Introduction
The herbicides that inhibit 4-hydroxyphenylpyruvate dioxygenase (HPPD) are commonly used for postemergence (POST) weed control in corn. Herbicides that inhibit the HPPD enzyme control susceptible species by blocking the conversion of 4-hydroxyphenylpyruvic acid to homogentisic acid, which ultimately depletes the plant of plastoquinone and tocopherols (Lindblad et al. Reference Lindblad, Lindstedt and Lindstedt1970; Matsumoto et al. Reference Matsumoto, Mizutani, Yamaguchi and Kadotani2002; Schulz et al. Reference Schulz, Ort, Beyer and Kleinig1993; Secor Reference Secor1994; Trebst et al. Reference Trebst, Depka and Holländer-Czytko2002; Tsegaye et al. Reference Tsegaye, Shintani and DellaPenna2002). Plastoquinone is an essential electron acceptor in the photosynthetic electron transport chain and is also a cofactor for the phytoene desaturase enzyme, which is responsible for the production of carotenoids (Ahrens et al. Reference Ahrens, Lange, Müller, Rosinger, Willms and van Almsick2013; Hess Reference Hess2000; Norris et al. Reference Norris, Barrette and DellaPenna1995; Pallett et al. Reference Pallett, Little, Sheekey and Veerasekaran1998; Schulz et al. Reference Schulz, Ort, Beyer and Kleinig1993). The depletion of carotenoids, plastoquinone, and tocopherols limits the ability of a plant to quench reactive oxygen species (ROS) that eventually destroy cell components, causing cell and plant death under continued sunlight exposure (Ahrens et al. Reference Ahrens, Lange, Müller, Rosinger, Willms and van Almsick2013; Krieger-Liszkay Reference Krieger-Liszkay2005; Kruk et al. Reference Kruk, Holländer-Czytko, Oettmeier and Trebst2005; Lee et al. Reference Lee, Prisbylla, Cromartie, Dagarin, Howard, Provan, Ellis, Fraser and Mutter1997; Siefermann-Harms Reference Siefermann-Harms1987).
Herbicides such as atrazine that inhibit the photosystem II (PS II) protein complex are commonly applied in a mixture with HPPD inhibitors to improve weed control (Armel et al. Reference Armel, Hall, Wilson and Cullen2005; Chahal and Jhala Reference Chahal and Jhala2018; Chahal et al. Reference Chahal, Jugulam and Jhala2019; Johnson et al. Reference Johnson, Young and Matthews2002; Kohrt and Sprague Reference Kohrt and Sprague2017; Whaley et al. Reference Whaley, Armel, Wilson and Hines2006; Willemse et al. Reference Willemse, Soltani, Hooker, Jhala, Robinson and Sikkema2021a; Williams et al. Reference Williams, Boydston, Peachey and Robinson2011; Woodyard et al. Reference Woodyard, Bollero and Riechers2009). The PS II inhibitors compete with plastoquinone for the QB binding niche on the D1 protein of the photosynthetic electron transport chain (Hess Reference Hess2000). The displacement of plastoquinone causes a buildup of energy that ultimately yields triplet chlorophyll and singlet oxygen, which cause lipid peroxidation in cells as the quenching capabilities of the antioxidant system becomes overloaded (Hess Reference Hess2000; Krieger-Liszkay Reference Krieger-Liszkay2005). The complementary modes of action of HPPD and PS II inhibitors could be a reason why weed control is improved with the addition of a PS II inhibitor to an HPPD inhibitor (Abendroth et al. Reference Abendroth, Martin and Roeth2006; Armel et al. Reference Armel, Hall, Wilson and Cullen2005; Creech et al. Reference Creech, Monaco and Evans2004). The complementary activity between HPPD and PS II inhibitors occurs because 1) the HPPD inhibitor increases the efficiency of PS II inhibitor binding to the D1 protein by depleting the plant of plastoquinone; and 2) the HPPD inhibitor depletes the plant of several antioxidants, whereas the PS II inhibitor causes an influx of ROS, which intensifies lipid peroxidation (Abendroth et al. Reference Abendroth, Martin and Roeth2006; Armel et al. Reference Armel, Hall, Wilson and Cullen2005; Creech et al. Reference Creech, Monaco and Evans2004; Kim et al. Reference Kim, Jung, Hwang and Cho1999).
Label recommendations for mesotrione, topramezone, tembotrione, and isoxaflutole specify that 278, 500, 576, and 800 to 1,063 g ai ha−1 of atrazine, respectively, is to be mixed with these HPPD inhibitors to improve weed control (Anonymous 2018, 2020a, 2020b, 2021a). In contrast, the tolpyralate label does not explicitly define the dose of atrazine to mix with tolpyralate, and instead states that a minimum of 560 g ai ha−1 of atrazine is required to mix with 30 to 40 g ai ha−1 of tolpyralate (Anonymous 2021b). Metzger et al. (Reference Metzger, Soltani, Raeder, Hooker, Robinson and Sikkema2019b) reported that when tolpyralate at 30 or 40 g ai ha−1 was mixed with a constant dose of 1,000 g ai ha−1 of atrazine there were no differences in weed control of several weed species between the two tolpyralate doses. Metzger et al. (Reference Metzger, Soltani, Raeder, Hooker, Robinson and Sikkema2018a) documented that tolpyralate + atrazine (30 + 1,000 g ai ha−1) controlled eight weed species >90% and that control of wild mustard and ladysthumb were improved by the addition of atrazine to tolpyralate; however, the dose of atrazine used in the study was greater than the lowest labeled dose of atrazine to mix with tolpyralate. Langdon et al. (Reference Langdon, Soltani, Raeder, Robinson, Hooker and Sikkema2020) observed comparable control of the same weed species as Metzger et al. (Reference Metzger, Soltani, Raeder, Hooker, Robinson and Sikkema2018a) with tolpyralate + atrazine but at 560 g ai ha−1 of atrazine.
Numerous studies have reported dose responses of tolpyralate and tolpyralate + atrazine for the control of several weed species. Osipitan et al. (Reference Osipitan, Scott and Knezevic2018) determined that 11 to 17 g ai ha−1 of tolpyralate was required to achieve 90% control of several annual broadleaf weed species when mixed with 560 g ai ha−1 of atrazine. Metzger et al. (Reference Metzger, Soltani, Raeder, Hooker, Robinson and Sikkema2018b, Reference Metzger, Soltani, Raeder, Hooker, Robinson and Sikkema2019a) and Willemse et al. (Reference Willemse, Soltani, Metzger, Hooker, Jhala, Robinson and Sikkema2021b) determined the dose response of several weed species to tolpyralate (3.75 to 120 g ai ha−1) alone and mixed with atrazine (125 to 4,000 g ai ha−1) at a 1:33.3 ratio. The dose of tolpyralate needed to control velvetleaf, common lambsquarters, common ragweed, Amaranthus spp., and green foxtail was ≤15.5 g ai ha−1; however, the addition of atrazine to tolpyralate was required to obtain 90% control of wild mustard, barnyardgrass, and ladysthumb (Metzger et al. Reference Metzger, Soltani, Raeder, Hooker, Robinson and Sikkema2018b). For 90% control of all these weed species with tolpyralate + atrazine, the dose required was ≤13.1 + 436 g ai ha−1 (Metzger et al. Reference Metzger, Soltani, Raeder, Hooker, Robinson and Sikkema2018b). For 95% control of multiple herbicide–resistant Canada fleabane (Erigeron canadensis L.) and waterhemp [Amaranthus tuberculatus (Moq.) J. D. Sauer] the predicted dose of tolpyralate could not be calculated; however, when tolpyralate was mixed with atrazine the predicted dose of tolpyralate + atrazine to achieve 95% control of multiple herbicide–resistant Canada fleabane was 22.3 + 741.7 g ai ha−1 and for waterhemp the dose could not be computed (Metzger et al. Reference Metzger, Soltani, Raeder, Hooker, Robinson and Sikkema2019a; Willemse et al. Reference Willemse, Soltani, Metzger, Hooker, Jhala, Robinson and Sikkema2021b).
Although these studies provide valuable information on the efficacy of tolpyralate alone and with atrazine, the commercial use pattern of tolpyralate is at doses of 30 to 40 g ai ha−1, and it is generally recommended to co-apply at least 560 g ai ha−1 of atrazine unless one is in a geographic area where atrazine use is restricted or prohibited (Anonymous 2021b). Applying too low of a dose of atrazine to complement tolpyralate for the weed species present in a corn field may result in unacceptable weed control. Contrariwise, applying a dose of atrazine greater than required to control the suite of weed species in the field can result in increased environmental loading of atrazine and greater than necessary herbicide expenditures. Providing corn producers with the effective dose (ED) of atrazine required to control the suite of weed species in a field will provide growers with the information they need to select the lowest ED of atrazine required to complement tolpyralate. Therefore, the objective of this study was to identify the ED of atrazine to complement 30 g ai ha−1 of tolpyralate for the control of several common annual broadleaf and grass weed species in corn.
Materials and Methods
Five conventionally tilled field trials were conducted during three field seasons (2019, 2020, and 2021) at University of Guelph research sites in Ontario, Canada: in Ridgetown (Ridgetown Campus; 42.45ºN, 81.88ºW), and near Exeter (Huron Research Station; 43.32ºN, 81.50ºW). Fertilization was completed prior to corn planting to meet corn requirements. Corn was planted in rows spaced 75 cm apart to a depth of 5 cm at a population of approximately 85,000 seeds ha−1. At the Huron Research Station, DKC44-13RIB® (Bayer CropScience Canada Inc., Calgary, AB, Canada) was planted in 2019, while in 2020 and 2021, DKC42-04RIB® was planted. At Ridgetown Campus, DKC42-60RIB® was planted in 2020 and DKC39-97RIB® was planted in 2021. Plots were 10 m long at the Huron Research Station and 8 m long at Ridgetown Campus. Plots were 3 m wide (four corn rows) at both locations. Trials were established using a randomized complete block design with four replications. A nontreated control plot was present in each replication. Further specifics for the five field trials including soil information, corn planting, and harvest dates, herbicide application dates, and corn development stage at herbicide application are presented in Table 1.
Table 1. Field trial data.
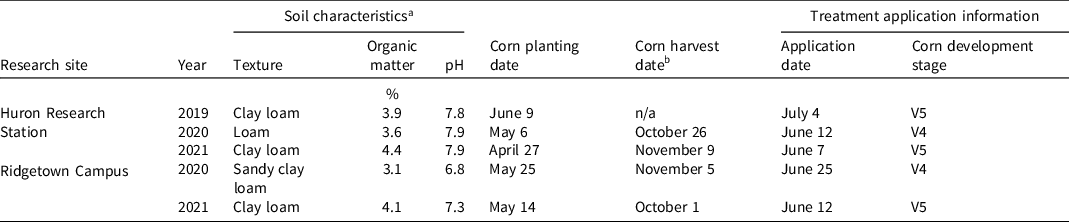
a Soil cores were taken to a depth of 15 cm, and subsequent analysis by A&L Canada Laboratories Inc. (London, ON, Canada) was used to determine soil characteristics.
b Corn was not harvested in 2019.
To determine the dose of atrazine (AAtrex® Liquid 480, 480 g ai L−1; Syngenta Canada Inc., Guelph, ON, Canada) required to complement tolpyralate (Shieldex® 400SC Herbicide, 400 g ai L−1; ISK Biosciences Corporation, Concord, OH, USA), 10 doses of atrazine (0, 50, 100, 200, 300, 400, 500, 750, 1,000, and 2,000 g ai ha−1) were mixed with a constant dose of 30 g ai ha−1 of tolpyralate. Methylated seed oil adjuvant (MSO Concentrate®; Loveland Products Inc., Loveland, CO, USA) was included in each treatment at 0.5% vol/vol. A CO2-pressurized backpack sprayer was used to spray herbicide treatments with a carrier volume of 200 L ha−1 at 240 kPa through four ULD120-02 spray nozzles (Pentair, New Brighton, MN, USA) at 50-cm spacing on the spray boom producing a spray width of 2 m. Treatments were applied POST to 15-cm-tall weed canopies. Seven weed species or genera were analyzed and present among the five environments: velvetleaf (two environments; average density 4 plants m−2), redroot pigweed (five environments; average density 13 plants m−2), common ragweed (five environments; average density 43 plants m−2), common lambsquarters (five environments; average density 58 plants m−2), wild mustard (three environments; average density 82 plants m−2), barnyardgrass (five environments; average density 81 plants m−2), and Setaria spp. (five environments; average density 57 plants m−2). Setaria spp. consisted of a heterogeneous population of green foxtail and giant foxtail and were grouped as one entity for data collection and analysis. All the weed species evaluated in this study except wild mustard are ranked among the top five for being either the most common and/or troublesome weed species for corn production in at least one U.S. state or one Canadian province (Van Wychen Reference Van Wychen2020).
Visible weed control by species was assessed 2, 4, and 8 wk after application (WAA) by visually comparing the aboveground biomass of each plot to the nontreated control and assigning a percent score ranging from 0% (no control) to 100% (complete control). Visible corn injury was recorded at 1, 2, and 4 WAA as an estimation of corn injury relative to the nontreated control on a percentage score of 0% to 100% with 0% representing no corn injury and 100% complete corn death. Weed control and corn injury assessments at 2 WAA were not completed at the Huron Research Station in 2019. Density determination for each weed species occurred 8 WAA by counting the number of weeds in two randomly placed 0.5-m2 quadrats in each plot. The aboveground biomass of the counted weeds was placed in paper bags separated by weed species then dried in a kiln drier to constant moisture for dry weight ascertainment. A small-plot combine was used to harvest the center two rows of each plot when the corn reached harvest maturity. Corn grain yields were corrected to 15.5% moisture prior to statistical analysis. Corn was not harvested from the Huron Research Station in 2019 because corn did not establish well due to wet planting conditions and drought during the growing season.
Statistical Analysis
Weed control at 2, 4, and 8 WAA was regressed against atrazine dose using the NLIN procedure in SAS software (v. 9.4; SAS Institute Inc., Cary, NC, USA) with either an exponential to a maximum equation (Equation 1) or with a log-logistic dose-response equation (Equation 2) to determine the ED of atrazine to complement tolpyralate for 80%, 90%, and 95% control. Weed density (plants m−2) and dry biomass (g m−2) data were regressed against atrazine dose using an inverse exponential equation (Equation 3) to determine the ED of atrazine to complement tolpyralate for 80%, 90%, and 95% reduction in density and dry biomass relative to the nontreated control. Grain corn yield (kg ha−1) was regressed against atrazine dose using the exponential to a maximum equation (Equation 1) to predict the ED of atrazine to complement tolpyralate for 80%, 90%, and 95% yield of the upper asymptote, a, of the equation. When an ED could not be computed or was beyond the doses used in this study, an em dash (—) is used in the tables. It would be improper to extrapolate an ED beyond the doses used in this study. Since tolpyralate applied alone was the 0 g ai ha−1 dose of atrazine, some EDs could not be computed because tolpyralate applied alone elicited a response (weed control or dry biomass reduction) >80% in the absence of atrazine. The following three equations for nonlinear regression analysis were used for this study.
Equation 1, exponential to a maximum:

where y = response parameter, a = upper asymptote, b = magnitude constant, e = Euler’s number, and c = slope.
Equation 2, log-logistic dose response:

where y = response parameter, C = lower asymptote, D = upper asymptote, e = Euler’s number, I 50 = dose for 50% response, and b = slope about I 50.
Equation 3, inverse exponential:

where y = response parameter, a = lower asymptote, b = reduction in y from intercept to a, e = Euler’s number, and c = slope from intercept to a.
Results and Discussion
Weed Control
The weed species or genera evaluated in this study varied in the predicted ED values of atrazine required to complement 30 g ai ha−1 of tolpyralate for the various response parameters.
Regression analysis indicated that 2 WAA, all seven weed species were 80% controlled with atrazine doses of ≤1,346 g ai ha−1 when co-applied with 30 g ai ha−1 of tolpyralate (Table 2). The broadleaf weed species required comparatively lower doses of atrazine compared to the grass weed species to achieve 80% control. Similarly, Metzger et al. (Reference Metzger, Soltani, Raeder, Hooker, Robinson and Sikkema2018b) reported that to control 10-cm weeds 2 WAA, compared to broadleaf weed species, green foxtail and barnyardgrass required comparatively greater tolpyralate + atrazine (1:33.3 ratio) doses to achieve 80% and 90% control. The broadleaf weed species required atrazine doses of 137 to 296 g ai ha−1, which is less than the label dose of 560 g ai ha−1 for 80% control. Barnyardgrass and Setaria spp. required atrazine doses >560 g ai ha−1 to achieve 80% control, and no doses of atrazine could be predicted for 90% and 95% control of these grass weed species. Similarly, Metzger et al. (Reference Metzger, Soltani, Raeder, Hooker, Robinson and Sikkema2019b) did not achieve >90% control of 20-cm barnyardgrass and green foxtail with tolpyralate + atrazine (30/40 + 1,000 g ai ha−1) 2 WAA. Redroot pigweed and wild mustard required atrazine doses of 734 and 586 g ai ha−1, respectively, to obtain 90% control; however, velvetleaf, common ragweed, and common lambsquarters were 90% controlled with doses <560 g ai ha−1. Redroot pigweed was not controlled by 95% 2 WAA; however, the four other broadleaf weed species were 95% controlled with atrazine doses >560 g ai ha−1. Similarly, Willemse et al. (Reference Willemse, Soltani, Metzger, Hooker, Jhala, Robinson and Sikkema2021b) documented that an ED to achieve 95% waterhemp control could not be computed with tolpyralate + atrazine (1:33.3 ratio) in a dose response involving six doses of tolpyralate (3.75 to 120 g ai ha−1) and six doses of atrazine (125 to 4,000 g ai ha−1). Wild mustard required 1,434 g ai ha−1 of atrazine to achieve 95% control, whereas velvetleaf, common ragweed, and common lambsquarters were 95% controlled with ≤802 g ai ha−1 of atrazine. Compared with the other broadleaf weed species evaluated in this study, wild mustard is generally less responsive to tolpyralate applied alone, and, in previous studies, the addition of atrazine to tolpyralate markedly improved wild mustard control (Metzger et al. Reference Metzger, Soltani, Raeder, Hooker, Robinson and Sikkema2018a, Reference Metzger, Soltani, Raeder, Hooker, Robinson and Sikkema2018b).
Table 2. Nonlinear regression parameters and predicted atrazine dose to complement 30 g ai ha−1 of tolpyralate to achieve 80%, 90%, and 95% control of various weeds 2 wk after application from field trials in 2020 and 2021.
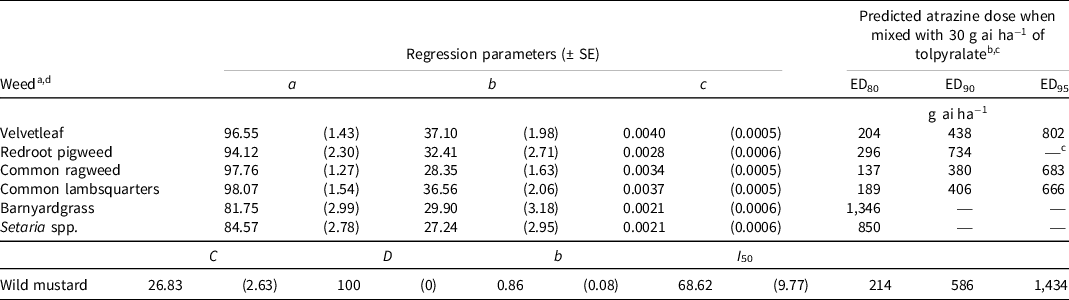
a Calculations for velvetleaf, redroot pigweed, common ragweed, common lambsquarters, barnyardgrass, and Setaria spp. were developed using Eq. 1, y = a – b [e (−c*dose)]; where y = response parameter, a = upper asymptote, b = magnitude constant, e = Euler’s number, and c = slope.
b EDn denotes the predicted effective dose of atrazine for percent control level n.
c An em dash (—) signifies that a predicted dose for percent control level n could not be computed.
d Calculations for wild mustard were developed using Eq. 2, y = C + (D – C)/{1 + e {−b*[log(dose) – log(I 50 )]}}; where y = response parameter, C = lower asymptote, D = upper asymptote, e = Euler’s number, I 50 = dose for 50% response, and b = slope about I 50.
At 4 WAA, the five broadleaf weed species were 80% controlled with ≤150 g ai ha−1 of atrazine when mixed with 30 g ai ha−1 of tolpyralate (Table 3). The predicted dose to achieve 80% common ragweed control could not be computed because tolpyralate applied alone provided >80% control of common ragweed, which is similar to that reported by Metzger et al. (Reference Metzger, Soltani, Raeder, Hooker, Robinson and Sikkema2018a). No dose of atrazine provided 80%, 90%, or 95% control of Setaria spp. or barnyardgrass. In contrast, Metzger et al. (Reference Metzger, Soltani, Raeder, Hooker, Robinson and Sikkema2018a) reported >95% control of 10-cm green foxtail and barnyardgrass with tolpyralate + atrazine (30 + 1,000 g ai ha−1) 4 WAA. Additionally, Metzger et al. (Reference Metzger, Soltani, Raeder, Hooker, Robinson and Sikkema2018b) documented the ED of tolpyralate + atrazine to be 11.3 + 377.3 g ai ha−1 or less to achieve 90% control of 10-cm green foxtail and barnyardgrass 4 WAA. The results of this study may be due to the advanced height and development stage of Setaria spp. and barnyardgrass of 15 cm tall and three to four tillers on average at application compared to 10 cm in the study completed by Metzger et al. (Reference Metzger, Soltani, Raeder, Hooker, Robinson and Sikkema2018b). This suggestion is supported by Metzger et al. (Reference Metzger, Soltani, Raeder, Hooker, Robinson and Sikkema2019b) who reported that tolpyralate + atrazine efficacy on green foxtail and barnyardgrass declines as weed size at the time of herbicide application increases, which did not occur with the broadleaf weed species in that study. All five broadleaf weed species were controlled by 90% with atrazine doses <560 g ai ha−1, with predicted doses ranging from 235 to 513 g ai ha−1. Velvetleaf, common ragweed, and common lambsquarters were controlled by 95% with atrazine doses <560 g ai ha−1; however, redroot pigweed required a dose of 1,136 g ai ha−1, and wild mustard required an atrazine dose of 890 g ai ha−1 to achieve 95% control.
Table 3. Nonlinear regression parameters and predicted atrazine dose to complement 30 g ai ha−1 of tolpyralate to achieve 80%, 90%, and 95% control of various weeds 4 wk after application from field trials in 2019, 2020, and 2021.
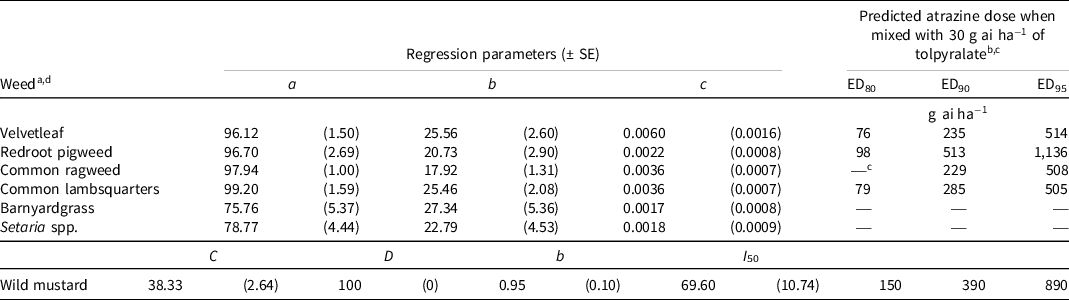
a Calculations for velvetleaf, redroot pigweed, common ragweed, common lambsquarters, barnyardgrass, and Setaria spp. were developed using Eq. 1, y = a – b [e (−c*dose)]; where y = response parameter, a = upper asymptote, b = magnitude constant, e = Euler’s number, and c = slope.
b EDn denotes the predicted effective dose of atrazine for percent control level n.
c An em dash (—) signifies that a predicted dose for percent control level n could not be computed.
d Calculations for wild mustard were developed using Eq. 2, y = C + (D – C)/{1 + e {−b*[log(dose) – log(I 50 )]}}; where y = response parameter, C = lower asymptote, D = upper asymptote, e = Euler’s number, I 50 = dose for 50% response, and b = slope about I 50.
At 8 WAA, the broadleaf weed species required ≤96 g ai ha−1 of atrazine to achieve 80% control when applied with 30 g ai ha−1 of tolpyralate (Table 4). Consistent with control 4 WAA, common ragweed was controlled by >80% with tolpyralate alone, so atrazine was not required for 80% control. In agreement with control results at 4 WAA, barnyardgrass and Setaria spp. could not be controlled by 80%, 90%, or 95% within the range of atrazine doses evaluated in this study. Osipitan et al. (Reference Osipitan, Scott and Knezevic2018) reported that comparatively higher doses of tolpyralate were required to achieve 90% control of green foxtail than for 90% control of velvetleaf or common lambsquarters when tolpyralate was applied alone or with 560 g ai ha−1 of atrazine. Langdon et al. (Reference Langdon, Soltani, Raeder, Robinson, Hooker and Sikkema2020) reported less than 90% control of barnyardgrass and green foxtail with tolpyralate + atrazine (30 + 560 g ai ha−1). Therefore, the dose of atrazine required to complement the highest label dose of tolpyralate (40 g ai ha−1) should be investigated in future studies. Velvetleaf, common ragweed, common lambsquarters, and wild mustard were controlled by 90% and 95% with atrazine doses less than the label dose of 560 g ai ha−1. Metzger et al. (Reference Metzger, Soltani, Raeder, Hooker, Robinson and Sikkema2018a) reported that tolpyralate at 30 g ai ha−1 co-applied with 1,000 g ai ha−1 of atrazine provided >95% control of velvetleaf, common ragweed, common lambsquarters, and wild mustard; however, the results of this study suggest that the atrazine dose can be approximately 0.5× the dose used by Metzger et al. (Reference Metzger, Soltani, Raeder, Hooker, Robinson and Sikkema2018a) to achieve excellent control of these weed species. Redroot pigweed required 1,231 g ai ha−1 of atrazine for 95% control. These results suggest that glyphosate or an effective very-long-chain fatty acid elongases inhibitor may be required in a corn weed management program to complement tolpyralate + atrazine for improved control of Setaria spp., barnyardgrass, and redroot pigweed (Langdon et al. Reference Langdon, Soltani, Raeder, Robinson, Hooker and Sikkema2020; Soltani et al. Reference Soltani, Brown and Sikkema2019).
Table 4. Nonlinear regression parameters and predicted atrazine dose to complement 30 g ai ha−1 of tolpyralate to achieve 80%, 90%, and 95% control of various weeds 8 wk after application from field trials in 2019, 2020, and 2021.
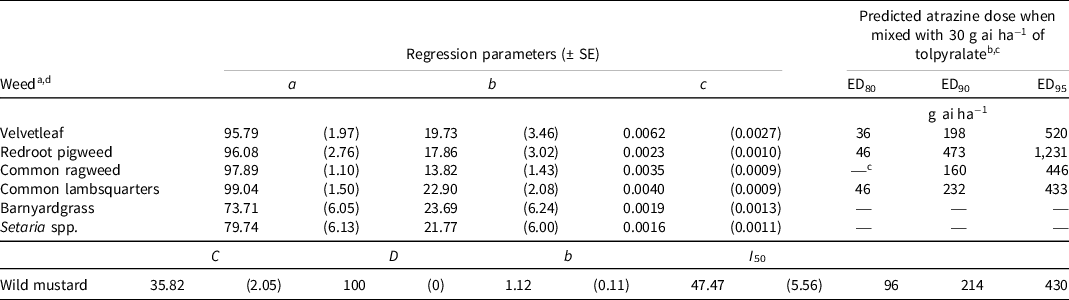
a Calculations for velvetleaf, redroot pigweed, common ragweed, common lambsquarters, barnyardgrass, and Setaria spp. were developed using Eq. 1, y = a – b [e (−c*dose)]; where y = response parameter, a = upper asymptote, b = magnitude constant, e = Euler’s number, and c = slope.
b EDn denotes the predicted effective dose of atrazine for percent control level n.
c An em dash (—) signifies that a predicted dose for percent control level n could not be computed.
d Calculations for wild mustard were developed using Eq. 2, y = C + (D – C)/{1 + e {−b*[log(dose) – log(I 50 )]}}; where y = response parameter, C = lower asymptote, D = upper asymptote, e = Euler’s number, I 50 = dose for 50% response, and b = slope about I 50.
Generally, the density and dry biomass data reflected the visible control data; however, this was not always the case. There was variability in the density of the weed species within each trial site, and interspecific competition among weeds within plots may have contributed to the lack of complementation of the weed density and dry biomass data to the visible control data. For example, weed species such as common ragweed and common lambsquarters were highly responsive to tolpyralate and the increasing doses of atrazine; therefore, the removal of these weed species allowed for the less responsive weed species to exhibit opportunistic growth by accumulating greater biomass per plant in plots, which was a function of less competition by control of the more sensitive weeds. Additionally, weed density and dry biomass data were collected from two 0.5-m2 quadrats per plot, which would contribute to variability in these objective response parameters because the quadrats were placed randomly and would not necessarily reflect the relative control for a given weed species based on an overview of the entire plot area. Similar limitations with density and dry biomass data in studies with multiple weed species in plots are also noted by Metzger et al. (Reference Metzger, Soltani, Raeder, Hooker, Robinson and Sikkema2018b, Reference Metzger, Soltani, Raeder, Hooker, Robinson and Sikkema2019b). Two ways to avoid the limitations with inconsistencies between density and dry biomass data and visible control data in future trials would be 1) to flag a specific number of weed species in each plot and collect only density and dry biomass data from the flagged weeds, or 2) to collect the density and biomass from the entire plot area; however, both practices would realistically be severely limited by time, space, and available resources. Despite the limitations with density and dry biomass data in multiple weed species studies, corn producers are often challenged with the need to control a variety of weed species; therefore, studies are needed to evaluate the efficacy of herbicides in typical weed community compositions. Visible weed control data provide a reliable measurement of efficacy despite the lack of objectivity by the viewer.
Consistent with control 8 WAA, no atrazine doses were computed to reduce the density of the grass weed species by 80%, 90%, or 95% because these levels of density reduction were not achieved with the range of atrazine doses used in this study or with tolpyralate applied alone (Table 5). In contrast, the density of broadleaf weed species was reduced by 80% with atrazine doses ranging from 83 to 757 g ai ha−1. A 90% and 95% density reduction of velvetleaf was not achieved in this study likely because of the low density of velvetleaf (4 plants m−2) in plots and the lack of differentiation between surviving plants that is accounted for in dry biomass data. Wild mustard density was not reduced by 90% or 95% in this study. Common ragweed, common lambsquarters, and redroot pigweed densities were reduced by 90% with atrazine doses of 309, 529, and 1,320 g ai ha−1, respectively. Common ragweed density was reduced 95% with an atrazine dose of 567 g ai ha−1, whereas common lambsquarters required 828 g ai ha−1 for the same density reduction.
Table 5. Nonlinear regression parameters and predicted atrazine dose to complement 30 g ai ha−1 of tolpyralate to achieve 80%, 90%, and 95% density reduction of various weeds relative to nontreated controls from field trials in 2019, 2020, and 2021.
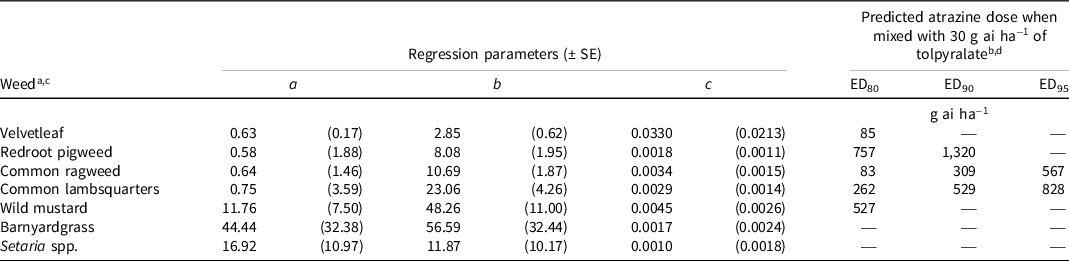
a Calculations for weeds were developed using Eq. 3, y = a + b [e (−c*dose)]; where y = response parameter, a = lower asymptote, b = reduction in y from intercept to a, e = Euler’s number, and c = slope from intercept to a.
b EDn denotes the predicted effective dose of atrazine for percent density reduction relative to the nontreated control level n.
c Average nontreated control densities: velvetleaf, 4 plants m−2; redroot pigweed, 13 plants m−2; common ragweed, 43 plants m−2; common lambsquarters, 58 plants m−2; wild mustard, 82 plants m−2; barnyardgrass, 81 plants m−2; Setaria spp., 57 plants m−2.
d An em dash (—) signifies that a predicted dose for percent density reduction relative to the nontreated control level n could not be computed.
The dry biomass data did not completely correspond with the density data. This is because dry biomass data includes the relative size of plants, while density data are simple count measures of whether live plants are present or not present. Redroot pigweed, common ragweed, and Setaria spp. did not require atrazine to achieve 80% dry biomass reduction because tolpyralate applied alone achieved this level of dry biomass reduction (Table 6). Additionally, tolpyralate did not require the addition of atrazine for 90% or 95% dry biomass reduction of common ragweed. Barnyardgrass dry biomass was not reduced by 80%, 90%, or 95% within the range of doses tested in this study, which is in agreement with control data recorded at 8 WAA. Velvetleaf, common lambsquarters, and wild mustard required low doses of ≤129 g ai ha−1 of atrazine to reduce the dry biomass by 80%. The dry biomass of velvetleaf, redroot pigweed, common lambsquarters, and wild mustard were reduced by 90% with atrazine doses <560 g ai ha−1, while Setaria spp. required 1,805 g ai ha−1 of atrazine for the same dry biomass reduction. The dry biomass of velvetleaf and Setaria spp. were not reduced by 95% within the range of atrazine doses tested in this study. A 95% dry biomass reduction of wild mustard, common lambsquarters, and redroot pigweed was achieved with atrazine doses of 161, 667, and 1,833 g ai ha−1, respectively.
Table 6. Nonlinear regression parameters and predicted atrazine dose to complement 30 g ai ha−1 of tolpyralate to achieve 80%, 90%, and 95% dry biomass reduction of various weeds relative to nontreated controls from field trials in 2019, 2020, and 2021.
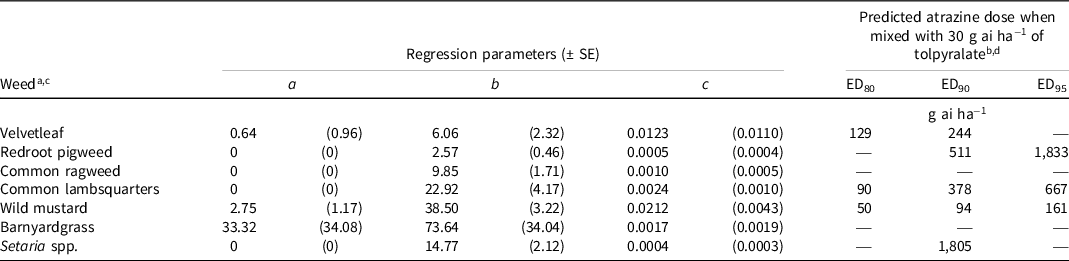
a Calculations for weeds were developed using Eq. 3, y = a + b [e (−c*dose)]; where y = response parameter, a = lower asymptote, b = reduction in y from intercept to a, e = Euler’s number, and c = slope from intercept to a.
b EDn denotes the predicted effective dose of atrazine for percent dry biomass reduction relative to the nontreated control level n.
c Average nontreated control dry biomasses: velvetleaf, 9.41 g m−2; redroot pigweed, 19.67 g m−2; common ragweed, 208.46 g m−2; common lambsquarters, 92.43 g m−2; wild mustard, 80.39 g m−2; barnyardgrass, 175.47 g m−2; Setaria spp., 78.37 g m−2.
d An em dash (—) signifies that a predicted dose for percent dry biomass reduction relative to the nontreated control level n could not be computed.
Corn Injury and Grain Yield
Corn injury was less than 5% and considered commercially acceptable at 1 and 2 WAA (data not presented). Where corn injury did occur, it was very minor chlorotic leaf speckling of leaves exposed at the time of application, which was also reported by Metzger et al. (Reference Metzger, Soltani, Raeder, Hooker, Robinson and Sikkema2018b) and Willemse et al. (Reference Willemse, Soltani, Metzger, Hooker, Jhala, Robinson and Sikkema2021b). Corn injury was 0% by 4 WAA. The low levels of corn injury exhibited in this study are consistent with previous research that tested various dose combinations of tolpyralate + atrazine (Langdon et al. Reference Langdon, Soltani, Raeder, Robinson, Hooker and Sikkema2020; Metzger et al. Reference Metzger, Soltani, Raeder, Hooker, Robinson and Sikkema2018b).
Because a weed-free control plot was not included in this study, the predicted atrazine doses calculated from the regression analysis were predicted to obtain 80%, 90%, and 95% of the yield of the upper asymptote of Equation 1 (Table 7). The predicted dose of atrazine needed to obtain 80%, 90%, and 95% of the yield of the upper asymptote was 2, 188, and 374 g ai ha−1, respectively, when mixed with 30 g ai ha−1 of tolpyralate.
Table 7. Nonlinear regression parameters and predicted atrazine dose to complement 30 g ai ha−1 of tolpyralate to achieve 80%, 90%, and 95% corn grain yield of the upper asymptote of Eq. 1 from field trials in 2020 and 2021.

a Corn grain yield was calculated using Eq. 1, y = a – b [e (−c*dose)]; where y = response parameter, a = upper asymptote, b = magnitude constant, e = Euler’s number, and c = slope.
b EDn denotes the predicted effective dose of atrazine for percent corn yield relative to a level n.
c Corn grain yield is in kilograms per hectare.
This study concludes that weed species varied in the required atrazine dose for 80%, 90%, and 95% control 8 WAA when co-applied with 30 g ai ha−1 of tolpyralate. Based on predicted values from regression analyses, velvetleaf, common ragweed, common lambsquarters, and wild mustard could be controlled by 95% with atrazine doses of 40 to 130 g ai ha−1 lower than the label recommendation of 560 g ai ha−1. In contrast, redroot pigweed required an atrazine dose of 1,231 g ai ha−1 for 95% control. Therefore, the recommended atrazine dose to complement tolpyralate is field-specific and should be recommended based on the suite of weed species in the field. A predicted atrazine dose could not be predicted for 80%, 90%, or 95% control of barnyardgrass or Setaria spp., and tolpyralate alone did not provide these levels of control, which suggests that another herbicide is needed in the herbicide program or a higher dose of tolpyralate is required to achieve control of these grass weed species. Using tolpyralate + atrazine in a two-pass system by including an effective preemergence herbicide may improve weed control in corn. Future research should investigate the required dose of atrazine to complement 40 g ai ha−1 of tolpyralate.
Acknowledgments
We thank Christy Shropshire and Todd Cowan for their technical support; Dr. Michelle Edwards for her statistical support; the University of Guelph, Ridgetown Campus summer staff for their field support; and ISK Biosciences Inc., Grain Farmers of Ontario (GFO), and the Ontario Agri-Food Innovation Alliance for the funding to conduct this research. No other conflicts of interest have been declared.