Alcoholism has become a serious health problem all over the world. The incidence of alcoholic liver disease (ALD) has been increasing rapidly in China(Reference Fan, Zhu and Li1). ALD is also the major cause of liver cirrhosis in the Western world. Treatment of ethanol-related illness costs more than 1·5 billion dollars/year and the illness causes loss of productivity in the USA alone(Reference Shaheen, Hansen and Morgan2). The most widely diagnosed forms of ALD are alcoholic fatty liver, acute alcoholic hepatitis and alcoholic cirrhosis(Reference Walsh and Alexander3).
Many pathways are considered to be involved in ALD, such as oxidative stress, mitochondrial damage and inflammatory factors(Reference Tilg and Diehl4, Reference Albano5). Oxidative stress that plays a critical role in the process of liver damage is the result of an imbalance between pro-oxidants and antioxidants. The evidence of alcohol-induced oxidative stress is extensive(Reference Arteel6, Reference Yuan, Gong and Li7). As alcohol takes a high percentage of the total energy intake in alcoholics, the balance of carbohydrate, lipids and proteins is altered. The metabolic disturbance of nutrients may also be involved in ALD(Reference Bunout8). Furthermore, the gradually increasing activity of hepatic alcohol dehydrogenase (ADH) was observed to be due to the long-term intake of alcohol(Reference Wang, Wang and Liu9).
Although many efforts have been ploughed into the treatment of ALD, there is no universally satisfactory therapy to prevent or reverse the ALD process in humans(Reference Bhandarkar and Khan10). Some endogenous bioactive peptides, such as ghrelin(Reference Golestan Jahromi, Nabavizadeh and Vahedian11), bradykinin(Reference Sancho-Bru, Bataller and Fernandez-Varo12), vasoactive intestinal peptide(Reference Allam13, Reference Luo, Wang and Feng14) and brain natriuretic peptide(Reference Sonoyama, Tamura and Miyashita15), have been reported to have protective effects against liver injury induced by concanavalin A, carbon tetrachloride or acetaminophen. Although incomprehensive and non-systemic, several exogenous bioactive peptides including Ganoderma lucidum peptides(Reference Shi, Sun and He16), Shahepatic(Reference Lu, Li and Ye17) and corn peptides(Reference Guo, Sun and He18) have been reported to alleviate liver injury. Hepatoprotective agents from both plants and animals are attracting more attention today. Marine collagen peptides (MCP) are compounds of low-molecular-weight peptides derived from the skin of chum salmon (Oncorhynchus keta) by enzymatic hydrolysis. The various multifunctional properties of MCP have been described already, including anti-hypertension(Reference Zhang, Wang and Xu19), extending the life span(Reference Liang, Pei and Wang20), anti-skin ageing(Reference Tanaka, Koyama and Nomura21) and anti-ulcer(Reference Zolotarev Iu, Badmaeva and Bakaeva22). Previous research has indicated that MCP have a strong antioxidative activity(Reference Pei, Yang and Zhang23) and could improve lipid metabolism(Reference Saito, Kiyose and Higuchi24). However, the effect of MCP on alcoholic liver injury is not yet known.
In the present study, we investigated the effects of MCP on early alcoholic liver injury in rats. It has been proved that females are more susceptible to alcohol-induced liver injury than males(Reference Saunders, Davis and Williams25, Reference Jarvelainen, Lukkari and Heinaro26). So, we chose female rats in the present study. Liver injury was evaluated by biochemical parameters and histopathological changes. In addition, we detected malondialdehyde (MDA), superoxide dismutase (SOD), lipid, protein and ADH levels in serum or liver to find the possible mechanisms of the effects.
Materials and methods
Materials and reagents
Commercial kits used for the determination of total SOD and MDA in serum ADH and protein in liver tissue were purchased from Jiancheng Institute of Biotechnology (Nanjing, China). Ethanol, acetaldehyde and other chemicals used were of analytical grade and purchased from Beijing Chemical Company (Beijing, China).
Preparation and identification of marine collagen peptides
MCP, which were derived from the skin of wild-caught chum salmon (O. keta; average body weight 1·47 kg), were donated by CF Haishi Biotechnology Limited Company (Beijing, China). In brief, fish skin was cleaned and the adherent tissue was scraped manually by a scalpel. Then, the skin was minced and defatted by vigorous stirring in chill water. The materials were emulsified in distilled water and added into complex protease (3000 U/g protein), including 7 % trypsin, 65 % papain and 28 % alkaline proteinase. The hydrolysate was then centrifuged to remove impurity. After that, the liquid was separated through a ceramic membrane (200 μm) for its purification. The mineral salt was removed from the liquid through a procedure of nanofiltration. Then, the purified liquid was condensed by cryoconcentration under vacuum at 70°C, with an evaporation rate of 500 kg/h. When the concentration of the condensed liquid was almost 30 Baume degrees, it was decoloured with 12 % of active carbon at 75°C for 1 h, and then the carbon was removed by filtration. Most of the water was removed by spray drying with a pressure of 20 MPa, and MCP powder was thus obtained.
The MCP sample contains about 90 % hydrolysed protein, 6·0 % ash, 1·4 % carbohydrate, 2·5 % water and 0·1 % fat. HPLC (Waters Corporation, Milford, MA, USA) of the peptide sample was performed using a Phenomenex C18 column (10 × 250 mm) and a mixture of acetonitrile and 0·05 mol/l phosphate buffer (pH 3·2; 10:90) as the mobile phase with a flow rate of 2·0 ml/min, monitored at 208 nm using a Waters 486 tunable UV detector. Then, the molecular weight distribution of the sample was ascertained by LDI-1700 matrix-assisted laser desorption ionisation time-of-flight MS (Liner Scientific, Inc., Reno, NV, USA). In addition, the amino acid composition was further analysed by an H835-50 automatic amino acid analyser (Hitachi, Tokyo, Japan). The result indicated that the molecular weight distribution of MCP was 100–860 Da. Furthermore, 85·86 % of their molecular weight distributed between 300 and 860 Da. So, the main composition of MCP was oligopeptides. The amino acid composition of MCP is shown in Table 1.
Table 1 Amino acid composition of marine collagen peptides
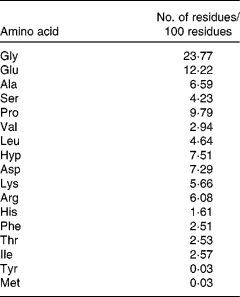
Animals and treatment
A total of fifty-two female Sprague–Dawley rats (10 weeks old, weighing 230–250 g) were obtained from the Animal Service of Health Science Center, Peking University. The conditions were as follows: a filter-protected, air-conditioned room with controlled temperature (25 ± 28°C), relative air humidity (60 ± 5 %) and a 12 h light–12 h dark cycle (lights on at 07.30–19.30 hours). Animal treatment and maintenance were carried out strictly in accordance with the Principle of Laboratory Animal Care (NIH publication no. 85-23, revised 1985) and the guidelines of the Peking University Animal Research Committee (www.lab.pku.edu.cn).
Experimental design
All rats were fed with a modelled AIN-93M rodent diet (Vital River Limited Company, Beijing, China), with casein as the main protein source. After the acclimatisation period of 1 week, animals were randomly divided into five groups: normal control group (n 10); alcohol control group (n 12); three groups (n 10, each) for low (0·225 g/kg per d), medium (0·45 g/kg per d) and high (0·9 g/kg per d) doses of MCP. Body weight and food consumption were measured every week. The rats were orally administered with alcohol (50%, v/v) at a dose of 6 g/kg per d, except the rats in the normal group that received equivalent distilled water. After 1 h, the rats of the three intervention groups were intragastrically administered with additional MCP while the others received an equal volume of vehicle as control. The study lasted for a total of 4 weeks. After 12 h of the last alcohol treatment, blood was obtained and serum was separated by centrifugation at 3000 rpm for 10 min. Portions of the liver were then obtained for histopathological and biochemical assays.
Biochemical assays
The levels of alanine aminotransferase (ALT), aspartate aminotransferase (AST), total proteins, albumin, albumin:globulin ratio, total cholesterol (TC) and TAG in serum were detected by an automatic biochemistry analyser (Hitachi, Tokyo, Japan), and SOD activity and MDA level in serum, and the contents of ADH and protein in the liver homogenate were determined by the detection kits according to the manufacturer's protocols.
Histopathological observation
Portions of the tissues were obtained from the same lobe of the liver in each rat and were immediately fixed in 10 % (v/v) formaldehyde, embedded in paraffin and cut into 5 μm-thick sections, which were then stained with haematoxylin and eosin and observed under a light microscope (Nikon Y-FL light microscope; Nikon Corporation, Tokyo, Japan).
Statistical analysis
Results are expressed as mean values and standard deviations. Data were tested for homogeneity at first and then were evaluated by means of a one-way ANOVA test followed by a post hoc least significant difference test if variances were equal or Tamhane's T2 test if variances were not equal. The statistics compared with the alcohol-treated control group were presented. A P value < 0·05 was considered significant.
Results
General state
There were forty-eight rats that survived over 4 weeks and gained weight during this period. There were four rats that did not survive over 4 weeks: one in the alcohol control group; two in the low-dose MCP group and one in the high-dose MCP group. After intragastrically administered with alcohol at a dose of 6 g/kg, most of the rats behaved excitedly such as unsteady walking and accelerated breathing. At the first week, the weights of the rats administered with alcohol were reduced significantly (P < 0·05). However, there was no significant difference in the following 3 weeks, indicating that all animals received adequate nutrition after adapting to alcohol. MCP could not restore the change in body weight significantly compared with alcohol-treated rats (Table 2).
Table 2 Effects of marine collagen peptides (MCP) on the change in body weight
(Mean values and standard deviations, n 8–11/group)

* Mean values was significantly different from those of the normal control group (P < 0·05).
Serum alanine aminotransferase and aspartate aminotransferase levels
Serum ALT and AST activities were used to evaluate early alcoholic liver injury. The AST level was increased nearly by 2-fold while the ALT level increased by 25 % only, respectively, over the values of normal rats after 4 weeks with alcohol intake by intragastric administration (AST: 47·009 (sd 15·58) v. 26·49 (sd 15·04); ALT: 51·52 (sd 7·83) v. 42·79 (sd 5·12)). The MCP treatment showed the protection against alcohol-induced liver injury in rats by the decrease in the elevation of ALT and AST levels, especially in the high-dose group (Table 3). There was no significant increase in the ratio of AST:ALT with alcohol intake (P = 0·271, alcohol control group v. normal control group). However, after the MCP treatment, there was a decrease in the ratio to nearly normal (P = 0·269, low-dose MCP group v. alcohol control group).
Table 3 Effects of marine collagen peptides (MCP) on serum alanine aminotransferase (ALT) and aspartate aminotransferase (AST) levels in rats
(Mean values and standard deviations, n 8–11/group)

Mean values were significantly different from those of the alcohol control group: *P < 0·05.
† Mean values were significantly different from those of the normal control group (P < 0·05).
Serum malondialdehyde and superoxide dismutase levels
Serum total SOD level was decreased after the alcohol treatment (81·57 (sd 31·46) v. 101·66 (sd 36·18) U/ml), but the intervention of MCP exhibited protection against alcohol-induced SOD depletion (low-dose group: P < 0·05; Fig. 1). Although the medium- and high-dose groups also had a reversion of SOD depletion, the difference was not significant. Serum MDA concentration was increased in the alcohol control group (21·66 (sd 8·93) nmol/ml), compared with the normal control group (6·11 (sd 6·38) nmol/ml), but the MDA level was lowered by the MCP intervention significantly (P < 0·01 in the low- and medium-dose groups, compared with the alcohol control group).

Fig. 1 Effects of marine collagen peptides (MCP) on serum superoxide dismutase (SOD) and malondialdehyde (MDA) levels in rats. Mean values were significantly different from those of the alcohol control group: *P < 0·05, **P < 0·01, ***P < 0·001. Mean values were significantly different from those of the normal control group †P < 0·05, ††P < 0·01.
Lipid and protein levels in serum
Serum TAG and TC levels were significantly increased in the alcohol control group (1·18 (sd 0·29) and 2·64 (sd 0·24) mmol/l), compared with the normal control group (0·46 (sd 0·14) and 1·87 (sd 0·48) mmol/l; Table 4). Meanwhile, MCP reversed the increases in serum TAG and TC levels (P < 0·05 and < 0·001, respectively). Serum total proteins and albumin levels were not significantly different in all groups. The results showed that the albumin:globulin ratio of the alcohol control group was higher than that of the normal control group (P < 0·05), and this ratio was even higher after the MCP treatment (not significant compared with the alcohol control group).
Table 4 Effects of marine collagen peptides (MCP) on lipid and protein levels in the serum of rats
(Mean values and standard deviations, n 8–11/group)

TC, total cholesterol; TP, total protein; ALB, albumin; A:G, albumin:globulin.
Mean values were significantly different from those of the alcohol control group: *P < 0·05, **P < 0·01, ***P < 0·001.
Mean values were significantly different from those of the normal control group: †P < 0·05, †††P < 0·001.
Activity of alcohol dehydrogenase in liver tissue
Although there was no significant change in ADH levels between each group, we found a raising trend of ADH levels in alcohol-treated rats compared with normal control rats (P = 0·127; Fig. 2). Also, the MCP treatment reversed the alcohol-induced change in ADH levels.

Fig. 2 Effect of marine collagen peptides (MCP) on alcohol dehydrogenase (ADH) levels of liver tissue in rats. Animals were killed 12 h after the last administration with or without alcohol. Values are means and standard deviations represented by vertical bars (n 8–11). The values were 12·86 (sd 5·27), 17·50 (sd 8·76), 14·08 (sd 5·04), 14·07 (sd 6·06), 13·00 (sd 4·85) U/mg protein, respectively.
Histopathological result
Histological features of the normal control and alcohol control groups are shown in Fig. 3(a) and (b). There were no pathological changes in the liver of normal control rats. In the rats of the alcohol control group, there were pathological changes in liver tissue characterised by swelling and irregular distribution of the cells. Also, part of the hepatocytes presented fatty degeneration. However, severe pathological changes such as inflammation and apoptosis reported in previous studies were not observed here. The MCP treatment reduced the histopathological changes characterised by more regular hepatic cords and sharp border of the hepatocytes (Fig. 3(c)–(e)).

Fig. 3 Representative histopathological changes of the liver in different groups. Histopathological examination of the liver from normal control rats and rats that received alcohol with or without marine collagen peptides (MCP) for 4 weeks (400 × ). (a) Normal control group; (b) alcohol control group; (c) low-dose MCP group; (d) medium-dose MCP group; (e) high-dose MCP group.
Discussion
MCP from fish skin have been used in food processing. The present study was designed to examine the effect of MCP on early alcoholic liver injury. Different methods of building an alcoholic liver injury model have been developed to study the hepatic protectant. In a study by Wang et al. (Reference Wang, Feng and Zhuang27), rats were administered orally with alcohol at a dose of 6 g/kg body weight for 8 d to induce liver injury. In another study by Kono et al. (Reference Kono, Arteel and Rusyn28), high-fat liquid diets were used with ethanol at a dose of 10 g/kg continuously for 4 weeks to build a liver injury model. In the present study, female rats were administered with alcohol at a dose of 6 g/kg for 4 weeks to cause early liver injury. The results showed a reduced body weight following the alcohol treatment in the first week, which was not restored by MCP; however, until after 4 weeks, no effect was observed on body weight in either treatment. Besides, the increase in blood biochemical parameters (Table 3) and pathological change in liver injury were observed in the alcohol control group (Fig. 3). The serum marker enzymes (AST and ALT) are cytoplasmic in nature, but upon liver injury, these enzymes enter into the circulatory system due to altered permeability of the membrane(Reference Li, Chen and Yu29). Steatosis is usually characterised by a large number of hepatocytes that are occupied by TAG. The MCP exhibited the protective effect on alcohol-induced liver injury by the reversion of these above markers.
Many hypotheses regarding the mechanisms of alcohol-induced hepatocyte injury have been advanced. The most approbatory hypothesis is oxidative stress, which is proposed to be involved in numerous diseases including ALD. Oxidative stress associated with alcohol toxicity is mainly caused by reactive oxygen species, hydroxyethyl radicals and NO in the process of alcohol metabolism. Consequently, breakdown of the cell membrane, hepatic inflammation and hepatocytic apoptosis representing hepatocyte injury by alcohol have been observed(Reference Albano5). The present results showed that alcohol-treated rats possessed a higher MDA level and lower SOD activity in serum than normal control rats, which confirmed the involvement of oxidative stress in alcohol-induced liver injury. MDA is the most abundant by-product of lipid peroxidation, and thus could be considered as an indicator of oxidative stress. Di Luzio & Hartman(Reference Di Luzio and Hartman30) characterised lipid peroxidation after chronic exposure to alcohol for the first time, which was confirmed by other investigators(Reference Shi, Sun and He16, Reference Eaton, Record and Bartlett31). SOD is an enzyme that catalytically reduces O2− to H2O2. Many studies have suggested that alcohol intake lead to the reduction of SOD activity(Reference Guo, Sun and He18, Reference Wang, Feng and Zhuang27, Reference McKim, Konno and Gabele32). Based on the above results including the reversed MDA and SOD levels (Fig. 1), it was confirmed that the state of oxidative stress could be restored by the MCP treatment, especially at a low inclusion of 0·225 g/kg per d. It can be concluded that there is some capacity to restore oxidative damage caused by alcohol. A similar antioxidative influence of MCP was observed in previous studies in our laboratory while the capacity was observed in the medium dose of diets supplemented with MCP at concentrations of 4·5 %(Reference Liang, Pei and Wang20).
Abnormal lipid metabolism can be seen in many patients with ALD(Reference Lieber33). The most common disturbance in lipid metabolism induced by alcohol abuse is excessive accumulation of lipids in the liver (Fig. 3(b)). Alcohol also stimulates lipid peroxidation of the hepatocellular membrane, as discussed previously. Furthermore, serum TAG and TC levels began to change with alcohol administration. Alcohol intake inhibits lipolysis and increases serum TAG levels, with the mechanism still being not clear. It has also been reported that TC and HDL-C had positive correlations with alcohol, but LDL-C showed a less strong negative correlation in human subjects(Reference Castelli, Doyle and Gordon34–Reference Wannamethee and Shaper36). Karsenty et al. (Reference Karsenty, Baraona and Savolainen37) found that TC increased in baboons pair-fed on cholesterol-free diets containing 50 % of energy either as ethanol or as additional carbohydrate for several years. In the present study, we observed that serum TAG and TC levels rose up after alcohol intake while the MCP treatment reversed the alteration. However, MCP could not make the contents revert to a normal level. A latest study showed that plasma TAG in rats were significantly decreased 2 h later after the intake of soyabean oil and fish collagen peptide mixtures compared with oil intake only. The group fed an AIN-93G purified diet containing 0·17 % fish collagen peptide for 14 d had lower levels of plasma total lipids and TAG compared with the control group(Reference Saito, Kiyose and Higuchi24). The present study confirmed that lipid metabolism was restored by MCP to alleviate liver injury.
We also found some other hypotheses that might be involved in the effect of MCP on alcohol-treated rats, though not proved this time. The ratio of AST:ALT in the serum of patients with alcoholic hepatitis is generally higher than that of normal subjects and patients with acute liver injury, as described by Nyblom et al. (Reference Nyblom, Berggren and Balldin38) and Das & Vasudevan(Reference Das and Vasudevan39). In the present study, alcohol induced an increasing AST:ALT ratio compared with normal rats (P = 0·271). MCP inhibited the increase in ratio, though the difference is not significant (Table 3). In the patients of alcoholic liver injury, inflammation is an important pathological change and is a critical process leading to severe cirrhosis(Reference Albano and Vidali40). An anti-inflammatory substance has an improvement in early stages of liver injury(Reference Mandal, Pritchard and Nagy41). The results of the AST:ALT ratio suggest that the MCP treatment may be able to alleviate alcohol-induced hepatitis. However, it was not confirmed in the present study. In addition, ADH in hepatocytes is the specific enzyme that makes alcohol oxidise into acetaldehyde, playing an important role in the process of alcoholic liver injury. Wang et al. (Reference Wang, Wang and Liu9) found that the activity of hepatic ADH increased gradually along with the occurrence of ALD in rats induced by alcohol at a dose of 8 g/kg per d continuously for 4 weeks. In the present study, there was an increase in the ADH activity of the alcohol control group compared with that of the normal group (though not significant, P = 0·127), whereas the MCP treatment reversed the alcohol-induced increase in ADH activity (Fig. 2). Furthermore, experiments in human subjects and animals have shown that alcohol intake leads to a negative nitrogen balance, in spite of adequate protein intake. In the present study, there was not much difference in the contents of total proteins, albumin and globulin in serum between the alcohol and normal control groups. However, the albumin:globulin ratio was significantly different between the two groups (P < 0·05). Surprisingly, the albumin:globulin ratio could not be reversed by the MCP treatment and even raised a little (not significant) instead. There is no precise explanation about the alteration. It can be involved in the multi-effects of alcohol, including the immune system, biosynthesis of albumin, absorption of nutriments and so on(Reference Bunout8).
It has been well documented that alcohol-induced liver disease results from the dose- and time-dependent consumption of alcohol(Reference Isayama, Froh and Bradford42). The results of the AST:ALT ratio and ADH activity without a significant difference could be attributed to the short time or the low dose of alcohol intake (6 g/kg per d for 4 weeks). The design of the present experiment was to build the model of early alcohol-induced liver injury and promote a preliminary study of MCP on rats of this model; consequently, numerous changes in severe alcoholic liver injury reported by other investigators were not observed here.
Being safe and multi-functional, MCP have a strong potential for long-term use as a supplement agent for kinds of illness involving oxidative stress, ALD, for example. However, further studies such as those with a longer-term design and human trials are needed.
In conclusion, the present study indicated that MCP could inhibit early alcoholic liver injury in female rats based on the improvement of oxidative stress and lipid metabolism. Further studies are required to prove whether inflammation and ADH are involved in the protective mechanisms.
Acknowledgements
This study was supported (grant 2006BAD27B08) by the Foundation from the Ministry of Science and Technology of the People's Republic of China. The authors gratefully thank the company CF Haishi Biotechnology Limited for providing the samples used in the present study. The authors have no conflicts of interest to disclose. Y. L. gave the original idea and was in charge of the whole trial. B. L. conducted the animal experiment and wrote the manuscript. F. Z., Y. Y., Q. J., Z. Z. and J. W. assisted with the animal trial and biochemical assays. B. L. and F. Z. were responsible for the critical revision of the manuscript.