-supplemented groups were higher than that of the LZ-fed group, whereas only the CZ570-fed group had a higher gene expression level of IL-10 than the HZ-fed group (P< 0·05). There were cubic effects (P< 0·05) of the coated ZnO dose on the gene expression levels of IGF-1, TJ and inflammatory cytokines, except IL-10 which showed a quadratic (P< 0·01) response to the coated ZnO dose.
Table 6 Gene expressions of insulin-like growth factor 1 (IGF-1), tight junctions and inflammatory cytokines in the jejunal mucosa of weaned piglets fed different levels of coated zinc oxide (Mean values with their standard errors)
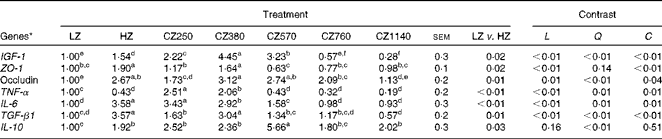
LZ, piglets fed at a dose of 250 mg Zn (ZnO)/kg; HZ, piglets fed at a dose of 2250 mg Zn (ZnO)/kg; CZ250, piglets fed at a dose of 250 mg Zn (coated ZnO)/kg of basal diet; CZ380, piglets fed at a dose of 380 mg Zn (coated ZnO)/kg of basal diet; CZ570, piglets fed at a dose of 570 mg Zn (coated ZnO)/kg of basal diet; CZ760, piglets fed at a dose of 760 mg Zn (coated ZnO)/kg of basal diet; CZ1140, piglets fed at a dose of 1140 mg Zn (coated ZnO)/kg of basal diet; L, linear; Q, quadratic; C, cubic; ZO-1, zonula occludens protein-1; TGF-β1, transforming growth factor β 1.
a,b,c,d,e,fMean values within a row with unlike superscript letters were significantly different (P< 0·05).
* Relative mRNA levels were normalised using TBP (TATA box-binding protein, reference gene).
Microbiota composition in the intestine
The PCR-DGGE profiles of V3 amplicons obtained from the microbiota in the jejunum and faeces are presented in online supplementary Attachments 1 and 2. Microbiota richness and the Shannon diversity index determined by DGGE analyses in the jejunal digesta and faeces of weaned piglets fed different levels of coated ZnO are presented in Fig. 2. As shown in Fig. 2, almost all the piglets showed the same variation trend, and microbiota richness and the Shannon diversity index decreased with the increasing levels of coated ZnO up to 380 mg Zn/kg, and then continuously increased. As shown in Fig. 2(A), compared with the LZ-fed group, the CZ250 and CZ380-fed groups had lower microbiota richness in the jejunal digesta (P< 0·05), whereas only the CZ380-fed group had the lower value (P< 0·05) in the faeces (Fig. 2(B)). In the jejunal digesta, Shannon diversity indices were lower (P< 0·05) in the CZ250- and CZ380-fed groups than in the LZ-fed group, but were not significantly different from the other groups (Fig. 2(C)). In the faeces of the CZ380- and CZ570-fed groups, Shannon diversity indices were found to be lower (P< 0·05; Fig. 2(D)). Among the coated ZnO-fed groups, the CZ380-fed group had the lowest richness and Shannon diversity index of microbiota in both jejunal digesta and faeces.
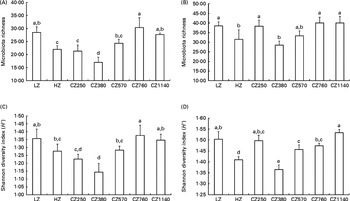
Fig. 2 Microbiota richness and Shannon diversity index determined by denaturing gradient gel electrophoresis analyses in (A, C) the jejunal digesta and (B, D) the faeces of weaned piglets fed different levels of coated zinc oxide (ZnO). Values are means, with their standard errors represented by vertical bars. a,b,c,dMean values with unlike letters were significantly different (P< 0·05). LZ, piglets fed at a dose of 250 mg Zn (ZnO)/kg; HZ, piglets fed at a dose of 2250 mg Zn (ZnO)/kg; CZ250, piglets fed at a dose of 250 mg Zn (coated ZnO)/kg of basal diet; CZ380, piglets fed at a dose of 380 mg Zn (coated ZnO)/kg of basal diet; CZ570, piglets fed at a dose of 570 mg Zn (coated ZnO)/kg of basal diet; CZ760, piglets fed at a dose of 760 mg Zn (coated ZnO)/kg of basal diet; CZ1140, piglets fed at a dose of 1140 mg Zn (coated ZnO)/kg of basal diet.
Dendrograms showing the clustering of the DGGE profiles of the jejunal digesta and faeces are presented in Fig. 3. In the jejunal digesta, the similarity indices in the profiles of each group ranged from 0·68 to 0·82, and the microbial communities of each group formed different small clusters according to the levels of coated ZnO added in the diets. The similarity index was the highest (0·82) for the microbial communities of the CZ250- and CZ380-fed groups, and interestingly, those of the CZ570- and HZ-fed group clustered together, whose similarity index was about 0·79. In addition, microbial communities of the CZ760- and CZ1140-fed groups tended to form a cluster. In the faeces, similarly, some different small clusters were also formed, such as those of the CZ250- v. CZ570-fed group and the CZ760- v. CZ1140-fed group, with the similarity index of both being approximately 0·76. The microbial communities of the HZ-fed group formed a cluster with those of the CZ250- v. CZ570-fed group, whose similarity index was approximately 0·72.
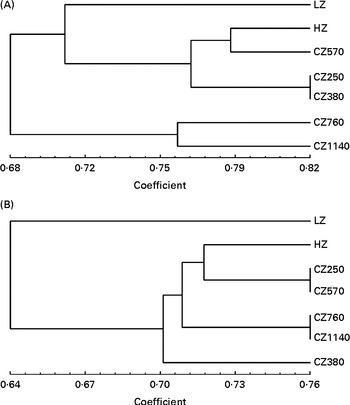
Fig. 3 Cluster analysis of denaturing gradient gel electrophoresis profiles of microbial communities in (A) the jejunal digesta and (B) the faeces of weaned piglets fed different levels of coated zinc oxide (ZnO). LZ, piglets fed at a dose of 250 mg Zn (ZnO)/kg; HZ, piglets fed at a dose of 2250 mg Zn (ZnO)/kg; CZ250, piglets fed at a dose of 250 mg Zn (coated ZnO)/kg of basal diet; CZ380, piglets fed at a dose of 380 mg Zn (coated ZnO)/kg of basal diet; CZ570, piglets fed at a dose of 570 mg Zn (coated ZnO)/kg of basal diet; CZ760, piglets fed at a dose of 760 mg Zn (coated ZnO)/kg of basal diet; CZ1140, piglets fed at a dose of 1140 mg Zn (coated ZnO)/kg of basal diet.
As shown in Fig. 4, the relative abundances of Lactobacillus in faecal samples had a negative correlation with the levels of coated ZnO added, in which the CZ250-, CZ380- and CZ570-fed groups had the higher relative abundances than the LZ- and HZ-fed groups (P< 0·05). The relative abundances of E. coli increased when coated ZnO was added at the levels of 380 and 570 mg Zn/kg, but decreased when added up to the level of 760 mg Zn/kg. In addition, in the HZ-fed group, the relative abundance of Lactobacillus significantly decreased, in contrast to that of E. coli which significantly increased compared with the LZ-fed group (P< 0·05).

Fig. 4 Relative abundances of (A) Lactobacillus □ and (B) Escherichia coli □ in the faecal samples from weaned piglets fed different levels of coated zinc oxide (ZnO). Values are means, with their standard errors represented by vertical bars. a,b,c,dMean values with unlike letters were significantly different (P< 0·05). LZ, piglets fed at a dose of 250 mg Zn (ZnO)/kg; HZ, piglets fed at a dose of 2250 mg Zn (ZnO)/kg; CZ250, piglets fed at a dose of 250 mg Zn (coated ZnO)/kg of basal diet; CZ380, piglets fed at a dose of 380 mg Zn (coated ZnO)/kg of basal diet; CZ570, piglets fed at a dose of 570 mg Zn (coated ZnO)/kg of basal diet; CZ760, piglets fed at a dose of 760 mg Zn (coated ZnO)/kg of basal diet; CZ1140, piglets fed at a dose of 1140 mg Zn (coated ZnO)/kg of basal diet.
Discussion
Early-weaned piglets, under the influence of weaning stress, usually encounter digestive disorders, diarrhoea and weakened immune system, which could jeopardise their health severely( Reference Owusu-Asiedu, Nyachoti and Marquardt 1 ). Piglet weaning stress is caused by three aspects of factors: psychology; nutrition; environment. ZnO, as a kind of micronutrient Zn source, has been used to alleviate the stress of weaned piglets for a long period. Much research has shown that pharmacological doses of Zn (2000–4000 mg Zn/kg) from ZnO, as a replacement of in-feed antibiotics, could improve growth performance and decrease the incidence of diarrhoea in piglets( Reference Carlson, Hill and Link 4 , Reference Case and Carlson 21 , Reference Castillo, Martín-Orúe and Manzanilla 22 ). In the present study, no improvements in average daily gain, average daily feed intake or feed:gain ratio were found in the piglets of the HZ-fed group, which is consistent with some previous reports( Reference Schell and Kornegay 23 , Reference Tokach, Tokach and Goodband 24 ). There was no incidence of diarrhoea in the present study as the diarrhoea index of all the groups remained quite low ( < 1), which could be due to the diets containing spray-dried plasma protein that can reduce the episodes of diarrhoea( Reference Niewold, van Dijk and Geenen 25 ). However, the diarrhoea index decreased in the HZ-fed group, as well as in the coated ZnO-fed groups (CZ380 and CZ570). The aforementioned results confirmed the assumption that ZnO probably functions at the site of the gastrointestinal tract to reduce the incidence of diarrhoea, mainly in its molecular form. A small percentage of ZnO can reach its site of action, because most of the ZnO, which is an easily dissociative substance in the gastric juice, is dissociated into free Zn ions when passing through the stomach. This is the reason why the addition level of ZnO has to reach up to 2000–4000 mg Zn/kg for the purpose of reducing the incidence of diarrhoea. When coated ZnO was added, as a replacement of ZnO (uncoated), the percentage of the ZnO molecule reaching the gastrointestinal tract is markedly increased, which is due to the protective enteric coating of the inner ZnO. Therefore, in the present study, low levels of coated ZnO (380 or 570 mg Zn/kg) were comparable with the pharmacological level of ZnO (uncoated, 2250 mg Zn/kg) in alleviating the incidence of diarrhoea. Hu et al. ( Reference Hu, Song and Li 8 ) reported that feeding diosmectite–ZnO at a low dose of 500 mg Zn/kg alleviated the incidence of diarrhoea in piglets during days 0–14 post-weaning, which was similar to the feeding of ZnO at a high concentration (2250 mg Zn/kg). Kim et al. ( Reference Kim, Hansen and Mullan 11 ) demonstrated that the addition of lipid-coated ZnO at a dose of 100 mg Zn/kg was dramatically effective in controlling post-weaning diarrhoea when piglets were challenged with the enterotoxigenic E. coli (ETEC) strain, compared with the addition of ZnO at a dose of 3000 mg Zn/kg. The difference in the optimal addition level of coated ZnO in the two different studies may be caused by two factors: the difference in the ingredient and its proportion of enteric coating, and the difference in Zn concentration of the basal diet. In the present study, Zn concentration of the basal diet was 29·39 mg/kg, whereas in the study of Kim et al. ( Reference Kim, Hansen and Mullan 11 ), it was 198 mg/kg. Additionally, we found that there were quadratic and cubic effects of coated ZnO on diarrhoea index. Once the dose of coated ZnO was increased to a certain level, no benefit would be found in alleviating the incidence of diarrhoea. As it is well known, supplementation with a pharmacological level of ZnO could cause excessive Zn residues in animals and environment pollution, because a large amount of unabsorbed Zn is excreted in the faeces. In the present study, the Zn concentrations in the serum, liver and kidney of the HZ-fed group were significantly higher than that of the LZ-fed group, but were not significantly different for all the coated ZnO-fed groups when compared with the LZ-fed group. This observation demonstrated that animals were able to effectively regulate Zn absorption within the concentration limits of Zn; nevertheless, once Zn dosage exceeds a certain threshold, the regulatory function would not work well. In addition, the results of the present study indicated that Zn concentrations in the faeces increased with the increasing levels of Zn. Therefore, lower levels of Zn would result in lighter pollution of the heavy metal Zn.
Small-intestinal epithelium is the main digestive and absorptive site of nutrients; moreover, it plays an important role as the intestinal immune barrier in resisting the invasion of environmental pathogens. Consequently, the integrity of the intestinal villus–crypt morphological structure is very important for animal health. Weaning stress is often associated with villus atrophy and crypt hyperplasia( Reference Montagne, Boudry and Favier 26 ), while a pharmacological level of ZnO can help control the symptoms: increasing villus height and reducing crypt depth in the small intestine of weaned piglets( Reference Li, Van Kessel and Caine 27 , Reference Li, Yin and Li 28 ). In the present study, piglets fed diets containing a high dose of Zn had a higher villus height and/or a lower crypt depth in the duodenum, jejunum and ileum than those fed the low-dose Zn diets, which corresponds with previous results, and villus heights in the duodenum of piglets fed the coated ZnO diets at the levels of 250, 380 or 570 mg Zn/kg did not differ from those of the HZ-fed piglets. This observation indicated that low levels of coated ZnO had similar effects to those of high levels of ZnO in improving intestine morphology. Gastrointestinal development of piglets is regulated by external and intrinsic factors( Reference Pluske, Hampson and Williams 29 ); the former mainly include intrauterine growth retardation, weaning stress and diet, while the latter are mediators involving enterocyte proliferation and differentiation. IGF-1, which can be synthesised locally in the gastrointestinal tract, is an important mediator of enterocyte proliferation and differentiation( Reference Jones and Clemmons 30 ). The results of Li et al. ( Reference Li, Yin and Li 28 ) suggested that 3000 mg Zn (ZnO)/kg can enhance the mRNA expression of IGF-1 and IGF-1 receptor (IGF-1R) in the mucosa of weaned piglets. Alexander & Carey( Reference Alexander and Carey 31 ) demonstrated that oral IGF-1 significantly increased villus heights in the jejunum and ileum as well as the intestinal weight of piglets. In the present study, the induced gene up-regulation of IGF-1 in the jejunal mucosa was found in the HZ-, CZ250-, CZ380- and CZ570-fed groups. Furthermore, these three coated ZnO-fed groups had higher IGF-1 mRNA levels than the HZ-fed group, and there were linear, quadratic and cubic effects of coated ZnO on the mRNA expression levels of IGF-1. These observations demonstrated that both high-dose ZnO and low-dose coated ZnO could enhance the mRNA expression levels of IGF-1 in the mucosa, while the improvements in small-intestinal morphology by feeding coated ZnO may be just partly attributed to an enhancement of IGF-1 expression in the gastrointestinal tract.
One of the major functions for the small-intestinal epithelium is serving as a barrier against noxious antigens and pathogens. Impairment of intestinal epithelium can increase intestinal permeability, which will promote the translocation of intestinal bacteria and the entering of toxic or allergenic substances from the gut into the body( Reference Wijtten, Meulen and Verstegen 32 ). TJ, a network of proteins connecting the epithelial cells, plays a crucial role in maintaining gut barrier function. TJ mainly consists of transmembrane protein complexes and the cytosolic proteins ZO, thus the expression levels of ZO-1 and occludin, the key proteins of TJ, are consistently associated with barrier function( Reference Musch, Walsh-Reitz and Chang 33 ). It has been reported that the addition of ZnO at a dose of 2000 mg Zn/kg reduced intestinal permeability by increasing the expression of the TJ proteins ZO-1 and occludin in weaned piglets( Reference Zhang and Guo 34 ), which is, at least partly, the reason for reducing the incidence of diarrhoea. In the present study, both mRNA levels of ZO-1 and occludin were enhanced with the addition of ZnO at the level of 2250 mg Zn/kg, and the same effect was also found at the low level of coated ZnO (380 mg Zn/kg). However, once the ZnO amount in the small intestine exceeds a certain level, TJ proteins will be impaired, resulting in the dysfunction of the intestinal mucosal immune barrier.
Absorptive enterocytes, the major group of intestinal epithelial cells, which cover the surface of the small intestine, can express polymeric Ig receptor( Reference Goto and Kiyono 35 ). This polymeric Ig receptor binds to the dimeric IgA secreted by lamina propria plasma cells, on the basal membrane of polarised intestinal epithelial cells for the transcytosis of dimeric IgA to apical membrane. Endoproteolytic cleavage of polymeric Ig receptor near the plasma membrane releases secretory component with cargo (dimeric IgA) into the lumen for the formation of the SIgA complex( Reference Johansen and Kaetzel 36 ). SIgA has been shown to play a crucial role in the mucosal immune system of the intestine as the first line of specific defence, including the ability to neutralise toxins produced by pathogenic bacteria and to prevent adherence and invasion by pathogenic bacteria. It has been reported that low concentrations of SIgA are associated with increased bacterial adherence to the mucosa( Reference Rodrigues, Cara and Fretez 37 ). SIgA secretion can be regulated by nutrients, such as fatty acids( Reference Pérez-Cano, Ramírez-Santana and Molero-Luís 38 ), amino acids( Reference Imaeda, Miura and Serizawa 39 ) and vitamins( Reference Yang, Yuan and Tao 40 ). As it is well known, Zn takes part in regulating intestinal mucosal immunity. In the present study, a high level of ZnO at 2250 mg Zn/kg and a lower level of coated ZnO at 570 mg Zn/kg had almost the same function in up-regulating the SIgA level, and intestinal SIgA levels were also increased when piglets received coated ZnO at the levels of 380, 760 and 1140 mg Zn/kg. In contrast, previous studies showed that Zn supplementation had no effects on increasing levels of SIgA from the stool or urine of human subjects( Reference Bates, Evans and Dardenne 41 , Reference Raqib, Roy and Rahman 42 ). It may lead to different results that Zn supplementation comes from different Zn sources: ZnO or zinc acetate.
It has been reported that development of intestinal inflammation could be the consequence of abnormal penetration of commensal bacteria through the breakage of the intestinal epithelial barrier via an acceleration of apoptotic intestinal epithelial cells and subsequent hyperactivation of mucosal immune cells to produce acute and chronic pathological inflammatory reactions( Reference Goto and Kiyono 35 ). With the penetration of intense bacteria into intestinal tissues, hyperproduction of pro-inflammatory cytokines, such as TNF-α and IL-6, would be synthesised and then secreted in mucosal immune cells, causing further damage to the mucosal barrier system. Therefore, pro-inflammatory cytokines can be as the monitoring markers of inflammatory reactions( Reference Borovikova, Ivanova and Zhang 43 ). In contrast, anti-inflammatory cytokines are suggested to play a vital role in anti-inflammatory response during inflammation conditions. IL-10 suppresses the production of pro-inflammatory cytokines by means of inhibiting the translocation of NF-κB, which is involved in the regulation of inflammatory cytokine expression( Reference Oberholzer, Oberholzer and Moldawer 44 ). The present study has demonstrated that pharmacological concentration of ZnO inhibited the gene expression of TNF-α, but enhanced that of IL-10 and TGF-β1, which corresponds with the previous results. The gene up-regulation of IL-10, TGF-β1, TNF-α and IL-6 in the jejunal mucosa was observed in piglets fed diets containing coated ZnO at the levels of 250 and 380 mg Zn/kg, whereas TNF-α mRNA level markedly decreased with the addition of coated ZnO up to the level of 570 mg Zn/kg, and IL-10 mRNA level was more than twice as much as that of HZ with the addition of coated ZnO at the level of 570 mg Zn/kg. Sargeant et al. ( Reference Sargeant, Miller and Shaw 45 ) reported that ZnO reduced the inflammatory response of epithelial IPEC J2 cells (intestinal porcine epithelial cells) infected by ETEC, counteracted the up-regulation of IL-6 mRNA, but increased TNF-α mRNA level. Hu et al. ( Reference Hu, Song and Li 8 ) suggested that the mRNA levels of TNF-α and IL-6 in the mucosa of piglets were reduced with the addition of ZnO at the level of 2250 mg Zn/kg. In summary, lower concentration of coated ZnO, to a certain extent, has the function of inhibiting the overproduction of pro-inflammatory cytokines and promoting the expression of anti-inflammatory cytokines.
Intestinal microecology, consisting of the four main components – the epithelium, the secretions, the nutrients and the microbiota, is a quite dynamic process( Reference Floch 46 ), so its balance is crucial in intestinal health. The microbiota is highly diverse, in health, it keeps constantly changing with the variables of intestinal ecology caused by geography and diet, but is dynamically stable. Once the stability of the intestinal microbiota is disturbed, it will lead to the overgrowth of indigenous and exogenous pathogenic bacteria, and animals will be more vulnerable to intestinal disease (diarrhoea)( Reference Bhandari, Xu and Nyachoti 47 ). It has been proved that antibiotics can decrease the incidence of diarrhoea by way of exerting an impact on intestinal microbiota according to the decrease in richness and the Shannon diversity index( Reference Wang, Wang and Wang 48 ). The nutritional manipulation of animals during the weaning period could also change the composition of the microbiota in the gastrointestinal tract( Reference Lubbs, Vester and Fastinger 49 , Reference Gong, Yu and Liu 50 ). In the present study, microbiota species richness and the Shannon diversity index were used to assess microbiota diversity in the intestine of piglets, the former is a statistical estimator of the number of distinct species present, and the latter is a weighing of the abundance of distinct species. The results indicated that supplementation of coated ZnO at the level of 380 mg Zn/kg or of ZnO at the level of 2250 mg Zn/kg to weaned piglets reduced the microbiota species richness and Shannon diversity index in the jejunal digesta and faeces, suggesting that both high-dose ZnO and low-dose coated ZnO have an effect on intestinal microbiota diversity as antibiotics do. In addition, the cluster analysis of the DGGE profiles of the jejunal digesta and faeces demonstrated that the intestinal microbiota of piglets fed a low concentration of coated ZnO (250, 380 or 570 mg Zn/kg) had a higher similarity to that of piglets fed a high concentration of ZnO (2250 mg Zn/kg). The above-mentioned analysis indicated that a low concentration of coated ZnO can exert a vital impact on the composition of the intestinal microbiota, as does a high concentration of ZnO. As enteropathogenic E. coli is one of the main factors causing diarrhoea in piglets, a hypothesis was put forward that pharmacological concentration of ZnO can protect weaned piglets against diarrhoea mainly by inhibiting the growth of enteropathogenic E. coli in the digestive tract. However, the results of many experimental studies did not support the hypothesis. Jensen-Waern et al. ( Reference Jensen-Waern, Melin and Lindberg 51 ) reported that supplementation of 2500 mg Zn (dietary ZnO)/kg had no effect on the population of E. coli in the faeces. Similar results were observed in the study by Li et al. ( Reference Li, Van Kessel and Caine 27 ), which reported that there was no effect of supplementary ZnO (3000 mg Zn/kg) on Enterobacteriaceae and Lactobacillus populations in the ileal digesta or faeces. Interestingly, Højberg et al. ( Reference Højberg, Canibe and Poulsen 52 ) found that the amounts of Lactobacillus were reduced, whereas the coliforms were more numerous in piglets, when receiving the high-ZnO dose (2500 mg Zn/kg). The results of the study by Broom et al. ( Reference Broom, Miller and Kerr 53 ) suggested that supplementation with 3100 mg Zn (ZnO)/kg decreased the colony counts of lactic acid bacteria, especially the dominant species of Lactobacillus (Lactobacillus reuteri), but increased the relative abundance of Enterobacteriaceae spp., except for E. coli that was not affected. In the present study, when piglets were fed ZnO at a dose of 2250 mg Zn/kg, the relative abundance of Lactobacillus was down-regulated, while that of E. coli was up-regulated, which were similar to the results reported by Højberg et al. ( Reference Højberg, Canibe and Poulsen 52 ) Additionally, lower concentrations of coated ZnO (380 and 570 mg Zn/kg) had significant positive effects on the growth of both Lactobacillus and E. coli. Therefore, from these results, we conclude that it is likely that ZnO protects weaned piglets from diarrhoea not by the direct antibacterial effect on E. coli. Roselli et al. ( Reference Roselli, Finamore and Garaguso 7 ) found that ZnO treatment (1 mm) reduced the number of ETEC adhesion and invasivity of Caco-2 cells, while the number of ETEC grown in a medium with and without ZnO (1 mm) had no difference. This observation indicated that ZnO may not retard the growth or development of ETEC, but can inhibit the adhesion and internalisation of intestinal epithelial cells, and thus decrease the breakage of the intestinal epithelial barrier.
In conclusion, a low concentration of coated ZnO (380 or 570 mg Zn/kg) can alleviate diarrhoea by promoting intestinal development, protecting the intestinal mucosal barrier from damage, stimulating the mucosal immune system and regulating the intestinal microbiota, as does a high concentration of ZnO. Moreover, compared with a high concentration of ZnO, a low concentration of coated ZnO prevented the accumulation of excessive Zn in animals and reduced the Zn concentration of excreted faeces, so as to achieve the goal of saving Zn source and reducing environmental pollution of Zn. Additionally, it must be noted that the dose of coated ZnO used in the present study reduced significantly compared with that of ZnO supported by clay mineral carriers in previous studies; however, it is still supra-physiological for piglets. The present results demonstrate that using coated ZnO is a feasible means to further reduce the dose of ZnO to near-physiological concentration of Zn for piglets.
Supplementary material
To view supplementary material for this article, please visit http://dx.doi.org/10.1017/S0007114514000300
Acknowledgements
The present study was supported by the Shuangzhi Project of Sichuan Agricultural University, and GuangZhou Wisdom Bio-Tech Company Limited, which had a role in the design of this article.
The contributions of the authors were as follows: Z. W., A. Z., S. W. and Y. L. designed the trial; J. S., M. H., X. Z., Y. C. and A. Z. conducted the feeding experiment; J. S. and Y. C. were responsible for the laboratory analysis; J. S. analysed the data and wrote the manuscript; Z. W., B. X., L. W., L. M., X. Z., H. Z. and Q. P. revised the manuscript; H. Z. and Q. P. offered technical assistance.
None of the authors has any conflicts of interest to declare.