Hypoplastic left heart syndrome is incompatible with life if left untreated. However, a pathway of palliation is possible through a series of staged operations culminating in a Fontan circulation. The medical and surgical management of hypoplastic left heart syndrome highlights the most innovative and courageous interventions for children with CHD. Operative survival rates for this previously near-fatal diagnosis in the neonatal period approach 90% at experienced centres.Reference Ohye, Schranz and D’Udekem 1 This group of patients has also been among the most carefully studied with a number of multi-centre trials that have evaluated various aspects of their treatment.Reference Ohye, Schranz and D’Udekem 1
During all stages of palliation, children with hypoplastic left heart syndrome are at risk of circulatory failure and mortality. Selected patients may undergo heart transplantation. The care of children with hypoplastic left heart syndrome is constantly evolving and efforts are undertaken in all stages to decrease mortality and improve quality of life. The purpose of this review article is to summarise recent advances in the critical care management of patients with hypoplastic left heart syndrome as summarised in a focused session at the 12th International Conference of the Paediatric Cardiac Intensive Care Society held on 9 December, 2016, in Miami Beach, Florida.
Fetal considerations and delivery
In recent years, a number of advances have occurred regarding the fetal and peri-natal management of hypoplastic left heart syndrome. Recent multi-centre data indicate that prenatal diagnosis rates for hypoplastic left heart syndrome vary from 39 to 75%.Reference Morris, Ethen and Penny 2 , Reference Atz, Travison and Williams 3 A prenatal detection rate of 97% has been reported in the context of a nationwide screening programme.Reference van Velzen, Clur and Rijlaarsdam 4
Establishing a prenatal diagnosis of hypoplastic left heart syndrome has several potential benefits.Reference Donofrio, Moon-Grady and Hornberger 5 First, an opportunity is created to provide parental education and counselling. Clinicians should provide expectant parents detailed information about staged surgical palliation, the potential for unplanned cardiac reinterventions (reoperations or interventional catheterisation), and the potential need to consider cardiac transplantation at any point during the child’s life. Life expectancy should be discussed, including the fact that only two-thirds of patients will survive to 5 years of age and that mortality following Fontan palliation is approximately 1% per year. The potential for complications and comorbidities should be discussed, including vocal cord paralysis, need for feeding tubes, arrhythmias, protein-losing enteropathy, and plastic bronchitis, among others. Common neurodevelopmental issues in patients with hypoplastic left heart syndrome should be specifically addressed. Evaluation for non-cardiac anomalies and genetic syndromes may be performed, as the presence of these comorbidities may affect outcomes.Reference Patel, Hickey and Mavroudis 6 Expectant parents should also be informed about available resources to address the above issues, such as high-performing cardiac centres, comprehensive single-ventricle clinics, neurodevelopmental clinics, and family support groups. After secondary evaluation at a paediatric cardiac centre, reported termination of pregnancy rates for hypoplastic left heart syndrome vary from 12 to 48%.Reference Atz, Travison and Williams 3 , Reference Beroukhim, Gauvreau, Benavidez, Baird, LaFranchi and Tworetzky 7 – Reference Kipps, Feuille and Azakie 9 The possibility to decline palliative surgery and opt for comfort care after birth should also be discussed with parents.
Selected fetuses may be candidates for in utero interventions aimed at altering the natural history of the heart defect. For example, in fetuses with aortic stenosis that will likely evolve into hypoplastic left heart syndrome, transcatheter transuterine balloon dilation of the aortic valve may lead to subsequent growth of left-sided heart structures, thereby providing the opportunity to pursue a two-ventricular circulation postnatally in a subset of patients.Reference Freud, McElhinney and Marshall 10 – Reference Emani, McElhinney and Tworetzky 12 In fetuses with hypoplastic left heart syndrome and a highly restrictive or intact atrial septum, creation or stenting of an atrial communication has also been reported.Reference Moon-Grady, Morris and Belfort 11 , Reference Marshall, Levine and Morash 13 , Reference Kalish, Tworetzky and Benson 14
More recent experimental efforts to alter in utero physiology in hypoplastic left heart syndrome include the provision of chronic maternal hyper-oxygenation. The rationale for this therapy is based on the principle that increased fetal pulmonary blood flow that occurs with higher oxygen concentrations leads to greater venous return to the left heart, which may, in turn, facilitate the growth of left-sided heart structures.Reference Lara, Morris and Maskatia 15 Fetuses with hypoplastic left heart syndrome are known to have reduced brain volume and metabolism.Reference Sun, Macgowan and Sled 16 Recent data suggest that these brain findings may be partly because of reduced cerebral oxygen delivery and consumption.Reference Limperopoulos, Tworetzky and McElhinney 17 Ongoing studies will determine whether provision of maternal supplemental oxygen may mitigate some of the abnormalities in cerebral development in fetuses with hypoplastic left heart syndrome (ClinicalTrails.gov NCT02965638). Global growth restriction is more frequently seen in fetuses with hypoplastic left heart syndrome compared with healthy controls. Recently described vascular and structural abnormalities in the placenta could be contributory, which presents another potential target for future investigation.Reference Jones, Olbrych and Smith 18
When compared with healthy controls, neonates with hypoplastic left heart syndrome are more likely to be born prematurely.Reference Williams, Ravishankar and Zak 19 Birth at earlier gestational ages, even during the “early term” period of 37 to 38 weeks, has been associated with adverse short- and long-term outcomes in neonates with critical CHD.Reference Costello, Pasquali and Jacobs 20 – Reference Calderon, Stopp and Wypij 22 Single-centre studies and a recent report from the National Paediatric Cardiology Quality Improvement Collaborative have demonstrated that neonates with a prenatal diagnosis of hypoplastic left heart syndrome are generally delivered at an earlier gestational age when compared with similar patients with a postnatal diagnosis.Reference Kipps, Feuille and Azakie 9 , Reference Brown, Cohen and O’Brien 23 Concerns for in utero fetal demise could potentially be contributory to these earlier deliveries. However, reported rates of in utero demise for fetuses with hypoplastic left heart syndrome are <2% and risk factors were present in all afflicted cases.Reference Rychik, Szwast and Natarajan 8 , Reference Allen, Benson and Haug 24 Thus, in the absence of compelling fetal or maternal indications for earlier delivery, it seems reasonable to delay any scheduled deliveries of neonates with hypoplastic left heart syndrome until 39 weeks of gestation.Reference Donofrio, Moon-Grady and Hornberger 5
Recent population-based data indicate that approximately one-third of live-born neonates with hypoplastic left heart syndrome die before any surgical intervention.Reference Morris, Ethen and Penny 2 Many of these deaths are related to delayed postnatal diagnosis and haemodynamic instability. Prenatal diagnosis facilitates birth at or near a quaternary care paediatric cardiac centre, thereby reducing the preoperative morbidity and mortality associated with postnatal diagnosis and remote delivery.Reference Morris, Ethen and Penny 2 , Reference Brown, Cohen and O’Brien 23 , Reference Thakur, Munk, Mertens and Nield 25 Given the variability that exists among centres for outcomes following the Norwood operation, prenatal diagnosis may also facilitate triage of cases to high-performing centres.Reference Morris, Ethen and Penny 2 , Reference Hornik, He and Jacobs 26 , Reference Pasquali, Jacobs and He 27
Despite the potential benefits of prenatal diagnosis of hypoplastic left heart syndrome summarised above, data are lacking that important outcomes such as operative mortality, overall infant mortality, and neurodevelopmental outcomes are improved. A recent meta-analysis concluded that the preoperative condition – e.g. lowest pH, moderate–severe ventricular dysfunction, use of vasoactive medications – of the patients is better in those with prenatal diagnosis but found no difference in preoperative or operative mortality.Reference Thakur, Munk, Mertens and Nield 25 Other recent multi-centre studies that also utilised data from tertiary care centres support these findings.Reference Atz, Travison and Williams 3 , Reference Brown, Cohen and O’Brien 23 A recent population-based study concluded that higher prenatal diagnosis rates and triage of hypoplastic left heart syndrome neonates to high-volume surgical centres may lead to improvement in infant survival.Reference Morris, Ethen and Penny 2 Larger population-based studies are needed to determine whether prenatal diagnosis of hypoplastic left heart syndrome has an impact on infant mortality and long-term outcomes.
Stage 1: the Norwood procedure
Introduction
The palliative approach to children with hypoplastic left heart syndrome typically begins with a Stage 1 Palliation. The most common surgical Stage 1 procedure is the Norwood procedure, which was first described in 1981 (Fig 1).Reference Norwood, Lang, Casteneda and Campbell 28 Recently, as an alternative to the Norwood operation, a few cardiac centres have pioneered a hybrid technique integrating interventional catheterisation and surgical procedures (Fig 2).Reference Karamlou and Peyvandi 29 , Reference Caldarone, Benson, Holtby, Li, Redington and Van Arsdell 30 The hybrid procedure has the potential advantage of avoiding the need for cardiopulmonary bypass during the neonatal period with potential detrimental effects on myocardial function and the brain.
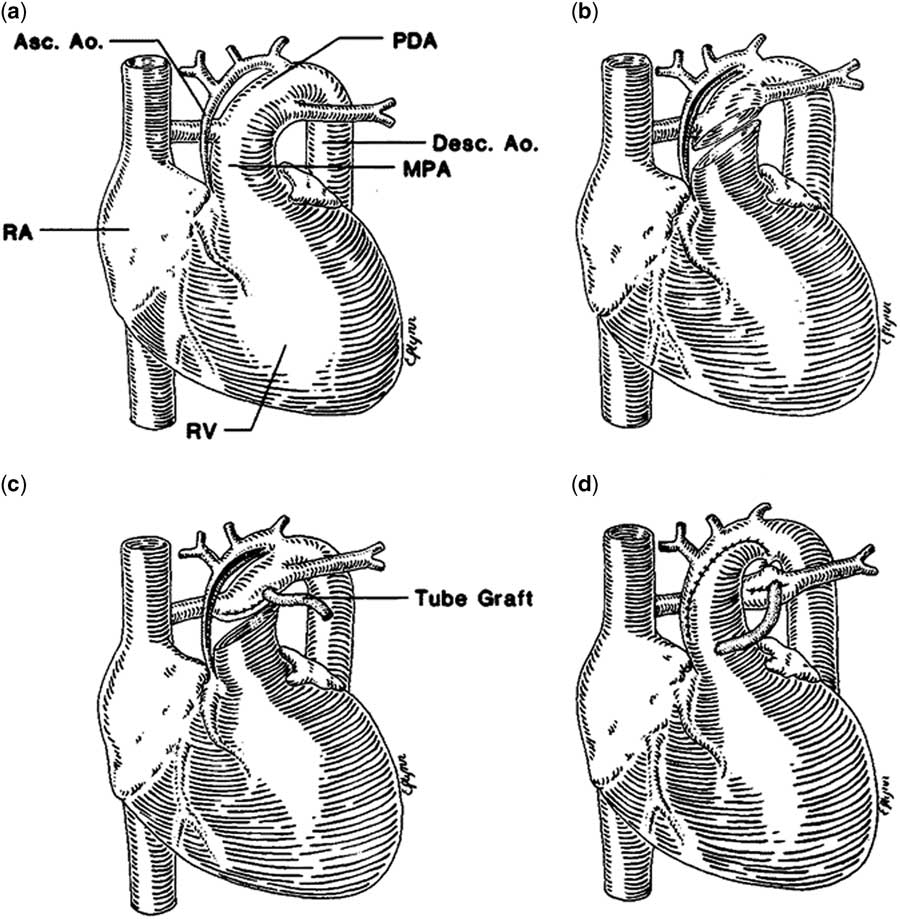
Figure 1 Norwood operation. Reprinted with permission from Norwood et al.Reference Egan, Hill, Boettner and Holzer 36 The heart with aortic atresia has a diminutive ascending aorta (Asc. Ao) and a large patent ductus arteriosus (PDA) ( a ). The main pulmonary artery (MPA) is transected and an incision is made in the ASc.Ao and aortic arch ( b ). The distal MPA is oversewn, and the distal anastomosis of the 4-mm shunt is established ( c ). The MPA is anastomosed to the Asc.Ao and aortic arch, the ductus arteriosus is ligated, and the shunt is completed ( d ). Desc. Ao=descending aorta; RA=right atrium; RV=right ventricle.

Figure 2 Hybrid approach to hypoplastic left heart syndrome (HLHS). Reprinted with permission from PCICS. Stage 1 hybrid procedure: a stent is placed in the patent ductus arteriosus (PDA) providing unobstructed systemic perfusion. Two bands are placed around both pulmonary arteries (PA) to control pulmonary blood flow. Finally, a balloon atrial septostomy is performed to allow for unobstructed pulmonary venous return and sufficient intra-atrial mixing.
Concurrently with its successes in palliating infants with hypoplastic left heart syndrome, the Norwood procedure garnishes special consideration because it continues to be associated with one of the highest mortality rates among common congenital heart procedures.Reference O’Brien, Clarke and Jacobs 31 Furthermore, these complex patients often have significant morbidities that lead to significant resource utilisation,Reference Kogon, Kanter, Alsoufi, Maher and Oster 32 including prolonged ventilator times and extended ICU stays.Reference McHugh, Pasquali, Hall and Scheurer 33
Preoperative considerations
Before surgery, the neonate with hypoplastic left heart syndrome needs to be optimised medically to help stabilise them for a lengthy operative procedure. With the rise of fetal diagnosis, most neonates will not present in extremis with systemic hypoperfusion after the ductus arteriosus begins to close. For those who are prenatally diagnosed, they will often require minimal respiratory support and maintenance on a low-dose prostaglandin infusion – often 0.01 to 0.03 μg/kg/minute.
As the pulmonary vascular resistance begins to fall in the early period, manifestations of pulmonary overcirculation can become more apparent – including tachycardia, tachypnoea, rising lactic acid values, decreasing urine output. A variety of medical interventions can be performed to help support the infant and balance the ratio of systemic and pulmonary blood flow. This may include intubation, with sedation and paralysis, to decrease oxygen consumption, increasing haematocrit for oxygen delivery, vasoactive agents such as milrinone for systemic perfusion, and other techniques to increase pulmonary vascular resistance such as sub-atmospheric oxygen, hyper-carbic gas mixtures, increasing positive end-expiratory pressure. Unfortunately, many of these solutions tend to be short term and there needs to be a more aggressive method for limiting pulmonary blood flow.
Depending on the clinical presentation of the neonate, many centres will delay the timing of the Stage 1 palliation until the child is in a more stable clinical condition. During these periods, the role of using a hybrid variation such as long-term prostaglandin infusion with branch pulmonary artery banding, extracorporeal membrane oxygenation, or proceeding with a Stage 1 palliation will depend on institutional preferences.
Intraoperative considerations
When considering the first stage of palliation for a hypoplastic left heart syndrome, there are three primary goals.
∙ First, provide unobstructed systemic blood flow: neonates with hypoplastic left heart syndrome are unable to provide adequate cardiac output through the native left ventricular outflow tract.Reference Noonan and Nadas 34 Before the Stage 1 palliation, these patients are dependent on systemic blood flow from a ductus arteriosus, the patency of which is maintained with a prostaglandin infusion. To overcome this anatomic liability, the Norwood operation fashions a reconstructed aorta using the native diminutive ascending aorta and the native main pulmonary aorta. The main pulmonary artery is transected and anastomosed to the ascending aorta and aortic arch and the undersurface of the aortic arch is augmented.Reference Norwood, Lang and Hansen 35 Together, this complex aortic arch reconstruction provides unobstructed egress from the right ventricle to the systemic circulation. For patients undergoing a hybrid variation, a stent is deployed to maintain ductal patency. In selected patients, a second stent is placed in the aortic isthmus to prevent the development of a retrograde coarctation.Reference Egan, Hill, Boettner and Holzer 36
∙ Second, provide a stable and controlled source of pulmonary blood flow: because the pulmonary vascular resistance becomes much lower than the systemic vascular resistance within the first few days of life, systemic perfusion can be compromised if the two sources of blood flow are left in parallel. To avoid compromising systemic perfusion, the pulmonary vascular bed must receive blood in a limited manner.Reference Barnea, Santamore, Rossi, Salloum, Chien and Austin 37 During the initial attempts to perform the Norwood procedure, pulmonary blood flow was provided using a right ventricle to pulmonary artery shunt.Reference Norwood, Lang, Casteneda and Campbell 28 However, all these early patients died, and this source of pulmonary blood flow was abandoned in favour of a modified Blalock–Taussig shunt. An appropriately sized modified Blalock–Taussig shunt provides a stable, restrictive connection between the subclavian or innominate artery and the pulmonary arteries. However, the right ventricle to pulmonary artery shunt was reintroduced by Sano and colleagues in the early 2000s and became a popular alternative.Reference Norwood, Lang, Casteneda and Campbell 28 , Reference Pizarro, Malec and Maher 38 – Reference Sano, Ishino, Kawada and Arai 41 In the hybrid variation, bilateral pulmonary artery bands are placed to limit blood flow to the lungs.Reference Karamlou, Overman and Hill 42
∙ Third, remove any restriction to pulmonary venous return: to allow adequate flow from the pulmonary vascular system and encourage pulmonary vascular resistance to fall appropriately, unrestricted flow from the pulmonary veins is necessary. Unobstructed left atrial egress is achieved with an atrial septectomy, as a part of the Norwood operation, or with a stent in the atrial septum, when done with a hybrid procedure.Reference Gibbs, Rothman, Rees, Parsons, Blackburn and Ruiz 43
Together, accomplishing these three aspects of the Stage 1 palliation allows the neonate to survive and grow for future palliations. The objectives of this first stage include the following: preserving ventricular function by removing any systemic outflow obstruction while maintaining coronary perfusion, maintaining competency of the tricuspid valve and the neo-aortic valve, encouraging long-term growth of the pulmonary arteries and allowing pulmonary vascular resistance to fall, and promoting somatic growth and development. Achieving each of these goals is important for optimising the prospects for successful Fontan palliation.
As mentioned earlier, one of the main decisions when approaching the Norwood operation is the source of pulmonary blood flow (Fig 3). Physiologically, the modified Blalock–Taussig shunt places an infant at higher risk for coronary ischaemia, because of the diastolic runoff, but may allow improved growth of pulmonary arteries with a subsequent delayed second stage of palliation. In contrast, the right ventricle to pulmonary artery shunt has been associated with a more stable initial post-operative period without the risk for coronary compromise, but its placement directly injures the systemic right ventricle with a ventriculotomy.Reference Ohye, Ludomirsky, Devaney and Bove 40 Furthermore, the right ventricle to pulmonary artery shunt may present with lower oxygen saturations because of diminished pulmonary blood from the often obligate pulmonary regurgitation during diastolic filling of the ventricle.Reference Ohye, Gaynor and Ghanayem 44 Hence, both of these techniques carry a different set of advantages and disadvantages.
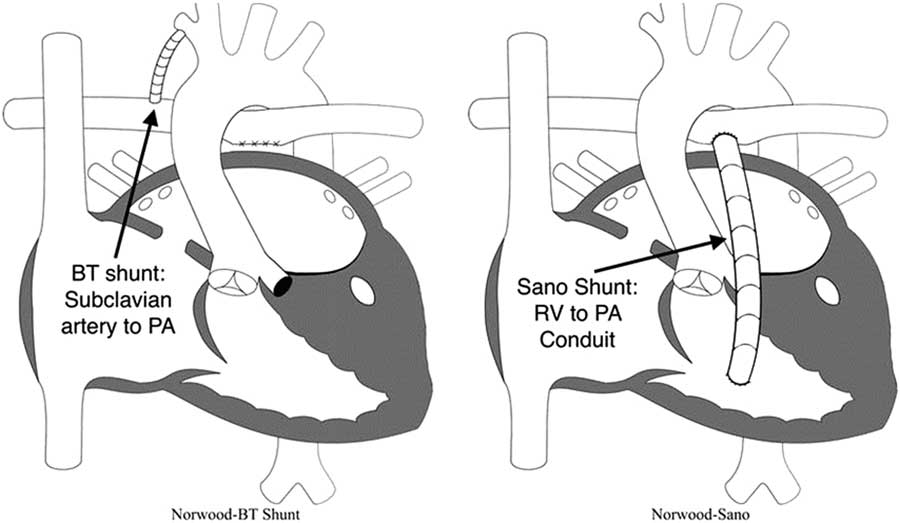
Figure 3 Modified Blalock–Taussig (BT) shunt and right ventricle (RV) to pulmonary artery (PA) shunt (also known as “Sano shunt”) variations of the Norwood operation. Reprinted with permission from PCICS.
The landmark multi-centre single-ventricle reconstruction trial was a randomised comparison of these two shunt types.Reference Ohye, Sleeper and Mahony 45 In the 1st year, the right ventricle to pulmonary artery shunt group appeared to have a lower attrition rate, but the transplant-free benefit compared with the modified Blalock–Taussig shunt did not persist over time.Reference Newburger, Sleeper and Frommelt 46 The latter group also required fewer interventions on the pulmonary arteries, but those patients did have a higher rate of cardiopulmonary resuscitation in the post-operative period.Reference Ohye, Sleeper and Mahony 45 Ultimately, without a proven intermediate-term benefit of one shunt type over the other, selection of shunt type often relies on surgeon preference.
There are also certain risk factors for each patient that may affect outcomes (Table 1). Infants with the mitral stenosis/aortic atresia variation of hypoplastic left heart syndrome tend to have a higher mortality rate; this subgroup of patients demonstrate higher rates of coronary abnormalities and hence are more likely to be sensitive to coronary ischaemia.Reference Vida, Bacha and Larrazabal 47 As a result, the right ventricle to pulmonary artery shunt could be protective compared with the modified Blalock–Taussig shunt for this specific anatomic variation.Reference Ohye, Sleeper and Mahony 45 , Reference Siehr, Maeda and Connolly 48 Other anatomic risk factors include a hypoplastic ascending aorta and a preoperative restrictive atrial septum or any aetiology for obstructed pulmonary venous return.Reference Sata, Sinzobahamvya, Arenz, Zartner, Asfour and Hraska 49 For these specific risk factors, there is often institutional preference about the ideal source of pulmonary blood flow.
Table 1 Preoperative risk factors prior to stage-1 palliation.

Neonates with non-cardiac congenital anomalies, pre-maturity, and low birthweight – generally <2.5 kg – have higher operative mortality.Reference Patel, Hickey and Mavroudis 6 , Reference Alsoufi, Mori and Gillespie 50 , Reference Kalfa, Krishnamurthy and Levasseur 51 Finally, patients with preoperative metabolic acidosis, those requiring inotropic support, right ventricular dysfunction, tricuspid regurgitation, and pulmonary valve regurgitation are also in higher-risk categories.Reference Shamszad, Gospin, Hong, McKenzie and Petit 52 , Reference Tabbutt, Ghanayem and Ravishankar 53
The optimal timing for performing a Norwood operation remains a matter of some debate. Most centres agree that performing a Norwood procedure on a child older than 2–4 weeks of age carries greater risk, probably because of the development of pulmonary vascular disease that complicates the early post-operative period.Reference Alsoufi, Manlhiot and Al-Ahmadi 54 Within this early window, there remains some debate with regards to timing based on balancing cardiovascular stability with neurodevelopmental outcomes.Reference Lynch, Buckley and Schwab 55
Post-operative considerations
Early after the Stage 1 palliation, neonates are quite vulnerable for cardiopulmonary instability, and at least some manifestation of a low cardiac output syndrome commonly develops. After being exposed to cardiopulmonary bypass, there is a period of systemic inflammatory response with many acute changes in the relative balance of circulation between the pulmonary and systemic circulation. Techniques to optimise cardiac output include managing pulmonary blood flow, limiting oxygen consumption, and managing systemic and pulmonary vascular resistance. Interestingly, many of the same techniques used preoperatively are also effective in the post-operative period, with the added benefit of surgical interventions, such as an open chest to help relieve tamponade physiology and technical variations to limit pulmonary blood flow, by altering the size of the shunt.
Typically, oxygen saturations of 75–85% are targeted after the Norwood operation. However, the correlation between the systemic oxygen saturation and true pulmonary to systemic blood flow ratio after the Norwood operation is poor.Reference Taeed, Schwartz and Pearl 56 In the early post-operative period, adjuncts for cardiac output, including near-infrared spectroscopy, serum lactic acid, and blood gasses, can be trended. Excessive cyanosis after the Norwood operation may be caused by pulmonary venous desaturation, systemic venous desaturation, decreased pulmonary to systemic blood flow ratio, or some combination of these factors (Table 2).
Table 2 Causes of hypoxaemia in patients with functionally univentricular hearts.
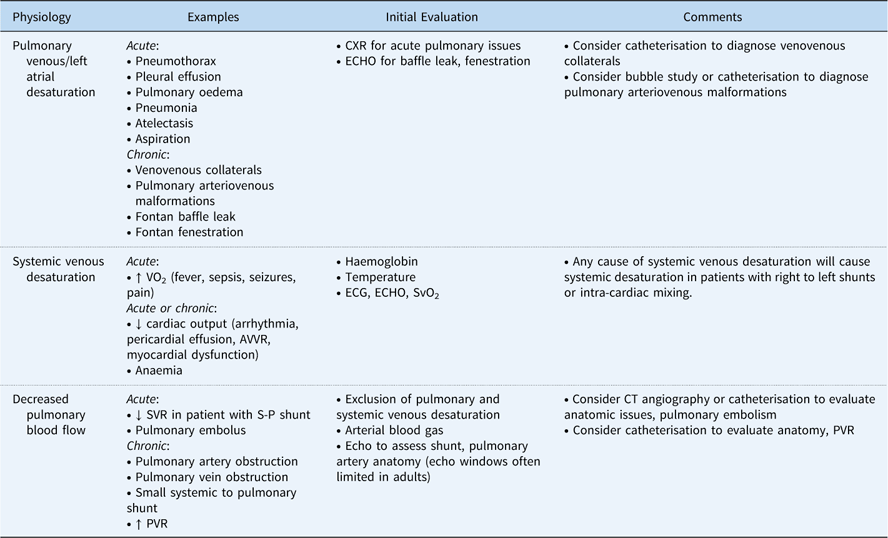
AVVR=atrioventricular valve regurgitation; CXR=chest radiograph; ECG=electrocardiogram; ECHO=echocardiogram; PVR=pulmonary vascular resistance; S-P=systemic to pulmonary; SVR=systemic vascular resistance; SvO2=systemic venous oxygen saturation; VO2=oxygen consumption
Cardiac arrest
Neonates with single-ventricle physiology have an increased risk of cardiac arrest (12.7%) with high mortality (62.3%).Reference Gupta, Jacobs and Pasquali 57 Since the inception of this consensus statement, the American Heart Association has published an excellent review on cardiopulmonary resuscitation in infants and children with cardiac disease, including single-ventricle patients.Reference Marino, Tabbutt and MacLaren 58 In neonates with single-ventricle physiology, it is difficult to obtain effective pulmonary blood flow during resuscitation as pulmonary blood flow is shunt dependent and will probably be affected by the relationship between pulmonary vascular resistance and either the aortic relaxation (diastolic) pressure – for modified Blalock–Taussig shunts – or the systemic vascular resistance – for modified Blalock–Taussig and right ventricle to pulmonary artery shunts. When cardiac arrest develops, providers should begin conventional cardiopulmonary resuscitation and additional management should be considered promptly. These include the urgent opening of the sternum in the immediate post-operative period, treatment of arrhythmias or use of external pacing if indicated, treatment of possible shunt thrombosis, and early consideration of mechanical circulatory support.Reference Marino, Tabbutt and MacLaren 58
Mechanical circulatory support
Mechanical circulatory support is occasionally utilised after the Norwood procedure. Indications include hypoxia from an acute interruption to pulmonary blood flow or profound systemic hypoperfusion because of inadequate systemic blood flow. Extracorporeal membrane oxygenation provides short-term support during this period, facilitates the investigation of residual lesions, and provides time for myocardial recovery. In a recent Extracorporeal Life Support Organisation Registry study, the use of extracorporeal support following the Norwood operation was associated with an overall survival to hospital discharge rate of 31%.Reference Sherwin, Gauvreau and Scheurer 59 Survival is remarkably better for patients cannulated for hypoxaemia (81%) compared with those cannulated for hypotension (29%).Reference Allan, Thiagarajan, del Nido, Roth, Almodovar and Laussen 60 In patients with a modified Blalock–Taussig shunt on extracorporeal membrane oxygenation (ECMO), pulmonary overcirculation can cause systemic hypoperfusion, and higher ECMO flows are needed to provide adequate systemic perfusion as clipping the shunt is contra-indicated.Reference Jaggers, Forbess and Shah 61 In patients with a right ventricular to pulmonary artery shunt, minimising the flow seems to be the most common approach to promote right ventricle to pulmonary artery shunt flow and therefore pulmonary flow. Extracorporeal support in children following the hybrid Stage 1 carries a poor survival rate of 16%.Reference Roeleveld, Wilde, Hazekamp, Rycus and Thiagarajan 62
Long-term extracorporeal support outcomes after a Norwood-type operation are not well described. Duration of extracorporeal support and serum lactate clearance within 24 hours of emergency cannulation to extracorporeal membrane oxygenation may influence long-term survival.Reference Polimenakos, Rizzo, El-Zein and Ilbawi 63 Friedland-Little et alReference Friedland-Little, Uzark, Yu, Lowery, Aiyagari and Hirsch-Romano 64 found no significant difference in functional status or quality of life among those who required extracorporeal support and those who did not after the Norwood operation. When compared with healthy normative data, 18% of paediatric cardiac extracorporeal membrane oxygenation survivors showed significant physical limitations and significantly lower Paediatric Quality of Life Inventory score in children, but not among adolescents.Reference Elias, Achuff and Ittenbach 65
Short-term mechanical support without an oxygenator, with a ventricular assist device, is also performed in selected centres with outcomes comparable with extracorporeal membrane oxygenation support.Reference Poh, Chiletti and Zannino 66
The operative mortality for neonates with hypoplastic left heart syndrome undergoing Stage 1 palliation continues to decline.Reference Pasquali, Ohye and Lu 67 , Reference Feinstein, Benson and Dubin 68 As demonstrated in the single-ventricle reconstruction trial, survival of Stage 1 palliation can approach 90% at many centres. As the discharge survival rate improves, there has been a growing recognition of the many complications that arise during their hospital stay and a renewed focus on eliminating these. Some of the common complications include arrhythmias, respiratory compromise, infections, bleeding difficulties, seizures, renal dysfunction, and feeding difficulties.Reference Feinstein, Benson and Dubin 68 – Reference Oster, Chen and Dagincourt 70 An aggressive approach to identifying and resolving these post-operative problems is warranted.
Following hospital discharge, infants with hypoplastic left heart syndrome have an ongoing need for high-level medical care. In the current era, there has been a growing awareness of the importance of closely following these outpatients to optimise growth in order to minimise inter-stage mortality. Parents are burdened with multiple medication administrations, frequent readmissions, and complex feeding regimens.Reference Kogon, Jain, Oster, Woodall, Kanter and Kirshbom 71 To address this fragile period, the establishment of home monitoring programmes has been associated with improved weight gain and inter-stage survival.Reference Ugonabo, Hirsch-Romano and Uzark 72
Stage 2: superior cavopulmonary connection
Introduction
The second stage in single-ventricle palliation is the superior cavopulmonary connection. Contemporary variants include the bidirectional Glenn or the hemi-Fontan procedure. The major goal of the Stage 2 procedure is to reduce the volume load on the single ventricle by directing the systemic venous return from the upper body directly to the lungs via the superior cavopulmonary connection. The reduced volume load has been shown to improve diastolic function and reduce ventricular wall thickness at the time of the Fontan procedure.Reference Pridjian, Mendelsohn and Lupinetti 73 – Reference Fogel, Weinberg, Chin, Fellows and Hoffman 76 The superior cavopulmonary connection is now routinely used by most centres as a staging procedure before Fontan completion.Reference Scheurer, Hill and Vasuki 77
The bidirectional Glenn is an end-to-side anastomosis of the superior caval vein to the pulmonary artery (Fig 4a). The hemi-Fontan procedure maintains the native SVC to the right atrium junction and is an anastomosis of this confluence to the branch pulmonary artery using a homograft dam to redirect superior caval vein flow to the pulmonary arteries (Fig 4b). Although they are different procedures, the post-operative physiology is almost identical. The comprehensive Stage 2 follows the hybrid Stage 1 procedure, and in addition to the cavopulmonary anastomosis it involves a Norwood-type arch reconstruction, atrial septectomy, and removal of the ductal stent and pulmonary artery bandsReference Galantowicz, Cheatham and Phillips 78 The post-operative course is different from the typical bidirectional Glenn or hemi-Fontan, given that the Norwood-type operation spared in the neonatal period is performed at this time.
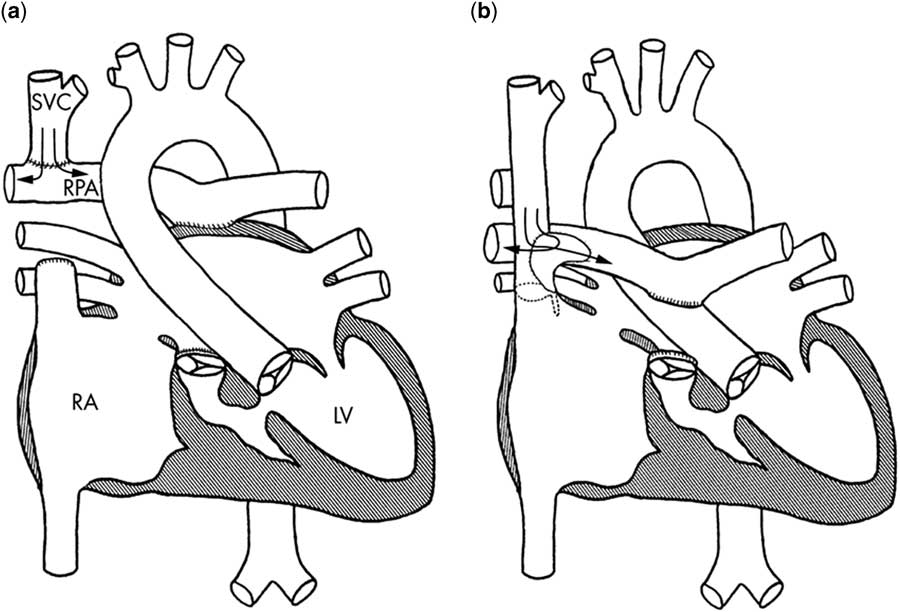
Figure 4 Current superior cavopulmonary connections. The bidirectional Glenn ( a ) and the hemi-Fontan ( b ) are different surgical techniques, but offer similar physiology. The bidirectional Glenn anastomosis is constructed by disconnecting the main pulmonary artery (oversewn at valvular level), dividing the superior caval vein (SVC) and connecting the distal SVC to the right pulmonary artery (RPA). The hemi-Fontan operation consists of a right atrial to RPA anastomosis, the RPA is augmented anteriorly with a pulmonary allograft patch to create a wide pathway that conducts blood from the SVC to the pulmonary artery; a portion of the allograft gusset is used to close the junction of the right atrium (RA) with the SVC. LV=left ventricle. In case of a comprehensive stage 2 hybrid procedure (not shown), a neo-aorta is constructed using the native pulmonary artery and hypoplastic native aorta and a atrial septectomy is performed. A SVC is made to the RPA. The pulmonary artery bands are removed and the pulmonary arteries are repaired (if necessary). Reprinted with permission from Kaulitz R. Current treatment and prognosis in children with functionally univentricular hearts. Arch Dis Child 2005; 90: 757–762.
Preoperative considerations
Infants with hypoplastic left heart syndrome typically undergo a detailed preoperative evaluation before the second stage of palliation. This evaluation is needed in order to determine the candidacy for a superior cavopulmonary connection and identify the residual issue that needs to be addressed before or during the operation.
First, the superior cavopulmonary connection is dependent on passive pulmonary blood flow. Post-operative physiology is optimal with low pulmonary artery pressure and low pulmonary vascular resistance, allowing unobstructed blood flow to the lungs.Reference Scheurer, Hill and Vasuki 77 The presence of elevated pulmonary artery pressure or less commonly elevated pulmonary vascular resistance or pulmonary venous obstruction preoperatively will likely be not well tolerated following the operation. An assessment of atrioventricular valve and right ventricular function and the systemic outflow tract is needed.
Initial assessment includes electrocardiogram, echocardiogram, and cardiac catheterisation before undergoing a Stage 2 palliation. The catheterisation provides an assessment of pulmonary vascular resistance and provides the option to dilate the pulmonary artery and/or pulmonary valve if necessary.Reference Brown, Gauvreau and Moran 79 Patients who underwent Stage 1 hybrid palliation may develop pulmonary artery stenosis because of the bilateral pulmonary artery banding and require more frequent intervention than patients who underwent conventional Stage 1 Norwood palliation.Reference Dave, Rosser, Knirsch, Hubler, Pretre and Kretschmar 80 , Reference Davies, Radtke, Klenk and Pizarro 81 Second, infants who undergo a Norwood Stage 1 are at risk for aortic arch narrowing or re-coarctation during the inter-stage period, which may cause elevated end-diastolic pressure in the systemic ventricle and subsequent elevated pulmonary pressure and atrioventricular valve incompetence.Reference Scheurer, Hill and Vasuki 77 , Reference Eagam, Loomba, Pelech, Tweddell and Kirkpatrick 82 Dilation of the aortic arch, reducing the left ventricular outflow tract gradient during the preoperative cardiac catheterisation, may optimise post-operative haemodynamics. Third, atrioventricular valve competence is critically important for optimal single-ventricle physiology.Reference Scheurer, Hill and Vasuki 77 A severely incompetent valve may require surgical intervention during the second stage, increasing the complexity of the operation. Last, pulmonary vein desaturation noted preoperatively may be the result of lung disease and may help explain low oxygen saturation post-operatively. Patient characteristics thought to provide optimal outcome for superior cavopulmonary connection palliation are shown in Table 3.
Table 3 Optimal pre-SCPC characteristics.
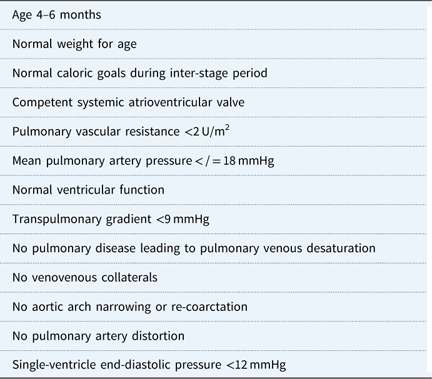
In carefully selected patients, cross-sectional imaging with either a cardiac CT angiogram or MRI can be safely performed and may be sufficient in lieu of a cardiac catheterisation.Reference Brown, Gauvreau and Moran 79 , Reference Brown, Gauvreau and Powell 83 , Reference Han, Vezmar and Lesser 84 The possible benefit of CT angiogram includes decreased vascular access and anaesthesia risk, lower radiation and contrast exposure, and fewer adverse events.Reference Han, Vezmar and Lesser 84 The advantage of conventional angiography over CT angiogram or MRI is the ability of immediate intervention.
Risk factors for adverse outcomes after superior cavopulmonary connection
Infants with hypoplastic left heart syndrome who meet caloric goals in the inter-stage period between the first and second stage have a shorter hospital stay after the second stage.Reference Menon, McCandless and Mack 85 Additional independent risk factors for post-operative complications following Stage 2 palliation include lower weight for age, female sex, and aortic atresia with mitral stenosis.Reference Menon, McCandless and Mack 85 Most patients are aged between 4 and 6 months at the time of their Stage 2 operation with a mean hospital length of stay of 8 days.Reference Baker-Smith, Goldberg and Rosenthal 86 Younger children and those with a non-cardiac anomaly had longer hospital stays. On multi-variate analysis, factors associated with a length of stay greater than 14 days were the need for reoperation or cardiac catheterisation, the development of post-operative complications, and the use of non-oral forms of nutrition.Reference Baker-Smith, Goldberg and Rosenthal 86 Ventricular dysfunction, atrioventricular valve regurgitation, and unbalanced atrioventricular septal defects are risk factors for decreased survival to Fontan.Reference Lee, Aiyagari, Hirsch, Ohye, Bove and Devaney 87
Post-operative considerations
Optimising post-operative haemodynamics after Stage 2 palliation requires an understanding of the specific cardiopulmonary interactions associated with the post-operative anatomy. Maintaining a low transpulmonary gradient encourages pulmonary blood flow and optimises cardiac output. The concept that positive pressure ventilation reduces pulmonary blood flow in patients after the bidirectional Glenn and Fontan procedure is widely accepted and drives the practice of early extubation in this population.Reference Redington, Penny and Shinebourne 88 , Reference Lofland 89 It has been shown that cerebral near-infrared spectroscopy and cardiac index increase and central venous pressure decrease after extubation, suggesting an improved cerebral perfusion pressure after the removal of positive pressure.Reference Huang, Zhou and Zhu 90
The goal of post-operative ventilation is to target functional residual capacity to achieve adequate gas exchange with spontaneous breathing. Pulmonary vascular resistance is lowest when functional residual capacity is achieved.Reference Bronicki and Anas 91 Both atelectasis and overdistension of the lungs cause an increase in pulmonary vascular resistance. Spontaneous breathing, generated with negative intrathoracic pressure, increases right atrial transmural pressure, reduces right atrial pressure, and improves systemic venous return.Reference Bronicki, Penny, Anas and Fuhrman 92
The pulmonary venous return may be reduced in the setting of diastolic dysfunction, atrioventricular valve insufficiency, a restrictive atrial septum, or loss of atrioventricular synchrony. Interventions to treat any of these modifiable conditions are essential to optimise pulmonary venous return, thereby improving cardiac output and the aortic saturation.
Typically, oxygen saturations of 70–80% are targeted post-operatively. An approach to hypoxaemia following a superior cavopulmonary connection may be found in Table 2. In the setting of hypoxia, optimising oxygen saturations can be achieved by augmenting the “mixed” venous saturation – from the inferior caval vein – or pulmonary venous saturation. Transfusing with packed red blood cells in the setting of anaemia and supporting the overall cardiac output will optimise the “mixed” venous saturation. Diagnosing and treating pulmonary pathologies such as pulmonary oedema, pleural effusion, pneumothorax, atelectasis, or a malposition endotracheal tube will improve the pulmonary venous saturation and therefore the aortic saturation. In the setting of severe hypoxaemia, modest hypercapnia may augment cerebral and pulmonary blood flow and improve the arterial oxygen saturation.Reference Li, Hoskote, Hickey and Stephens 93 If indicated, oxygen consumption can be lowered by general measures such as analgesia, sedation, and preventing hyperthermia. If hypoxaemia persists, pulmonary venous collaterals or a restrictive atrial septum should be considered.
Cardiac arrest
In patients with a superior cavopulmonary connection, chest compressions will create systemic blood flow and only minimal pulmonary blood flow. This reduction in pulmonary blood flow will limit oxygenation and cardiac output.Reference Marino, Tabbutt and MacLaren 58 Also the elevation in superior caval vein pressure and cerebral venous pressure during chest compressions will limit cerebral blood flow and increase the risk for neurological injury.Reference Jolley, Thiagarajan and Barrett 94 Chest recoil will produce blood flow through the lungs, as well as from the inferior caval vein into the systemic venous atrium, providing important pre-load for the next compression.Reference Marino, Tabbutt and MacLaren 58
Mechanical circulatory support
Mechanical circulatory support presents a challenge in patients after the Stage 2 operation related to cannulation, adequate extracorporeal membrane oxygenation support, and adequate cerebral drainage with high risk of neurologic injury.Reference Booth, Roth, Thiagarajan, Almodovar, del Nido and Laussen 95 However, it may be the only option to support decompensated heart failure that is refractory to medical management. In 2014, an Extracorporeal Life Support Organisation review of 103 children supported on extracorporeal membrane oxygenation after Stage 2 palliation showed 41% survival to hospital discharge, with 14% of the survivors suffering a neurological complication.Reference Jolley, Thiagarajan and Barrett 94 A more recent review of the extracorporeal life support organisation registry of infants on extracorporeal membrane oxygenation after comprehensive Stage 2 palliation showed only six infants requiring mechanical support with four surviving to hospital discharge.Reference Gomez, Duffy and Hersey 96 Longer-term mechanical support with ventricular assist devices in this population is emerging as another potential option to bridge these infants to heart transplant.Reference Niebler, Shah and Mitchell 97 Given the complex cardiopulmonary and neurologic interactions associated with supporting a patient after the Stage 2 operation on mechanical circulatory support, careful consideration of the risks must be weighed. The limited available data suggest that mechanical circulatory support is an option in the setting of refractory heart failure.
Stage 3: the total cavopulmonary connection
Introduction
The Fontan operation is the final procedure in staged palliation for patients with single-ventricle physiology. The procedure completes the separation of pulmonary from systemic circulation and relies on passive return of blood from the systemic vascular bed directly to the pulmonary vascular bed. The goal of the total cavopulmonary connection is to achieve normal oxygen saturation.
In 1971, Fontan published the first series of three patients with tricuspid atresia repaired with Fontan-type palliation.Reference Fontan and Baudet 98 In this small case series, Fontan describes a number of the important tenets of post-operative care and potential complications that still apply today: early elimination of positive pressure ventilation to augment venous return, the risks of atrial arrhythmias, and concern for prolonged pleural effusions.Reference Fontan and Baudet 98
Since its initial description, the Fontan procedure has undergone a number of modifications. Currently, the procedure is performed largely in one of two ways: lateral tunnel Fontan, which incorporates an intra-atrial conduit from the inferior caval vein to the pulmonary artery, and the extracardiac Fontan, which utilises a conduit connecting the inferior caval vein to the pulmonary arteries with the intention of avoiding common atrial distension with concomitant atrial arrhythmias. In a randomised trial, Lemler et al.Reference Lemler, Scott, Leonard, Stromberg and Ramaciotti 99 demonstrated the possible benefit of a fenestration of the Fontan baffle to decrease pleural drainage. Additional variations on each of these techniques have been described including the “intra-extracardiac” Fontan purported to reduce post-operative arrhythmias (Fig 5).Reference Sinha, Zurakowski and He 100
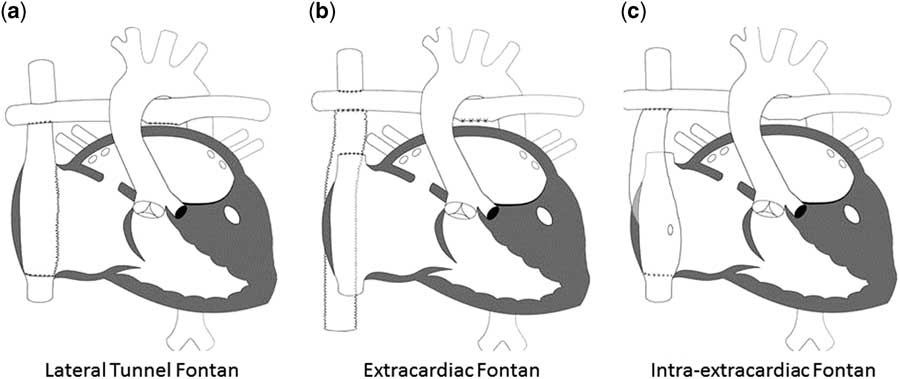
Figure 5 The different types of Fontan circulation. ( a ) Lateral tunnel Fontan connects the inferior caval vein to the right pulmonary artery, so that the blood is directed from the inferior caval vein to the pulmonary artery. ( b ) The extracardiac cavopulmonary connection consists of a direct anastomosis of the superior caval vein to the right pulmonary artery and in the interposition of an extracardiac prosthesis between the inferior caval vein and the right pulmonary artery. The advantage of the procedure is that it can be performed without myocardial ischaemia, there are fewer suture lines in the right atrium, and there are no foreign material in the right atrium. ( c ) The intra/extracardiac conduit fenestrated Fontan procedure incorporates an atrial incision that avoids injury to the sinus node, the sinus node artery, and the crista terminalis. Then, a conduit is sutured to the internal orifice of the inferior caval vein. The short intra-atrial segment is fenestrated. A suture tacks the atrial wall to the external surface of the conduit. The distal anastomosis of the intra/extracardiac is then connected to the Glenn anastomosis and pulmonary artery.
Preoperative considerations
The Fontan operation is typically performed between 18 months and four years of age. Despite the initial series presented by Fontan, recent literature supports that outcomes are optimised when the palliation is performed before adolescence.Reference Forsdick, Iyengar and Carins 101 Patient characteristics thought to provide optimal outcome for Fontan palliation are shown in Table 3 and include low ventricular complianceReference Garofalo, Cabreriza and Quinn 102 and adequate pulmonary artery size.Reference Fontan, Fernandez and Costa 103 , Reference Knott-Craig, Julsrud, Schaff, Puga and Danielson 104
Pre-Fontan catheterisation remains an important tool to assess haemodynamics and address anatomic considerations before surgery. Cardiac MRI is useful for assessment of anatomy, ventricular function, and collateral blood flow; however, interventions to occlude these collaterals are not possible. Despite the limitations of cardiac magnetic imaging, studies have demonstrated that in select pre-Fontan patients, patients undergoing cardiac magnetic imaging evaluation alone have similar post-operative outcomes compared with patients undergoing cardiac catheterisation.Reference Fogel, Pawlowski and Whitehead 105
While Fontan palliation can be completed on any underlying anatomic variant, heterotaxy syndrome has been shown to be a risk factor for morbidity and mortality.Reference Azakie, Merklinger, Williams, Van Arsdell, Coles and Adatia 106 – Reference Gentles, Mayer and Gauvreau 108 The poor outcome for patients with heterotaxy may be related to systemic venous anomalies and intrinsic conduction abnormalities, which increase complexity of the repair and short- and long-term morbidity. The shunt type used at initial Stage 1 repair in hypoplastic left heart syndrome patients has not been shown to affect short-term morbidity or mortality.Reference Ballweg, Dominguez and Ravishankar 109 In patients following hybrid palliation, pulmonary artery stenosis is a concern as mentioned before. However, despite requiring more interventions, Fontan candidacy is maintained.Reference Davies, Radtke, Klenk and Pizarro 81 The optimal pre-Fontan characteristics are summarised in Table 4.
Table 4 Optimal pre-Fontan characteristics
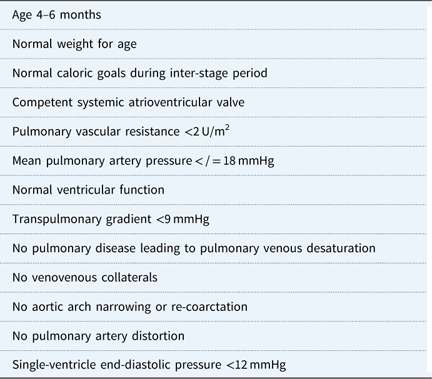
BSA=body surface area; PVR=pulmonary vascular resistance
Post-operative considerations
Post-operative care of the Fontan should focus on early extubation, judicious use of volume resuscitation, and ensuring atrioventricular synchrony. The impact of surgical variations on post-operative arrhythmias is unclear. There are multiple reports describing the impact of surgical approach on arrhythmias. A single-centre study comparing the intra-extra cardiac Fontan with the lateral tunnel demonstrated arrhythmia reduction with the intra-extracardiac variation, two weeks post-operatively.Reference Sinha, Zurakowski and He 100 Other studies have not shown a difference in arrhythmia based on surgical approach, neither directly post-operatively nor after 1 year of follow-up.Reference Hakacova, Lakomy and Kovacikova 110
In patients with Fontan physiology, spontaneous ventilation augments venous return, which may decrease the need for volume resuscitation and probably decrease chest tube drainage, which will have important short- and long-term benefits.Reference Shekerdemian, Bush, Shore, Lincoln and Redington 111 Negative-pressure cuirass ventilation has been shown to augment pulmonary blood flow in the post-operative period.Reference Shekerdemian, Bush, Shore, Lincoln and Redington 111 , Reference Penny and Redington 112 Although not practical in most ICUs, this study highlights the principle that early extubation should be prioritised. Mutsuga et alReference Mutsuga, Quinonez and Mackie 113 have shown that in select patients early extubation is safe. In a single-centre study assessing risk factors for prolonged recovery following Fontan procedure, greater volume resuscitation was the only independent risk factor for prolonged recovery.Reference Salvin, Scheurer and Laussen 114 Factors that might affect the amount of volume resuscitation in the early post-operative period include elevated pulmonary vascular resistance, increased end-diastolic pressure in the systemic ventricle, and inflammatory response. Patients requiring high-volume resuscitation are similarly at risk for increased pleural drainage, which may be minimised with surgical adjustments such as the baffle fenestration.Reference Lemler, Scott, Leonard, Stromberg and Ramaciotti 99
Typically, oxygen saturations>95% are targeted in the immediate post-operative period in non-fenestrated Fontan circulations and >80–85% in fenestrated Fontan circulations. In the setting of hypoxia, one should consider systemic venous desaturation, e.g. low cardiac output owing to an uncovered obstruction to systemic blood flow secondary to volume reduction, pulmonary venous desaturation, e.g. lung disease or pleural effusion, or decreased pulmonary to systemic blood flow ratio, e.g. increased pulmonary vascular resistance, venous collaterals (Table 2).
Cardiac arrest
As in children with superior cavopulmonary connection, survival from cardiac arrest in patients with total cavopulmonary connections is low (approximately 60%).Reference Gupta, Jacobs and Pasquali 57 During chest compressions, systemic blood flow is created with only minimal pulmonary blood flow. The pulmonary arteries are mainly filled with inferior caval vein flow during chest recoil, dependent on pulmonary vascular resistance. The flow through the superior caval vein is dependent on cerebral vascular resistance and pulmonary vascular resistance.Reference Marino, Tabbutt and MacLaren 58 The American Heart association recognises the poor outcomes of resuscitation after cardiac arrest in all three stages of palliation and stresses providers to recognise and intervene when pre-arrest low cardiac output and impaired oxygen delivery develop.Reference Marino, Tabbutt and MacLaren 58
Mechanical circulatory support
Support of the failing Fontan can be challenging related to cannulation and achieving adequate extracorporeal membrane oxygenation flow.Reference Booth, Roth, Thiagarajan, Almodovar, del Nido and Laussen 95 Multiple single-centre reports have shown success with various modalities for supporting the failing Fontan.Reference Imielski, Niebler, Kindel and Woods 115 – Reference Woods, Ghanayem, Mitchell, Kindel and Niebler 117 In a recent report from the Interagency Registry for Mechanically Assisted Circulatory Support (INTERMAX), of 16,182 adult patients, only 17 (0.1%) had a functionally univentricular heart defect. However, survival for these 17 patients (~70% at 12 months) was similar to adult congenital heart patients with two ventricles and with that of adults without CHD.Reference Arnaoutakis, Blitzer and Fuller 118 Understanding the aetiology of Fontan failure is important: both the heart, i.e. ventricular systolic and diastolic function, and the lungs can contribute to suboptimal haemodynamics leading to Fontan failure, and certain mechanical support devices may be superior to others dependent on aetiology of Fontan failure.Reference Giridharan, Ising and Sobieski 119
Stage 4: transplantation
The child with hypoplastic left heart syndrome is at risk for circulatory failure from the time of birth. Although the greatest risk of death is in the first several months of life, there is an ongoing risk of death and major morbidity that persists.Reference Rychik 120 For those who develop end-stage circulatory failure, heart transplantation is currently the only therapy that offers long-term survival. Unfortunately, many patients with hypoplastic left heart syndrome and other forms of single-ventricle heart disease have comorbidities and other factors that place them at high risk for poor outcomes both before and after transplantation. In a large study from the Paediatric Heart Transplant Study group assessing patients with hypoplastic left heart syndrome who were listed for transplantation after the Norwood operation, nearly 25% died before transplantation.Reference Alsoufi, Mahle and Manlhiot 121 Another example of the challenges associated with cardiac transplantation in hypoplastic left heart syndrome comes from the single-ventricle reconstruction trial where only 48% of infants with hypoplastic left heart syndrome who were listed for heart transplantation were alive 1 year later.Reference Kulkarni, Neugebauer and Lo 122
The pre-transplant condition of the patient is one of the most important determinants of post-transplant survival. Those with severe end-organ dysfunction at the time of heart transplantation are at a very high risk of death after transplantation, and it is often challenging to maintain adequate end-organ functioning in patients with hypoplastic left heart syndrome and severe heart failure.Reference Almond, Gauvreau, Canter, Rajagopal, Piercey and Singh 123 While the use of ventricular assist devices has improved the pre-transplant condition for many patients, they are uncommonly used in patients with hypoplastic left heart syndrome, and among infants post the Norwood operation, the outcomes have been particularly poor.Reference Arnaoutakis, Blitzer and Fuller 118 , Reference Weinstein, Bello and Pizarro 124 , Reference Rossano, Woods and Berger 125 As a consequence, these patients are at high risk of major comorbidities including respiratory failure, renal insufficiency, and poor nutrition. The majority of patients with severe end-organ dysfunction and mechanical ventilation or extracorporeal membrane oxygenation will not survive the hospitalisation after transplantation.Reference Almond, Gauvreau, Canter, Rajagopal, Piercey and Singh 123
Another important factor among patients with hypoplastic left heart syndrome is the development of anti-human leucocyte antigen antibodies.Reference O’Connor, Pahl, Webber and Rossano 126 Several factors can lead to the development of these antibodies including pregnancy and transfusions of blood and blood products. However, for patients with hypoplastic left heart syndrome, it is the use of cadaveric homograft tissue that is likely the greatest contributor.Reference Shaddy, Hunter and Osborn 127 When present, these antibodies can complicate what donors are considered acceptable, thus potentially prolonging the waiting list duration. After transplant, antibodies directed at the allograft – i.e. donor-specific antibodies – can lead to antibody-mediated rejection, cardiac allograft vasculopathy, and early graft failure.Reference O’Connor, Pahl, Webber and Rossano 126 , Reference Tambur, Pamboukian and Costanzo 128 – Reference Tran, Fixler, Huang, Meza, Lacelle and Das 130 Although there are a variety of treatments for the treatment of these antibodies including plasmapheresis, steroids, intravenous immunoglobulin, anti-lymphocyte globulin, anti-CD20 monoclonal antibody (rituximab), and proteasome inhibitors (bortezomib), refractory disease due to these donor-specific antibodies is not uncommon.Reference Colvin, Cook and Chang 131 In one study, children who developed donor-specific antibodies after transplantation experienced more than twice the rate of rejection events and had a 5-year survival of only 21%.Reference Tran, Fixler, Huang, Meza, Lacelle and Das 130
The overall survival for patients with hypoplastic left heart syndrome after transplant varies with the stage of palliation. Infants transplanted after the Norwood operation and before the bidirectional Glenn/hemi-Fontan operation have the greatest risk of death after transplantation. Data from the Paediatric Heart Transplant Study reported a 1-year survival of 70% of infants transplanted after the Norwood operation, compared with nearly 90% of these transplanted for cardiomyopathy and 82% for other forms of post-operative CHD.Reference Everitt, Boyle and Schechtman 132 For patients transplanted after the bidirectional Glenn/hemi-Fontan operation, the overall survival appears to be similar to patients with other forms of CHD.Reference Kovach, Naftel and Pearce 133 For patients who undergo transplantation after the Fontan, operation factors associated with early mortality include early Fontan failure, mechanical ventilation at the time of transplant, and preserved ventricular function.Reference Kovach, Naftel and Pearce 133 – Reference Simpson, Pruitt and Kirklin 135 However, advances have been made over time and several recent studies have reported the overall survival of heart transplantation after the Fontan operation as similar to other forms of CHD.Reference Simpson, Pruitt and Kirklin 135 , Reference Miller, Simpson and Epstein 136
It is clear that patients with hypoplastic left heart syndrome and other forms of single-ventricle heart disease are at high risk for circulatory failure, and thus heart transplantation remains an important part of their care. Much work is needed in the prevention and treatment of this circulatory failure and in improving the pre- and post-transplant outcomes of this high-risk population.
Contraindications
The contraindications to transplant for patients with hypoplastic left heart syndrome are similar to those of other potential candidates. Potential medical contraindications would include irreversible diseases in other organs, severe fixed pulmonary hypertension, or severe pulmonary vein stenosis. In addition, if social support is insufficient to achieve compliance with outpatient care, this would be considered a relative contraindication to transplant.Reference Mehra, Canter and Hannan 137
Conclusion
The combination of prenatal diagnosis, early preoperative evaluation, improved peri-operative care, and a greater appreciation for post-operative complications has helped to make the Norwood operation one of the most remarkable measures of success despite its technical complexities. Subsequent staged cavopulmonary connection has also evolved over the years, with increasing attention to neuromonitoring and long-term outcomes. The use of mechanical support, such as extracorporeal membrane oxygenation and ventricular assist devices, is emerging in this population. For selected children with circulatory failure, heart transplantation might be considered.
Acknowledgements
None.