Introduction
Producers frequently combine multiple herbicides into a single application to achieve greater weed control as well as save application costs by reducing the number of trips across the field (Jordan Reference Jordan1995; Rhodes and Coble Reference Rhodes and Coble1984; Zhang et al. Reference Zhang, Webster, Blouin and Leon2005). For example, many herbicides with broadleaf activity are mixed with graminicides for broad-spectrum control. Nonselective POST herbicides (e.g., glufosinate, a glutamine synthetase inhibitor) may be combined with residual herbicides such as S-metolachlor (very-long-chain fatty-acid inhibitor) to extend herbicidal activity in the soil. One advantage of combining herbicides with different sites of action (SOAs) is increasing the longevity of a single herbicide or SOA. This is important for producers facing herbicide-resistant weed issues, especially as a tremendous amount of selection pressure can be placed on POST applications due to the size and number of weeds present at application (Hydrick and Shaw Reference Hydrick and Shaw1994; Lanclos et al. Reference Lanclos, Webster and Zhang2002; Norsworthy et al. Reference Norsworthy, Ward, Shaw, Llewellyn, Nichols, Webster, Bradley, Frisvold, Powles, Burgos, Witt and Barrett2012; Webster and Shaw Reference Webster and Shaw1997).
Before mixing herbicides with different SOAs, it is important to consider the possibility that some combinations may demonstrate compatibility issues as well as antagonistic or synergistic interactions. Contact herbicides can antagonize the activity of systemic herbicides; for example, glufosinate has been documented to antagonize the efficacy of clethodim on goosegrass [Eleusine indica (L.) Gaertn.] by disrupting cell membranes via accumulation of toxic levels of ammonia, which results in reduced translocation of clethodim (Burke et al. Reference Burke, Askew, Corbett and Wilcut2005). Mixing systemic and contact herbicides such as dicamba and glufosinate has been documented to increase efficacy on Palmer amaranth (Amaranthus palmeri S. Watson) compared with an application of either herbicide alone (Barnett et al. Reference Barnett, Mueller and Steckel2013; Chahal and Johnson Reference Chahal and Johnson2012). Despite some research showing increased control of Palmer amaranth with this mixture, antagonism has been observed on Palmer amaranth as well (Botha Reference Botha2013). It is possible that dicamba residue remaining in the tank could interact with the subsequently added chemical, which is likely to be a contact herbicide during peak soybean-growing season. Such an interaction might cause more intense initial phytoxocity of the contact herbicide or increased severity of visible auxin symptomology. Tank contamination with sublethal rates of dicamba may also increase the severity of visible auxin symptomology by inducing additional plant stress. However, antagonism between dicamba tank contamination and contact herbicides resulting in less visible auxin symptomology to sensitive soybean would not be unexpected. Soybean displaying minor to moderate contact symptomology (chlorotic and necrotic lesions) commonly associated with protoporphyrinogen oxidase (PPO)-inhibiting herbicide applications, could limit translocation of dicamba and therefore reduce the degree of auxin symptomology.
Before the commercialization of glyphosate-resistant (GR) soybean, acetolactate synthase (ALS)- and PPO-inhibiting herbicides were commonly utilized in POST applications for broadleaf weed control in soybean. With ALS and GR weed populations increasing as a result of extensive use of those SOAs, PPO-inhibiting herbicides became an essential chemical alternative for producers managing those resistant weed populations (Legleiter et al. Reference Legleiter, Bradley and Massey2009).
Synthetic auxin herbicides such as 2,4-D and dicamba have been used for decades because of their effectiveness in controlling broadleaf weeds (Egan and Mortensen Reference Egan and Mortensen2012). In addition to broadleaf control, synthetic auxin herbicides appeal to growers due to the present lack of effective SOAs for managing herbicide-resistant weeds (Sterling and Hall Reference Sterling and Hall1997).
PPO-inhibiting herbicides are highly effective when used for both PRE and POST applications but may result in crop injury with unfavorable environmental conditions. Symptomology of labeled POST-applied PPO-inhibiting herbicides for use in soybean, such as acifluorfen or lactofen, often results in transient necrotic lesions on trifoliates with minor leaf crinkling (Aulakh et al. Reference Aulakh, Chahal and Jhala2016). Although contact herbicides such as glufosinate and acifluorfen do not share the same SOA, both initiate cell membrane disruption and therefore have the potential to reduce dicamba uptake and translocation, which may help to counteract reduced rates of dicamba in a tank-contamination scenario. Additionally, antagonism between a contact and a systemic herbicide may be mitigated through sequential timing of applications. Antagonism between mixtures of paraquat and amitrole can be alleviated by utilizing sequential applications instead of a mixture (Putnam and Ries Reference Putnam and Ries1967). According to what is known regarding herbicide antagonism and synergism, activity of dicamba may be lessened or intensified depending on the duration and timing of exposure relative to a contact herbicide application due to the physiological nature of systemic and contact herbicides. However, little to no research has addressed the interaction of labeled contact herbicides, such as PPO and glutamine synthetase-inhibiting herbicides, and dicamba during an off-target exposure on sensitive soybean. Contrary to documented antagonism between contact and systemic herbicides, experiments conducted by Kelley et al. (Reference Kelley, Wax, Hager and Riechers2005) revealed that labeled POST-applied herbicides in soybean synergistically interacted with reduced rates of dicamba. Furthermore, dicamba applied alone caused considerably more soybean injury than other common POST herbicides alone at field use rates (≈40% increase) and resulted in an 18% increase in auxin injury when combined with fomesafen at 330 g ai ha−1 at the V3 growth stage compared with dicamba alone.
With the introduction of the Roundup Ready 2 Xtend® soybean technology, injury from dicamba tank contamination has become common in non–dicamba resistant (non-DR) cultivars, especially for producers who utilize custom applicators (Soltani et al. Reference Soltani, Nurse and Sikkema2016). Dicamba residues also have a high affinity to be deposited and sequestered in spray application equipment, specifically hoses and screens, creating risks for growers choosing to plant both DR and dicamba-susceptible soybean cultivars (Cundiff et al. Reference Cundiff, Reynolds and Mueller2017). Commonly applied POST herbicides such as glyphosate and glufosinate act as effective tank cleaners due to the adjuvants within the formulation, often resulting in dicamba injury following a POST application as a result of the dissolved residues moving back into solution (Cundiff et al. Reference Cundiff, Reynolds and Mueller2017). Non-DR cultivars have sustained yield losses up to 18% following exposure to a 1% tank-contamination rate of dicamba, assuming 560 g ae ha−1 as the labeled rate (Griffin et al. Reference Griffin, Bauerle, Stephenson, Miller and Boudreaux2013). Dicamba injury poses greater risk to reproductively growing soybean in comparison to vegetative soybean (Solomon and Bradley Reference Solomon and Bradley2014); however, research has shown yield loss associated with dicamba exposure to V3 soybean when dicamba was combined with other POST herbicides (Kelley et al. Reference Kelley, Wax, Hager and Riechers2005). Dicamba applied to sensitive soybean at 5.6 g ae ha−1 (1% of labeled use rate) at the V3 growth stage reduced grain yield up to 34%. Because soybean sensitivity to dicamba is high relative to other dicotyledon (dicot) crops such as cotton (Gossypium hirsutum L.) (Egan et al. Reference Egan, Barlow and Mortensen2014), POST applications of dicamba in-crop can present a major concern, especially with the introduction of Xtend® cropping systems. Extensive research has documented that dicamba exposure during late vegetative or early reproductive growth stages can lead to soybean yield loss (Auch and Arnold1978; Scholtes et al. Reference Scholtes, Sperry, Reynolds and Irby2019; Wax et al. Reference Wax, Knuth and Slife1969). According to the Roundup PowerMax® label, glyphosate may be applied until reproductive growth stage three (R3). An application at R3 could negatively affect yield if tank contamination by dicamba occurred (Anonymous 2012). Reduced rates of dicamba (0.75, 1.5, 3, 6, 15, 30, and 60 g ae ha−1) applied in combination with glyphosate (1,260 g ae ha−1) on both V3 and R1 soybean demonstrated significant injury and yield loss despite greater auxin symptomology on V3 applications (Soltani et al. Reference Soltani, Nurse and Sikkema2016).
Research has been conducted looking at the impact dicamba exposure has on injury, yield, and yield components of sensitive soybean cultivars; however, few have addressed whether commonly applied contact herbicides that are labeled in soybean can interact with dicamba exposure via tank contamination or through volatilization, especially in geographies where contamination would be a concern. In 2017, with the launch and extensive use of dicamba in Xtend soybean production systems, dicamba-like symptoms were commonly observed on soybean, and the severity of the injury often appeared to be greater on non-DR soybean treated with a labeled, POST contact herbicide, such as PPO inhibitors or glufosinate (JK Norsworthy, personal observation). This observation led to research aimed at evaluating the impact dicamba tank contamination or exposure could have on a sensitive soybean cultivar when applied with labeled contact herbicides or when soybean exposure to sublethal rates of dicamba occurs near the V3 timing of a glufosinate application.
Materials and Methods
Common Methodology
Experiments were conducted at the Milo J. Schult Agricultural Research and Education Center in Fayetteville, AR, on a Captina silt loam (fine-silty, siliceous, active, mesic Typic Fragiudults) composed of 14% sand, 76% silt, 10% clay, and 4.5% organic matter with a pH of 6.5 in 2018 (36.09278°N, 94.16667°W), and a Leaf silt loam (fine, mixed, active, thermic Typic Albaquults) with 34% sand, 53% silt, 13% clay, and 1.5% organic matter with a pH of 6.2 in 2019 (36.09528°N, 94.17306°W). Before planting, the field was prepared with a disk followed by a hipper, which formed 91-cm-wide beds for planting. An indeterminate, medium/tall, 4.7 maturity group glufosinate-resistant soybean cultivar (‘CZ 4748LL’, BASF Corporation, Research Triangle Park, NC 27709) was planted on May 9, 2018, and May 15, 2019, at 360,000 seeds ha−1. Trials consisted of plots measuring 7.6-m-long by 1.8-m-wide (two rows wide) with a nontreated row on either side of the plot. Alleys 3 m in width along with spray shields during application were implemented to minimize risk of dicamba movement onto adjacent plots via physical drift. Both trials received a broadcast PRE application of flumioxazin (Valent Corporation, Walnut Creek, CA 94596) at 70 g ai ha−1 plus pyroxasulfone (BASF Corporation) at 90 g ai ha−1 at planting. Additional herbicide applications of glufosinate (BASF Corporation) at 656 g ai ha−1 and S-metolachlor (Syngenta, Greensboro, NC 27419) at 1,312 g ai ha−1 were post-directed to row middles to avoid foliar contact with soybean, and mechanical weeding and hand weeding were incorporated until complete canopy coverage was achieved. In weeks that 2.5 cm of rainfall did not occur, trials were furrow irrigated in 2018 and overhead irrigated in 2019 to simulate standard production practices and create a high-yielding environment. The test sites each year were fertilized based on soil test recommendations for soybean (Slaton et al. Reference Slaton, Roberts and Ross2013) and amended with plant essential nutrients based on soil test results acquired from each location.
Treatments were applied with a CO2-pressurized backpack sprayer calibrated to deliver 140 L ha−1 at 4.8 km h−1 equipped with four AIXR 110015 flat-fan nozzles (TeeJet Technologies, Springfield, IL 62703). Visible injury to soybean was rated by comparing the two treated rows to the adjacent two nontreated rows on both sides of each plot using the Behrens and Lueschen scale for dicamba injury scoring from 0% to 100% at 21 and 28 d after treatment (DAT), which primarily focused on dicamba damage to terminal growth (Behrens and Lueschen Reference Behrens and Lueschen1979; Egan and Mortensen Reference Egan and Mortensen2012) (Table 1). Soybean canopy height was measured for three plants within each plot at 21 and 28 DAT. The date of soybean maturity was recorded when 95% of the soybean pods within the treated area reached a mature color. Subsequently, both rows of each plot were harvested using a small-plot combine (ALMACO, Nevada, IA 50201), and grain yield was determined by adjusting the harvested weights to 13% moisture. Additionally, a grain subsample was collected from the combine during harvest and later used to determine 100-seed weight. Weather data were recorded for each treatment application for both experiments in 2018 and 2019 (Table 2).
Table 1. Behrens and Lueschen (Reference Behrens and Lueschen1979) soybean dicamba injury index.
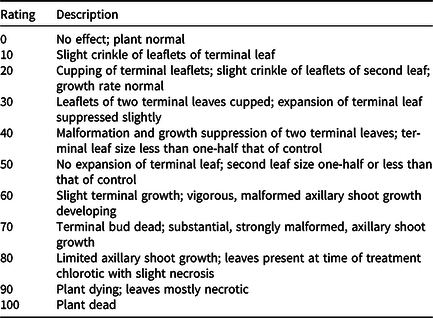
Table 2. Environmental parameters for 2018 and 2019 field applications in Fayetteville, AR.

a Tank contamination = dicamba tank-contamination experiment; timing of exposure = timing of dicamba exposure experiment.
b DATV3, days after the V3 treatment.
Dicamba Tank-Contamination Experiment
The experiment was arranged as a three-factor factorial randomized complete block design with four replications, with the first factor being site-year. The second factor represented the labeled rates of POST herbicides: glufosinate alone at 656 g ai ha−1, acifluorfen (UPL, King of Prussia, PA 19406) alone at 560 g ai ha−1, a glufosinate plus acifluorfen mixture at their respective labeled rates, or no additional herbicide. The third factor was four levels of simulated tank-contamination rates of the Engenia® (BASF Corporation) formulation of dicamba at 0, 0.056, 0.56, and 5.6 g ae ha−1 with a 1X rate being 560 g ae ha−1 for POST application in DR soybean (Anonymous 2018). All treatments were applied when soybean reached the V3 growth stage.
Timing of Dicamba Exposure Experiment
The experiment was arranged as a two-factor randomized complete block design with four replications, considering site-year and timing of dicamba exposure as fixed effects. Dicamba was applied at 2.8 g ae ha−1 at three growth stages before glufosinate at the V3 growth stage, in combination with glufosinate at the V3 growth stage, and at three subsequent timings following the glufosinate application. These dicamba application timings included: cotyledon, V1, V2, and V3 stage of soybean growth and 3, 7, and 10 d after the V3 treatment (DATV3). Visible auxin injury to soybean was assessed 21 d after the glufosinate application at the V3 growth stage (Behrens and Lueschen Reference Behrens and Lueschen1979) (Table 1).
Statistical Analysis
All normally distributed data for both experiments (soybean height, maturity, 100-seed weight, and yield) were analyzed with JMP Pro v. 14.3 (SAS Institute, Cary, NC 27513) using the Fit Model Function. All injury data were not normally distributed, and therefore a beta distribution was assumed. Injury data relative to the nontreated were analyzed with SAS v. 9.4 using PROC GLIMMIX (Gbur et al. Reference Gbur, Stroup, McCarter, Durham, Young, Christman, West and Kramer2012). For the timing of dicamba exposure experiment, contrasts were constructed with SAS under the same assumptions of the beta distribution. For the tank-contamination experiment, site-year, herbicide, and dicamba rate were considered fixed effects in the model, while block (replication) was considered a random effect. For the timing of dicamba experiment, site-year and dicamba timing were considered fixed effects, and block was considered a random effect. All data were subject to ANOVA, and means were separated using Fisher’s protected LSD (α = 0.05).
Results and Discussion
Dicamba Tank-Contamination Experiment
An interaction between site-year and dicamba rate was observed at 21 and 28 DAT (Table 2). Further analysis indicated that greater dicamba injury was observed in 2018 than in 2019, and an increase in dicamba rate likewise resulted in an increase in auxin injury observed for both site-years (Tables 3 and 4). The difference in severity of visible injury among site-years could potentially be a result of variable weather conditions from 2018 to 2019 (Table 2). The presence of soil moisture, irrigation events, or fluctuations in temperature all have the capacity to influence the activity of dicamba (Al-Khatib and Peterson Reference Al-Khatib and Peterson1999; Grantz et al. Reference Grantz, Lee, Willett and Norsworthy2020; Willett et al. Reference Willett, Grantz, Lee, Thompson and Norsworthy2018). Auxin symptomology elicited by tank-contamination rates of dicamba appeared in newer, vegetative trifoliates, ultimately causing leaf cupping, stunting, stacking of nodes, and chlorosis (Table 1).
Table 3. The P-values for soybean dicamba injury, height, maturity, 100-seed weight, and yield from 2018 and 2019 in Fayetteville, AR. a
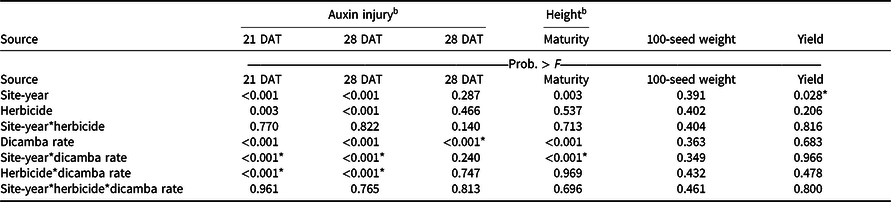
a P-values within columns denoted by asterisks indicate significance. Interactions take precedence over main effects.
b DAT, days after treatment.
Table 4. Interaction of site-year and dicamba rate averaged over contact herbicide on soybean auxin injury and maturity in 2018 and 2019 at Fayetteville, AR. a
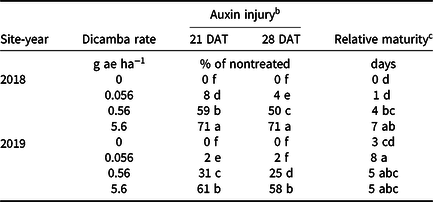
a Means within a column followed by the same letter are not different according to Fisher’s protected LSD (α = 0.05).
b DAT, days after treatment.
c Data expressed as days delayed relative to the nontreated.
The combination of contact herbicides with dicamba exacerbated the extent of auxin injury compared with dicamba alone (Figures 1 and 2; Tables 3 and 5). Glufosinate alone, acifluorfen alone, and the mixture of the two herbicides resulted in 7%, 18%, and 21% injury to soybean, respectively, without the addition of dicamba when averaged across site-years (data not shown). POST injury is common from labeled applications of PPO-inhibiting herbicides such as acifluorfen, fomesafen, and lactofen on soybean (Aulakh et al. Reference Aulakh, Chahal and Jhala2016). Labeled rates of glufosinate plus acifluorfen applied as a mixture with dicamba at 5.6 g ae ha−1 caused a 20% and 28% increase in auxin symptomology at 21 and 28 DAT, respectively, as compared with dicamba applied alone at 5.6 g ae ha−1 (Table 5). Similar results were documented with acifluorfen and glufosinate alone when applied with dicamba at 5.6 g ae ha−1, suggesting that the presence of contact herbicides may increase the severity of visible auxin symptomology on soybean, which is consistent with research conducted by Kelley et al. (Reference Kelley, Wax, Hager and Riechers2005). Although antagonism is routinely observed on weed species when contact herbicides are combined with a systemic herbicide such as glufosinate and clethodim or glufosinate and dicamba (Burke et al. Reference Burke, Askew, Corbett and Wilcut2005; Meyer and Norsworthy Reference Meyer and Norsworthy2020), a glufosinate-resistant soybean cultivar will not show contact symptomology of either glufosinate or acifluorfen to the extent that a weed will. When dicamba was applied at the lower rates of 0.056 and 0.56 g ae ha−1, there was less consistency with the enhanced auxin injury phenotype. Glufosinate mixed with dicamba at 0.056 g ae ha−1 resulted in increased auxin injury at both 21 and 28 DAT, and dicamba at 0.56 g ae ha−1 resulted in increased auxin injury at 28 DAT. When acifluorfen alone or the mixture of acifluorfen and glufosinate were combined with dicamba at 5.6 g ae ha−1, increased auxin injury was observed, however; this trend was not evident at lower contamination rates of dicamba, similar to what was observed with dicamba contamination of glufosinate (Table 5).
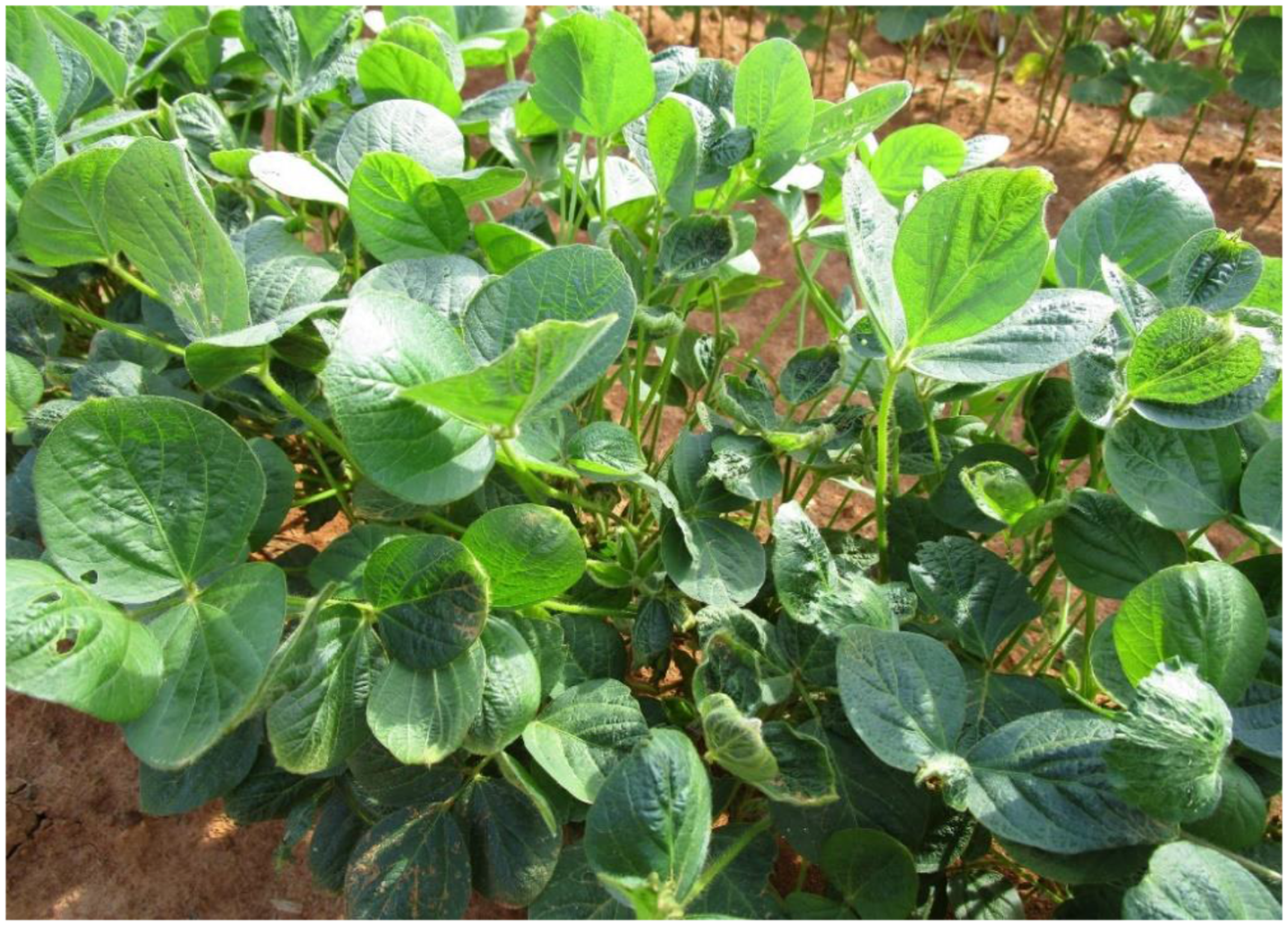
Figure 1. Synthetic auxin symptomology associated with a dicamba application alone at 5.6 g ae ha−1 28 d following a V3 application to soybean in Fayetteville, AR, in 2018.
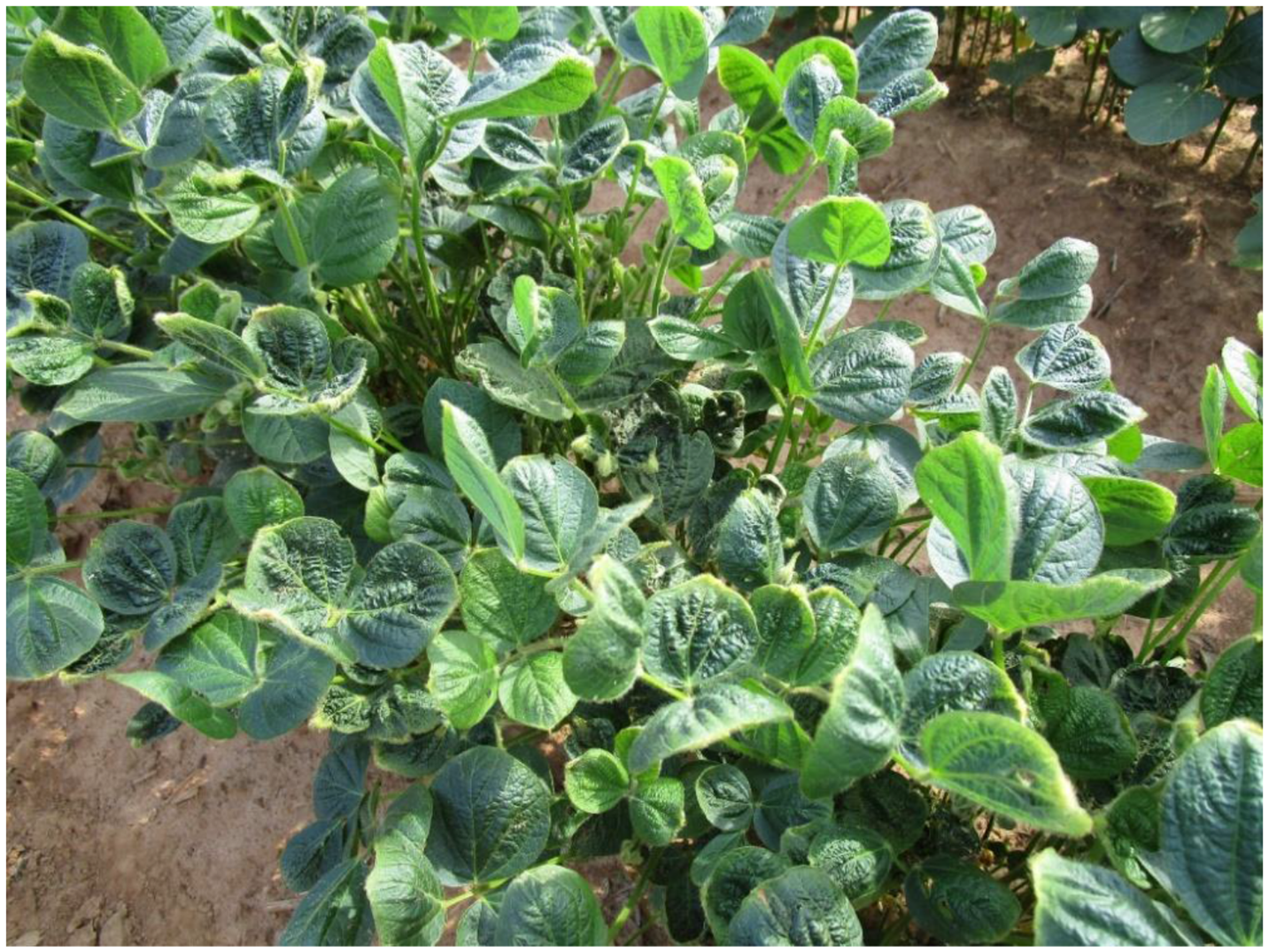
Figure 2. Synthetic auxin symptomology associated with a contamination rate of 5.6 g ae ha−1 dicamba in combination with 656 and 560 g ai ha−1 glufosinate and acifluorfen, respectively, 28 d following a V3 application to soybean in Fayetteville, AR, in 2018.
Table 5. Interaction of herbicide and dicamba rate averaged over site-year on soybean auxin injury from 2018 and 2019 at Fayetteville, AR. a

a Means within a column followed by the same letter are not different according to Fisher’s protected LSD (α = 0.05).
b DAT, days after treatment.
Despite increased auxin symptomology resulting from mixing dicamba with contact herbicides, penalties to soybean height were only a function of dicamba rate (Table 3). Soybean height of plants treated with dicamba at 5.6 g ae ha−1 was reduced by 30% compared with plots treated with acifluorfen, glufosinate, or a combination (Table 6). In general, and as seen in other research, foliar auxin symptomology was more apparent than height reductions (Solomon and Bradley Reference Solomon and Bradley2014). Previous research has shown that reductions in soybean height often result in yield loss (Weidenhamer et al. Reference Weidenhamer, Triplett and Sobotka1989).
Table 6. Soybean height at 28 d after treatment averaged over site-year and contact herbicide from 2018 and 2019 at Fayetteville, AR. a
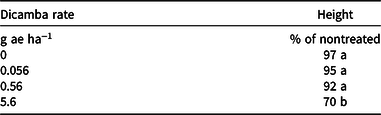
a Means within a column followed by the same letter are not different according to Fisher’s protected LSD (α = 0.05).
Increases in auxin symptomology from the addition of contact herbicides did not translate to a significant maturity delay. Instead, maturity delays were a function of dicamba rate (Tables 3 and 4). In 2018, a consistent increase in delay of maturity was observed with dicamba at 0.56 and 5.6 g ae ha−1, with a 4- and 7-d delay in maturity relative to plots treated with only acifluorfen, glufosinate, or a combination. Soybean maturity in 2019 was more variable (Table 4). Solomon and Bradley (Reference Solomon and Bradley2014) documented a 5- to 8-d maturity delay when dicamba was applied at V3 to soybean at 28 g ae ha−1, whereas the same rate caused a 23- to 26-d delay with an R2 application. However, soybean injury, height reductions, and maturity delays resulting from dicamba tank contamination did not adversely impact grain yield, with the only difference being a 350 kg ha−1 increase in yield from 2018 to 2019 (data not shown). Behrens and Lueschen (Reference Behrens and Lueschen1979) determined that dicamba injury to soybean of approximately 60% to 70% from a single vegetative exposure may impact yield; however, the amount of visible dicamba injury observed in these irrigated experiments was not enough to reduce yield. Other research has shown that soybean subjected to reduced rates of dicamba at early growth stages can counteract synthetic auxin injury by producing more pods and mitigating yield loss (Wax et al. Reference Wax, Knuth and Slife1969). Another explanation of why yield loss was not observed could be that soybean has been documented to combat abiotic stresses by compensating one yield component for another, with higher rates of dicamba exposure encouraging greater lateral growth (Kelley et al. Reference Kelley, Wax, Hager and Riechers2005; Robinson et al. Reference Robinson, Simpson and Johnson2013).
Timing of Dicamba Exposure Experiment
Visible dicamba injury to soybean typically persists for approximately 28 d, with injury peaking 14 to 21 d after exposure (Al-Khatib and Peterson Reference Al-Khatib and Peterson1999). At 21 DAT, an interaction between site-year and dicamba application timing occurred with respect to visible soybean injury from dicamba, with environmental data at application potentially explaining some variability (Tables 2 and 7). In 2019, auxin injury and height reductions from the V2 dicamba exposure were more apparent than the surrounding V1 and V3 dicamba exposures compared with what was observed in 2018 (Table 8). Auxin injury to soybean caused by dicamba may increase as a result of an irrigation event following exposure, allowing an opportunity for foliar and root uptake of the herbicide (Willett et al. Reference Willett, Grantz, Lee, Thompson and Norsworthy2018). Weather data were not assessed in this experiment but could potentially be an environmental parameter responsible for differences in auxin injury in 2018 and 2019. Based on contrasts, applications where dicamba preceded glufosinate resulted in less visible injury than treatments where dicamba followed glufosinate (35% and 56%, respectively). A dicamba plus glufosinate mixture was less injurious as well compared with treatments in which dicamba followed glufosinate (45% and 56%, respectively).
Table 7. The P-values with site-year considered as a fixed-effect from ANOVA for soybean dicamba injury at 21 d after treatment, height at 21 d after treatment, maturity, 100-seed weight, and yield from 2018 and 2019 at Fayetteville, AR. a
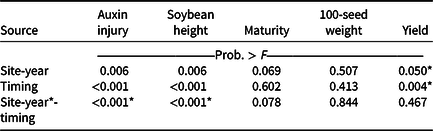
a P-values within columns denoted by asterisks indicate significance. Interactions take precedence over main effects.
Table 8. Interaction of site-year and dicamba timing on soybean auxin injury and height 21 d after treatment as well as contrasts for dicamba injury and soybean height when glufosinate was preceded, combined, or followed by dicamba exposure from 2018 and 2019 in Fayetteville, AR. a
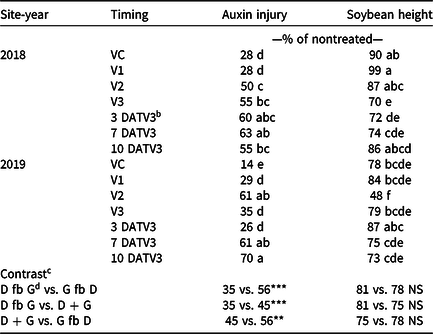
a Means within a column followed by the same letter are not different according to Fisher’s protected LSD (α=0.05).
b Abbreviation: DATV3, time of dicamba exposure in days after glufosinate applied at the V3 stage of soybean days after treatment V3
c Contrasts: * significant (P < 0.05); ** significant (P < 0.01); *** significant (P < 0.001); NS, nonsignificant (P ≥ 0.05).
d Abbreviations: D, dicamba; fb, followed by; G, glufosinate.
The antagonistic effects of mixtures of glufosinate and systemic herbicides such as dicamba or 2,4-D vary among species (Merchant et al. Reference Merchant, Sosnoskie, Culpepper, Steckel, York, Braxton and Ford2013). However, metabolism of glufosinate when applied to a glufosinate-resistant soybean cultivar would likely eliminate the role of the contact herbicide in causing antagonism between contact and systemic herbicides. The compounding stress from metabolism of both glufosinate and dicamba on a glufosinate-resistant soybean cultivar may explain the increased dicamba symptomology associated with a dicamba exposure preceded by a glufosinate application or when dicamba and glufosinate are applied as a mixture.
Across all treatments, soybean grain yield improved by 470 kg ha−1 (10%) from 2018 to 2019 (data not shown). Despite multiple treatments injuring soybean beyond 50% relative to the nontreated, dicamba exposure at 7 and 10 DATV3 (V5 to V6 soybean) were the only treatments to reduce yield, possibly due to soybean approaching reproductive development (Table 9). Overall, soybean is more likely to recover from early-season than late-season dicamba exposure (Auch and Arnold Reference Auch and Arnold1978; Slife Reference Slife1956; Solomon and Bradley Reference Solomon and Bradley2014; Wax et al. Reference Wax, Knuth and Slife1969).
Table 9. Soybean yield averaged over site-year from 2018 and 2019 at Fayetteville, AR. a
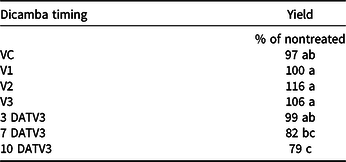
a Means within a column followed by the same letter are not different according to Fisher’s protected LSD (α=0.05).
bAbbreviation: DATV3, time of dicamba exposure in days after glufosinate applied at the V3 stage of soybean.
Practical Implications
Producers have the luxury of planting a multitude of soybean cultivars with various herbicide-resistance traits to combat an ever-evolving weed spectrum, sometimes with resistance to several herbicide sites of action (Anonymous 2020; Heap Reference Heap2020). Fortunately, many producers are still able to rely on contact herbicides such as glufosinate and fomesafen (where PPO-inhibiting herbicide resistance is not present) to effectively control Palmer amaranth. Unfortunately, adoption of DR soybean for control of problematic broadleaf weeds in some geographies has presented new challenges for producers choosing to plant non-DR technologies. In these geographies, frequent off-target movement of dicamba leads to symptomology on non-DR soybean because of the sensitivity of soybean to extremely low doses (Auch and Arnold Reference Auch and Arnold1978; Egan et al. Reference Egan, Barlow and Mortensen2014; Jones Reference Jones2018; Solomon and Bradley Reference Solomon and Bradley2014).
Findings from the tank-contamination experiment indicate that dicamba contamination in a sprayer or off-target movement of the herbicide onto soybean has the potential to interact with POST contact herbicides applied directly to the crop, leading to greater dicamba symptomology on soybean than from a dicamba exposure alone. Although yield was not negatively affected by visible injury from a single exposure of dicamba with or without the addition of contact herbicides, the increased injury could slow canopy development (Priess et al. Reference Priess, Norsworthy, Roberts and Spurlock2020) and require an additional POST herbicide application to maintain a high level of weed control. Multiple exposures of dicamba to sensitive soybean cultivars can prolong auxin injury and ultimately delay maturity, particularly when exposure to dicamba occurs in conjunction with contact herbicides. Based on findings from the timing of the dicamba exposure experiment, soybean exposed to dicamba at or following a glufosinate application will likely elicit greater dicamba symptomology than a prior exposure, with yield reductions more likely to occur from late-season dicamba exposure, as seen in other research (Auch and Arnold Reference Auch and Arnold1978; Slife Reference Slife1956; Solomon and Bradley Reference Solomon and Bradley2014; Wax et al. Reference Wax, Knuth and Slife1969).
To expand upon the interaction of contact herbicides and dicamba, supplementary tank-contamination research could be beneficial by focusing on the influence of dicamba exposure with flumioxazin, a commonly applied PRE PPO-inhibiting herbicide in soybean. With many producers in the Midsouth having simultaneous cotton and soybean acres, there is potential for flumioxazin residue to remain in the sprayer when applying POST herbicides in cotton and soybean. Based on this research, the combination of dicamba and flumioxazin could amplify auxin symptomology.
Acknowledgments
Facilities and equipment for this research were provided by the University of Arkansas System Division of Agriculture. No conflicts of interest have been declared.