Introduction
Metabolic dysfunction-associated steatotic liver disease (MASLD), previously called non-alcoholic fatty liver disease, is a disease with a global prevalence around 25% and increasing(Reference Cotter and Rinella1). MASLD is caused by the accumulation of triacylglycerol in hepatocytes over time and is characterised by fatty liver (hepatic steatosis) without any significant signs of inflammation. MASLD is a disorder caused by a combination of sedentary lifestyle, high-calorie diet, genetic factors, physiological and metabolic factors(Reference Wei, Wang and Evans2). Metabolic dysfunction-associated steatohepatitis (MASH) occurs with the progression of MASLD and the onset of an inflammatory process. The basis of the pathogenesis of MASH is the stress response to the excessive supply of nutrients to the liver, which promotes fibrosis and ultimately leads to cirrhosis(Reference Harrison, Allen and Dubourg3).
Hesperidin (HES), a polyphenolic plant compound, belongs to the flavanone class and was first isolated from citrus peel by the French chemist Lebreton in 1828(Reference Roohbakhsh, Parhiz and Soltani4). Hesperidin, also known as hesperetin-7-O-rutinoside, is a flavanone consisting structurally of the aglycone form and the disaccharide rutinose(Reference Choi, Lee and Lee5). The aglycone form is called hesperetin (HST)(Reference Actis-Goretta, Dew and Lévèques6). Various pharmacological effects of hesperidin are supported by different studies in the literature. Some of these effects are cardioprotective, anti-inflammatory, antidiabetic and antioxidant(Reference Welbat, Naewla and Pannangrong7–Reference Alharbi, Alshehri and Alshehri10).
In this review, we will discuss how hesperidin impacts liver health by examining its influence on inflammation, liver enzymes, lipid profile and blood sugar regulation, all of which are intricately linked to liver function.
Methods and materials
This narrative review is based on peer-reviewed articles. PubMed, Web of Science and Science Direct databases were used. Only animal studies were included in this review, covering the years between 2014 and 2024. The following keywords were used: ‘hesperidin’ OR ‘hesperetin 7-O-rutinoside’ OR ‘hesperetin’ OR ‘hesperetin 7-O-glucoside’ AND ‘NAFLD’ OR ‘non-alcoholic fatty liver disease’ OR ‘liver fibrosis’ OR ‘NASH’ OR ‘fatty liver’ OR ‘non-alcoholic steatohepatitis’. The authors checked all parts of the article separately. In case of disagreement, the authors discussed for a common decision. As a result of this process, fourteen were identified (Table 2). Apart from these, some human studies related to the topic are also presented in Table 3.
Table 1. Sources of hesperidin

Table 2. Effects of hesperidin and hesperetin on liver diseases

MASLD, metabolic dysfunction-associated steatotic liver disease; HFD, high-fat diet; LFD, low-fat diet; TC, total cholesterol; TG, triacylglycerol; LDL, low-density lipoprotein; HDL, high-density lipoprotein; THP-1, the human leukaemia cell line-1; ALT, alanine aminotransferase; AST, aspartate aminotransferase; HD-16, hesperetin derivative-16; CCL4, carbon tetrachloride-4; LDH, lactate dehydrogenase; ALP, alkaline phosphatase; GGT, gamma-glutamyl transferase; TGF-β1, transforming growth factor beta 1; IL-6, interleukin-6; SIRT3, sirtuin 3.
Table 3. Randomised controlled studies of hesperidin and its effects on some diseases linked with MASLD
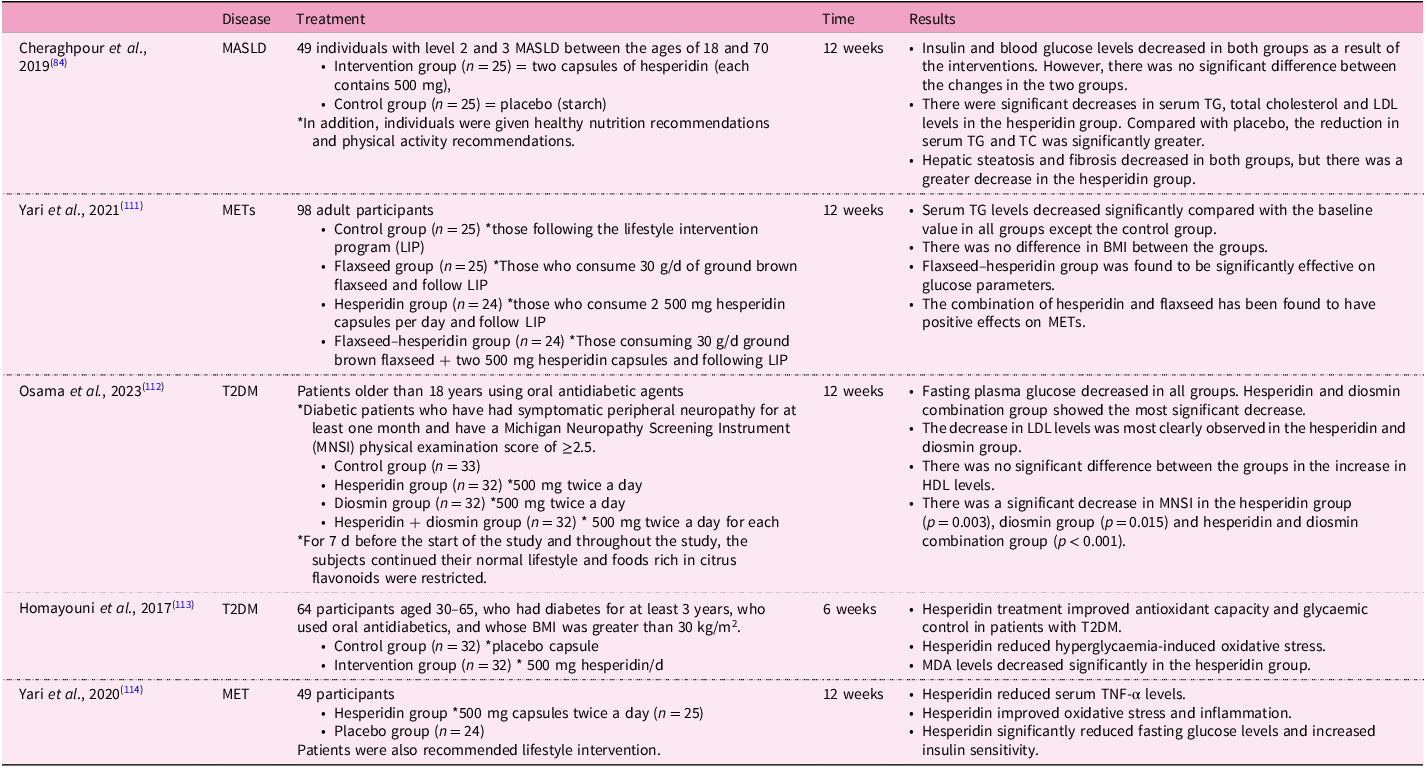
MASLD, metabolic dysfunction-associated steatotic liver disease; MET, metabolic syndrome; T2DM, type 2 diabetes; TC, total cholesterol; TG, triacylglycerol; LDL, low-density lipoprotein; HDL, high-density lipoprotein; BMI, body mass index; MDA, malondialdehyde; TNF-α, tumour necrosis factor-α.
Definition of hesperidin and hesperetin
Flavonoids are a group of polyphenolic structures present in citrus fruits, and they are divided into four groups: flavones, flavanols, flavanones and flavanols. Flavanones are predominantly present in citrus fruits such as oranges, grapefruits and lemons. Therefore, they are sometimes also referred to as citrus flavonoids or bioflavonoids(Reference Najmanová, Vopršalová and Saso11).
The origin of the name hesperidin is based on the word ‘hesperidium’ used for citrus tree fruits(Reference Stanisic, Liu and dos Santos12). Hesperidin is found in different concentrations in various foods(Reference Tayal, Munjal and Gauttam13). Hesperidin is detected in citrus fruits, particularly in the peel parts such as zest and albedo, in larger amounts compared with seeds and juicy cells(Reference Roohbakhsh, Parhiz and Soltani4). The predominant type of flavanone found in citrus fruits, HES, and its aglycone form HST are particularly abundant in sweet unripe oranges (Citrus sinensis) at high levels (1–2%)(Reference Wdowiak, Walkowiak and Pietrzak14). Hesperetin has similar values to hesperidin in plants because it is a metabolite of hesperidin. It is found in higher proportions in unripe fruits compared with ripe fruits. HES is also a bioflavonoid(Reference Roohbakhsh, Parhiz and Soltani4).
Chemical properties of hesperidin
Hesperidin (hesperetin-7-rutinoside) is a polyphenolic compound of plant origin. Although it belongs to the flavonoid group, it is more specifically a flavanone(Reference Imperatrice, Cuijpers and Troost15). Hesperidin is a flavanone glycoside that has an aglycone portion called hesperetin and a sugar portion called rutinoside (shown in Fig.1). In addition, hesperidin can be enzymatically converted to the aglycone hesperetin(Reference Wdowiak, Walkowiak and Pietrzak14).

Figure 1. Chemical structures of hesperidin and hesperetin. This figure was created by PubChem.
Hesperidin is abundant in citrus fruits such as lemon, lime, tangerine, orange and grapefruit and is the most dominant flavonoid form in citrus fruits (Rutaceae family)(Reference Choi, Lee and Lee5,Reference Yamamoto, Jokura and Hashizume16) . Pure hesperidin is a tasteless and odourless molecule that occurs as long hair-like needles that are skin-coloured or light yellow. Its melting point is between 258°C and 262°C. Its molecular formula is C28H34O15 and its molecular weight is 610.57 Da. It is slightly soluble in methanol and almost insoluble in chloroform, acetone and benzene(Reference Calomme, Pieters and Vlietinck17). However, it is easily soluble in pyridine. Since it can form complex crystals with other glycosides of similar structure, its solubility and other physical properties are significantly affected. Therefore, it is not easy to obtain in pure form. For purification, it must be washed with hot water, extracted with 95% methyl alcohol and then crystallised.(Reference Garg, Garg and Zaneveld18).
Glucosyl hesperidin (G-hes) is water soluble, being 10 000 times more soluble in water than hesperidin alone. In addition, it is absorbed much faster and more effectively(Reference Yamada, Tanabe and Arai19). This form, G-hes, was synthesised by Hijiya(Reference Hijiya HaM20) and colleagues using regioselective transglucosylation with cyclodextrin glucanotransferase from Bacillus stearothermophilus (Reference Yamada, Tanabe and Arai19,Reference Sugasawa, Katagi and Kurobe21) . G-hes and hesperidin exhibit similar effects(Reference Ohtsuki, Abe and Mitsuzumi22). For example, in one study, when HES and G-hes were administered to mice at equal doses, similar improvements were observed in serum cholesterol profiles(Reference Yoshida, Tsuhako and Sugita23). In another study, G-hes was found to have anti-obesity and antidiabetic effects. G-hes can easily convert to hesperidin via the α-glucosidase enzyme(Reference Yamada, Tanabe and Arai19).
Hesperidin can be easily isolated from products of citrus processing, making it economical. Conversely, in industry, hesperetin can be produced using bacterial enzymes to modify hesperidin. This results in a more complex aglycone (hesperetin) production process than obtaining hesperidin from the outset, thereby incurring production costs(Reference Wdowiak, Walkowiak and Pietrzak14).
Sources of hesperidin
Apart from citrus fruits, hesperidin has been detected in many different plant genera, including within the families Fabaceae and Betulaceae(Reference Garg, Garg and Zaneveld18). Hesperidin concentrations in some plant parts are summarised in Table 1.
The Mediterranean diet is also a high source of hesperidin and hesperetin. It is a diet rich in vegetables and fruits that is frequently recommended for patients with chronic diseases(Reference Mayr, Tierney and Kucianski24). Therefore, it is very rich in polyphenols, especially citrus bioflavonoids(Reference Guasch-Ferré, Merino and Sun25). Following consumption of the Mediterranean diet, an increase in the plasma levels of hesperidin, hesperetin, naringin and routine flavonoids was observed(Reference Al-Aubaidy, Dayan and Deseo26).
Stability of hesperidin
Majumdar et al. found that the concentrations of hesperidin aqueous solutions did not change for 2 months in the pH range between 1 and 7.4 in samples, unlike for samples with pH 9(Reference Majumdar and Srirangam27). The degradation rate constants, most likely by alkaline hydrolysis, were 0.03 and 23 at pH 9 at 25°C and 40°C, respectively. This indicates hesperidin can undergo alkaline hydrolysis when exposed to elevated pH levels and higher temperatures.
Zhang and colleagues studied how storage conditions and heat processing during pasteurisation affect hesperidin levels in orange juice(Reference Zhang, Ling and Yan28). Although hesperidin levels decreased at both room temperature and 4°C, the decrease was faster at room temperature. The study concluded that, as the storage time of orange juice increases, hesperidin could precipitate, leading to a decrease in its concentration. It is noted that the decomposition of vitamin C may be responsible for the precipitation of hesperidin as the storage time prolongs.
A study on the stability of hesperidin was carried out by Bisega et al. Honey, onion and apple samples were used in the study(Reference Biesaga and Pyrzyńska29). The apple matrix largely stabilised these compounds, while the highest degradation occurred in the honey samples. Therefore, it was concluded that hesperidin degradation may also be related to the food matrix.
Metabolism of hesperidin
Absorption and transport of hesperidin
The absorption and metabolism of citrus flavonoids in the intestine vary depending on their chemical structure; This significantly affects their bioactivity(Reference Wang, Zhao and Wen30). The presence of polyphenols in the form of glycosides, where one or more sugar moieties are attached, contributes to their variable bioavailability(Reference Actis-Goretta, Dew and Lévèques6).
Hesperidin is a β-glucoside, whereas hesperetin is its aglycone form(Reference Wdowiak, Walkowiak and Pietrzak14). Absorption of glycosides and aglycones is different. The sugar residues markedly influence the absorption because free aglycones can be absorbed in the stomach, whereas the entire glycoside may not be absorbed(Reference Najmanová, Vopršalová and Saso11). Flavonoid aglycones such as hesperetin are moderately hydrophobic and are relatively easier and faster to absorb from the lumen compared with flavonoid glycosides (hesperidin)(Reference Wang, Zhao and Wen30). In studies in which hesperetin and hesperidin were consumed by healthy human subjects, the peak concentration in plasma occurred 4 h after consuming hesperetin, whereas for hesperidin-rich juices, the peak concentration was achieved after 7 h. This indicates faster absorption of hesperetin(Reference Nielsen, Chee and Poulsen31,Reference Kanaze, Bounartzi and Georgarakis32) .
The main absorption sites for flavanones are the small intestine and colon. In a study where naringenin was injected into different parts of the rat intestines to observe flavanone absorption, the absorption rates of naringenin were found to be similar in the duodenum (47%), jejunum (39%) and ileum (42%). The colon had the highest absorption rate (68%)(Reference Xu, Kulkarni and Singh33).
The non-detection of hesperidin in human plasma or urine after oral consumption of hesperidin-rich orange juice supports the idea that hesperidin is broken down to hesperetin and related metabolites during absorption(Reference Matsumoto, Ikoma and Sugiura34).
The reason that most ingested hesperidin is not hydrolysed by small-intestinal β-glucosidases is due to the presence of a routine component that requires its metabolism and absorption in the colon(Reference Borges, Lean and Roberts35). It has been suggested that the rutinose molecule, hesperetin-7-rutinoside, is hydrolysed by the intestinal microbiota in the large intestine(Reference Actis-Goretta, Dew and Lévèques6). Hesperidin is absorbed in the colon via the microbiota, where it is converted into the aglycone form, hesperetin. This is then metabolised and hydrolysed(Reference Pereira-Caro, Fernández-Quirós and Ludwig36).
Summary of studies in the literature indicate that hesperidin, also known as hesperidin-7-rutinoside, when orally consumed by humans or animals, is absorbed either by removal of the rutinoside portion to convert it to the aglycone (hesperetin) form or by conversion of hesperidin to hesperetin 7-glucoside by bacterial enzymes (β-glucosidases) produced by enterobacteria in the intestine(Reference Matsumoto, Ikoma and Sugiura34,Reference Ávila-Gálvez, Giménez-Bastida and González-Sarrías37) . It is believed that, after absorption, it is converted into glucuronidated and sulphated metabolites and released into the bloodstream (shown in Fig.2)(Reference Choi, Lee and Lee5,Reference Aschoff, Riedl and Cooperstone38) . Following the cleavage of hesperidin to hesperetin, the passage of aglycone hesperetin into enterocytes occurs via proton-dependent active transport and passive diffusion(Reference Najmanová, Vopršalová and Saso11).

Figure 2. Absorption pathways of hesperidin and hesperetin. This figure was created with BioRender.com. The aglycone form, hesperetin, is absorbed more easily than hesperidin. Hesperidin is first converted to hesperetin with the help of lactase-phlorizin hydrolase or absorbed into enterocytes via SGLT-1. LPH, lactase-phlorizin hydrolase; SGLT1, sodium/glucose cotransporter 1; HPT3G, hesperetin-3-O-β-d-glucuronide; HPT7G, hesperetin-7-O-β-d-glucuronide.
There are two hypotheses regarding how flavonoid glycosides are absorbed in the small intestine(Reference Manach, Scalbert and Morand39) Firstly, the glycoside may undergo hydrolysis by lactase-phlorizin hydrolase, after which the free aglycone diffuses passively or with facilitation through the epithelial cells. Secondly, the glycoside molecule might be transported into enterocytes via SGLT1 carrier and subsequently glycosylated by β-glucosidase enzymes found in intestinal cells. Both ways lead to intracellular aglycones that are conjugated to glucuronides or sulphates(Reference Nielsen, Chee and Poulsen31).
In a study conducted with human subjects, individuals were orally given low-dose orange juice containing hesperidin, high-dose orange juice containing hesperidin and orange juice with added hesperidinase, thereby containing hesperetin-7-glucoside(Reference Nielsen, Chee and Poulsen31). Concentration of hesperetin-7-glucoside was measured in plasma and urine. Subjects consuming orange juices containing low and high doses of hesperidin achieved similar plasma peak concentrations at approximately 7 h. However, in the group consuming orange juice containing hesperidinase, the peak concentration was achieved after 4 h. This finding supports the notion that flavonoids with rutinoside groups are absorbed following hydrolysis by colonic microflora(Reference Nielsen, Chee and Poulsen31). An animal study showed that the disaccharide portion of hesperidin was removed by intestinal bacteria and absorbed as hesperetin in the intestine. This study demonstrated that hesperetin undergoes immediate glucuronidation in the intestinal epithelium, converting it into hesperetin glucuronides(Reference Garg, Garg and Zaneveld18). Matsumoto et al.(Reference Matsumoto, Ikoma and Sugiura34) showed that, after oral intake of hesperidin, it is converted into hesperetin glucuronides in the intestinal epithelium and then reaches the liver. This molecule undergoes deglucuronidation, demethylation, remethylation and reglucuronidation in the liver, leading to the release of hesperetin conjugates such as hesperetin-7-O-β-d-glucuronide (HPT7G) and hesperetin-3-O-β-d-glucuronide (HPT3G), as well as homoeriodictyol conjugates into the plasma. It was also reported in this study that HPT7G was found in higher amounts than HPT3G. In another animal study, the influence of hesperidin metabolites on endothelial function and blood pressure was investigated, and intravenous administration of HPT7G reduced blood pressure similarly to hesperetin, exhibiting vasodilatory and anti-inflammatory effects. Meanwhile, HPT3G had little effect on these parameters(Reference Choi, Lee and Lee5,Reference Yamamoto, Jokura and Hashizume16) .
Numerous studies indicate that, after oral consumption, hesperidin is hydrolysed to its aglycone form by β-glucosidases and then converted to glucuronides and sulphates in the colon. Subsequently, absorbed hesperetin undergoes immediate metabolism into glucuronide and sulphate conjugates in the intestinal epithelium and liver(Reference Choi, Lee and Lee5,Reference Yamada, Tanabe and Arai19,Reference Matsumoto, Ikoma and Sugiura34) . Three hours after intake, hesperetin appears in the plasma primarily as glucuronides, accounting for 87% of the total. The remaining portion consists of sulfoglucuronides. It reaches peak concentration between 5 and 7 h(Reference Pla-Pagà, Companys and Calderón-Pérez40).
However, hesperidin exhibits low solubility in water (0.01%) and limited bioavailability(Reference Ávila-Gálvez, Giménez-Bastida and González-Sarrías37,Reference Manach, Morand and Gil-Izquierdo41,Reference Vallejo, Larrosa and Escudero42) . Different methods such as micronisation and encapsulation have been proposed, especially in pharmaceutical production. With these methods, it is possible to increase the bioavailability and stability of hesperidin(Reference Vallejo, Larrosa and Escudero42,Reference Crescenti, Caimari and Alcaide-Hidalgo43) .
Bioavailability of hesperidin
The bioavailability of polyphenols varies significantly. One of the primary factors influencing human absorption is the glycoside structure of the polyphenols present in plants. This glycoside structure, in which polyphenols are attached to one or more sugar molecules, is considered one of the primary factors determining the absorption level in humans. The general bioavailability of hesperidin, also known as hesperetin-7-O-rutinoside, is comparatively low owing to a rutinoside molecule in its structure(Reference Actis-Goretta, Dew and Lévèques6). In a study aimed at increasing the bioavailability of hesperidin, the rutinoside molecule was enzymatically removed from hesperidin under in vitro conditions, resulting in the production of hesperetin-7-O-glucoside. The study reported that the bioavailability of hesperetin was approximately tripled(Reference Nielsen, Chee and Poulsen31).
In another study conducted to screen and identify metabolites of hesperidin and hesperetin, and to determine similarities and differences in pharmacodynamics and pharmacokinetics, Sprague-Dawley rats were orally administered equal amounts of hesperidin and hesperetin. It was shown that the bioavailability of hesperetin exceeded that of hesperidin(Reference Jiao, Xu and Jiang44).
Examining the factors associated with hesperidin and MASLD
Effects on inflammation – possible mechanisms
The combined causes of MASLD include a sedentary life and a high-calorie diet that also causes obesity, environmental factors, genetic elements, and physiological and metabolic factors. Consumption of Western-style diets, particularly elevated intake of simple sugars and saturated fats, obesity, hyperlipaemia and insulin resistance increase the prevalence of MASLD. Lifestyle modifications for the management of MASLD and comorbid diseases and elimination of the underlying causes of the disease are the issues that the scientific perspective focuses on(Reference Hassani Zadeh, Mansoori and Hosseinzadeh45–Reference Morshedzadeh, Ramezani Ahmadi and Behrouz47).
Despite the complex and uncertain pathogenesis of MASH, oxidative stress is widely known as a main reason. In healthy liver tissue, there exists a dynamic balance between oxidation and antioxidant systems. Hepatocytes have the ability to effectively neutralise ROS by using antioxidant mechanisms(Reference Li, Wang and Liu48).
In MASH, ROS production in mitochondria increases and the activity of ROS scavenging mechanisms decreases, leading to ROS overproduction and oxidative stress, resulting in the formation of toxic lipid peroxides 4-hydroxy-2-nonenal (4-HNE) and malondialdehyde (MDA)(Reference Serviddio, Bellanti and Vendemiale49). Consequently, the accumulation of ROS and lipid peroxides negatively affects protein production following mitochondrial dysfunction. Protein carbonylation and destruction of phospholipid membranes result in hepatocyte damage and apoptosis. In hepatocytes, overaccumulation of ROS can activate the nuclear factor-κB (NF-κB)-mediated inflammatory pathway. NF-κB is a transcription factor that regulates liver inflammation and modulates the inflammation–fibrosis–cancer axis in liver disorders. Chemokines and cytokines increase with NF-κB increase (shown in Fig.3). Activation of hepatic stellate cells and Kupffer cells plays an important role in the development of liver fibrosis. This activation may be mediated by the release of inflammatory cytokines(Reference Lee, Park and Roh50,Reference Liu, Baker and Bhatia51) . Chronic inflammation and subsequent continuous increase in NF-κB and cytokines may also trigger hepatic carcinogenesis (shown in Fig.3)(Reference Goldberg and Schwertfeger52). There are studies in the literature showing that the phytochemical hesperidin is effective in stopping cell growth and preventing apoptosis by inhibiting NF-κB(Reference Lee, Yeh and Kao53,Reference Ghorbani, Nazari and Jeddi-Tehrani54) . In addition, in a study, rats with carbon tetrachloride (CCL4)-induced liver cirrhosis significantly reduced MDA levels after hesperidin treatment, indicating its antioxidant effect. In this study, the levels of NF-κB increased 11-fold in rats with CCL4-induced liver cirrhosis compared with the control group, but administration of hesperidin treatment inhibited NF-κB and transforming growth factor beta 1 (TGF-β) expression. Interestingly, in this study, administering hesperidin to non-cirrhotic normal rats lowered NF-κB levels to a level below the control values(Reference Pérez-Vargas, Zarco and Shibayama55).

Figure 3. Summary of the effect of hesperidin on inflammatory pathways. This figure was created with BioRender.com. HES, hesperidin; ER, endoplasmic reticulum; ROS, reactive oxygen species; MDA, malondialdehyde; 4-HNE, 4-hydroxy-2-nonenal; NF-κB, nuclear factor-κB; TNF-α, tumour necrosis factor alpha; IL-6, interleukin-6; IL-1β, interleukin-1β; CCL2, chemokine ligand 2; Nrf2, nuclear factor erythroid 2-related factor.
In another study, rats with liver cancer induced by diethyl nitrosamine and CCL4 were treated with hesperidin, and MDA levels decreased significantly(Reference Mahmoud, Mohammed and Khadrawy56). The levels of TNF-α, TGF-β1 and Smad3 in these rats also decreased significantly. In addition, very low levels of collagen accumulation were observed in the groups receiving hesperidin treatment. Consistently, in another study, hesperidin treatment showed a protective effect against ethanol-induced damage by reducing MDA levels(Reference El-Sisi, Sokar and Shebl57).
In summary, activated Kupffer cells increase liver damage by generating several metabolites that lead to cellular injury. Some of these metabolites are superoxide radicals and pro-inflammatory cytokines(Reference Chen, Ding and Li58). Eventually, this condition evolves from a simple fatty liver disease to NASH. Therefore, controlling oxidative stress and excessive ROS production in the liver is a critical strategy to prevent NASH(Reference Li, Wang and Liu48).
Nuclear factor erythroid 2-related factor 2 (Nrf2) is a protein which has a vital role in antioxidant system and controls the transcription of various antioxidant genes, providing protection from oxidative damage induced by inflammation(Reference Itoh, Chiba and Takahashi59). Nrf2 is the main controller of antioxidant stress systems. The Nrf2/ARE signalling pathway plays a significant role in combatting continuous oxidative stress in cellular defence, regulating the expression of antioxidant genes. When oxidative stress increases, Nrf2 moves to the nucleus and attaches to the antioxidant response element (ARE). This starts the down-regulation of antioxidant gene transcription, resulting in a decrease in intracellular ROS content and inhibition of oxidative stress(Reference Aleksunes and Manautou60). One study showed that Nrf2 deficiency leads to NF-κB activation and increases oxidative stress, thereby worsening liver steatosis and injury, and then leads to fibrosis in mice with MASLD(Reference Liu, Dou and Ni61). Other studies have also shown that hesperidin has an anticarcinogenic effect by activating the Nrf2/ARE/HO-1 signalling pathway(Reference Mahmoud, Mohammed and Khadrawy56,Reference Chen, Gu and Ye62,Reference Subramanian, Anandan and Jayapalan63) . Increasing evidence suggests that the activation of Nrf2 may protect hepatocytes from oxidative stress and, thus, lipotoxicity, thereby attenuating MASLD. Considering these aspects, Nrf2 has been considered as a new focus to reduce oxidative stress in the liver and treat the disease(Reference Li, Wang and Liu48).
One of the important pathways in NF-κB activation is activated by endoplasmic reticulum (ER) stress(Reference Di Conza and Ho64). The ER regulates protein synthesis, proper folding and post-translational modification. It is susceptible to the build-up of unfolded or misfolded proteins in its lumen, disrupting ER homeostasis. If the accumulation of these unproper protein cargo exceeds the ER’s folding capacity, the unfolded protein response (UPR) is triggered(Reference Han, Back and Hur65). One of the main pathways regulated by ER membrane receptors during UPR is the pancreatic ER kinase-like ER kinase (PERK) pathway. Under ER stress, PERK phosphorylates the α subunit of eukaryotic translation initiation factor-2, which in turn activates the NF-κB pathway. Subsequently, activated NF-κB increases the synthesis of TNF-α and IL-6(Reference Bi, Naczki and Koritzinsky66,Reference Yoshida, Matsui and Yamamoto67) . One study showed that hesperidin could attenuate liver ER stress in NEFA-stimulated human THP-1 cells(Reference Xie, Gao and Lei68).
Hepatic fibrosis, which represents a response to the chronicity of liver injury, is marked by the deposition of extracellular matrix particularly α-SMA, collagen I. Permanent fibrosis usually results in cirrhosis or liver failure(Reference Chen, Li and Chen69,Reference Jung and Yim70) . Activation of hepatic stellate cell is one of the key factors responsible for the accumulation of extracellular matrix. The persistent activation of hepatic stellate cells results in the continuous production of inflammatory cytokines, such as TGF-β1, and the development of liver fibrosis. Inhibiting this activation appears to be an effective therapeutic strategy to prevent liver fibrosis. The adenosine 5-monophosphate activated protein kinase (AMPK) pathway is associated with fibrosis of liver(Reference Yang, Yang and Zhang71). AMPK inactivation increases hepatic fibrogenesis. Sensitivity to AMPK phosphorylation reduces liver fibrosis. SIRT3, a regulator belonging to the histone deacetylase family, is located in mitochondria. It contributes to homeostasis and stress response, which are some of the physiological activities of the cell. With the up-regulation of AMPK/SIRT3 signalling, mitochondrial function can improve and the progression of the disease slows down(Reference Han, Li and Li72,Reference Wang, Li and Gu73) . In one study, mice with CCl4-induced liver fibrosis were given hesperetin-16 (HD-16), an increased water-soluble form of hesperetin, and hesperetin-16 reduced inflammation and fibrogenesis in the liver by moderating the AMPK/SIRT3 pathway. In the same study, HD-16 treatment of TGF-β-activated LX-2 cells decreased the expression of genes involved in fibrogenesis, indicating its antifibrotic effect(Reference Li, Jiang and Wang74).
Another study focusing on fibrosis of liver highlights that one of the significant factors leading to fibrosis is the Hedgehog signalling pathway(Reference Yang, Tao and Li75). It is suggested that activation of the Hedgehog-GLIoma pathway increases the transformation of quiescent stellate cells into fibrogenic myofibroblasts. This pathway is associated with several cellular responses, including proliferation, differentiation and cell viability. Abnormal activation of this pathway is often shown by the up-regulation of Gli-1 and plays a role in liver cancer(Reference Kim, Cho and Hong76). In this study, the mRNA levels of fibrotic genes decreased in hepatic stellate cells isolated from mice treated with a derivative of hesperetin compared to hepatic fibrosis mice(Reference Chen, Li and Chen77). These findings indicate that hesperetin interferes with the onset of liver fibrogenesis in mice. In addition, it has been shown that Gli-1 levels in hepatic stellate cells isolated from mice treated with hesperetin decreased compared with hepatic fibrosis mice(Reference Chen, Li and Chen77,Reference Iredale, Benyon and Pickering78) .
Sarcopenia is a severe condition in the elderly, marked by muscle loss and increased inflammation, including elevated pro-inflammatory cytokines and ROS(Reference Tarantino, Sinatti and Citro79). One of the main elements of the disease is the increase in inflammation and, thus, the increase in pro-inflammatory cytokines and ROS. A study investigating the link between sarcopenia and inflammation found that daily administration of hesperidin to animals for 8 weeks improved muscle strength in older mice(Reference Oh, Jin and Lee80). At the end of the study, the muscle fibres and strength of elderly mice treated with hesperidin increased. This result was achieved by improving the M1/M2 macrophage imbalance through the anti-inflammatory properties of hesperidin. Moreover, hesperidin reduced muscle protein degradation, increased protein synthesis and promoted myogenesis, leading to stronger muscle fibres. In addition, there are shared mechanisms involved in developing sarcopenia and MASLD, and the two may coexist in the elderly. In one study, people with MASLD were found to have consistently lower muscle mass than healthy people over a 12-year period(Reference Lee, Lee and Lee81).
The impact of hesperidin on lipid profile and liver enzymes
We mentioned above that there is no definitive treatment method for MASLD. Lifestyle change is still considered the most current treatment approach(Reference Mokhtari, Gibson and Hekmatdoost82). In one study, an in vitro model of lipid accumulation related to NEFA was created, and this cell line was treated with hesperidin. There was a decrease in lipid accumulation in the cells(Reference Tang, Wang and Shi83). In a study with human subjects with MASLD, there were positive effects of dietary intervention and physical activity on the treatment of the disease. In addition to these interventions, the group receiving hesperidin supplementation showed significantly greater reductions in liver enzyme levels, serum triacylglycerol (TG) and total cholesterol levels, and hepatic steatosis and fibrosis amounts compared with the control group(Reference Cheraghpour, Imani and Ommi84). In an animal study, hesperidin treatment improved serum TG levels in a MASLD mouse(Reference Sukkasem, Chatuphonprasert and Jarukamjorn85). In another animal study, high-dose hesperidin lowered serum Alanine transaminase (ALT), Aspartate transaminase (AST) and total and direct bilirubin levels, while low-dose hesperidin had no effect on liver enzymes(Reference Kong, Wang and Luo86). There are promising studies in the literature regarding the effect of hesperidin on blood lipids. For example, in a randomised controlled trial conducted with obese individuals, hesperidin intervention was added to calorie restriction. The hesperidin intervention did not affect body weight when combined with calorie restriction, but it did lead to notable decreases in LDL-cholesterol and total cholesterol levels. Liver enzymes (ALT, AST, Alkaline phosphatase (ALP), and Gamma-glutamyl transferase (GGT)) decreased in both groups compared with baseline, but there was no significant difference between the two groups. Total antioxidant capacity (TAC) and MDA levels were also evaluated in this study. The TAC and MDA levels of both groups decreased compared with baseline, but hesperidin intervention did not cause a greater decrease than the calorie-restricted group(Reference Ribeiro, Dourado and Cesar87). A randomised controlled study showed that G-hes decreased serum total cholesterol levels(Reference Katada, Oishi and Yanagawa88).
In an animal study, pre-treatment with hesperidin was protective against the toxic effects of cadmium and ameliorated the impaired lipid profile caused by cadmium. Hesperidin increased HDL-cholesterol levels while decreasing LDL-cholesterol and total cholesterol levels(Reference Aja, Ekpono and Awoke89). However, whether the hesperidin treatment impacts the lipid profile is controversial. In an animal study, although hesperidin reduced the appearance of steatosis the liver, it did not affect the lipids(Reference Xie, Gao and Lei68).
Hesperidin intervention can reduce fat accumulation in the liver. In one study, hesperidin prevented the increase of lipid deposition in the liver. In addition, due to a selective enrichment of a group of intestinal bacteria such as Bacteroidota proven to improve obesity and metabolic syndrome symptoms, and the promotion of arginine synthesis by these bacteria, hesperidin is considered a therapeutic agent for MASLD(Reference Li, Yao and Wang90).
The effects of hesperidin on plasma glucose
Although its frequency is increasing day by day and it has a significant prevalence in the world, the pathogenesis is not completely understood(Reference Riazi, Azhari and Charette91). Thus, MASLD has become an important public health problem within the scope of metabolism and liver disorders. MASLD, a multisystem metabolic disease, is often accompanied by type 2 diabetes (T2DM) and metabolic syndrome (MET), which together promote disease progression(Reference Rong, Zou and Ran92). The liver has a fundamental role in lipogenesis and gluconeogenesis, including cholesterol metabolism. Metabolic dysfunctions in the diseased liver can lead to various pathological conditions. The increasing prevalence of obesity, T2DM and MET promotes the pathophysiological changes that lead to MASLD. MASLD is thought to be the most common liver disorder in western communities(Reference Feldstein93). Changes in insulin response associated with obesity, metabolic syndrome and diabetes can lead to progression of liver damage due to imbalance in β-oxidation, lipid metabolism and autophagy. Therefore, these diseases are thought to be interconnected(Reference Bechmann, Hannivoort and Gerken94). For instance, it is known that, in the presence of diabetes, increased glucose auto-oxidation and protein glycation lead to increased formation of free radicals. The increase in free radicals also leads to an increase in lipid peroxidation(Reference Kumawat, Sharma and Singh95). The accumulation of MDA leads to permanent damage in hepatocytes. The contribution of the accumulation of lipid peroxidation products in liver damage is inevitable. Therefore, diabetes and liver diseases can trigger each other’s occurrence directly or indirectly(Reference Li, Wang and Liu48,Reference Dokumacioglu, Iskender and Musmul96) .
Disruptions exceeding a certain limit in glucose metabolism can lead to MASLD; thus, there is an interconnection between the disorder, glucose homeostasis and lipid metabolism. Some studies in the literature indicate that hesperidin can positively affect glucose metabolism and insulin activity(Reference Ribeiro, Dourado and Cesar87,Reference Jung, Lee and Jeong97) . In one animal study, elevated blood glucose and insulin levels were reduced with hesperidin treatment. In the same study, a group of mice was given glucose intraperitoneally followed by hesperidin administration. Hesperidin is thought to reduce increased blood sugar levels and thereby reduce glucose tolerance(Reference Nie, Wang and Li98,Reference Ahmed, Mahmoud and Abdel-Moneim99) .
Hesperidin is thought to regulate blood glucose levels by modulating enzymes in glucose metabolism such as glucose-6-phosphatase. In one study, administration of hesperidin to mice reduced the levels of glucose-6-phosphate dehydrogenase and fatty acid synthase enzymes. In addition, after hesperidin treatment, significant increases in glucokinase mRNA levels were noted. In this study, hesperidin caused a significant decrease in hepatic GLUT2 expression while increasing adipocyte GLUT4 levels compared with the control group(Reference Jung, Lee and Park100). Other studies suggest that another effect of hesperidin on glucose metabolism may occur via glucose transporters(Reference Kerimi, Gauer and Crabbe101,Reference Shen, Xu and Lu102) .
Conclusion
Hesperidin and hesperetin are being investigated as promising treatments for many diseases. They are thought to play an effective role in inflammatory processes, particularly in liver diseases, but further studies are needed to determine the optimum dosage. In addition, their low water solubility limits their potential benefits. We believe that deriving water-soluble forms could enhance their beneficial effects. Although we consider them as promising agents in preventing and treating liver diseases, especially due to their anti-inflammatory and lipid-lowering effects, it is important to note that more research is required. Based on their effects in in vivo and in vitro studies, we must emphasise the necessity for further investigation.
Author contributions
A.S: methodology, investigation, writing, visualisation; Z.G: conceptual design, methodology, review & editing, supervision.
The authors have reviewed and approved the final version of the manuscript.
Financial support
None.
Competing interests
The authors declare none.