Introduction
Argentina is the world’s third largest soybean producer following Brazil and the United States, and the fourth largest corn producer following United States, China, and Brazil (CAPECO 2020). Since the introduction of glyphosate-resistant (GR) cultivars in 1996, the area in Argentina planted with soybean increased at a rate of nearly 1 million hectares per year during the first 15 years. By 2003, nearly all soybean in Argentina was planted with GR cultivars (Ministry of Agriculture 2020; ArgenBio 2020). The adoption of transgenic GR soybean co-evolved with the adoption of no-till production systems that are now used on more than 90% of the cropped area in the country (AAPRESID 2020). The adoption of GR soybean in no-till systems has inevitably led to a significant increase in the use of herbicides such as glyphosate to control weeds during fallow periods (chemical fallow). Beyond the benefits of soil erosion, energy use and soil water use efficiency in a no-till system, the over-reliance on herbicides to control weeds and low adoption of other agronomic practices such as increased crop seeding rates or cover crops, has led to the selection of herbicide resistant weed biotypes (Scursoni et al. Reference Scursoni, Duarte Vera, Oreja, Kruk and de la Fuente2019).
Currently, the primary herbicide-resistant weed species in Argentina are smooth pigweed and Palmer amaranth (Amaranthus palmeri S. Wats.). Both have spread quickly and currently occur on more than 20 million hectares (REM 2021). The first report of herbicide resistance in Argentina was that of smooth pigweed (Amaranthus hybridus L.) to acetolactate synthase (ALS)-inhibiting herbicides (Tuesca and Nisenshon Reference Tuesca and Nisensohn2001). A few years later, johnsongrass [Sorghum halepense (L.) Pers.] was the first GR biotype documented (Vila-Aiub et al. Reference Vila-Aiub, Balbi, Gundel, Ghersa and Powles2007). Currently, 40 biotypes of 23 weed species have evolved resistance to four different herbicide sites of action in cropping systems in Argentina. Globally, smooth pigweed has evolved resistance to herbicides with five different sites of action, with numerous cases of multiple herbicide resistance in several countries (Heap Reference Heap2021). In Argentina, smooth pigweed biotypes have evolved resistance to ALS-inhibiting herbicides; glyphosate; ALS-inhibitors and glyphosate; glyphosate, 2,4-D and dicamba; and 2,4-D and dicamba (AAPRESID 2021). Interestingly, resistance to auxin herbicides in smooth pigweed has been identified only in Argentina.
Smooth pigweed is a very competitive weed that can potentially reduce crop yields by up to 90% with plant densities higher than 30 plants m− 2 (Costea et al. Reference Costea, Weaver and Tardif2003). Vitta et al. (Reference Vitta, Faccini and Nisensohn2000) recorded 20% soybean yield loss with an initial weed cover of 20%. Weed-crop competition models (Cousens Reference Cousens1985) predict approximately 60% soybean yield loss with smooth pigweed densities of 10 plants m−2.
The adoption of no-till systems has favored the infestation of small-seeded weeds such as smooth pigweed, horseweed, barnyardgrass [Echinochloa crus-galli; (L.) P. Beauv.] and goosegrass [Eleusine indica (L). Gaertn] due to favorable conditions for rapid emergence from the soil surface or subsurface (Buhler Reference Buhler1992; Cardina et al. Reference Cardina, Regnier and Harrison1991; Chauhan and Johnson Reference Chauhan and Johnson2008, Reference Chauhan and Johnson2009; Faccini and Vitta Reference Faccini and Vitta2007; Wu et al. Reference Wu, Walker, Rollin, Tan and Werth2007). The increase in smooth pigweed in Argentinian production areas is a serious problem for farmers because of the increasing reports of poor herbicide activity. In recent years, inquiries regarding the presence of herbicide resistance in smooth pigweed accessions have been frequent. Therefore, the objective of this research was to quantify the responses of 50 smooth pigweed accessions, distributed across a wide geographic area in Argentina, to dicamba and 2,4-D (growth regulator herbicides), topramezone (a 4-hydroxphenylpyruvate dioxygenase [HPPD] inhibitor), fomesafen (a protoporphyrinogen oxidase [PPO] inhibitor), and glyphosate (a 5-enolpyruvylshikimate-3-phosphate synthase [EPSPS] inhibitor).
Materials and Methods
Plant Material
Smooth pigweed seed material was collected from 50 crop fields during February and April 2018. Seeds were collected from soybean production fields in which smooth pigweed plants were observed prior to crop harvest. In each field site, smooth pigweed mature inflorescences were randomly collected from an average of 50 individuals. Inflorescences were then hand-threshed to obtain seeds of each accession. The seed collection sites encompassed the main soybean crop production areas of Argentina representing around 20 million hectares, much of which has historically been grown in a soybean monoculture (Figure 1). However, over the last few years other crops such as corn and wheat, have been introduced into the rotation.

Figure 1. Distribution of smooth pigweed accessions collected in 2018 across cropping systems in Argentina. Blue dots are the locations of the accessions that were collected.
Seedling Establishment
The experiments were carried out at two sites: Faculty of Agronomy, University of Buenos Aires (UBA) and Faculty of Agricultural Sciences, University of Rosario (UNR). At UBA, seeds were germinated at fluctuating temperatures 20/30 C (12-h/12-h) and a 12-h photoperiod. At uniform 1-leaf stage, seedlings were transplanted into 0.5-L plastic pots. At UNR, seeds were germinated on moistened filter paper and incubated at alternate temperatures of 25/35 C (10-h/14-h) and a 16-h photoperiod. By 24 to 48 h, pre-germinated seeds were transplanted into 0.5-L pots. At both experimental sites, seedlings were watered as needed, and plants were maintained under outdoor conditions.
Herbicide Treatments and Plant Survival Evaluation
At both sites, herbicides were applied in December 2018. The herbicides and the recommended rates used in each treatment are described in Table 1. In addition, a nontreated control was included for comparison. The experimental unit consisted of a pot containing four plants. There were six replicates of each treatment in each accession arranged in a completely randomized design. Plants were treated at the 4- to 7-leaf stage (5 to 8 cm tall) using a cabinet sprayer with a spray volume of 140 L ha−1 at a pressure of 300 kPa. Survival was assessed 30 d after treatment (DAT). Plants were recorded alive if they were actively growing after treatment and dead if there was no presence of photosynthetically active tissue. Survival was expressed as the proportion of surviving individuals in relation to the total number of treated seedlings.
Table 1. Herbicides and rates used for screening 50 smooth pigweed accessions collected across cropping systems in Argentina in 2018. a

a Abbreviations: EPSPS, 5-enolpyruvylshikimate-3-phosphate synthase; HPPD, 4-hydroxphenylpyruvate dioxygenase; PPO, protoporphyrinogen oxidase.
b As classified by the Weed Science Society of America.
c Rizospray Extremo was added at 200 ml ha−1.
d Added Dash MSO Max was added at 250 ml ha−1.
Using a process similar to that described by González-Torralva et al. (Reference González-Torralva, Norsworthy, Piveta, Varanasi, Barber and Brabham2020), plant survival (%) in response to the herbicides in each accession was used to obtain descriptive statistics (mean, median, quartile 25%, and quartile 75%), using INFOSTAT software (Di Rienzo et al. Reference Di Rienzo, Casanoves, Balzarini, Gonzalez, Tablada and Robledo2011). This analysis was carried out to evaluate the sensitivity of smooth pigweed accessions to commonly used herbicides with different sites of action, but not to evaluate the efficacy of each herbicide. In addition, the response of each accession was categorized according to the survival percentage (e.g., susceptible [0%], very low survival [1% to 20%], low survival [21% to 40%], medium survival [41% to 60%], and high survival [61% to 100%]).
Results and Discussion
Synthetic Auxin
On average across accessions, plant survival to 2,4-D and dicamba was lower compared to survival rates with other herbicides. Plant survival was 2.2% and 2.6% for 2,4-D and dicamba, respectively (Figure 2). Overall, 84% of smooth pigweed accessions were susceptible to 2,4-D (i.e., no surviving individuals; Figure 3). However, a single accession showed greater than 70% survival to 2,4-D (Figure 3). Interestingly, no accessions with similar survival levels were recorded in nearby fields. No accessions showed greater than 20% plant survival to dicamba, and the number of accessions with no survival was lower than that with 2,4-D (Figure 3). Although the number of plants that survived treatment with 2,4-D and dicamba were low, it must be considered that the rates applied with both auxin herbicides in this study were higher than those commonly used in wheat and maize crops, since they are the recommended rates for use on genetically modified soybean resistant to 2,4-D and dicamba. Interestingly, four accessions showed some degree of survival to both 2,4-D and dicamba (Table 2). Dellaferrera et al. (Reference Dellaferrera, Cortés, Panigo, De Prado, Christoffoleti and Perreta2018) identified four accessions resistant to dicamba, and three of those were also resistant to 2,4-D, whereas only one accession was also resistant to glyphosate. Interestingly, these accessions were also from the main crop production area in Argentina, but were located in Santa Fe Province, almost 300 km from the Buenos Aires province area where the accession with a high degree of survival to 2,4-D was identified in the present study. Tehranchian et al. (Reference Tehranchian, Norsworthy, Powles, Bararpour, Bagavathiannan, Barber and Scott2017) also demonstrated that low-dose selection with dicamba during three Palmer amaranth generations resulted in high levels of cross-resistance to both dicamba and 2,4-D.
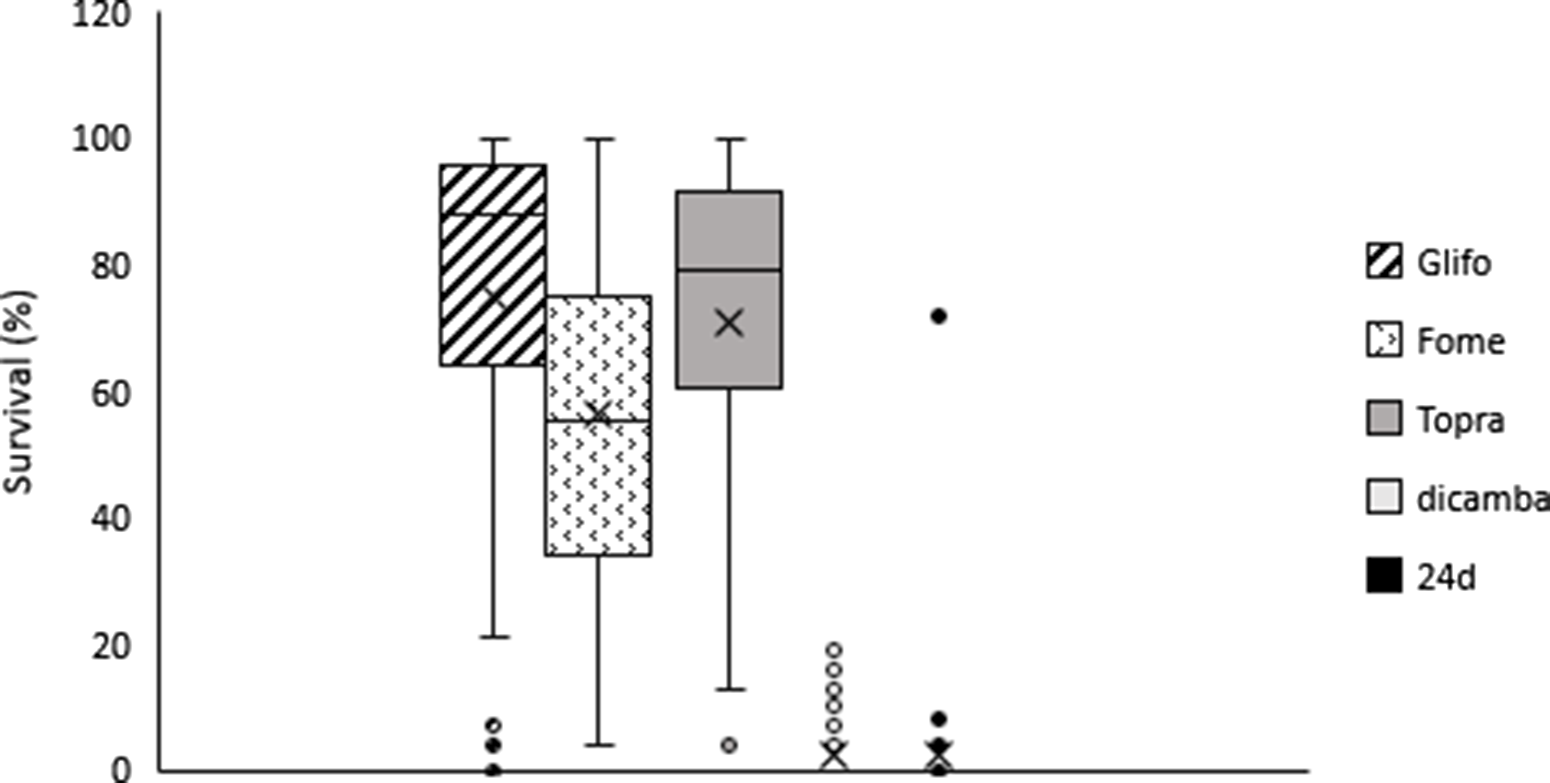
Figure 2. Survival dispersion (%) for glyphosate, fomesafen, topramezone, dicamba, and 2,4-D treatments. Box low limit Q1, box max limit Q3, horizontal line: Median, x arithmetic average, whiskers show max and low limit, •, ¤ atypical points.
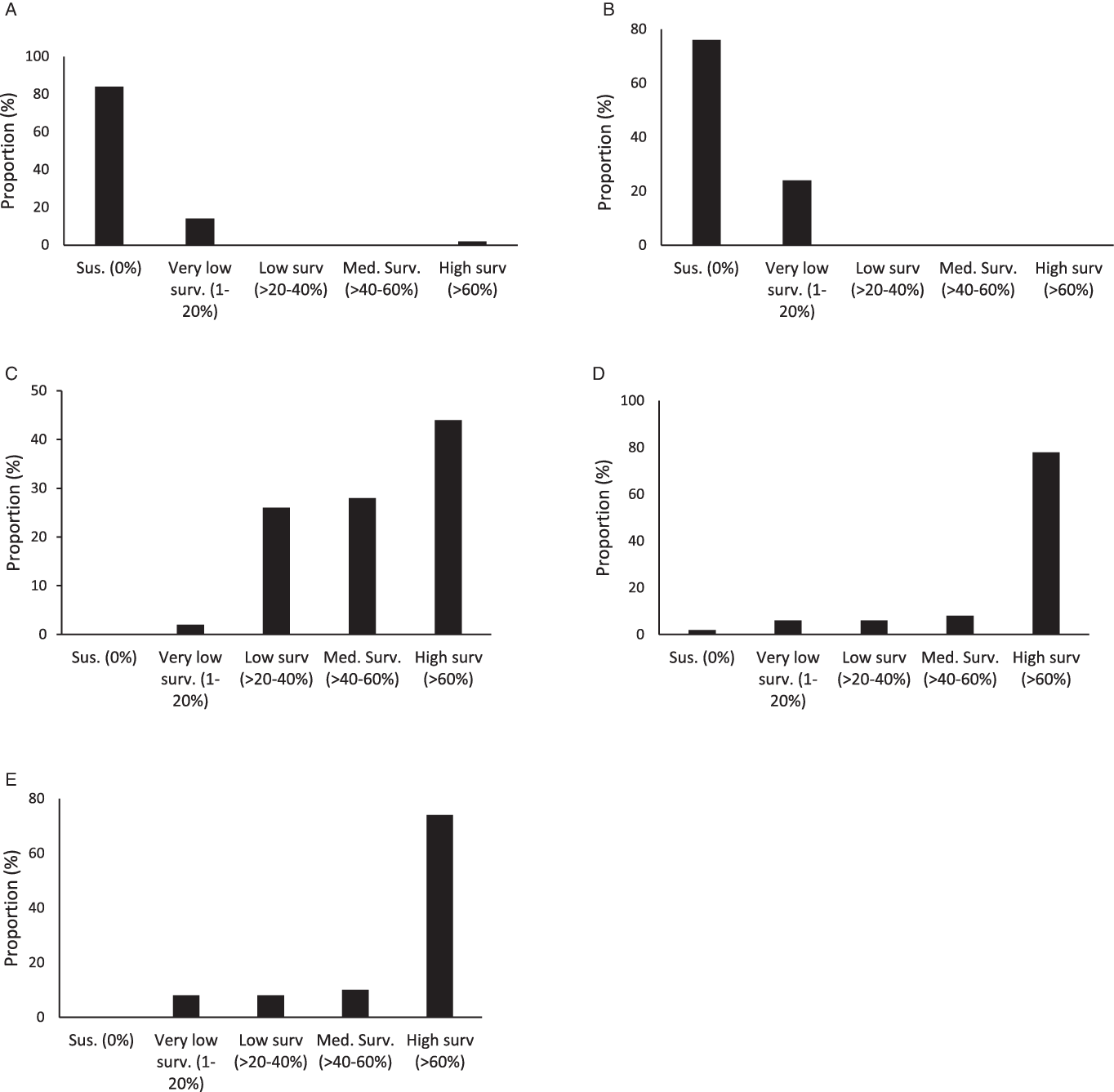
Figure 3. Proportion (%) of accessions with different ranges of plant survival to 2,4-D (A), dicamba (B), fomesafen (C), glyphosate (D), and topramezone (E) among the 50 accessions studied.
Table 2. Survival (%) of smooth pigweed accessions screened for sensitivity to different herbicides.a

a Susceptible (0%, white), very low survival (1% to 20%, green), low survival (21% to 40%, light blue), medium survival (41% to 60%, yellow), and high survival (61% to 100%, red).
These results constitute a warning sign for the current and future management of smooth pigweed accessions in auxinic-resistant soybean cultivated in Argentina. Results show low levels of variability in 2,4-D and dicamba sensitivity at the population level, highlighting the current importance of auxinic herbicides as chemical tools for the control of smooth pigweed. However, continuous use of these chemical tools without sustainable weed management programs will risk the future utility of these herbicides. Farmer responses to the Agricultural Resource Management Survey for 2018 suggest that approximately 43% of U.S. soybean acreage was planted with dicamba-resistant seeds in 2018 (Wechsler et al., Reference Wechsler, Smith, Mcfadden, Dodson and Williamson2020). The increase in dicamba-resistant seed use from its launch in 2016 to 2018 is similar to the rate at which soybean farmers adopted GR varieties in the years immediately following their commercial introduction, from 1996 to 1998 (USDA-ERS 2021). An unprecedented increase in GR soybean area in Argentina has occurred since its launch (Rossi Reference Rossi2006). It is likely that the adoption of dicamba-resistant and 2,4-D–resistant traits will follow a similar pattern of adoption in Argentina.
Fomesafen (A PPO Inhibitor)
Survival to fomesafen was observed in all smooth pigweed accessions. On average across accessions, plant survival to fomesafen was 56% (Figure 2). Seventy two percent of accessions showed survival greater than 40% (medium and high survival) (Figure 3), in which regrowth of damaged individuals was evident 2 wk after treatment.
The activity of PPO-inhibiting herbicides can be reduced with low light intensity, low relative humidity, low temperature, or water stress. Furthermore, spray coverage is important for optimal activity of contact herbicides (Coetzer et al. Reference Coetzer, Al-Khatib and Loughin2001; Kudsk and Kristensen Reference Kudsk and Kristensen1992; Wichert et al. Reference Wichert, Bozsa, Talbert and Oliver1992). The present study was carried out under controlled conditions, avoiding thermal and water stress, with adequate coverage and water volume. Therefore, plant survival is likely due to resistance to PPO-inhibiting herbicides. Currently, only one case of PPO resistance in smooth pigweed has been reported in Bolivia (Heap Reference Heap2021). Although no cases of fomesafen resistance have been registered in Argentina, there have been many anecdotal reports about the low efficacy of some field applications, which is likely due to developing resistance. However, resistance to PPO inhibitors has been identified in redroot pigweed (Amaranthus retroflexus L.) in Brazil and China, waterhemp [A. tuberculatus (Moq.) Sauer] in Canada and the United States, and Palmer amaranth in the United States (Heap Reference Heap2021). Target-site resistance is usually associated with most of the cases of PPO resistance involving a deletion of the amino acid glycine (ΔG210), and several PPO amino acid substitutions (Giacomini et al. Reference Giacomini, Umphres, Nie, Mueller, Steckel, Young, Scott and Tranel2017; Varanasi et al. Reference Varanasi, Brabham and Norsworthy2018b). In addition, Varanasi et al. (Reference Varanasi, Brabham, Norsworthy, Nie, Young, Houston, Barber and Scott2018a) characterized the first case of non-target site resistance to PPO inhibitors, which is likely mediated by cytochrome P450 and glutathione S-transferase in Palmer amaranth.
Topramezone (An HPPD Inhibitor)
Plant survival to topramezone was observed in all smooth pigweed accessions. On average across accessions, plant survival was 71% (Figure 2). More than 70% of the accessions exhibited survival greater than 60% (Figure 3). A recent report on smooth pigweed from Arkansas in the United States documented that 50% of accessions showed 49% survival when treated with the HPPD inhibitor tembotrione (González-Torralva et al. Reference González-Torralva, Norsworthy, Piveta, Varanasi, Barber and Brabham2020).
Topramezone is one of the most commonly used postemergence herbicides in corn in Argentina, and is usually mixed with atrazine (a photosystem II [PS II] inhibitor) to control Amaranthus species, and this may account for the low performance of topramezone in the present study. Mixtures of both herbicides (HPPD + PS II inhibitors) often show a synergistic effect, which is explained by the reduction of plastoquinone synthesis, and which is further competitively displaced by atrazine at the binding site in the PS II D1 protein (Gronwald Reference Gronwald and Resistance1994). Kohrt and Sprague (Reference Kohrt and Sprague2017) identified a synergistic response in the control of Palmer amaranth when mesotrione was applied with atrazine.
Glyphosate (An EPSPS Inhibitor)
More than 50% of the tested accessions showed plant survival greater than 88% to glyphosate (Figure 2), and 78% of accessions showed survival greater than 60% (Figure 3). Only one accession was sensitive to glyphosate. These results agree with the spread of glyphosate resistance often reported in agricultural fields in Argentina. Molecular studies carried out by Perotti et al. (Reference Perotti, Larran, Palmieri, Martinatto, Alvarez, Tuesca and Permingeat2019) revealed the presence of both a triple target site of EPSPS mutation (TAP-IVS: T102I, A103V, and P106S) and an increase in EPSPS gene copy number in a smooth pigweed accession from the main soybean production area. Remarkably, the resistance index (RI; DL50R/DL50S) observed in this particular accession was very high compared to GR species exhibiting other glyphosate resistance mechanisms (Sammons and Gaines Reference Sammons and Gaines2014; Vila-Aiub et al. Reference Vila-Aiub, Han, Yu, García and Powles2021).
Conclusions
Argentinian smooth pigweed accessions showed the highest sensitivity levels to auxin herbicides, with a particular single accession showing a survival greater than 60% when exposed to 2,4-D. Smooth pigweed accessions were least sensitive to fomesafen and topramezone, as none of the 50 accessions showed complete mortality. These herbicides are two of the most commonly used herbicides in soybean and corn for the control of Amaranthus species in Argentina. Finally, nearly 80% of the smooth pigweed accessions showed high survival rates to glyphosate. Interestingly, most of the accessions showed medium to high levels of survival to fomesafen, topramezone, and glyphosate treatments.
This study is the first attempt to characterize the response of smooth pigweed accessions to the most commonly used herbicides across the main cropping areas in Argentina. The results show that most of the accessions showed low levels of survival to auxin herbicides. The differences in herbicide sensitivity in the smooth pigweed accessions demonstrate the presence of genetic variability on which herbicide selection pressure is currently in action. It is imperative to implement weed management practices that preserve the efficacy of these important postemergence herbicides such as 2,4-D and dicamba now, given the likelihood of widespread adoption of these traits within Argentinian soybean production in the near future.
Acknowledgments
We thank HRAC Argentina, particularly agronomist Germán Ferrari, for his collaboration in this study and data analysis. The study was financially supported by HRAC Argentina (Committee of Action of Resistance to Herbicides of Argentina) and CASAFE (Chamber of Agricultural Health and Fertilizers). No conflicts of interest have been declared.