Introduction
Waterhemp is becoming an increasingly challenging weed to control because it continues to evolve resistance to more herbicide modes of action. Its extended emergence pattern and rapid growth rate contribute to its competitiveness. Although the presence of waterhemp in the United States dates to the early 1900s, its significance as an agricultural weed was not realized until almost a century later (Hartzler Reference Hartzler2019). Originally, waterhemp was primarily found in floodplains and marshes; however, waterhemp biotypes have adapted to much drier and hotter conditions, allowing it to spread rapidly throughout North America (Costea et al. Reference Costea, Weaver and Tardif2005).
Waterhemp has rapidly evolved resistance to many different herbicide modes of action due to its high fecundity and wide genetic diversity. Waterhemp is a dioecious species; to produce viable offspring separate plants must cross-pollinate, which results in great genetic diversity among offspring (Bell and Tranel Reference Bell and Tranel2020; Montgomery et al. Reference Montgomery, Sadeque, Giacomini, Brown and Tranel2019). Since the first report in 1993 of waterhemp resistance to acetolactate synthase (ALS)–inhibiting herbicides (a Group 2 herbicide as categorized by the Weed Science Society of America [WSSA]), waterhemp has evolved resistance to six additional modes of action including the synthetic auxins (Group 4), and those that inhibit photosystem II (PS II, Group 5), 5-enolpyruvylshikimate-3-phosphate synthase (EPSPS, Group 9), protoporphyrinogen oxidase (PPO, Group 14), very long-chain fatty acid elongases (VLCFAEs, Group 15), and 4-hydroxyphenylpyruvate dioxygenase (HPPD, Group 27) (Heap Reference Heap2021). In the United States and Ontario, Canada, six- and five-way herbicide-resistant waterhemp has been reported, respectively (Heap Reference Heap2021; Shergill et al. Reference Shergill, Barlow, Bish and Bradley2018; Symington et al. Reference Symington, Soltani and Sikkema2022). Despite the presence of multiple herbicide-resistant (MHR) biotypes, when used properly, effective herbicides are still a critically important tool in a diversified, integrated waterhemp management program.
Waterhemp has many characteristics that contribute to its success as a weed. It is a competitive weed that can drastically reduce crop yield. In studies completed in the United States and Ontario, Canada, waterhemp interference has reduced soybean and corn yields by up to 73% and 74%, respectively, when no control measures were implemented (Steckel and Sprague Reference Steckel and Sprague2004; Vyn et al. Reference Vyn, Swanton, Weaver and Sikkema2007). Waterhemp has a season-long emergence pattern that is unlike many other annual broadleaf weeds (Jhala et al. Reference Jhala, Norsworthy, Ganie, Sosnoskie, Beckie, Mallory-Smith, Liu, Wei, Wang and Stoltenberg2021). In Canada and the United States, waterhemp begins emerging in May and continues to emerge into the fall (Costea et al. Reference Costea, Weaver and Tardif2005). Vyn et al. (Reference Vyn, Swanton, Weaver and Sikkema2006) and Schryver et al. (Reference Schryver, Soltani, Hooker, Robinson, Tranel and Sikkema2017) documented that in Ontario, waterhemp began emerging after seedbed preparation in conventionally tilled fields and continued to emerge throughout the summer months. Vyn et al. (Reference Vyn, Swanton, Weaver and Sikkema2006) noted that peak waterhemp emergence occurred in mid-June with some emerging through October (Schryver et al. Reference Schryver, Soltani, Hooker, Robinson, Tranel and Sikkema2017). Hartzler et al. (Reference Hartzler, Buhler and Stoltenberg1999) reported that waterhemp continued to germinate and emerge later into the growing season than velvetleaf, woolly cupgrass, and giant foxtail. Leon and Owen (Reference Leon and Owen2006) reported that in no-till systems, the majority of waterhemp emerged during the latter part of June, whereas in tilled systems, most waterhemp emerged in May and early June; emergence declined substantially over the remainder of the growing season. Franca (Reference Franca2015) found that 90% of cumulative waterhemp emergence occurred by the end of June regardless of tillage treatment; soil temperature followed by soil moisture were the greatest indicators of waterhemp emergence. Waterhemp germinates between 25 to 35 C (Guo and Al-Khatib Reference Guo and Al-Khatib2003). Waterhemp is also resilient and can thrive in water-stressed and shaded environments (Sarangi et al. Reference Sarangi, Irmak, Lindquist, Knezevic and Jhala2016; Steckel et al. Reference Steckel, Sprague, Hager, Simmons and Bollero2003).
Waterhemp produces copious amounts of seed, which increases the weed problem in succeeding years. When not subjected to competition, a single waterhemp plant can produce up to 4.8 million seeds (Hartzler et al. Reference Hartzler, Battles and Nordby2004). Waterhemp seed production is highly influenced by surrounding plant competition and time of emergence. Steckel et al. (Reference Steckel, Sprague, Hager, Simmons and Bollero2003) reported that even late emerging waterhemp and other plants subjected to up to 68% shade can produce an abundance of seed. Late flushes of waterhemp are often uncontrolled, allowing these plants to contribute viable seeds to the weed seed bank in the soil.
With the evolution of MHR waterhemp, the development of two-pass weed control programs is increasing, with an effective soil-applied residual herbicide in the first pass (Beckie Reference Beckie2011; Gonzini et al. Reference Gonzini, Hart and Wax1999; Mahoney et al. Reference Mahoney, Shropshire and Sikkema2014; Wuerffel et al. Reference Wuerffel, Young, Matthews and Young2015). Mahoney et al. (Reference Mahoney, Shropshire and Sikkema2014) reported ≥99% control of pigweed, a close relative of waterhemp, with various soil-applied residual herbicides applied preemergence (PRE). Meyer et al. (Reference Meyer, Norsworthy, Young, Steckel, Bradley, Johnson, Loux, Davis, Kruger, Bararpour and Ikley2015) found that a PRE application of dicamba + acetochlor (1,120 + 2,307 g ae/ai ha−1) was effective at controlling MHR waterhemp; it provided >90% control at 6 to 7 wk after application, which was similar to PRE followed by early postemergence (POST) herbicide programs. A study conducted by Harder et al. (Reference Harder, Nelson and Smeda2012) also demonstrated the importance of using PRE herbicides for controlling waterhemp. With the exception of the POST application of mesotrione + crop oil concentrate + diammonium sulfate (110 g ai ha−1 + 1% vol/vol + 9.5 kg ha−1) in 2002, control with a POST herbicide did not exceed 72%, whereas control with PRE herbicides was >84%; in 2003, control with PRE herbicides ranged from 90% to 95%, whereas POST herbicides controlled waterhemp by 0% to 68% with one POST herbicide providing 87% control. These results corroborate those of other studies that have shown that PRE-residual herbicides provide greater waterhemp control than POST-alone herbicide programs (Johnson et al. Reference Johnson, Breitenbach, Behnken, Miller, Hoverstad and Gunsolus2012; Legleiter et al. Reference Legleiter, Bradley and Massey2009; Taylor-Lovell et al. Reference Taylor-Lovell, Wax and Bollero2002). Legleiter et al. (Reference Legleiter, Bradley and Massey2009) found that PRE-only and POST-only herbicides reduced waterhemp seed production by 61% to 94% and 21% to 71%, respectively.
The Group 15 herbicides include eight chemical families (Boger et al. Reference Boger, Matthes and Schmalfu2000; Shaner Reference Shaner2003). Herbicides from four chemical families were evaluated in the current study: the chloroacetamide, chloroacetanilide, isoxazoline, and oxyacetamide chemical families, which represent the most frequently used Group 15 herbicides for Ontario row crop production. Some of the most common active ingredients that belong to the Group 15 herbicides are acetochlor, dimethenamid-p, flufenacet, S-metolachlor, and pyroxasulfone (Boger et al. Reference Boger, Matthes and Schmalfu2000; Shaner Reference Shaner2003). Group 15 herbicides inhibit VLCFAEs (Tanetani et al. Reference Tanetani, Kaku, Kawai, Fujioka and Shimizu2009; Trenkamp et al. Reference Trenkamp, Martin and Tietjen2004). Very long-chain fatty acids are important components of cell membranes and are important for lipids, cell division, polar auxin transport, cuticular wax development, and regulation of cell morphology. The Group 15 herbicides inhibit shoot elongation, which causes most susceptible seedlings to fail to emerge; those that do emerge appear distorted (Shaner Reference Shaner2014; Tanetani et al. Reference Tanetani, Kaku, Kawai, Fujioka and Shimizu2009). The Group 15 soil-residual herbicides are used primarily for small-seeded annual grass control; however, they do have some activity on some small-seeded broadleaf weeds, including waterhemp (Weisshaar and Boger Reference Weisshaar and Boger1987). Acetochlor is registered in the United States for control of 11 monocotyledonous and nine dicotyledonous weeds (Anonymous 2020a, 2020b).
With the increasing prevalence of MHR waterhemp in Ontario, it is crucial that growers have access to weed control programs that provide full-season control to minimize weed seed return to the soil and maximize farm profitability. The use of Group 15 herbicides is one component of a waterhemp control strategy. Although some studies have been conducted on waterhemp control with soil-applied residual herbicides (Jhala et al. Reference Jhala, Malik and Willis2015; Steckel et al. Reference Steckel, Sprague and Hager2002; Strom et al. Reference Strom, Gonzini, Mitsdarfer, Davis, Riechers and Hager2019), the research presented in this study focuses on MHR waterhemp control with several Group 15 herbicides. The objective of this research was to quantify the level and length of MHR waterhemp control provided by several Group 15 herbicides applied PRE in Ontario.
Materials and Methods
Four site-years of data were collected between 2021 and 2022 from commercial fields with naturally occurring MHR waterhemp. Waterhemp seed was collected and cleaned in the fall of 2019, 2020, and 2021 from female plants throughout each site. Seed samples were stratified for 6 wk to aid with seed germination and screened in the greenhouse for herbicide resistance using imazethapyr, atrazine, metribuzin, glyphosate, lactofen, and mesotrione; all sites contained five-way resistant biotypes to the herbicides in Groups 2, 5, 9, 14, and 27. All sites demonstrated high resistance levels to the herbicides in Groups 2, 5, 9, and 27; the level of Group 14 resistance varied between sites. Field trials were conducted near Cottam, ON (42.149046°N, 82.683986°W) in 2021 and 2022 (E1 and E3, respectively). and near Newbury, ON (42.690833°N, 81.822589°W) (E2 and E4), in 2021 and 2022. Soil characteristics for each site are presented in Table 1. The sites were vertically tilled in the fall followed by a pass with a tandem disc and another pass of a field cultivator in the spring. The previous crop in Cottam 2021 and Newbury 2022 was soybean, whereas the previous crop in Cottam 2022 and Newbury 2021 was corn.
Table 1. Year, site, and soil characteristics for four field trials conducted in southwestern Ontario in 2021 and 2022. a, b

a Abbreviations: CEC, cation exchange capacity; Env, environment; OM, organic matter.
b Soil analysis was performed by A&L Canada Laboratories Inc. (2136 Jetstream Road, London, ON, N5V 3P5 Canada) from soil cores taken to depths of 15 cm.
Trials were set up as a randomized complete block design with four replications. All trials were carried out with no crop planted to quantify waterhemp emergence and control in the absence of crop competition. Treatments included six Group 15 herbicides applied at different rates. There were 12 treatments in total: 1) an emulsifiable concentrate (EC) formulation of acetochlor (Harness®; Bayer Crop Science, St. Louis, MO) applied at 1,225, 2,100, and 2,950 g ai ha−1; 2) a capsule suspension formulation of acetochlor (Warrant®; Bayer Crop Science, St. Louis, MO) applied at 1,050, 1,375, and 1,700 g ai ha−1; 3) dimethenamid-p (Frontier® Max; BASF Canada, Mississauga, ON) applied at 693 g ai ha−1; 4) flufenacet (Define SC®; Bayer Crop Science, Calgary, AB) applied at 500 and 750 g ai ha−1; 5) S-metolachlor (Dual II Magnum®; Syngenta Canada, Guelph, ON) applied at 1,600 g ai ha−1; and 6) pyroxasulfone (Zidua® SC; BASF Canada, Mississauga, ON) applied at 246.5 g ai ha−1. All rates were established on the basis of label recommendations or proposed label rates where applicable for corn or soybean. A nontreated control was included in each replicate. Plots were 8 m long and 2 m wide with a 2-m alley between each replicate. Each plot was split into a front and back half to conduct two separate assessments within the same plot. Each half measured 4 m long and 2 m wide. In the front half of each plot, three randomly placed, permanent 0.25-m2 quadrats were established prior to herbicide application to measure waterhemp emergence. All quadrats were placed in the center 1 m of each plot to avoid lower rates at plot edges. All quadrats remained in place for the duration of the trial. All herbicide treatments were applied prior to waterhemp emergence with the use of a CO2-pressurized backpack sprayer that was calibrated to deliver 200 L ha−1 at 240 kPa. The boom consisted of four ultra-low drift (ULD 120-02; Hypro, Pentair Ltd., London, UK) nozzles that were spaced 50 cm apart to deliver a spray width of 2 m. Table 2 lists the application date for each site-year.
Table 2. Year, site, application date, rainfall, and average temperature for four field trials conducted in southwestern Ontario in 2021 and 2022.

a Thirty-year average values are based on data collected from the Ridgetown campus weather station (Ridgetown, ON), central to all sites, from 1991 to 2022.
Waterhemp control and emergence assessments commenced at 2 WAA and were performed at biweekly intervals with the final assessment at 12 WAA. Visible waterhemp control ratings were assessed from the back half of each plot by assigning a value of 0 to 100, which represented the estimated waterhemp biomass reduction relative to the nontreated control in each replicate; 0 represented no MHR waterhemp control and 100 indicated complete waterhemp control. At each assessment timing, the number of waterhemp that had emerged in the prior 14 d within each quadrat in the front half of the plot was recorded and then removed with an application of glufosinate (Liberty® 200 SN; BASF Canada, Mississauga, ON) at 500 g ha−1 that was applied perpendicular to the plot length across the front 4 m of each replicate.
Statistical Analysis
All data analyses were conducted using SAS software (v. 9.4; SAS Institute Inc., Cary, NC). Control data were analyzed using linear mixed-model variance analysis, the GLIMMIX procedure. Herbicide treatment was considered the fixed effect, whereas environment (site-year), replicate within environment, and treatment by environment were random effects. Data across all environments were pooled for analysis. The assumption that residuals were random, independent of treatment and design effects, have a mean of zero, homogenous, and normally distributed was confirmed by plotting the studentized residuals and referencing the Shapiro-Wilk test statistic.
Waterhemp emergence data were regressed against time in days using the NLIN procedure with SAS software. Two different equations were evaluated and compared for each treatment. Where waterhemp emergence over the course of the study followed a negative linear relationship, Equation 1 was used.

where y = response parameter, m = slope, and b = y intercept.
Where waterhemp emergence followed a descending dose-response curve, then Equation 2 was used.

where y = response parameter, c = upper asymptote, d = lower asymptote, b = slope about I, and I = days eliciting a response equidistant between c and d.
Where P values were significant at P < 0.05 for a sum of squares reduction test for each treatment, sites were separated. Predicted parameter values from the nonlinear regression were used to compute the number of days that each treatment provided a 50%, 80%, and 95% reduction in waterhemp emergence relative to the nontreated control for each 2-wk period. Where values were not computable, they were deemed to be not estimable, represented by NE in Table 3. Where values were able to be computed but they fell outside of the assessment range evaluated 0 to 84 d, they were expressed as NA, or not applicable.
Table 3. Predicted number of days that each herbicide reduces multiple herbicide-resistant waterhemp emergence by 50%, 80%, and 95% for four field trials conducted in a non-cropped area in southwestern Ontario in 2021 and 2022. a
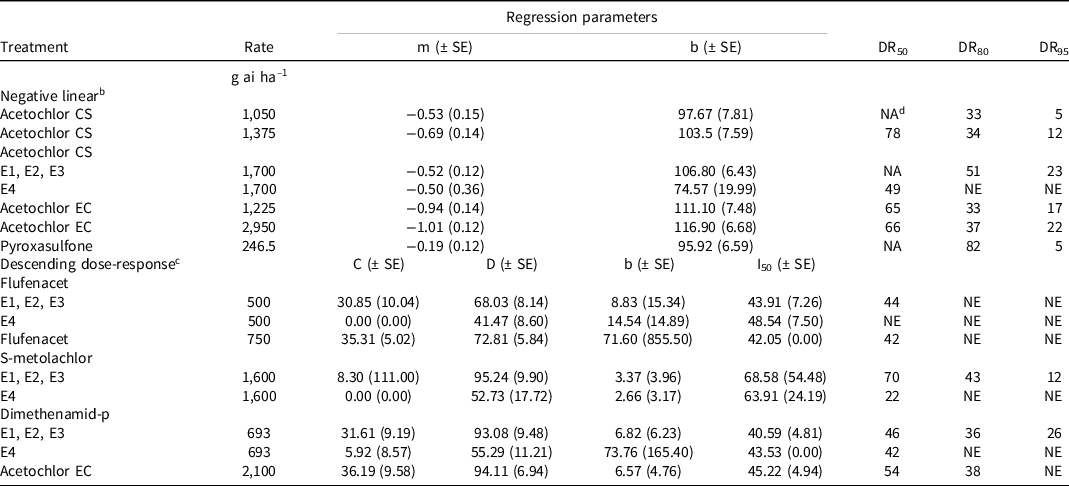
a Abbreviations: CS, capsule suspension; EC, emulsifiable concentrate; E1, Cottam 2021; E2, Newbury 2021; E3, Cottam 2022; E4, Newbury 2022; DR50, DR80, and DR95 denote the predicted number of days that each treatment provides 50%, 80%, and 95% density reductions of multiple herbicide-resistant waterhemp, respectively (based on the respective nonlinear regression equation and its parameters); NE, nonestimable; NA, not applicable; SE, standard error.
b Negative linear equation represented by y = m*x + b where m = slope and b = y-intercept.
c Descending dose response equation represented by y = c + (d – c)/(1 + e (b * (log(x) – log(I)))) where c = upper asymptote, d = lower asymptote, b = slope about I, and I 50 = days eliciting a response equidistant between c and d.
d Number of days exceeds trial period evaluated.
Results and Discussion
Rainfall (≥28 mm) occurred within 2 wk of herbicide application at all sites in 2021 and 2022 (Table 2). This amount was likely sufficient to dissolve the herbicides into the soil water solution. The average monthly temperatures in all four environments were equal to or higher than the 30-yr average (Table 2).
Multiple Herbicide-Resistant Waterhemp Emergence
Cumulative waterhemp emergence was between 493 and 2,583 plants m−2 in the 12 wk following herbicide application in the nontreated control (Figure 1). On average, 91% of waterhemp emergence occurred within 4 wk of the herbicide application; this correlated to the second or third week of June. Waterhemp continued to emerge during the months of June, July, August, and September but at progressively lower rates. Similarly, Franca (Reference Franca2015) noted that by the end of June, 90% of cumulative waterhemp had emerged. These trends are similar to those observed by Leon and Owen (Reference Leon and Owen2006), in which regardless of the tillage system, most waterhemp emerged in May and June, and emergence declined over the remainder of the summer and fall months.

Figure 1. Cumulative waterhemp emergence for each environment based on the average of four replications of the nontreated control treatment. E1, Cottam 2021; E2, Newbury 2021; E3, Cottam 2022; E4, Newbury 2022.
At the E1 (Cottam 2021), E2 (Newbury 2021), and E3 (Cottam 2022) locations, it was estimated that flufenacet (500 g ha−1) reduced waterhemp emergence by 50% for 44 days after application (DAA; Table 3); the number of DAA that flufenacet reduced waterhemp emergence by 80% and 95% was not estimable because it never provided >80% reduction in emergence. At E4 (Newbury 2022) the number of DAA that flufenacet reduced waterhemp emergence by 50%, 80%, and 95% was nonestimable. The high rate of flufenacet (750 g ha−1) was predicted to provide a 50% reduction in waterhemp emergence for 42 DAA; the number of DAA that flufenacet reduced waterhemp emergence by 80% or 95% was nonestimable. At E1, E2, and E3, it is estimated that S-metolachlor reduced waterhemp emergence by 50%, 80%, and 95% for 70, 43, and 12 DAA; in contrast, at E4 (high-density environment) it is estimated that S-metolachlor reduced waterhemp density by 50% for 22 DAA; the number of DAA that S-metolachlor reduced waterhemp emergence by 80% and 95% was nonestimable. Similarly, dimethenamid-p was estimated to reduce waterhemp emergence by 50%, 80%, and 95% at 46, 36, and 26 DAA at the E1, E2, and E3 locations, respectively; in contrast at the high-density environment (E4) dimethenamid-p was estimated to reduce waterhemp emergence by 50% for 42 DAA; an 80% and 95% reduction in waterhemp emergence at E4 was nonestimable. The low rate of acetochlor CS (1,050 g ha−1) was predicted to provide a 50% reduction in waterhemp emergence for greater than the duration of the assessments, while an 80% and 95% reduction was estimated for 33 and 5 DAA, respectively. The medium rate of acetochlor CS (1,375 g ha−1) was predicted to reduce waterhemp emergence by 50%, 80%, and 95% for 78, 34, and 12 DAA, respectively. The length of residual waterhemp control with the high rate of acetochlor CS (1,700 g ha−1) varied between environments. At E1, E2, and E3, the 50% predicted emergence reduction was beyond the assessment range, whereas the estimated 80% and 95% reductions were 51 and 23 DAA, respectively. At E4, a 50% reduction in emergence was predicted for 49 DAA but emergence reductions for 80% or 95% were nonestimable. With acetochlor EC at the low rate (1,225 g ha−1), 50%, 85%, and 95% reductions in waterhemp emergence were estimated for 65, 33, and 17 DAA, respectively. Acetochlor EC (2,100 g ha−1), was predicted to reduce waterhemp emergence by 50% and 80% for 54 and 38 DAA, respectively; a 95% reduction in waterhemp emergence was nonestimable. The high rate of acetochlor EC (2,950 g ha−1) was estimated to reduce waterhemp emergence by 50%, 80%, and 95% for 66, 37, and 22 DAA, respectively. Pyroxasulfone (246.5 g ha−1) was estimated to reduce waterhemp emergence by 80% and 95% for 82 and 5 DAA, respectively; the 50% emergence reductions could not be estimated because they exceeded the assessment range.
Based on the results of these trials, the longer residual activity of Group 15 herbicides was achieved in lower density waterhemp environments. Shorter 50%, 80%, and 95% emergence reductions were achieved at the E4 location, which contained the greatest cumulative emergence of waterhemp of the four sites (Figure 1). Similarly, Willemse et al. (Reference Willemse, Soltani, Benoit, Hooker, Jhala, Robinson and Sikkema2021) reported that in environments with greater waterhemp density and biomass, control was as much as 34% lower than in low-density waterhemp environments. Therefore, in high weed pressure fields, active scouting is needed for the timely application of POST herbicides to control later emerging waterhemp flushes.
Multiple Herbicide-Resistant Waterhemp Control
Across all herbicides tested, flufenacet (500 g ha−1) controlled waterhemp the least, at 54% at 2 WAA, and it was similar to that of flufenacet (750 g ha−1), S-metolachlor, dimethenamid-p, and acetochlor CS (1,050, 1,375, and 1,700 g ha−1), which varied numerically from 59% to 77% (Table 4). Acetochlor EC at 1,225, 2,100, and 2,950 g ha−1 provided numerically better control than the aforementioned treatments 2 WAA at 88%, 91%, and 91% respectively. The medium and high rates of acetochlor EC provided greater control than flufenacet (500 and 750 g ha−1) and S-metolachlor. Pyroxasulfone controlled waterhemp 84% at 2 WAA, which was greater than flufenacet (500 g ha−1) and S-metolachlor but similar to all other treatments.
Table 4. Mean percent control of multiple herbicide-resistant waterhemp at 2, 4, 6, 8, 10, and 12 wk after PRE application of Group 15 herbicides in a non-cropped area for four field trials conducted in southwestern Ontario in 2021 and 2022. a, b

a Abbreviations: CS, capsule suspension; EC, emulsifiable concentrate; WAA, weeks after application.
b Means followed by the same letter (a–d) within a column are not significantly different according to Tukey-Kramer grouping at P < 0.05.
MHR waterhemp control increased from 2 to 4 WAA with all treatments (Table 4). Flufenacet (500 and 750 g ha−1) controlled MHR waterhemp 77% to 80%, which was lower than the medium rate of acetochlor CS, all rates of acetochlor EC, and pyroxasulfone. Waterhemp control with S-metolachlor was 87%; similar to all other treatments. Dimethenamid-p provided 92% waterhemp control, which was greater than the low rate of flufenacet. Acetochlor (CS and EC formulations) at the low, medium, and high rates and pyroxasulfone controlled waterhemp similarly at 91% to 98%. Hausman et al. (Reference Hausman, Tranel, Riechers, Maxwell, Gonzini and Hager2013) reported that acetochlor EC (1,680 g ha−1) and pyroxasulfone (210 g ha−1) controlled waterhemp 87% in corn and soybean at 4 WAA, which is similar to the results from the current study. Steckel et al. (Reference Steckel, Sprague and Hager2002) reported that acetochlor EC (1,960 g ha−1) provided 98% waterhemp control, and Oliveira et al. (Reference Oliveira, Jhala, Gaines, Irmak, Amundsen, Scott and Knezevic2017) documented that pyroxasulfone (270 g ha−1) applied PRE provided 95% control of waterhemp at 4 WAA, consistent with the results from this study.
MHR waterhemp control started declining from 4 WAA with all treatments except pyroxasulfone (Table 4). Waterhemp control with flufenacet (500 and 750 g ha−1), S-metolachlor, and dimethenamid-p decreased by 20%, 16%, 9%, and 9%, respectively, from 4 to 6 WAA. S-metolachlor provided 78% waterhemp control 6 WAA; waterhemp control with S-metolachlor applied PRE at similar rates was extremely variable from 6% to 95% (Hausman et al. Reference Hausman, Tranel, Riechers, Maxwell, Gonzini and Hager2013; Steckel et al. Reference Steckel, Sprague and Hager2002; Strom et al. Reference Strom, Gonzini, Mitsdarfer, Davis, Riechers and Hager2019); the results from this study were within this range. Waterhemp control with acetochlor CS declined by 9%, 12%, and 7% from 4 to 6 WAA for the low, medium, and high rates, respectively. The two formulations of acetochlor at all three rates provided similar waterhemp control. This corresponds to the acetochlor label, which claims up to 4 wk residual control (Anonymous 2020a, 2020b). Pyroxasulfone provided the highest control of waterhemp (99%) at 6 WAA, which was similar to all acetochlor treatments and dimethenamid-p.
MHR waterhemp control with all treatments decreased from 6 to 12 WAA in each respective 2-wk increment (Table 3). Steckel et al. (Reference Steckel, Sprague and Hager2002) reported 61% and 57% control of waterhemp in corn with dimethenamid (1,050 g ha−1) and S-metolachlor (1,420 g ha−1) applied PRE, respectively at 8 WAA. This is consistent with the findings of 67% and 64% control with dimethenamid-p and S-metolachlor, respectively, in this study at 8 WAA. Another study by Hausman et al. (Reference Hausman, Tranel, Riechers, Maxwell, Gonzini and Hager2013) investigated waterhemp control in corn and soybean and concluded that in soybean S-metolachlor (1,425 g ha−1) applied PRE provided 55% waterhemp control; however, in corn S-metolachlor (1,600 g ha−1) applied PRE provided only 7% waterhemp control, demonstrating that waterhemp control with S-metolachlor is dependent on rate and environment. Acetochlor CS at 1,050, 1,375, and 1,700 g ha−1 controlled waterhemp by 67%, 73%, and 81%, respectively, at 8 WAA. Steckel et al. (Reference Steckel, Sprague and Hager2002) reported that acetochlor CS at a higher rate of 1,960 g ha−1 controlled waterhemp by 85% to 95%. Acetochlor EC at 1,225, 2,100, and 2,950 g ha−1 controlled waterhemp by 76%, 78%, and 83%, respectively, at 8 WAA. Pyroxasulfone controlled waterhemp 97%, which was similar to all rates of acetochlor EC and the medium and high rates of acetochlor CS. Among all treatments evaluated, only pyroxasulfone provided greater than 80% waterhemp control up to 10 WAA.
Waterhemp control was similar across herbicides at 10 and 12 WAA. At 12 WAA, all herbicides controlled waterhemp 21% to 53%, with the exception of pyroxasulfone (Table 4). Pyroxasulfone controlled waterhemp by 77%, which was greater than all treatments except the high rate of acetochlor CS and the low and high rate of acetochlor EC.
One limitation of this study is that waterhemp emergence may occur much differently in the presence of crop competition. Waterhemp emergence has been shown to be influenced by soil moisture and soil temperature (Franca Reference Franca2015), which will vary in a cropped field. The current study mimics fallow ground, and soil parameters such as soil moisture have been found to vary substantially in fallow ground situations (McGuire et al. Reference McGuire, Bryant and Denison1998; Tanaka and Aase Reference Tanaka and Aase1987); therefore, those same parameters would likely vary in a corn, soybean, or other row crops compared to the results observed from the current study. However, the presence of a crop such as corn or soybean will also act as a control method against waterhemp emergence due to competition and may reduce overall emergence. Soil residual herbicides require rainfall for activation; in the absence of rainfall to dissolve herbicides like those in Group 15, lower control, and shorter residual activity will likely be observed (Hartzler Reference Hartzler2021).
In conclusion, pyroxasulfone provided the highest waterhemp control and provided the longest residual control. Group 15 herbicides provided >80% waterhemp control for 6 WAA except for flufenacet and S-metolachlor. Dimethenamid-p, acetochlor EC, S-metolachlor, acetochlor CS, and pyroxasulfone reduced waterhemp emergence by 80% for up to 36, 38, 43, 51, and 82 DAA, respectively; flufenacet (500 and 750 g ha−1) never reduced waterhemp emergence more than 80% in this study. Pyroxasulfone applied PRE was the most efficacious Group 15 herbicide evaluated in this study for waterhemp control. It controlled MHR waterhemp >80% up to 10 WAA. Acetochlor provided good control of waterhemp; however, control began to decline after 6 WAA. S-metolachlor and dimethenamid-p provided intermediate control, and flufenacet was the least efficacious on waterhemp. However, in the absence of crop competition, these herbicides did not provide season-long control; late-emerging plants may be capable of contributing viable seeds to the soil weed seed bank. These herbicides are one component of a diversified, integrated waterhemp control program; however, POST-applied herbicides may be required to control late flushes that emerge beyond the residual period provided by Group 15 herbicides. An effective POST herbicide applied after the PRE application of pyroxasulfone or acetochlor would also reduce the selection pressure for the evolution of Group 15–resistant waterhemp. Given the confirmation of Group 15–resistant waterhemp in the United States and Canada, it is of utmost importance that selection pressure on currently effective herbicides is minimized.
Practical Implications
Waterhemp is a challenge to control and continues to evolve resistance to new herbicide modes of action. To achieve adequate control of this troublesome weed and reduce further seed return to the soil seed bank, the use of a soil-applied residual herbicide is necessary. However, not all soil-applied herbicides are effective in controlling MHR waterhemp, and the length of residual control varies with those herbicides that are effective. Group 15 resistant waterhemp has not been reported in Ontario. Group 15 herbicides are considered effective soil-applied chemistry to control waterhemp. The results of this research are helpful in quantifying the soil residual capacity of various Group 15 herbicides to control MHR waterhemp in Ontario. Additionally, herbicide manufacturers are currently awaiting registration from the Pest Management Regulatory Agency in Canada to approve acetochlor use in Canada. If acetochlor becomes registered for use in Ontario, this research will provide weed management practitioners with reliable data to demonstrate that pyroxasulfone or acetochlor are the most efficacious Group 15 herbicides to combat MHR waterhemp. This research also provides growers and agronomists with a strong base from which to establish a two-pass weed control strategy to control MHR waterhemp. The results of this study clearly demonstrate that a Group 15 herbicide such as pyroxasulfone or acetochlor can reduce waterhemp emergence. Given that waterhemp can emerge throughout the season and even late emerging plants can contribute seed to the soil weed seed bank, it is unlikely that any of these PRE Group 15 herbicides would provide sufficient weed control alone and should be applied in a two-pass system.
Acknowledgments
We thank Dr. Michelle Edwards for her statistical support; the University of Guelph, Ridgetown Campus summer staff for their field support; and Bayer Crop Science Inc., Ontario Bean Growers (OBG), and the Ontario Agri-Food Innovation Alliance for the funding to conduct this research. A co-author of this manuscript, Allan Kaastra, is the Senior Agronomic Development Representative, Bayer Crop Science Inc. Other authors have no conflict of interest to declare.