Introduction
By means of trace fossils, ichnology studies the evidence of interactions between organisms and substrates as a result of their life activity in the geological past. Therefore, ichnology is part of paleoecological analysis, which can be defined as the study of at least one of the three possible pairs of relationships established in ecosystems: (1) interaction between taxa, (2) influence of taxa on the environment, and/or (3) influence of the environment over the taxa.
Trace fossils are the biogenic structures (also called ethological structures) that record the activity of organisms in the geologic past, and are divided into three broad categories: (1) biogenic sedimentary structures, (2) bioerosion structures, and (3) other evidence of activity. The biogenic sedimentary structures are those produced by the activity of organisms on or in unconsolidated substrates. They include bioturbation structures (e.g., burrows, trails, footprints), and evidence of biodeposition (e.g., coprolites, fecal pellets) and biostratification (e.g., microbial mats). The bioerosion structures originate by mechanical and/or chemical biological activity on hard inorganic (rock) or organic (woody, calcareous or phosphatic skeletons) substrates. Structures such as eggshells, cobwebs, etc. fit in the catchall of other evidences. Seilacher (Reference Seilacher1953) proposed a classification system of trace fossils based on the reflected behavior. From this first proposal to the present, numerous additions and refinements to the initial scheme have been suggested. Such proliferation complicates an effective classification since many traces are the result of several simultaneous or successive behaviors (see Vallon et al., Reference Vallon, Rindsberg and Bromley2016 and references therein).
Our study has involved the compilation of available information on traces made by, received by, or directly related to echinoderms, from the Cambrian to the Recent. The highly diverse echinoderms can be common in the fossil record in various marine environments, which has facilitated their use in numerous paleobiological studies. In parallel, their ichnological record may be composed of both direct and indirect evidence: (1) direct evidence corresponding to the bioturbation and bioerosion structures resulting from the different burrowing and boring behaviors that may be conducted by many groups of echinoderms (mainly echinoids, asteroids, ophiuroids and holothurians); and by contrast, (2) indirect evidence, not directly produced by echinoderms as such, but produced in or on their skeletons (syn-vivo or post-mortem interactions) or, at least, in or on some of their component skeletal elements. These, mainly consist of traces related to predation or symbiotic relationships with various other organisms and even to the exploitation of their skeletons, ossicles and/or spines to construct burrow linings or domiciles.
The following sections constitute an extensive review of the present-day knowledge on the modern and fossil record of ichnologic structures related to echinoderms. The high ichnodiversity of echinoderm-related traces is tracked along the geological record. This allows the fidelity of the traces to be followed and records the potential ecological behavior of the echinoderm taxa in different geological periods.
Modern and fossil echinoderm ichnological record
Among both fossil and modern echinoderms, there are active and significant burrowers and borers (mainly echinoids, but also asteroids, ophiuroids, holothurians and crinoids); consequently, their burrowing and boring behaviors have a high potential to produce an extensive ichnological record (Fig. 1). To date, 22 ichnogenera have been erected (leaving aside ichnotaxonomic discussions; see Table 1), which correspond to bioturbation and bioerosion structures attributable to echinoderms.
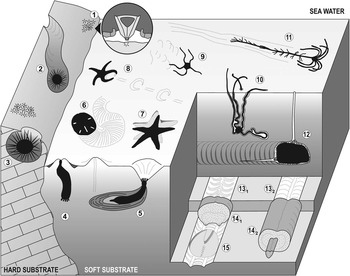
Figure 1 Diagram showing bioturbation and bioerosion of the main groups of extant echinoderms. Regular echinoid bioerosion: (1) bite traces (Gnatichnus-like) produced by grazing on hard substrates (detail of the Aristotle’s lantern within the circle); (2) long and continuous grooves (Ericichnus-like) attributed to a pascichnial/agrichnial or domichnial behavior; and (3) circular pits (Circolites-like) used as more or less permanent domiciles. Holothurian bioturbation: (4) simple vertical shaft and (5) thickly laminated burrow (Artichnus-like) produced by molpadid holothurians (modified from Ayranci and Dashtgard, Reference Ayranci and Dashtgard2013). Irregular echinoid bioturbation: (6) surficial and meniscate locomotion trace produced by the sand dollar Mellita quinquiesperforata. Asterozoan bioturbation: (7) sea star resting trace (Asteriacites-like); (8) sea star locomotion trace (Arcichnus-like; modified from Sutcliffe, Reference Sutcliffe1997); (9) ophiuroid locomotion trace (Ophioichnus-like; modified from Bell, Reference Bell2004); and (10) burrow produced by the brittlestar Hemipholis elongata (modified from Christensen and Colacino, Reference Christensen and Colacino2000). Crinoid bioturbation: (11) locomotion trace of the isocrinid Neocrinus decorus (Krinodromos-like; modified from Baumiller and Messing, Reference Baumiller and Messing2007). Irregular echinoid bioturbation: (12) spatangoid ploughing through the sediment (modified from Gibert and Goldring, Reference Gibert and Goldring2008); (13) horizontal biserial menisci (Laminites-preservation; modified from Uchman, Reference Uchman1995); (14) subhorizontal, meandering, meniscate backfill burrows bearing one (14.2; Bichordites-like) or two (14.1; Scolicia-like) drains (modified from Uchman, Reference Uchman1995); and (15) spatangoid resting trace (Cardioichnus-like; modified from Smith and Crimes, Reference Smith and Crimes1983). Depicted echinoderms are not to scale.
Table 1 Ichnotaxa ascribed to echinoderm bioturbation or bioerosion. S.R.: Stratigraphic range; Etho.: Ethology; *: type ichnospecies. [1] sensu Uchman (Reference Uchman1995); [2] sensu Radwanska (1999); [3] sensu Schlirf (Reference Schlirf2012); [4] sensu Knaust and Neumann (Reference Knaust and Neumann2016); [5] sensu Mángano et al. (Reference Mángano, Buatois, West and Maples1999). Stratigraphic Range: Cam., Cambrian; E.D., Early Devonian; L.Car., late Carboniferous; T., Triassic; M.T., Middle Triassic; J., Jurassic; M.J., Middle Jurassic; E.K., Early Cretaceous; L.K., Late Cretaceous; E., Eocene; Oli., Oligocene; M., Miocene; L.M., late Miocene; E.Plio., early Pliocene; t., today. Ethology: AGR, Agrichnia; CUB, Cubichnia; Do, Domichnia; FOD, Fodinichnia; MOR, Mortichnia; PAS, Pascichnia; REP, Repichnia.
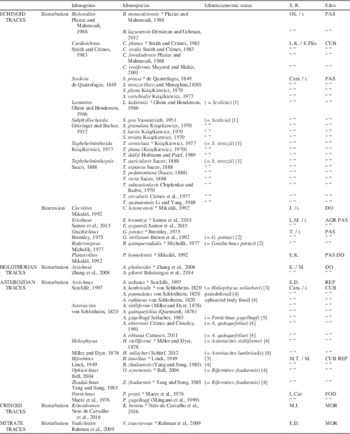
Echinoid bioturbation structures
Among bioturbation structures attributed to the burrowing activity of echinoderms, the ichnogenera Scolicia De Quatrefages, Reference De Quatrefages1849 and Bichordites Plaziat and Mahmoudi, Reference Plaziat and Mahmoudi1988 are the most common and best known, since the Jurassic and the Oligocene respectively. They consist of large horizontal to subhorizontal, meandering, meniscate backfill burrows bearing one or two drains respectively (Figs. 1.12–1.14, 2.1–2.4) (e.g., Bromley and Asgaard, Reference Bromley and Asgaard1975; Uchman, Reference Uchman1995; Bromley et al., Reference Bromley, Asgaard and Jensen1997; Gibert and Goldring, Reference Gibert and Goldring2007, Reference Gibert and Goldring2008; Seilacher, Reference Seilacher2007; Bernardi et al., Reference Bernardi, Boschele, Ferretti and Avanzini2010; Caruso and Monaco, Reference Caruso and Monaco2015).
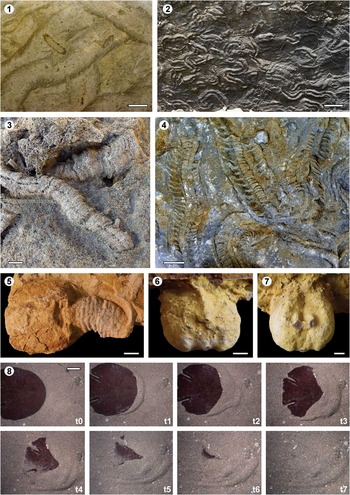
Figure 2 Echinoid bioturbation: (1) Bichordites ichnofabric (horizontal section) from the Miocene Bateig Limestone (Alicante, SE Spain; see Gibert and Goldring, Reference Gibert and Goldring2008); (2) Scolicia isp. from the Eocene of Zumaia (Gipuzkoa, N Spain); (3) Bichordites monastiriensis from the early Pliocene of the Guadalquivir Basin (Cádiz, SW Spain; see Aguirre et al., Reference Aguirre, Gibert and Puga-Bernabéu2010); (4) Bichordites isp. from the Eocene of Zumaia (Gipuzkoa, N Spain); (5) Cardioichnus planus associated to Scolicia isp. from the Pliocene of Sant Feliu de Llobregat (Barcelona, NE Spain); (6, 7) Cardioichnus reniformis from the Miocene of the Guadalquivir Basin (Lepe, Huelva, SW Spain), upper and lower views respectively; and (8) digging process of the sand dollar Echinodiscus auritus in the Red Sea (t0 to t7 time lapse is 1 minute). Scale bars are (1, 2) 5 cm; (3, 8) 2 cm; (4, 5, 6, 7) 1 cm.
Detailed neoichnological studies, both from aquaria and natural environments, have shown that these large meniscate traces result from the backfilling activity of spatangoid echinoids ploughing through the sediment (e.g., Kanazawa, Reference Kanazawa1992, Reference Kanazawa1995; Bromley et al., Reference Bromley, Asgaard and Jensen1997); although the older spatangoids date back to the Early Cretaceous (Villier et al., Reference Villier, Neraudeau, Clavel, Neumann and David2004), this backfilling activity is known from the Tithonian (Late Jurassic; Tchoumatchenco and Uchman, Reference Tchoumatchenco and Uchman2001). The occurrence of one or two drains (i.e., cylindrical tubes constructed to conduct water away from the burrow) is related to the presence of subanal tuft spines and corresponding tube feet of the echinoid tracemaker. Among modern spatangoids, the traces produced by the so-called Spatangus group exhibit a double drainage, while those generated by the Echinocardium group have a single one (Plaziat and Mahmoudi, Reference Plaziat and Mahmoudi1988). Various aquaria experiments have also shown that spatangoids may be fast and effective burrowers. For example, the large tropical species Moira atropos burrows at speeds of 2 cm/h and moves about 5 cm3 of sediment per hour (see Gingras et al., Reference Gingras, Pemberton, Dashtgard and Dafoe2008).
The ichnotaxa Subphyllochorda Götzinger and Becker, Reference Götzinger and Becker1932, Taphrhelminthopsis Sacco, Reference Sacco1888 and Taphrhelminthoida Książkiewicz, Reference Książkiewicz1977 were included in Scolicia by Uchman (Reference Uchman1995). This author regarded Subphyllochorda as the sole expression of Scolicia, Taphrhelminthopsis as a cast of washed-out Subphyllochorda or Scolicia, and Taphrhelminthoida as having the same morphology as that of Taphrhelminthopsis. Uchman (Reference Uchman1995) also considered the ichnogenus Laminites Ghent and Henderson, Reference Ghent and Henderson1966 as a preservational expression of different types of traces (Scolicia, Bichordites and other non-echinoid Palaeozoic burrows), lacking diagnostic features and only useful in order to informally characterize a particular preservational variant (Fig. 1.13).
Smith and Crimes (Reference Smith and Crimes1983) erected the ichnogenus Cardioichnus to designate ovate to sub-quadrate, bilobed resting traces (cubichnia) (Figs. 1.15, 2.5–2.7), which are commonly associated with Scolicia or Bichordites (e.g., Mayoral and Muñiz, Reference Mayoral and Muñiz2001; Bernardi et al., Reference Bernardi, Boschele, Ferretti and Avanzini2010); its fossil record ranges from the Late Cretaceous to the early Pliocene.
It is known that, among modern echinoids, clypeasteroids are able to produce several bioturbation structures (e.g., Seilacher, Reference Seilacher1979). Since the majority of these traces are surficial and are found in higher energy environments, they have a very low preservation potential and have not yet been identified in the fossil record (Figs. 1.6, 2.8). For example, Bell and Frey (Reference Bell and Frey1969) described the burrowing activity of the clypeasteroid Mellita quinquiesperforata and figured the surficial trails or the aggregations of small pits (linked with their five lunules) left by these sand dollars.
Echinoid bioerosion structures
The ichnogenera Gnathichnus Bromley, Reference Bromley1975, Circolites Mikuláš, Reference Mikuláš1992, Planavolites Mikuláš, Reference Mikuláš1992 and Ericichnus Santos et al., Reference Santos, Mayoral, Dumont, da Silva, Ávila, Gudveig Baarli, Cachão, Johnson and Ramalho2015 are unique bioerosion structures attributed to echinoderms, in particular to regular echinoids (e.g., Bromley, Reference Bromley1975; Warme, Reference Warme1975; Martinell, Reference Martinell1981, Reference Martinell1982, Reference Martinell1989; Meyer, Reference Meyer2011; Gibert et al., Reference Gibert, Domènech and Martinell2012; Schönberg and Wisshak, Reference Schönberg and Wisshak2014). Echinoid bioerosion may also have been very important in modern environments (e.g., Bronstein and Loya, Reference Bronstein and Loya2014 and references therein).
Gnathichnus corresponds to bite traces produced by echinoids plucking and grazing by using their jaw apparatus (and occasionally also their spines) on both organic and inorganic hard substrates (Figs. 1.1, 3.2). They are known from the Triassic and are very common in modern environments (see Bromley, Reference Bromley2004 and references therein). In addition, the Gnathichnus ichnofacies proposed by Bromley and Asgaard (Reference Bromley and Asgaard1993) highlighted the importance of this ichnotaxon as a component of trace fossil assemblages that record short-term bioerosion (praedichnia, pascichnia and fixichnia) in shellgrounds associated with moderate energy settings since the Jurassic (Gibert et al., Reference Gibert, Domènech and Martinell2007; Buatois and Mángano, Reference Buatois and Mángano2011). In addition, Radwańska (Reference Radwańska1999) regarded the ichnogenus Roderosignus Michalík, Reference Michalík1977 and the ichnospecies Gnatichnus stellarum Breton, Neraudeau and Cuenca-Boulat, Reference Breton, Neraudeau and Cuenca-Boulat1992 as synonyms of Gnathichnus and G. pentax Bromley, Reference Bromley1975, respectively.
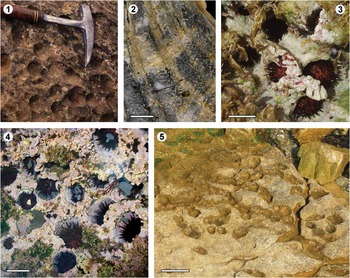
Figure 3 Echinoid bioerosion: (1) Circolites isp. from the Pliocene of Viladamat (Girona, NE Spain); (2) Gnathichnus pentax on the outer side of a pectinid valve from the Pliocene Roussillon Basin (SE France); (3) borings (Ericichnus-like) produced by regular echinoids (Echinometra lucunter) in a beach rock from San Salvador Island (Bahamas); (4) Specimens of Paracentrotus lividus boring hemispherical pits in the rocky coast of l’Estartit (Girona, NE Spain); and (5) modern Ericichnus-like borings, probably produced by Paracentrotus lividus, in the coast of Salou (Tarragona, NE Spain). Scale bars are (2) 0.5 cm; (3, 4) 5 cm; (5) 10 cm.
Circolites consists of bowl-shaped, hemispherical or deeper pits bored in hard or firm substrates and related to shallow waters (Figs. 1.3, 3.1, 3.4); its range is from the Jurassic to the Recent (e.g., Kempf, Reference Kempf1962; Peres and Picard, Reference Peres and Picard1964; Gibert et al., Reference Gibert, Martinell and Domènech1998; Bromley, Reference Bromley2004 and references therein). These bioerosion structures are mechanically produced in different kinds of substrates consisting of both sedimentary and non-sedimentary rocks (see table 1 of Santos et al., Reference Santos, Mayoral, Dumont, da Silva, Ávila, Gudveig Baarli, Cachão, Johnson and Ramalho2015). These traces have been recorded from a wide variety of substrates including beach rocks (Fig. 3.3), carbonate (e.g., Martinell and Domènech, Reference Martinell and Domènech1986; Domènech et al., Reference Domènech, Juilleret and Martinell2014), granite (e.g., Martinell, Reference Martinell1981), or basalt (e.g., Ramalho et al., Reference Ramalho, Helffrich, Schmidt and Vance2010); for example, in the case of granite, despite the fact that some of the components (e.g., quartz and feldspar) have a higher hardness than their teeth, echinoids are able to perforate these rocks using their jaw apparatus and spines to firstly detach the softer micaceous grains, which subsequently allows an easier and faster detachment of harder grains (Martinell, Reference Martinell1981). Schoppe and Werding (Reference Schoppe and Werding1996), describing the Circolites-like borings generated by the modern echinoid Echinometra lucunter in the Caribbean coast of Colombia, highlighted the presence of several organisms (as crabs, clingfish or brittle stars) cohabiting within these boreholes.
Ericichnus comprises a system of grooves with a sinuous pathway, which may be associated with isolated Circolites (Figs. 1.2, 3.5; Santos et al., Reference Santos, Mayoral, Dumont, da Silva, Ávila, Gudveig Baarli, Cachão, Johnson and Ramalho2015). Two ichnospecies have been described (Table 1; Santos et al., Reference Santos, Mayoral, Dumont, da Silva, Ávila, Gudveig Baarli, Cachão, Johnson and Ramalho2015): E. bromleyi from late Miocene to early Pliocene rocks of Santa Maria Island (Azores, Portugal), and E. asgaardi from the early Miocene of Foz da Fonte (Portugal). This kind of structure is also very common in modern settings (Fig. 3.3, 3.5).
Finally, Planavolites was erected by Mikuláš (Reference Mikuláš1992) in the early Cretaceous of the Czech Republic. This ichnogenus consists of flat and large depressions with an irregular oval or very elongated form, and was attributed to the continued boring activity of several generations of chitons, gastropods and echinoids (Mikuláš, Reference Mikuláš1992). Following Santos et al. (Reference Santos, Mayoral, Dumont, da Silva, Ávila, Gudveig Baarli, Cachão, Johnson and Ramalho2015), Planavolites and Ericihnus may be considered separate ichnogenera by: (1) displaying a different general structure and dimensions (while Planavolites is a simple and single structure, Ericichnus constitutes a complex system); and (2) the presence of Circolites associated along the Ericihnus systems (this is absent in Planavolites).
Holothurian bioturbation structures
Sea cucumbers are very important epi- and infaunal burrowers in modern seas (e.g., Dashtgard and Gingras, Reference Dashtgard and Gingras2012; Ayranci and Dashtgard, Reference Ayranci and Dashtgard2013). At the moment, however, only the ichnogenus Artichnus Zhang et al., Reference Zhang, Uchman, Chodyń and Bromley2008 has been attributed to the burrowing activity of holothurians. Artichnus consists of J- or ‘test tube’-shaped, cylindrical and blind burrows with a thick laminated lining which may consist of retrusive spreiten (Zhang et al., Reference Zhang, Uchman, Chodyń and Bromley2008; Belaústegui et al., Reference Belaústegui, Domènech and Martinell2014). At the moment, only two ichnospecies have been described: A. pholeoides Zhang et al., Reference Zhang, Uchman, Chodyń and Bromley2008 in the Eocene of Poland and A. giberti Belaústegui, Domènech and Martinell, Reference Belaústegui, Domènech and Martinell2014 in the Miocene of northeastern Spain (Figs. 1.5, 4.1, 4.2). Additionally, in the middle Miocene of the Algarve region (southern Portugal), Santos et al. (Reference Santos, Mayoral, Baarli, Cachão, Marques da Silva and Johnson2014) described a series of dwelling/equilibrium bioturbation structures that are attributed to the burrowing activity of gastrochaenid bivalves. Their descriptions and figures, however, fit perfectly with that of the ichnospecies A. giberti and the ichnogeneric assignment of this material should be revised.

Figure 4 Holothurian bioturbation: (1, 2) Artichnus giberti from the Miocene of El Camp de Tarragona Basin (NE Spain; see Belaústegui et al., Reference Belaústegui, Domènech and Martinell2014); and (3, 4) Holothuria tubulosa from the Cap de Creus (Girona, NE Spain), and detail of its excrements (Photographs courtesy of M. Ballesteros). Scale bars are (2, 4) 1 cm; (3) 3 cm.
Modern infaunal sea cucumbers are classified in three orders: Dendrochirotida, Molpadiida and Apodida (Nichols, Reference Nichols1969; Ayranci and Dashtgard, Reference Ayranci and Dashtgard2013). Some of them may construct simple burrows projecting the tentacles or the anus to the surface (e.g., Ruppert et al., Reference Ruppert, Fox and Barnes2004), or U-shaped burrows projecting both outside the sediment (e.g., Frey and Howard, Reference Frey and Howard1972; Bromley, Reference Bromley1990; Smilek and Hembre, Reference Smilek and Hembree2012) (Fig. 1.4, 1.5). This is because some holothurians must have the anus in contact with seawater for respiratory functions (Fretter and Graham, Reference Fretter and Graham1976; Ruppert et al., Reference Ruppert, Fox and Barnes2004; Zhang et al., Reference Zhang, Uchman, Chodyń and Bromley2008). Fretter and Graham (Reference Fretter and Graham1976) pointed out that the most efficient holothurian burrowers are the vermiform, apodous forms belonging to the family Synaptidae (order Apodida), which burrow by means of the tentacles and the muscular action of the body; they do not need to project the anus above the substratum for respiration (Fretter and Graham, Reference Fretter and Graham1976).
More recent neoichnological studies have shown how different holothurian taxa are able to excavate burrows comparable to the ichnogenera Arenicolites, Artichnus, Asterosoma, Diplocraterion, Skolithos or Thalassinoides, as well as surficial mounds and funnel-shaped structures (Dashtgard and Gingras, Reference Dashtgard and Gingras2012; Ayranci and Dashtgard, Reference Ayranci and Dashtgard2013). Finally, it is known that deposit-feeding sea cucumbers produce a great amount of excrement during life. These excretions consist of more or less continuous cylinders of processed sediment, which commonly exhibit equidistant constrictions along their length (Fig. 4.3, 4.4). They have, however, not yet been described in the fossil record.
Asterozoan bioturbation structures
In the fossil record, the most common traces attributed to asterozoans have been interpreted as resting traces (cubichnia) and included within the ichnogenus Asteriacites von Schlotheim, Reference Schlotheim1820. Asteriacites consists of star-shaped impressions, commonly with five radial arms that taper distally (Figs. 1.7, 5.1, 5.2) (Seilacher, Reference Seilacher1953; Knaust and Neumann, Reference Knaust and Neumann2016). Due to the lack of type material, Schirlf (2012) considered Asteriacites as a nomen dubium and proposed its synonymy with Heliophycus Miller and Dyer, Reference Miller and Dyer1878. Knaust and Neumann (Reference Knaust and Neumann2016) have, however, recently rediscovered von Schlotheim’s type material, confirming the legitimacy of Asteriacites as a valid ichnogenus, and consequently keeping H. stelliforme (the type ichnospecies) as synonym of A. stelliforme, as already proposed Osgood (Reference Osgood1970). This ichnogenus is very common from the Cambrian to the Recent (Table 1; e.g., Hess, Reference Hess1983; Crimes and Zhiwen, Reference Crimes and Zhiwen1986; Mikuláš, Reference Mikuláš1990; Mángano et al., Reference Mángano, Buatois, West and Maples1999; Jagt et al., Reference Jagt, Fraaije and van Bakel2009; Gurav et al., Reference Gurav, Kulkarni, Paranjape and Borkar2014; Baucon and Neto de Carvalho, Reference Baucon and Neto de Carvalho2016); since the older record of Asteriacites predate the first asteroid body fossil evidence (Ordovician; see Clarkson, Reference Clarkson1998), this could suggest that these organisms had already evolved from the Cambrian. Neoichnological observations have allowed noting that while starfish resting traces are morphologically more consistent, those produced by brittle stars may exhibit more variations due to the greater mobility of their arms (Seilacher, Reference Seilacher1953, Reference Seilacher2007; Ishida et al., Reference Ishida, Fujita and Kamada2004, Reference Ishida, Röper and Fujita2013).
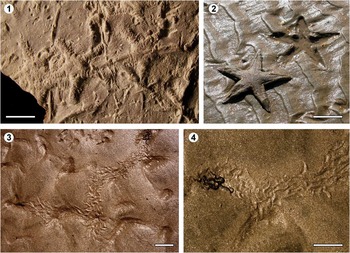
Figure 5 Asterozoan bioturbation: (1) Asteriacites isp. from the Eocene of Tavertet (Barcelona, NE Spain); (2) Astropecten irregularis and its resting trace (Asteriacites-like) in the Nueva Umbría Spit (Lepe, Huelva, SW Spain); and (3, 4) ophiuroid locomotion traces from Punta Chivato (Baja California, Mexico). Scale bars are (1) 0.5 cm; (2) 10 cm; (3, 4) 5 cm.
Four ichnogenera have been interpreted as the locomotion traces produced by asteroids and/or ophiuroids: Biformites Linck, Reference Linck1949, Zhadaichnus Yang and Song, Reference Yang and Song1985 (see also Yang et al., Reference Yang, Zhang and Yang2004), Arcichnus Sutcliffe, Reference Sutcliffe1997 and Ophioichnus Bell, Reference Bell2004. Although initially Knaust et al. (Reference Knaust, Warchoł and Kane2014) proposed Ophioichnus aysensis Bell, Reference Bell2004 as a junior subjective synonym of Zhadaichnus zhadaensis Yang and Song, Reference Yang and Song1985, Knaust and Neumann (Reference Knaust and Neumann2016) ultimately considered Ophioichnus and Zhadaichnus as synonyms of Biformites. In any case, these three ichnotaxa consist of isolated or clustered hook-shaped impressions (see Knaust and Neumann, Reference Knaust and Neumann2016) and are recorded from the Middle Triassic to the Miocene (Figs. 1.8, 1.9, 5.3, 5.4; Table 1). Locomotion traces of modern ophiuroids, very similar to Biformites/Ophioichnus/Zhadaichnus, were also figured by Schäfer (Reference Schäfer1972). By contrast, Arcichnus is characterized by an alternation (i.e., trackway) of horseshoe-shaped and straight tracks, which has been described in the Early Devonian of Germany (Sutcliffe, Reference Sutcliffe1997).
Finally, the ichnogenus Pentichnus Maerz, Kaesler and Hakes, Reference Maerz, Kaesler and Hakes1976, consisting of conical burrows with pentameral symmetry, was interpreted as the result of ophiuroid or asteroid burrowing activity; stalked crinoids were also proposed as possible tracemakers (Rindsberg, Reference Rindsberg1994). Although Chamberlain (Reference Chamberlain1971) and Seilacher (Reference Seilacher1983) interpreted these burrows as resting traces and included them within Asteriacites (as A. lumbricalis ‘hiding form’ and A. gugelhupf, respectively), subsequent authors agreed to separate these traces as different structures, i.e., domichnia instead of cubichnia (Mikuláš, Reference Mikuláš1990; Rindsberg, Reference Rindsberg1994; Mángano et al., Reference Mángano, Buatois, West and Maples1999). In fact, Mángano et al. (Reference Mángano, Buatois, West and Maples1999) proposed to better regard A. gugelhupf as Pentichnus gugelhupf. Pentichnus has been mainly recorded from Carboniferous sedimentary rocks (see Table 1).
Although they have not yet been identified in the fossil record, additional burrowing behaviors as well as bioturbation structures conducted and produced by modern brittle stars are known. Morton and Miller (Reference Morton and Miller1968) described and figured the manner in which the species Amphiura aster lives permanently buried (only the distal parts of their arms in contact with the surface) in spring-tidal flats of the New Zealand sea shore. Christensen and Colacino (Reference Christensen and Colacino2000), through aquarium observations, also described and figured the burrowing behavior of the brittle star Hemipholis elongata, which is very similar to those of A. aster (Fig. 1.10).
Asterozoan bioerosion structures
Asterozoans, mainly asteroids, are very active predators (e.g., Carter, Reference Carter1968), and there are even records of fossil sea stars showing the typical feeding posture (i.e., a humped posture over their prey with the arms wrapping around; see Blake and Guensburg, Reference Blake and Guensburg1994). The record of modern or fossil bioerosion structures preserved in the skeleton of their prey is, however, very rare. Gordillo and Archuby (Reference Gordillo and Archuby2012), under aquarium conditions, described how the periostracum (conchiolin layer) of the area around the byssus of mussel shells was removed by the action of acids discharged by the asteroid Cosmasterias lurida during predation. It seems, however, very unlikely that these traces can be recognized in the fossil record. It was also reported by Gordillo and Archuby (Reference Gordillo and Archuby2012) that, although some of the mussel shells may be crushed during these attacks, the resulting fracture patterns are indistinguishable from those caused by physical factors.
Crinoid bioturbation structures
Most crinoids exhibit a sessile lifestyle, but it is known that some stalked crinoids (comatulids and isocrinids) are able show locomotion. Although some aquarium observations have been carried out (e.g., Messing et al., Reference Messing, RoseSmyth, Mailer and Miller1988), Baumiller and Messing (Reference Baumiller and Messing2007) recorded for the first time the active locomotion of the isocrinid Neocrinus decorus by in situ observation with a submersible at a depth of 420 m near Grand Bahama Island. The resulting traces produced by the ‘elbow-crawl’ locomotion (Baumiller and Messing, Reference Baumiller and Messing2007) consist of a central and rectilinear groove (i.e., the mark left by the drag of the stalk) probably flanked by the imprints of the power strokes of the arms (Fig. 1.11). Since the submarine video filmed by Baumiller and Messing (Reference Baumiller and Messing2007) only permits clear observation of this central groove, these authors also figured the traces produced by the crawling comatulid Davidaster rubiginosa on a muddy substrate under aquarium condition; proposing that these traces could be comparable with those left by the arms of the isocrinid N. decorus.
Recently and for the first time in the trace fossil record, Neto de Carvalho et al. (Reference Neto de Carvalho, Pereira, Klompmaker, Baucon, Moita, Pereira, Machado, Belo, Carvalho and Mergulhão2016) observed a definite crinoid crawling trace and erected the new ichnotaxon Krinodromos bentou. This trace fossil consists of an irregular trail, bordered by shallow and large grooves, associated at its very end with an isocrinid crinoid body fossil from the Middle Jurassic of the Cabeço da Ladeira Lagerstätte (Portugal).
Despite the good knowledge of the different anchoring strategies of crinoids in soft sediments (Seilacher and MacClintock, Reference Seilacher and Macclintock2005; Donovan, Reference Donovan2006), some of which are potential producers of bioturbation structures, no modern or fossil traces have been ascribed to them.
Traces associated with mitrate body fossils
Associated with body fossils of the Devonian stylophoran Rhenocystis latipedunculata, Rahman et al. (Reference Rahman, Jefferies, Südkamp and Smith2009) erected the ichnotaxon Vadichnites transversus as horizontal straight to curved traces with low relief protuberances or closely spaced fine ridges transversely oriented across a shallow groove. This new ichnotaxon is argued to demonstrate that the appendage of R. latipedunculata was used in locomotion and that this movement took place appendage-first. Rahman et al. (Reference Rahman, Jefferies, Südkamp and Smith2009) interpreted these trace fossils as having been produced just before death probably in response to catastrophic burial by turbidity current deposits. Although Vallon et al. (Reference Vallon, Rindsberg and Bromley2016) do not recommended the use of this ethological category, arguing that it is based on holistic interpretations, rather than on trace fossil morphology, the ichnogenus Vadichnites could be considered as an example of mortichnia.
Finally, in order to obtain a rapid understanding of all these ichnotaxa, a recompilation of ichnogenera diagnoses is shown in Table 2. According to the last ichnotaxonomic studies, only the diagnoses of those broadly accepted ichnogenera have been included.
Table 2 Ichnogenera diagnoses. According to the last ichnotaxonomic discussions, only diagnoses of those broadly accepted ichnogenera have been included.
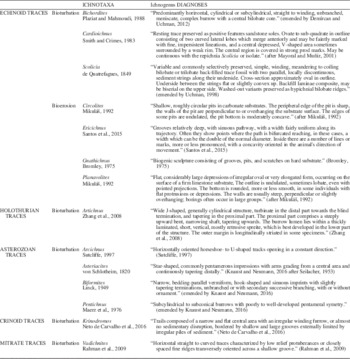
Traces not directly produced by echinoderms but closely related to them
Echinoderms possess multi-element skeletons composed of thousands of diverse ossicles (e.g., Donovan, Reference Donovan1991; Kroh and Nebelsick, Reference Kroh and Nebelsick2010). After death and under ‘normal’ conditions (i.e., excluding rapid burials, dysoxic conditions, etc.), the disarticulation of most parts of echinoderm skeletons, except for many echinoids that are commonly preserved as complete denuded tests (see Belaústegui et al., Reference Belaústegui, Nebelsick, Gibert, Domènech and Martinell2012 and references therein), is very fast (days to weeks) and their separate single ossicles may become very important components within the sediment (Ausich, Reference Ausich1997; Kroh and Nebelsick, Reference Kroh and Nebelsick2010; Dynowski, Reference Dynowski2012). Occasionally, the abundance of these ossicles on the seafloor may be condensed by the activity of burrowing organisms (e.g., the ichnogenus Crininicaminus, see below). In addition, the unique compositional and morphological features of the echinoderm skeletons promote their rapid growth and regeneration (Kroh and Nebelsick, Reference Kroh and Nebelsick2010). These qualities may facilitate the preservation of an ichnological record (e.g., predation, parasitism) on or in their skeletons.
Traces produced with or in echinoderm ossicles and/or spines
Ettensohn (Reference Ettensohn1981) erected the ichnogenus and ichnospecies Crininicaminus haneyensis, to describe cylindrical burrows with a lining mainly composed of crinoids ossicles from the Carboniferous of east-central Kentucky, USA. Subsequently, from the late Permian Kamiyasse Formation (northeastern Japan), Seike et al. (Reference Seike, Shiino and Suzuki2014) erected the new ichnospecies C. giberti differing from C. haneyensis by the arrangement of the crinoid stem plates (horizontal to the long axis of the tunnel in C. haneyensis, and vertical in C. giberti). In both cases, the authors attributed its probable construction to the burrowing activity of tube-dwelling worms.
Neumann et al. (Reference Neumann, Wisshak and Bromley2008) erected the ichnospecies Trypanites mobilis for borings produced in bulbous spines of psychocidarid echinoids from the Late Cretaceous (Cenomanian) to early Paleocene (Danian) strata of the North Sea Basin (Denmark). These authors proposed that these borings were produced post-mortem by sipunculid worms, which would have used these spines as mobile domiciles on soft-bottom habitats.
Bioclaustrations on echinoderm stereom
Embedment structures or bioclaustrations consists of cavities produced by endosymbiontic organisms that live within, by partially inhibiting, the growing skeleton of a host organism (e.g., Tapanila, Reference Tapanila2005; Cónsole-Gonella and Marquillas, Reference Cónsole-Gonella and Marquillas2014). Tapanila (Reference Tapanila2005) included all these traces within the new ethological category Impedichnia; although subsequent authors do not recommend its use (Vallon et al., Reference Vallon, Rindsberg and Bromley2016, and references therein). In any case, bioclaustrations produced in the stereom of different echinoderms, mainly crinoids, have been recorded and several ichnotaxa have been erected to name them.
The ichnogenus Tremichnus was erected by Brett (Reference Brett1985) to include simple circular-parabolic pits, with or without associated stereom swellings, primarily produced by a combination of embedment (i.e., inhibition of stereom growth) and true boring (i.e., removal of stereom), on echinoderms (mainly crinoids; Fig. 6.8) (see also, Brett, Reference Brett1978; Eckert, Reference Eckert1988; Feldman and Brett, Reference Feldman and Brett1998; Wilson et al., Reference Wilson, Reinthal and Ausich2014; Donovan, Reference Donovan2015; Vinn et al., Reference Vinn, Wilson, Ausich and Toom2015). Brett (Reference Brett1985) proposed parasites or commensal filter feeders as possible tracemakers. The stratigraphic record of Tremichnus ranges from the Middle Ordovician to the Middle Jurassic. In addition, an important ichnotaxonomic discussion has been developed around this ichnogenus together with Oichnus Bromley, Reference Bromley1981, Sedilichnus Müller, Reference Müller1977 and Fossichnus Nielsen, Nielsen and Bromley, Reference Nielsen, Nielsen and Bromley2003. Pickerill and Donovan (Reference Pickerill and Donovan1998) regarded Tremichnus as junior synonym of Oichnus. Bromley (Reference Bromley2004) followed this synonymy and in turn considered Sedilichnus as their possible senior synonym; nevertheless Bromley (Reference Bromley2004) kept using Oichnus. Subsequently, Zonneveld and Gingras (Reference Zonneveld and Gingras2014) formalized all these proposals and established Tremichnus, Oichnus and Fossichnus as junior synonyms of Sedilichnus. Finally, Wisshak et al. (Reference Wisshak, Kroh, Bertling, Knaust, Niel sen, Jagt, Neumann and Nielsen2015) noted that Sedilichnus is an atelonym and has no nomenclatural value. Consequently, they retained Oichnus and Tremichnus as valid ichnogenera and pointed out their respective synonyms Fossichnus and Balticapunctum Rozhnov, 1989. Wisshak et al. (Reference Wisshak, Kroh, Bertling, Knaust, Niel sen, Jagt, Neumann and Nielsen2015; see emended and differential diagnoses) differentiated Oichnus from Tremichnus because this latter is restricted to echinoderm host substrates and does not penetrate through the substrate.
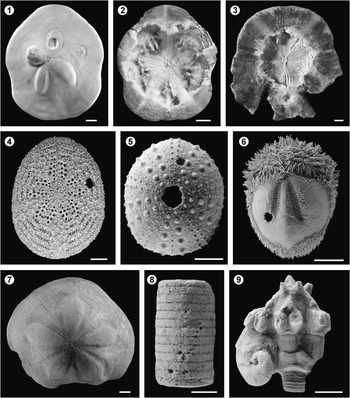
Figure 6 Traces produced on echinoderms. Predation and parasitism on clypeasteroid echinoids from the Northern bay of Safaga (Egypt, Red Sea): (1) Clypeaster humulis showing features related to sublethal predation and parasitism. The symmetry of the petalodium is totally disrupted. The left frontal petalodium is highly inflated due to a gall formation. The frontal petal shows a depression probably representing a healed gall. The right frontal petal is restricted to the distal part only. This may represent an early sublethal wound as the neighboring petals are distended in its direction; (2) Clypeaster humilis showing a massive wound on the oral surface revealing the petalodium from the inner side. Parallel scratches interpreted as tooth marks, attributed to balisted fish, are seen on the left; (3) Echindoscus auritus showing both non-lethal predation on the ambitus as well as a lethal wound at the center of the oral side of the test; (4) Echinocyamus crispus showing a clear bore hole (Oichnus-like) towards the upper right intersecting tubercles and ambulacral pores of the petalodium. Predation on modern echinoids from Santiago de Cuba: (5) Echinometra lucunter and (6) Brissus unicolor showing clear bore holes (Oichnus-like). Miocene of Valencia (E Spain): (7) Clypeaster sp. showing a very irregular ambitus, healed after crab or vertebrate predation. Parasitism on crinoids: (8) stem (Millericrinidae indet.) with simple pits (Tremichnus-like) from the Late Jurassic of the Albarracín Range (Teruel, E Spain); (9) Oenochoacrinus princeps parasitized by platyceratid gastropod from the Valporquero Formation of Colle (León, NW Spain; see Fernández-Martínez et al., Reference Fernández-Martínez, Fernández, Waters and Zamora2015) (Photographs 5 to 9 are courtesy of S. Zamora). Scale bars are (1, 2, 3, 5, 6, 7) 1 cm; (4) 0.5 mm; (8, 9) 0.5 cm.
Two names, Myzostomites and Schizoproboscina, were firstly and respectively proposed by Clarke (Reference Clarke1921) and Yakolev (Reference Yakolev1939) to designate swelling- or cyst-like structures produced by parasites on echinoderms, mainly crinoids and echinoids. Radwańska and Radwański (Reference Radwańska and Radwański2005), studying the myzostomid and copepod infestation of Jurassic echinoderms, revised the taxonomic validity of these names and highlighted that: (1) Myzostomites has been treated both as a body fossil (the worm M. clarkei Howell, Reference Howell1962) and a trace fossil (Häntzschel, Reference Häntzschel1975), (2) Schizoproboscina (with its species S. ivanovi Yakolev, Reference Yakolev1939) was accepted as a worm body fossil by Howell (Reference Howell1962), and (3) the Schizoproboscina material described by Yakolev (Reference Yakolev1939) and revised by Arendt (Reference Arendt1961) shares many similarities with the Myzostomites type material of Clarke (Reference Clarke1921). Radwańska and Radwański (Reference Radwańska and Radwański2005) regarded Schizoproboscina as a junior synonym of Myzostomites, and consequently, M. ivanovi (Yakovlev, 1939) as the valid name for the type material of Clarke (Reference Clarke1921). Additionally, Brett (Reference Brett1985) proposed to replace M. clarkei with Tremichnus cysticus Brett, Reference Brett1985; however, Radwańska and Radwański (Reference Radwańska and Radwański2005) considered this modification invalid according to the ICZN rules. Following Radwańska and Radwański (Reference Radwańska and Radwański2005), the ichnogenus Myzostomites (Yakolev, Reference Yakolev1939) should comprise paired round borings connected by an internal U-shaped canal produced on echinoderms (mainly crinoids), which in most cases promotes an overgrowth (swelling-like or cyst) of their stereom.
Castexia Mercier, Reference Mercier1936 includes spherical endocysts, convexly elevated upon the echinoid test, with a pentagonal or subdecagonal outline, and five to 16 teardrop-shaped, peripherally dispersed orifices (occasionally, a subcentral orifice may occur). The wall of these endocysts is constructed by the stereom overgrowth of the host echinoderm (exclusively echinoids) that reacts to the activity of the producer, thus embedding it. Copepods (Crustacea) have been proposed as probable tracemaker from the Ordovician onward (see extensive review in Klompmaker and Boxshall, Reference Klompmaker and Boxshall2015). These endocysts are especially prevalent in the Middle to Late Jurassic (Mercier, 1939; Radwańska and Radwański, Reference Radwańska and Radwański2005 and references therein). In addition, Radwańska and Radwański (Reference Radwańska and Radwański2005) regarded the ichnotaxon Canceripustula nocens Solovyev, Reference Solovyev1961 as a junior synonim of Castexia douvillei Mercier, 1939.
Radwańska and Radwański (Reference Radwańska and Radwański2005) also described ‘Halloween pumpkin-mask’ cysts consisting of bulbous exocysts with numerous circular orifices, recorded on Late Jurassic echinoid tests and more rarely on Early Jurassic crinoid stems. As in the case of Castexia, these exocysts have been interpreted as the result of stereom overgrowth promote by copepod parasitism. Similar fossil exocysts or swelling structures have described by Franzen (Reference Franzen1974), Smith (Reference Smith1988), Mehl et al. (Reference Mehl, Mehl and Häckel1991), Radwańska and Poirot (Reference Radwańska and Poirot2010) and Klompmaker and Boxshall (Reference Klompmaker and Boxshall2015). It is also known that the modern copepod Pionodesmotes Bonnier, Reference Bonnier1898 inhabits the internal test surface of live echinoids within galls, which are connected to the exterior by an irregular opening bored through the stereom (Richard, Reference Richard1907; Jangoux, Reference Jangoux1987). They have, however, not yet been recognized in the fossil record.
Finally, the ichnogenus Ostiocavichnus was erected by Bohatý et al. (Reference Bohatý, Ausich, Nardin, Nyhuis and Schröder2012) from Devonian crinoids of Germany and Poland. It comprises gall-like swellings produced on crinoid pluricolumnals by epizoozoan rugose corals. These traces consist of elliptical or subcircular concavities resulting from the encasing of the coral by stereomic coating (Bohatý et al., Reference Bohatý, Ausich, Nardin, Nyhuis and Schröder2012).
Swelling structures comparable to the ichnogenera cited in this subsection but not ascribed to any of them, and produced by diverse parasites (e.g., myzostomid worms, copepods) on different kinds of echinoderms (mainly crinoids and echinoids, Fig. 6.1, and their spines), have also been described (e.g., Franzen, Reference Franzen1974; Welch, Reference Welch1976; Werle et al., Reference Werle, Frest and Mapes1984; Abdelhamid, Reference Abdelhamid1999; Radwańska and Radwański, Reference Radwańska and Radwański2005; Hess, Reference Hess2010; Thomka et al., Reference Thomka, Malgieri and Brett2014; Wilson et al., Reference Wilson, Reinthal and Ausich2014 and references therein).
Traces produced by symbiosis (mainly parasitism) on echinoderms
The existence of diverse symbiotic relations between echinoderms and various kinds of organisms have been recorded both in the fossil record and in the Recent (e.g., Tapanila, Reference Tapanila2008; Boucot and Poinar, Reference Boucot and Poinar2010); subsequently, some of these relations may generate an ichnological record.
The parasitism of platyceratid gastropods on crinoids (Fig. 6.9), more rarely on blastoids, is well known and documented in the fossil record (e.g., Baumiller, Reference Baumiller1990, Reference Baumiller2002, Reference Baumiller2003; Baumiller and Gahn, Reference Baumiller and Gahn2002, Reference Baumiller and Gahn2003; Gahn and Baumiller, Reference Gahn and Baumiller2003; Baumiller et al., Reference Baumiller, Gahn and Savill2004; Donovan, Reference Donovan2015). Baumiller (Reference Baumiller1990) described the presence of drill-holes (Oichnus-like) on Mississippian crinoids, which are interpreted as the non-predatory drilling activity of platyceratid gastropods. This interpretation is based on the occurrence of a platyceratid shell located just above a conical hole present on the tegmen of one of these Mississippian crinoids. Similar drill holes, also attributed to parasitic platyceratids, have also been documented on Devonian blastoids and crinoids (Baumiller, Reference Baumiller1996 and Gahn et al., Reference Gahn, Fabian and Baumiller2003, respectively).
Circular to subcircular traces, produced on the surface of an early Maastrichtian holasteroid echinoid test (northern Germany), and consisting of a more or less pronounced rim surrounding a central depression, were interpreted by Neumann and Wisshak (Reference Neumann and Wisshak2006) as the attachment scars produced by probable parasitic foraminifera during a syn-vivo infestation; since the characteristic rim of these traces would be the result of the host-skeletal overgrowth around the attached parasite as defense mechanism.
Wisshak and Neumann (Reference Wisshak and Neumann2006) described 27 U-shaped borings (Caulostrepsis isp.) produced on the test of a Late Cretaceous holasteroid echinoid of Germany. Since stereom regeneration is observed in the walls of these borings, these authors interpreted the trace as the result of a symbiotic association (syn-vivo infestation) between boring polychaetes (probably spionids) and the echinoid.
Donovan et al. (Reference Donovan, Jagt and Dols2010) interpreted a non-penetrative shallow scar (rounded to pentagonal in outline) present on the test of a Late Cretaceous holasteroid echinoid from the Maastrichtian type area (the Netherlands, Belgium), as the possible basal attachment mark left by a sessile, unmineralized invertebrate such as a sea anemone.
Arendt (Reference Arendt2012) erected the ichnogenus Augoichnus to include shallow depressions, with a commonly ovoid outline, which margin is rimmed by one or two grooves, produced on the cup, brachial spines and anal sacs of early Permian crinoids (Krasnoufimsk, Russia), and also attributed its origin to the attachment of parasitic gastropods (not platyceratids).
Saint-Seine (Reference Saint-Seine1950, Reference Saint-Seine1959) also documented several types of damage on echinoid tests (Micraster and Clypeaster) from different ages and localities, which could be attributed to the activity of parasites (maybe predators). This damage consists mainly of shallow, irregular or meandering scars or depressions produced in the outer surfaces of the tests; which in the latter case are always regenerated.
Finally, Jangoux (Reference Jangoux1990) enumerated a series of diseases that may affect echinoderms, including those produced by both micro- and macro-organisms, which may be potential producers of an ichnological record.
Traces produced by invertebrate predation on echinoderms
It is well known that some echinoderms are active predators on a great variety of invertebrates (e.g., asterozoans; Carter, Reference Carter1968), including other echinoderms (e.g., Quinn, Reference Quinn1965; Aronson, Reference Aronson1987; Baumiller et al., Reference Baumiller, Mooi and Messing2008). Echinoderms, however, are also commonly preyed upon by crustaceans, polychaetes and gastropods (e.g., Meyer and Ausich, Reference Meyer and Ausich1983; Smith, Reference Smith1984; Aronson, Reference Aronson1987; Baumiller and Gahn, Reference Baumiller and Gahn2003; Kowalewski and Nebelsick, Reference Kowalewski and Nebelsick2003). Therefore, both the attacks with a fatal outcome as well as non-lethal predation can potentially produce ichnological evidence in the echinoderm skeletons.
Drilling predation (Oichnus-like structures) on echinoids is widely documented (Fig. 6.4–6.6); these borings are mainly attributed to the activity of cassid gastropods, although other invertebrates have been also proposed (e.g., Beu et al., Reference Beu, Henderson and Nelson1972; Gibson and Watson, Reference Gibson and Watson1989; Nebelsick, Reference Nebelsick1998; Nebelsick and Kowalewski, Reference Nebelsick and Kowalewski1999; Ceranka and Złotnik, Reference Ceranka and Złotnik2003; Kowalewski and Nebelsick, Reference Kowalewski and Nebelsick2003; Donovan and Pickerill, Reference Donovan and Pickerill2004; Grun et al., Reference Grun, Sievers and Nebelsick2014; Meadows et al., Reference Meadows, Fordyce and Baumiller2015; Grun and Nebelsick, Reference Grun and Nebelsick2016). In fact, the ichnospecies Oichnus halo was erected by Neumann and Wisshak (Reference Neumann and Wisshak2009) to include central holes with parallel smooth walls surrounded by one or more circular depressions. At the moment, this ichnospecies has only been described on the tests of Late Cretaceous to early Paleocene holasteroid echinoids from the northern Germany and Sweden. Based on similar traces produced by modern eulimid gastropods (genus Thyca) that parasitize on asteroids, Neumann and Wisshak (Reference Neumann and Wisshak2009) proposed the structures generated by this group of gastropods as the modern counterpart of these trace fossils; which are interpreted to result from a combination of attachment processes and predatory feeding behavior.
Donovan and Jagt (2002, Reference Donovan and Jagt2003, Reference Donovan and Jagt2004, Reference Donovan and Jagt2013) and Donovan et al. (Reference Donovan, Jagt and Lewis2008) described non-penetrative Oichnus-like structures in Cretaceous echinoids that could be interpreted as the result of parasites or even failed predatory attacks (see Donovan, Reference Donovan2015 for further references). In addition, Deline (Reference Deline2008) documented the presence of a borehole in the Late Ordovician echinoderm Enoploura (Stylophora). The preservation features of these borings may indicate a predatory origin, but parasitism is not ruled out.
Bite marks, consisting of shallow and rectilinear scratches and oval to circular pits, left by the jaw apparatus of cidaroid sea urchins on crinoid skeletons, while they feed upon, were documented by Baumiller et al. (Reference Baumiller, Salamon, Gorzelak, Mooi, Messing and Gahn2010) both in fossil and recent specimens.
Traces produced by vertebrate predation on echinoderms
Today, fish are probably the most important vertebrate predators of echinoderms (e.g., Meyer and Ausich, Reference Meyer and Ausich1983; Smith, Reference Smith1984; Baumiller and Gahn, Reference Baumiller and Gahn2003; Grun, Reference Grun2016), but there are others such as turtles, birds, sea otters or arctic foxes (e.g., Nebelsick, Reference Nebelsick1998; Nebelsick and Kowalewski, Reference Nebelsick and Kowalewski1999; Kowalewski and Nebelsick, Reference Kowalewski and Nebelsick2003; Sievers et al., Reference Sievers, Friedrich and Nebelsick2014; and references therein).
The record of fish predation on modern and fossil crinoids and ophiuroids, is identifiable by the occurrence of regenerating arms, which in turn evidences the usual nonlethal outcome of these attacks (e.g., Meyer and Ausich, Reference Meyer and Ausich1983; Aronson, Reference Aronson1987; Baumiller and Gahn, Reference Baumiller and Gahn2003, Reference Baumiller and Gahn2004; and references therein). Lethal fish attacks on clypeasteroids (see Frazer et al., Reference Frazer, Lindberg and Stanton1991) leave a gaping central cavity surrounded by, in part, parallel bite marks (Nebelsick and Kampfer, Reference Nebelsick and Kampfer1994; Nebelsick, Reference Nebelsick1998) (Fig. 6.2, 6.3). Clypeasteroid echinoids often show evidence of bite marks along the ambitus (e.g., Vadász, Reference Vadász1914; Zinsmeister, Reference Zinsmeister1980; Nebelsick and Kampfer, Reference Nebelsick and Kampfer1994; Lawrence and Vasquez, Reference Lawrence and Vasquez1996; Nebelsick, Reference Nebelsick1998; Santos et al., Reference Santos, Mayoral, Muñiz, Bajo and Adriaensens2003) (Fig. 6.3). These wounds can be healed since they do not reach into the central lumen of the test containing the vital organs and result in highly irregular outlines typical of sand dollars (Fig. 6.1–6.3, 6.7).
Fish tooth-marks (e.g., sharks, rays or durophagous fishes), mainly consisting of longish grooves or isolated to rowed pits or punctures, have been recognized on asterozoan ossicles (Neumann, Reference Neumann2000), rhabdocidaroid echinoid spines (Linichnus-, Machichnus- and Nihilichnus-like traces, Wilson et al., Reference Wilson, Borszcz and Zatoń2015; see also Donovan and Renema, Reference Donovan and Renema2016), and echinoid tests (Schormann, Reference Schorrnann1987). Fragmented echinoid remains can also occur as regurgitates from fish predation (Borszcz and Zatoń, Reference Borszcz and Zatoń2013 and references therein).
Echinoderms as benthic islands for boring and encrusting organisms
In environments with soft muddy or sandy seafloors, the availability of hard substrates (even small, isolated and relatively stable) may be crucial for the survival of a plethora of boring and encrusting invertebrate organisms, such as barnacles, bivalves, polychaetes, sponges, or bryozoans. Encrustation during life in echinoids is restricted to the spines of cidaroids which lack the epidermis that covers most echinoids during life (David et al., Reference David, Stock, de Carlo and de Ridder2009) or the rare encrustation of sand dollars by balanid barnacles (Merril and Hobson, Reference Merril and Hobson1970). Frequently, both in the fossil record and in the Recent, isolated dead echinoid tests commonly constitute benthic islands or preferential spots for the settlement and growth of these hard-substrate dwellers (Fig. 7; e.g., Nebelsick et al., Reference Nebelsick, Schmid and Stachowitsch1997; Borszcz, Reference Borszcz2012; Belaústegui et al., Reference Belaústegui, Gibert, Nebelsick, Domènech and Martinell2013; Borszcz et al., Reference Borszcz, Kuklinski and Zatoń2013; Rahman et al., Reference Rahman, Belaústegui, Zamora, Nebelsick, Domènech and Martinell2015; and references therein). Additionally, modern and fossil crinoids can also be considered as benthic islands for many epidionts; in these cases the colonization may occur on both living and dead specimens (e.g., Brett and Eckert, Reference Brett and Eckert1982; Liddell and Brett, Reference Liddell and Brett1982; Peters and Bork, Reference Peters and Bork1998; see also previous section concerning bioclaustrations). In addition, cystoid thecae used as benthic islands have been also documented in the fossil record (Thomka et al., Reference Thomka, Brett, Young, Bantel and Bissett2016).

Figure 7 Echinoderms as benthic islands: (1) Post-mortal encrustation of the early Miocene Clypeaster latirostris from the Molasse Zone (Austria) by balanid barnacles; (2) Post-mortal encrustation of the Recent spantagoid Ova canalifera from the Northern Adriatic Sea by serpulid worm tubes (see Nebelsick, Reference Nebelsick2004); (3) Post-mortal encrustation of the Recent sea urchin Echinus sp. from Ibiza (Balearic Islands, Spain) mainly by bryozoans and serpulid worms. Scale bars are (1, 2) 1 cm; (3) 5 cm.
From Miocene Clypeaster tests deposited in a soft and non-consolidated biocalcarenite of northeastern Spain (i.e., benthic islands), Belaústegui et al. (Reference Belaústegui, Gibert, Nebelsick, Domènech and Martinell2013) described two modes of occurrence for traces (cf. Gastrochaenolites dijugus) produced by gastrochaenid bivalves: (1) ‘intrastereom clavate borings’ restricted to the echinoid stereom; and (2) ‘semi-endoskeletal dwellings’, which penetrate across the test wall and extend as carbonate crypts into the sediment fill of the internal test cavity.
Jagt et al. (Reference Jagt, Fraaije and van Bakel2009) erected the new ichnospecies Petroxestes altera to describe shallow, elongate borings with narrowly rounded ends and irregular bioglyphs (grooves and scratches) produced in a hemipneustid echinoid (late Maastrichtian, Belgium). Jagt et al. (Reference Jagt, Fraaije and van Bakel2009) interpreted that this trace was produced by a sipunculid worm, after the death of the echinoid.
Conclusions
Modern and fossil bioturbation and bioerosion structures produced and interpreted as produced by echinoderms are reviewed. In the trace fossil record, 22 ichnogenera and 53 ichnospecies have been erected; among them, and following latest ichnotaxonomic discussions, 14 ichnogenera and 26 ichnospecies are considered as valid ichnotaxa. All of them correspond to bioturbation structures except four ichnogenera interpreted as bioerosion structures.
Echinoid and asterozoan traces exhibit the highest ichnodiversities both in the fossil record and today. By contrast, the ichnological record of other groups, such as crinoids or mitrates, is reduced to unique and exceptional specimens. In addition, although nowadays the different burrowing behaviors conducted by holothurians are really well known, their trace fossil record is limited at this time to a single ichnogenus and two ichnospecies.
This contribution highlights the importance of the combination of neo- and paleoichnological studies as a very powerful tool to interpret and to better understand the ecology and ethology of burrowing and boring invertebrates (echinoderms, in this particular case), and the trace fossil record.
Acknowledgments
The authors acknowledge the photographic material provided by S. Zamora (Instituto Geológico y Minero de España, Zaragoza, Spain) and M. Ballesteros (Universitat de Barcelona, Spain) and the comments of D. Knaust (Statoil, Norway). Many thanks to W. Gerber for excellent photographs and T. Grun (both Department of Geosciences, Tübingen) for help in sample preparation. The authors appreciate the comments provided by the editor B. Hunda (Cincinnati Museum Center, USA) and the reviews of A. Uchman (Jagiellonian University, Poland) and C. Brett (University of Cincinnati, USA); their suggestions have contributed to improve the original manuscript.
This study is part of the activities of the Research Group RNM 293 “Geomorfología Ambiental y Recursos Hídricos”, University of Huelva (FM). Z.B. is supported by a BDR post-doctoral fellowship of the University of Barcelona.