Introduction
Herbicides are used for managing weeds in diverse cropping systems in many countries (Jhala et al. Reference Jhala, Knezevic, Ganie, Singh, Chauhan and Mahajan2014a). The 4-hydroxyphenylpyruvate dioxygenase (HPPD)-inhibiting herbicides represent one of the latest discoveries of a new herbicide site of action that was introduced in the late 1990s (Mitchell et al. Reference Mitchell, Bartlett, Fraser, Hawkes, Holt, Townson and Wichert2001). Based on the site of action, HPPD inhibitor has been classified as Group 27 herbicides by the Weed Science Society of America and Herbicide Resistance Action Committee (Mallory-Smith and Retzinger Reference Mallory-Smith and Retzinger2017). The HPPD-inhibiting herbicides are broadly classified into chemical families: isoxazole (e.g., isoxaflutole), pyrazolone (e.g., pyrasulfotole, tolpyralate, topramezone), triketone (e.g., bicyclopyrone, mesotrione, and tembotrione; Figure 1), and isoxazolidinone (e.g., clomazone; Lee et al. Reference Lee, Prisbylla, Cromartie, Dagarin, Howard, Provan, Ellis, Fraser and Mutter1997). An additional class, azole carboxamides, has emerged in the patent literature, but these molecules have not been commercialized as of 2022 (Figure 1).

Figure 1. Chemical structures of some herbicides that inhibit 4-hydroxyphenylpyruvate dioxygenase (HPPD).
These herbicides inhibit the HPPD enzyme found in plants and animals that is essential for the synthesis of plastoquinone and tocopherols (Liu and Lu Reference Liu and Lu2016). The plastoquinone is in turn a co-factor in the formation of carotenoids, which protect chlorophyll in plants. Because of the lack of plastoquinone, tocopherols and carotenoid synthesis due to HPPD inhibition, sensitive plants suffer oxidative damage and chlorophyll destruction, turn white without deformation, and eventually die (Mitchell et al. Reference Mitchell, Bartlett, Fraser, Hawkes, Holt, Townson and Wichert2001). The HPPD-inhibiting herbicides are mainly used to control annual grass and broadleaf weeds, including herbicide-resistant biotypes primarily in grass crops such as sugarcane and corn (Grossman and Ehrhardt Reference Grossmann and Ehrhardt2007; Pallett et al. Reference Pallett, Cramp, Little, Veerasekaran, Crudace and Slater2001). After the evolution and widespread occurrence of weeds that became resistant to the acetolactate synthase (ALS) inhibitor, atrazine and glyphosate, the HPPD-inhibiting herbicides, played a key role for their management in agronomic crops, primarily in corn (Ganie and Jhala Reference Ganie and Jhala2017).
The HPPD-inhibitor herbicides are used primarily for selective preemergence (PRE) and postemergence (POST) use as a weed control mechanism primarily in corn, barley, oat, rice, sorghum, sugarcane, and wheat. Mesotrione is labeled for PRE use in weed control in sorghum and sugarcane. The HPPD- and photosystem (PS) II-inhibiting herbicides are applied in a mixture, because certain herbicides belonging to both sites of action interact synergistically and provide higher efficacy compared with being applied alone (Fluttert et al. Reference Fluttert, Soltani, Galla, Hooker, Robinson and Sikkema2022). For example, field experiments conducted in Nebraska reported that Palmer amaranth (Amaranthus palmeri L.) that was resistant to atrazine and an HPPD inhibitor was effectively controlled by their mix, even applied at labeled rates (Chahal and Jhala Reference Chahal and Jhala2018a).
The scientific literature is not available to cover the past, present, and future of the HPPD inhibitor. Therefore, the objectives of this review were to 1) summarize the history of HPPD-inhibitor herbicides and their use in the United States; 2) summarize HPPD-inhibitor resistant weeds, their mechanism of resistance, and management; 3) highlight the interactions of HPPD-inhibitor herbicides with other herbicides; and 4) summarize the future of HPPD-inhibitor herbicides, including products in the pipeline and HPPD-inhibitor-resistant crops.
History of HPPD-Inhibiting Herbicides
Inhibitors of HPPD (HPPD, EC 1.13.11.27) were the results of several concurrent industry research programs. Pyrazolones were first commercialized by the Sankyo company in 1980 with pyrazolynate in the United States (Figure 2). Pyrazoxyfen by Ishihara followed in 1985, benzofenap by Mitsubishi and Rhône-Poulenc in 1987, topramezone by BASF in 2006, and pyrasulfotole by Bayer Crop Science in 2007 (Figure 3). The Ishihara company has commercialized its corn herbicide tolpyralate, which was first registered in 2017 (Tsukamoto et al. Reference Tsukamoto, Kikugawa, Nagayama, Suganuma, Okita and Miyamoto2021).
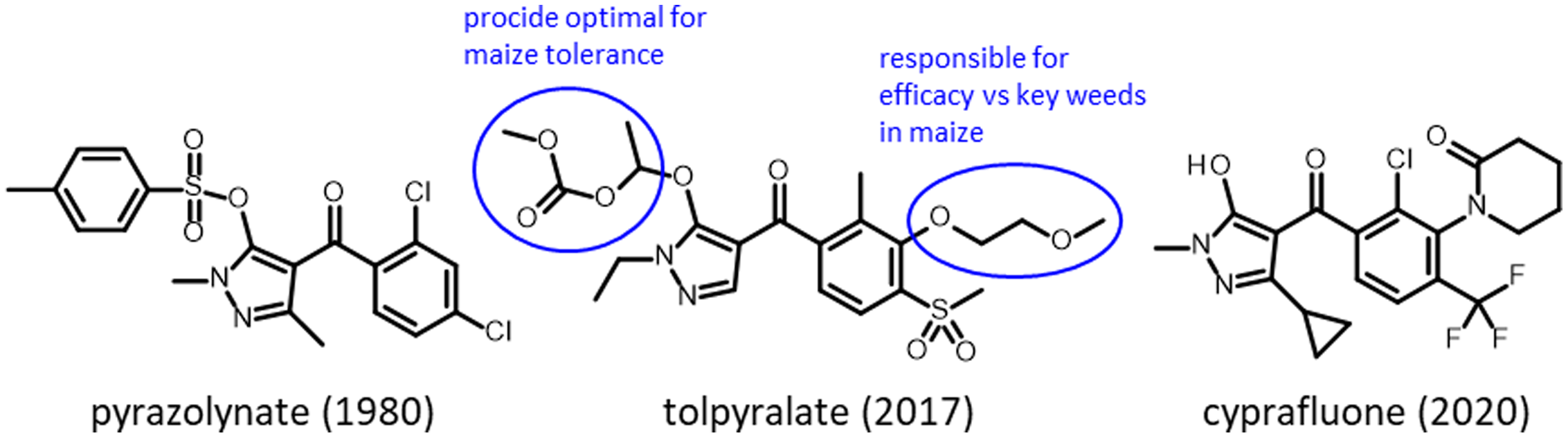
Figure 2. Chemical structures of pyrazolone herbicides, a chemical family of herbicides that inhibit 4-hydroxyphenylpyruvate dioxygenase (HPPD).

Figure 3. Timeline of commercialization of herbicides that inhibit 4-hydroxyphenylpyruvate dioxygenase (HPPD), their respective chemical classes, and manufacturer.
KingAgroot launched four new pyrazolone herbicides in China in 2020: cyprafluone, bipyrazone, fenpyrozone, and tripyrasulfone. Cyprafluone became KingAgroot’s first active ingredient to launch outside of China when granted registration in Pakistan in 2021 (KingAgroot 2021), with a plan for registration in other countries. Cyprafluone controls grass weeds such as Japanese foxtail (Alopecurus japonicus Steud.) and littleseed canarygrass (Phalaris minor Retz.) in wheat (Triticum aestivum L.). Wang et al. (Reference Wang, Liu, Jin, Peng, Zhang and Wang2020) reported that bipyrazone applied POST has a potential for broadleaf weed control in wheat in China.
Concurrently, a research group at Stauffer, a legacy company of ICI and now Syngenta, discovered the triketone-type HPPD inhibitor in 1982 through a chemical ecology approach. Researchers at Reed Gray observed that few weeds grew under crimson bottlebrush [Callistemon citrinus (Curtis) Skeels] in the California chaparral. Bioassay-guided isolation of crimson bottlebrush extracts led to the identification of fractions that can induce bleaching in developing seedlings. The active fractions contained the natural product leptospermone, a natural inhibitor of HPPD (Dayan et al. Reference Dayan, Duke, Sauldubois, Singh, McCurdy and Cantrell2007; Owens et al. Reference Owens, Nanayakkara and Dayan2013). The herbicidal activity of leptospermone and a series of synthetic triketone analogues were patented in 1980 (Figure 4). Structure-activity relationship studies characterized the chemical toxophore that is responsible for inhibiting HPPD (Ahrens et al. Reference Ahrens, Lange, Müller, Rosinger, Willms and van Almsick2013).
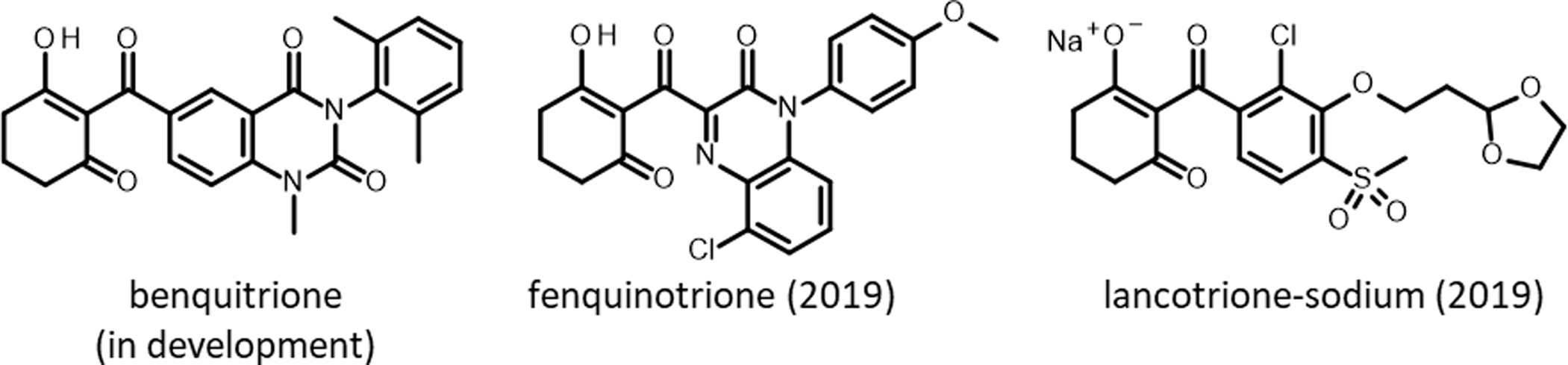
Figure 4. Chemical structures of triketone herbicides, a chemical family of herbicides that inhibit 4-hydroxyphenylpyruvate dioxygenase (HPPD).
Triketones were first introduced to growers in 1991 by Zeneca (now Syngenta) with sulcotrione. Since then, a steady stream of triketones have been launched: benzobicyclon (by SDS Biotech in 2001), mesotrione (by Syngenta in 2002), tembotrione (by Bayer Crop Science in 2007), tefuryltrione (by Bayer in 2009), and bicyclopyrone (by Syngenta in 2015; Beaudegnies et al. Reference Beaudegnies, Edmunds, Fraser, Hall, Hawkes, Mitchell, Schaetzer, Wendeborn and Wibley2009). Kumiai Chemical registered fenquinotrione (Figure 4), trademarked as Effeeda, to control broadleaf weeds and sedges in rice in Japan. The molecule’s 4-methoxyphenyl group confers resistance to rice while maintaining weed control efficacy via selective metabolism (Yamamoto et al. Reference Yamamoto, Tanetani, Uchiyama, Nagamatsu, Kobayashi, Ikeda and Kawai2021). Kumiai collaborated with Certis to register fenquinotrione in the European Union for weed control in cereals and rice. Certis submitted a registration package in 2021. If successful, it is expected that the product will be available around 2025. Ishihara launched lancotrione-sodium in 2019 for control of broadleaf weeds and sedges in rice, including weeds that are resistant to sulfonylurea herbicides. Originally invented at Central China Normal University, benquitrione is the first in a series of quinazoline-2,4-diones triketone herbicides from Guang-Fu Yang’s laboratory, co-developed with Shandong Cynda (Figure 4; Wang et al. Reference Wang, Lin, Cao, Ming, Chen, Hao, Yang and Yang2015).
The first HPPD inhibitor was discovered serendipitously by Japanese companies, with pyrazolynate discovered by Sankyo and commercialized in 1980, and pyrazoxyfen discovered by Ishihara in 1985 (Figure 4). Both were commercialized for weed control in rice before their site of action was understood. It is now known that these compounds were pro-herbicides that are bio-activated into free hydroxypyrazole active pharmacophore, which inhibits HPPD.
Studies carried out by Rhone-Poulenc (now Bayer Crop Science) in the late 1980s led to the discovery of isofluxatole, an isoxazole heterocyclic proherbicide that is bio-activated to a diketonitrile by soil and plant enzymes (Pallett et al. Reference Pallett, Cramp, Little, Veerasekaran, Crudace and Slater2001; Figure 4). The bleaching caused by the HPPD inhibitor is similar to that observed with an inhibitor of phytoene desaturase (PDS), but the mechanism by which this bleaching occurred eluded researchers. The link between triketone molecules and their inhibition of HPPD was first elucidated using mammalian systems related to tyrosine metabolism. Subsequent investigations in plant systems established that HPPD catalyzes a key step in plastoquinone and tocopherol synthesis (Schultz et al. Reference Schultz, Soll, Fiedler and Schultze-Siebert1985), and further studies demonstrated that plastoquinone was an essential co-factor for phytoene desaturase (Norris et al. Reference Norris, Barrette and DellaPenna1995). This established the link between inhibition of HPPD and the bleaching symptoms that can be observed in the foliage of treated plants. In brief, plants treated with an HPPD inhibitor accumulate tyrosine and are depleted in plastoquinone. Without plastoquinone, PDS cannot function, which halts carotenoid biosynthesis, resulting in bleaching of the new growth, which is known as the “triketone effect” (Lee et al. Reference Lee, Prisbylla, Cromartie, Dagarin, Howard, Provan, Ellis, Fraser and Mutter1997).
Use of HPPD Inhibitor in the United States
In a survey conducted by the United States Department of Agriculture–National Agricultural Statistics Service (USDA-NASS) in 2018, the use of HPPD-inhibiting herbicides, including isoxaflutole, tembotrione, mesotrione, bicyclopyrone, and topramezone, was estimated at about 193,000, 214,000, 1,898,000, 102,000, and 41,000 kg, respectively (Figure 5).
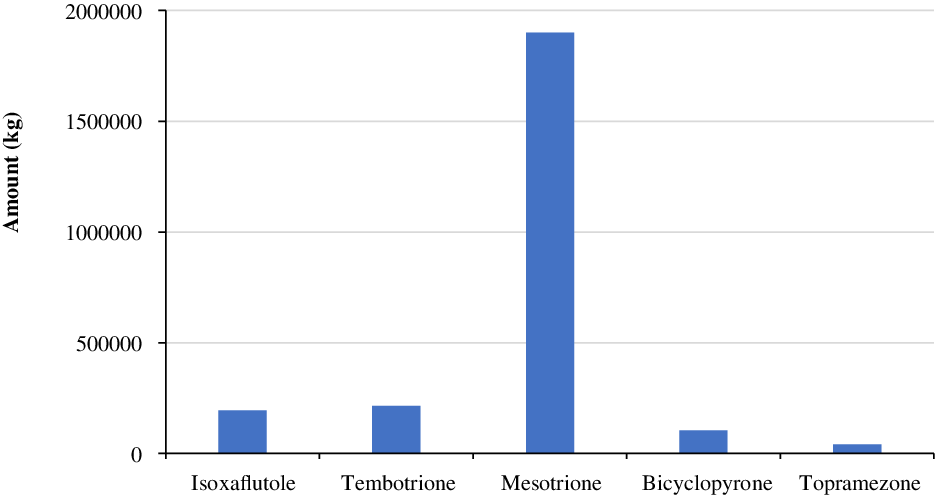
Figure 5. Annual use of major herbicides that inhibit 4-hydroxyphenylpyruvate dioxygenase (HPPD) in corn production in the United States in 2018 (Source: USDA-NASS 2018).
Mesotrione. Mesotrione belongs to the triketone family of HPPD-inhibitor herbicides and represents one of the most used active ingredients in corn (applied to about 42% of planted corn in 2018) with an average of one application (75 to 150 g ha−1) per year (Figure 6; USDA-NASS 2018). The Midwestern states, including Iowa, Illinois, Kansas, Minnesota, and Nebraska, led the use of mesotrione with an average annual use of >10,000 kg in 2018 at the rate of >1.27 kg mesotrione per square mile in each state (Figure 6). Increased use of mesotrione in recent years is primarily attributed to increasing demand for controlling glyphosate-resistant weeds (Chahal and Jhala Reference Chahal and Jhala2018b; Ganie et al. Reference Ganie, Stratman and Jhala2015; Ganie and Jhala Reference Ganie and Jhala2017). Mesotrione is a systemic herbicide applied alone or in mixture for selective PRE and POST control of grass and broadleaf weeds in field corn, seed corn, yellow popcorn, sweet corn, and grain sorghum (Abit et al. Reference Abit, Al-Khatib, Currie, Stahlman, Geier, Gordon, Olson, Claassen and Regehr2010; Armel et al. Reference Armel, Wilson, Richardson and Hines2003; Currie and Geier Reference Currie and Geier2018; Janak and Grichar Reference Janak and Grichar2016; Stephenson et al. Reference Stephenson, Bond, Walker, Bararpour and Oliver2004; Williams et al. Reference Williams, Pataky, Nordby, Riechers, Sprague and Masiunas2005).
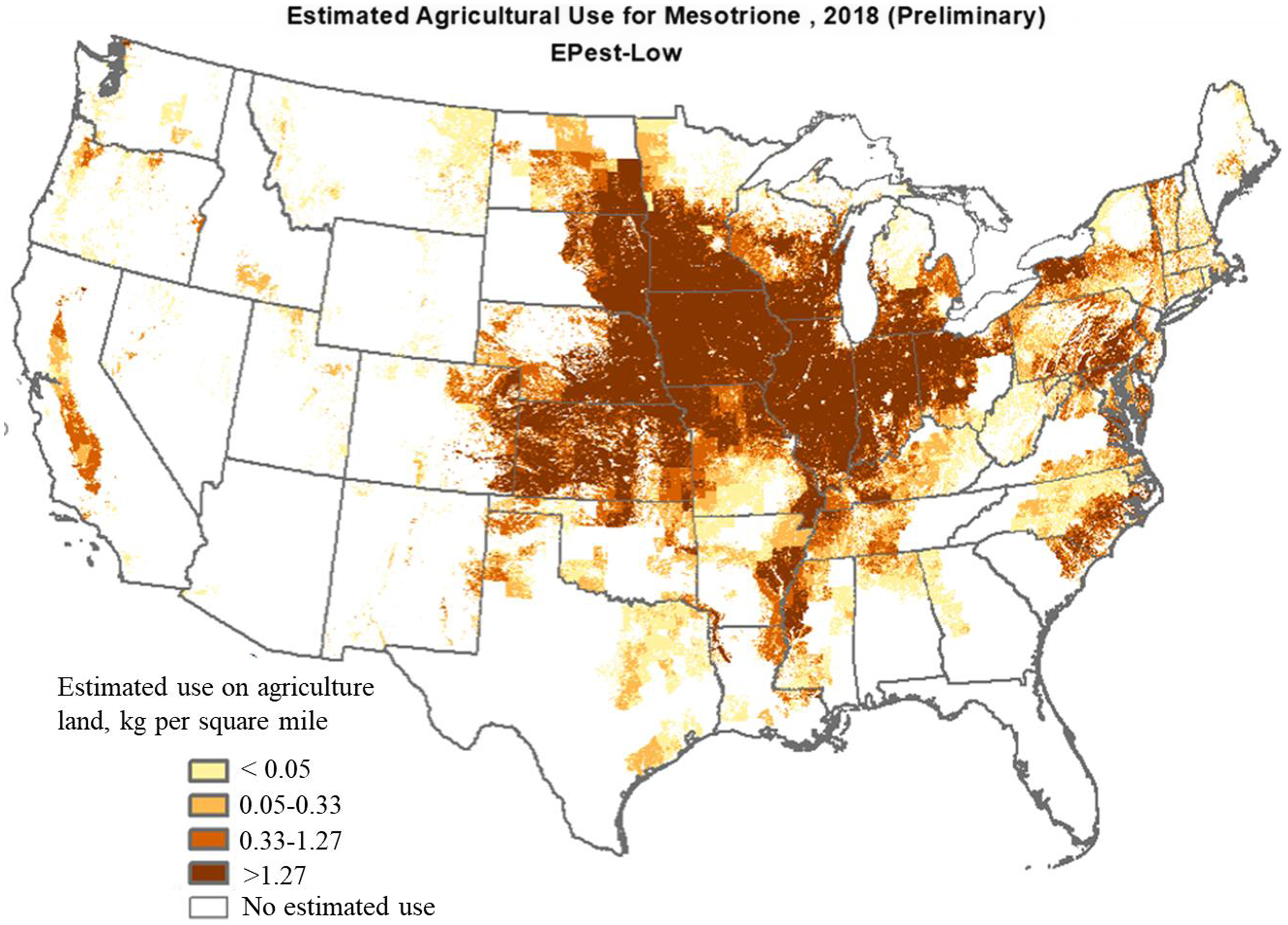
Figure 6. Mesotrione use in agricultural land across the United States in 2018 (adapted from the U.S. Geological Survey by the U.S. Department of the Interior).
Mesotrione in a pre-mixture or tank-mixture with other herbicides can provide effective control of ALS-, PS II-, and glyphosate-resistant weeds (Chahal and Jhala Reference Chahal and Jhala2018a; Ganie et al. Reference Ganie, Stratman and Jhala2015). In addition to corn and grain sorghum, the use of mesotrione applied PRE in spring cereals, including barley (Hordeum vulgare L.), durum wheat, oats (Avena sativa L.), and spring wheat, has been found to be safe and provides adequate selective control of broadleaf weeds, including common lambsquarters (Chenopodium album L.), common ragweed (Ambrosia artemisiifolia L.), and wild buckwheat (Polygonum convolvulus L.) in a study conducted in Ontario, Canada (Soltani et al. Reference Soltani, Shropshire and Sikkema2011, Reference Soltani, Shropshire, Cowan and Sikkema2014); however, mesotrione use in those crops is limited (Walsh et al. Reference Walsh, Newman and Chatfield2021).
Tembotrione
Tembotrione is a member of the triketone family of HPPD inhibitors that is used for selective POST control of grass and broadleaf weeds in corn (Stephenson et al. Reference Stephenson, Bond, Landry and Edwards2015). Tembotrione is the second-highest used HPPD inhibitor in the United States, with an annual use rate of >200,000 kg (Figure 7; USDA-NASS 2018). Minnesota, Illinois, Nebraska, Indiana, and Iowa were leading states for annual use (>20,000 kg) of tembotrione in corn production in 2018 (Figure 7). Tembotrione (Laudis; Bayer Crop Science, St Louis, MO) is applied alone or in a mixture from field corn emergence to the V8 growth stage or V7 (sweet corn). More recently, metabolic-based resistance (CYP-mediated metabolism) to tembotrione has been identified in several grain sorghum lines (Pandian et al. Reference Pandian, Varanasi, Vannapusa, Sathishraj, Lin, Zhao, Tunnell, Tesso, Liu, Prasad and Jugulam2020), indicating a future increase in tembotrione use in other crops.
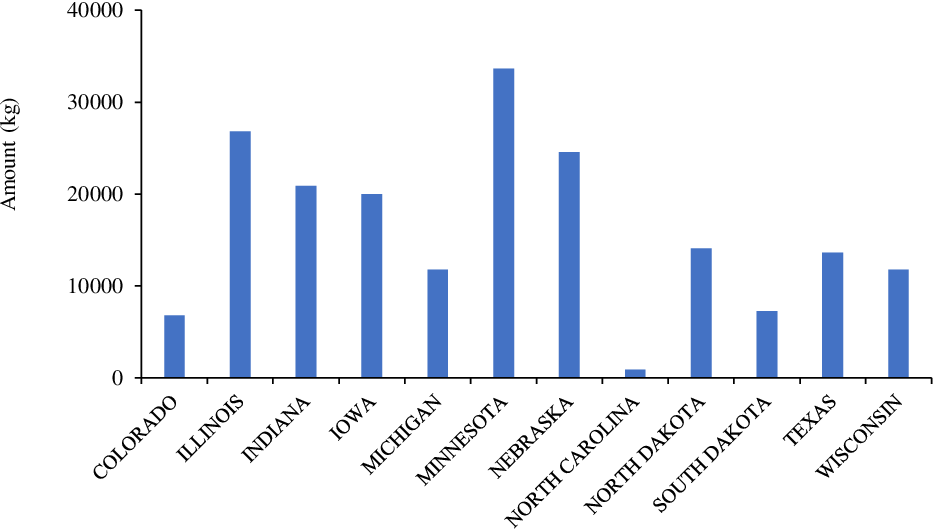
Figure 7. Tembotrione use in various corn-producing states in the United States (Source: USDA-NASS 2018).
Isoxaflutole
Isoxaflutole was the first member of the isoxazole class of HPPD inhibitor. Common brand names include Balance Flexx™ and Corvus™, and were the first HPPD-inhibiting herbicides introduced in North America in 1996 (Figure 1; Pallett et al. Reference Pallett, Little, Sheekey and Veerasekaran1998). It is a selective herbicide primarily used for PRE control of grass and broadleaf weeds in corn, and recently in isoxaflutole-resistant soybean (Mausbach et al. Reference Mausbach, Irmak, Sarangi, Lindquist and Jhala2021). Isoxaflutole is commonly mixed with PS II-inhibiting herbicides (e.g., atrazine) to improve weed control efficacy and spectrum (Benoit et al. Reference Benoit, Soltani, Hooker, Robinson and Sikkema2019; Chahal and Jhala Reference Chahal and Jhala2018a; Fluttert et al. Reference Fluttert, Soltani, Galla, Hooker, Robinson and Sikkema2022). According to the survey conducted by the USDA-NASS (2018), isoxaflutole was the third-most used HPPD inhibitor in corn (used in about 8% of planted corn) with an average of one application (72 g ha−1) per year (Figure 8). Iowa, Illinois, and Nebraska were the leading states for isoxaflutole use among various corn-producing states in 2018 (Figure 8). Isoxaflutole has been widely used as a part of herbicide-resistant weed management strategies (including ALS-, PS II-, and glyphosate-resistant) in corn (Benoit et al. Reference Benoit, Soltani, Hooker, Robinson and Sikkema2019; Chahal et al. Reference Chahal, Aulakh, Jugulam, Jhala and Price2015; Stephenson and Bond Reference Stephenson and Bond2012).
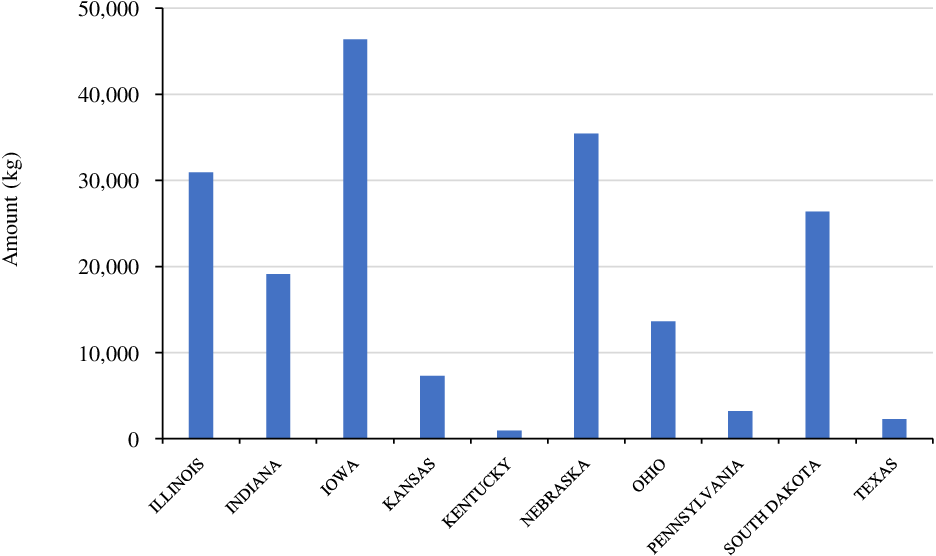
Figure 8. Isoxaflutole use in major corn-producing states in the United States (Source: USDA-NASS 2018).
Isoxaflutole can also be used for weed control in fallow fields depending on the subsequent rotational crop (Currie and Geier Reference Currie and Geier2016; Kumar and Jha Reference Kumar and Jha2015). In this context, isoxaflutole-resistant soybean has recently been developed in which isoxaflutole can be used as a part of an herbicide strategy to control ALS- and glyphosate-resistant weeds, including Palmer amaranth, waterhemp, and Canada fleabane (Erigeron canadensis L.; Ditschun et al. Reference Ditschun, Soltani, Robinson, Tardif, Kaastra and Sikkema2016; Mausbach et al. Reference Mausbach, Irmak, Sarangi, Lindquist and Jhala2021; Smith et al. Reference Smith, Soltani, Kaastra, Hooker, Robinson and Sikkema2019a).
Bicyclopyrone and Topramezone
Bicyclopyrone and topramezone are two other HPPD-inhibitor herbicides (via an active ingredient of an individual product or various premixtures) that are commonly used for grass and broadleaf weed control in field corn, seed corn, silage corn, yellow popcorn, sweet popcorn, and sugarcane (Sarangi and Jhala Reference Sarangi and Jhala2018). Topramezone belongs to the pyrazolone family with an annual use of >41,000 kg, whereas bicyclopyrone belongs to the triketone family with an annual use of >100,000 kg in corn production (Figure 9).
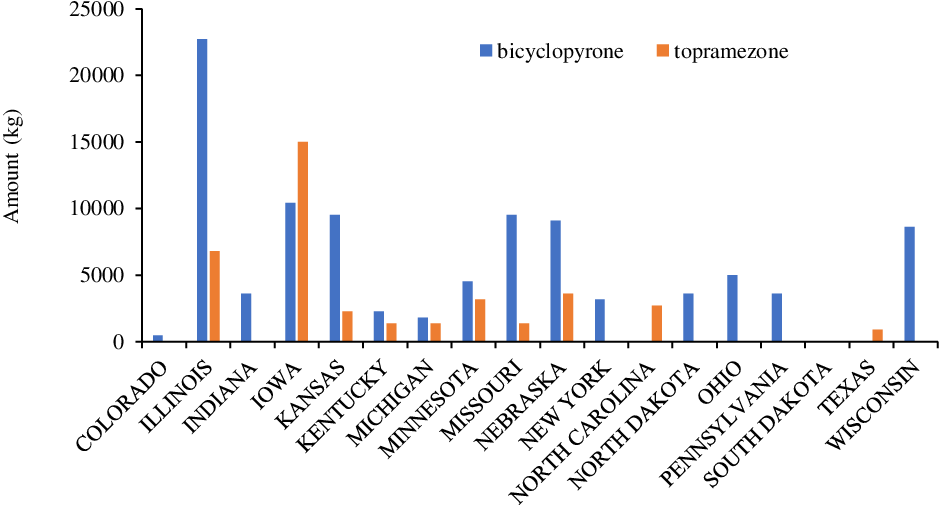
Figure 9. Bicyclopyrone and topramezone use in various corn-producing states in the United States (Source: USDA-NASS 2018).
According to the USDA-NASS (2018) survey, Illinois, Iowa, Kansas, Missouri, Nebraska, and Wisconsin were the leading states for annual use of bicyclopyrone with an estimate of >5,000 kg in corn crops, whereas Illinois and Iowa were the top states in annual use of topramezone (>5,000 kg) for weed control in corn (Figure 9). In addition to corn, topramezone and bicyclopyrone are known to provide effective weed control in other crops, including turf, sweet potato [Ipomoea batatas (L.) Lam.], wheat, chickpea (Cicer arietinum L.), and rice (Brosnan and Breeden Reference Brosnan and Breeden2013; Lindley et al. Reference Lindley, Jennings, Monks, Chaudhari, Schultheis, Waldschmidt and Brownie2020; Moore 2019).
Pyrasulfotole and Tolpyralate
Pyrasulfotole is a member of the pyrazolone family of HPPD inhibitors and is registered for use on cereal grains, including wheat, barley, rye, triticale, and grain sorghum (Kumar et al. Reference Kumar, Jha and Reichard2014; Reddy et al. Reference Reddy, Stahlman, Geier, Thompson, Currie, Schlegel, Olson and Lally2013; Torbiak et al. Reference Torbiak, Blackshaw, Brandt, Hamman and Geddes2021). Pyrasulfotole is an active ingredient of Huskie™ (a premixture of pyrasulfotole and bromoxynil; Bayer Crop Science, Saint Louis, MO) that is used for broadleaf weed control in sorghum. In contrast, tolpyralate is a new HPPD inhibitor that came to the market in 2020 and controls several annual grass and broadleaf weed species with a low use rate (30 to 50 g ha−1) in corn (Tsukamoto et al. Reference Tsukamoto, Kikugawa, Nagayama, Suganuma, Okita and Miyamoto2021; Willemse et al. Reference Willemse, Soltani, Metzger, Hooker, Jhala, Robinson and Sikkema2021c).
Benzobicyclon
Benzobicyclon, a pro-herbicide, is a member of the triketone family of HPPD inhibitors. It was first registered for use in rice crops in 2021 under the tradename Rogue® (Gowan Company, Yuma, AZ), mainly for control of aquatic weeds, sprangletop species (Leptochloa spp.), and suppression of weedy rice biotypes when applied post-flood. This is the only HPPD-inhibiting herbicide available for use rice production in the United States.
HPPD-Inhibitor-Resistant Weeds and Their Mechanisms of Resistance
Although HPPD-inhibitor herbicides have been in use for more than two decades, the evolution of HPPD-inhibitor-resistant weeds is relatively less widespread and slower than some other herbicide sites of action (Jhala et al. Reference Jhala, Sandell, Rana, Kruger and Knezevic2014b; Kaundun Reference Kaundun2021). As of 2022, three broadleaf weeds (Palmer amaranth, waterhemp, and wild radish) have evolved resistance to HPPD-inhibitor herbicides across the globe (Heap Reference Heap2022). Several populations of HPPD-inhibitor-resistant Palmer amaranth and waterhemp have evolved across the Midwestern United States (Jhala et al. Reference Jhala, Sandell, Rana, Kruger and Knezevic2014b; Hausman et al. Reference Hausman, Singh, Tranel, Riechers, Kaundun, Polge, Thomas and Hager2011), while HPPD-inhibitor-resistant wild radish has been documented in Western Australia. The first case of resistance to these herbicides was reported in a population of waterhemp from a corn field in Illinois that had a history of repeated HPPD-inhibitor use (Hausman et al. Reference Hausman, Singh, Tranel, Riechers, Kaundun, Polge, Thomas and Hager2011). The resistance in Palmer amaranth (Thompson et al. Reference Thompson, Peterson and Nathan2012) and wild radish (Lu et al. Reference Lu, Yu, Han, Owen and Powles2020) were not selected with HPPD-inhibitor herbicides; rather, these populations exhibited cross-resistance with the mechanisms that bestow resistance to different herbicide sites of action (Lu et al. Reference Lu, Yu, Han, Owen and Powles2020; Nakka et al. Reference Nakka, Godar, Wani, Thompson, Peterson, Roelofs and Jugulam2017).
Mechanisms of Resistance to HPPD Inhibitors
Palmer Amaranth
The first case of Palmer amaranth resistance to the HPPD inhibitor (also found to be resistant to PS II- and ALS-inhibitor formulas) was confirmed in a field in central Kansas where there was no history of HPPD inhibitor use, though there was a long history of use of herbicides that PS II and ALS (Jhala et al. Reference Jhala, Sandell, Rana, Kruger and Knezevic2014b; Thompson et al. Reference Thompson, Peterson and Nathan2012). This population was originally found to be resistant to Huskie®, a premix of pyrasulfotole (an HPPD inhibitor) and bromoxynil (a PS II inhibitor). Furthermore, this Palmer amaranth biotype was resistant to several HPPD inhibitor herbicides, including mesotrione, tembotrione, and topramezone (Thompson et al. Reference Thompson, Peterson and Nathan2012). Later, a Palmer amaranth population from a corn field in Nebraska that had a history of HPPD inhibitor use was found to be resistant to these herbicides (Jhala et al. Reference Jhala, Sandell, Rana, Kruger and Knezevic2014b). Populations of Palmer amaranth in Kansas and Nebraska exhibited up to 18-fold resistance to mesotrione, tembotrione, or topramezone (Jhala et al. Reference Jhala, Sandell, Rana, Kruger and Knezevic2014b; Nakka et al. Reference Nakka, Godar, Wani, Thompson, Peterson, Roelofs and Jugulam2017; Thompson et al. Reference Thompson, Peterson and Nathan2012). In both populations, the mechanism of resistance to the HPPD inhibitor was due to neither differential herbicide uptake/translocation nor mutations or amplification of the HPPD gene (Küpper et al. Reference Küpper, Peter, Zöllner, Lorentz, Tranel, Beffa and Gaines2018; Nakka et al. Reference Nakka, Godar, Wani, Thompson, Peterson, Roelofs and Jugulam2017). The Kansas Palmer amaranth biotype metabolized more than 90% of mesotrione at 24 h after treatment compared with sensitive plants (Nakka et al. Reference Nakka, Godar, Wani, Thompson, Peterson, Roelofs and Jugulam2017). Additionally, a 4-fold to 14-fold higher HPPD gene expression was found in this population (Nakka et al. Reference Nakka, Godar, Wani, Thompson, Peterson, Roelofs and Jugulam2017). Similarly, the rapid metabolism of tembotrione was attributed to the resistance in Palmer amaranth population from Nebraska (Küpper et al. Reference Küpper, Peter, Zöllner, Lorentz, Tranel, Beffa and Gaines2018). Although 4-hydroxylation of tembotrione followed by glycosylation was identified in both resistant and sensitive plants, the time taken to form metabolites was shorter in resistant plants compared with sensitive plants (Küpper et al. Reference Küpper, Peter, Zöllner, Lorentz, Tranel, Beffa and Gaines2018). More recently, a population of Palmer amaranth from Kansas (Riley County) was resistant to mesotrione and tembotrione (Shyam et al. Reference Shyam, Borgato, Peterson, Dille and Jugulam2021). The mechanism of resistance in this population is being investigated.
Waterhemp
Resistance to HPPD inhibitor herbicides has been documented in several populations of waterhemp across the Midwestern United States, including Illinois, Iowa, and Nebraska (Heap Reference Heap2022). A biotype of waterhemp known as MCR (for McLean County resistant) from Illinois was the first reported case of resistance to an HPPD inhibitor (Hausman et al. Reference Hausman, Singh, Tranel, Riechers, Kaundun, Polge, Thomas and Hager2011). This biotype was previously confirmed to be resistant to atrazine and ALS-inhibiting herbicides. MCR waterhemp had 10-fold and 35-fold resistance to mesotrione compared with two susceptible populations from Illinois (Hausman et al. Reference Hausman, Singh, Tranel, Riechers, Kaundun, Polge, Thomas and Hager2011). The mechanism of mesotrione resistance in MCR waterhemp was not due to reduced herbicide absorption/translocation nor because of alterations in the HPPD gene sequence or expression. However, compared with sensitive plants, MCR waterhemp rapidly metabolized mesotrione via hydroxylation of the cyclohexanedione ring of mesotrione (Ma et al. Reference Ma, Kaundun, Tranel, Riggins, McGinness, Hager, Hawkes, McIndoe and Riechers2013). Importantly, the time required to metabolize 50% of the absorbed mesotrione was ∼11.7 h in MCR compared with 25.4 to 27.8 h in the susceptible plants. Application of the cytochrome P450 inhibitor (malathion) increased the susceptibility of MCR plants to mesotrione, suggesting that the metabolism of mesotrione was mediated via P450 activity in this population (Ma et al. Reference Ma, Kaundun, Tranel, Riggins, McGinness, Hager, Hawkes, McIndoe and Riechers2013).
The HPPD-inhibitor-resistant waterhemp from Nebraska known as NEB showed a 2.4-fold and 45-fold level of resistance to mesotrione applied PRE and POST, respectively, compared with a known susceptible population (Kaundun et al. Reference Kaundun, Hutchings, Dale, Howell, Morris, Kramer, Shivrain and McIndoe2017). Similar to MCR waterhemp, mesotrione resistance in the Nebraska population was primarily due to higher levels of mesotrione metabolism via 4-hydroxylation (Kaundan et al., Reference Kaundun, Hutchings, Dale, Howell, Morris, Kramer, Shivrain and McIndoe2017). Furthermore, the metabolites of mesotrione were identified as 4-hydroxymesotrione and AMBA [2-amino-4-(methylsulfonyl) benzoic acid; (Kaundan et al. Reference Kaundun, Hutchings, Dale, Howell, Morris, Kramer, Shivrain and McIndoe2017)]. No duplication, alteration, or over-expression of the HPPD gene that can confer resistance was found in this population (Kaundan et al. Reference Kaundun, Hutchings, Dale, Howell, Morris, Kramer, Shivrain and McIndoe2017). Moreover, mesotrione-resistant waterhemp population from Illinois and Nebraska were also resistant to topramezone, which belongs to the pyrazolone subfamily of HPPD inhibitors. Both populations rapidly metabolized topramezone, and the metabolic profiles indicated two different putative hydroxylated forms of topramezone (hydroxytopramezone-1 and hydroxytopramezone-2), although hydroxytopramezone-1 was more abundant in the Illinois waterhemp population (Lygin et al. Reference Lygin, Kaundun, Morris, Mcindoe, Hamilton and Riechers2018). When metabolic profiles at 48 h after treatment were compared with naturally tolerant corn, the waterhemp population from Illinois had more hydroxylated metabolites, whereas corn plants produced desmethyl and benzoic acid metabolites of topramezone, suggesting that waterhemp initially metabolizes topramezone differently than corn (Lygin et al. Reference Lygin, Kaundun, Morris, Mcindoe, Hamilton and Riechers2018).
More recently, the mechanism of resistance to syncarpic acid-3, a nonselective, noncommercial HPPD inhibitor, was investigated in an Illinois population of waterhemp (Concepcion et al. Reference Concepcion, Kaundun, Morris, Hutchings, Strom, Lygin and Riechers2021). Although the Phase I metabolite, likely produced due to P450-mediated hydroxylation was detected in this population, this metabolite was not found to be responsible for resistance; rather, the glutathione-syncarpic acid conjugate that formed as a result of Phase II metabolism was associated with resistance to syncarpic acid in the waterhemp population (Concepcion et al. Reference Concepcion, Kaundun, Morris, Hutchings, Strom, Lygin and Riechers2021).
Wild Radish. A population of wild radish from a Western Australian grain field with no prior history of HPPD inhibitor use is resistant to these herbicides (Lu et al. Reference Lu, Yu, Han, Owen and Powles2020). This population is also resistant to other herbicides such as PS II inhibitor, ALS inhibitor, and synthetic auxin (Lu et al. Reference Lu, Yu, Han, Owen and Powles2020). This wild radish population exhibited 4-fold to 6.5-fold resistance to mesotrione, tembotrione, and isoxaflutole (Lu et al. Reference Lu, Yu, Han, Owen and Powles2020). The resistant plants were able to metabolize mesotrione approximately 8-fold faster than the sensitive plants (Lu et al. Reference Lu, Yu, Han, Owen and Powles2020). It was also confirmed that the resistance was not due to reduced uptake/translocation of mesotrione, and no target site alterations were detected (Lu et al. Reference Lu, Yu, Han, Owen and Powles2020).
Although resistance to HPPD inhibitor herbicides has currently been reported in three weed species across the globe, if selection pressure continues, new cases of resistance evolution to HPPD inhibitor will increase. More importantly, the predominance of metabolic resistance to HPPD inhibitor herbicides, was found in all three weed species (Jugulam and Shyam Reference Jugulam and Shyam2019; Yu and Powles Reference Yu and Powles2014). Therefore, prudent strategies, including integration of nonchemical methods, need to be designed for sustainable weed management.
Management of HPPD-Inhibitor-Resistant Weeds
As of 2022, Palmer amaranth and waterhemp are the only two weed species in the United States that have evolved resistance to HPPD-inhibitor herbicides (Heap Reference Heap2022; Jhala et al. Reference Jhala, Sandell, Rana, Kruger and Knezevic2014b). Therefore, strategies described here focus on the management of HPPD-inhibitor-resistant Palmer amaranth and waterhemp primarily in corn and soybean production systems. Although the evolution of herbicide resistance in weed species cannot be completely averted, it can possibly be delayed by implementing diversified weed management practices (Norsworthy et al. Reference Norsworthy, Ward, Shaw, Llewellyn, Nichols, Webster, Bradley, Frisvold, Powles, Burgos, Witt and Barrett2012). Mixing herbicides that have different sites of action is often recommended to delay the evolution of herbicide-resistant weeds (Diggle et al. Reference Diggle, Neve and Smith2003; Evans et al. Reference Evans, Tranel, Hager, Schutte, Wu, Chatham and Davis2016). In corn, HPPD-inhibiting herbicides are mixed with PS II-inhibiting herbicides due to their synergistic activity for controlling triazine-resistant weeds (Chahal et al. Reference Chahal, Jugulam and Jhala2019; Hugie et al. Reference Hugie, Bollero, Tranel and Riechers2008; Woodyard et al. Reference Woodyard, Hugie and Riechers2009c). However, continued use of this mixture to control atrazine-resistant weeds in corn has resulted in the evolution and widespread occurrence of Palmer amaranth and waterhemp populations resistant to PS II and HPPD inhibitors (Jhala et al. Reference Jhala, Sandell, Rana, Kruger and Knezevic2014b).
Herbicide options to control Palmer amaranth and waterhemp in corn and soybean crops include inhibitors of ALS, PS II, HPPD, protoporphyrinogen oxidase (PPO), very long chain fatty acid (VLCFA), glyphosate, glufosinate, and synthetic auxins. However, Palmer amaranth and waterhemp populations with multiple resistance to ALS, PS II, HPPD, and PPO inhibitors, and glyphosate are increasingly common in the Midwestern United States (Heap Reference Heap2022; Jhala et al. Reference Jhala, Sandell, Rana, Kruger and Knezevic2014b; Schultz et al. Reference Schultz, Chatham, Riggins, Tranel and Bradley2015; Shyam et al. Reference Shyam, Borgato, Peterson, Dille and Jugulam2021; Varanasi et al. Reference Varanasi, Brabham, Norsworthy, Nie, Young, Houston, Barber and Scott2018). This has reduced herbicide options to control weeds in corn and soybean production systems (Sarangi et al. Reference Sarangi, Stephens, Barker, Patterson, Gaines and Jhala2019). Therefore, herbicide programs containing diverse herbicide sites of action and detoxification pathways are required to manage HPPD-inhibitor-resistant Palmer amaranth and waterhemp.
Fortunately, waterhemp biotypes with metabolic resistance to atrazine, a resistance mechanism present in a majority of populations in the Midwest (Tranel Reference Tranel2021), are sensitive to other PS II-inhibitor herbicides such as metribuzin (Jacobs et al. Reference Jacobs, Butts-Wilmsmeyer, Ma, O’Brien and Riechers2020). Therefore, metribuzin mixed with an HPPD-inhibitor can control populations that are resistant to an HPPD inhibitor and atrazine. For example, atrazine at 4.48 kg ha−1 applied PRE provided 26% control of PS II-inhibitor-resistant and HPPD-inhibitor-resistant waterhemp at 4 wk after treatment (WAT), whereas metribuzin 560 g ha−1 provided 95% control of the same population (Evans et al. Reference Evans, Strom, Riechers, Davis, Tranel and Hager2019). Similarly, in a greenhouse study, waterhemp that was resistant to PS II and HPPD inhibitors exhibited a synergistic response to metribuzin at 191 g ha−1 + mesotrione at 53 g ha−1 applied POST, indicating that this may be a viable option for controlling atrazine- and HPPD-inhibitor-resistant waterhemp in corn (O’Brien et al. Reference O’Brien, Davis and Riechers2018).
PRE herbicides serve as a foundation for herbicide-resistant weed management; however, using a PRE and a POST herbicide from the same site of action is not a recommended strategy because it can potentially lead to an increase in the frequency of resistance over time (Hausman et al. Reference Hausman, Tranel, Riechers, Maxwell, Gonzini and Hager2013; Wuerffel et al. Reference Wuerffel, Young, Tranel and Young2015). Therefore, herbicides from alternative sites of action should be included in herbicide programs when HPPD-inhibitor-resistant weeds are present in the field (Chahal and Jhala Reference Chahal and Jhala2018b). For example, a PRE application of isoxaflutole at 105 g ha−1 plus mesotrione 210 g ha−1 provided <65% control of HPPD-inhibitor-resistant waterhemp at 4 WAT compared with >85% control by using acetochlor 1,680 g ha−1 applied PRE in corn (Hausman et al. Reference Hausman, Tranel, Riechers, Maxwell, Gonzini and Hager2013). In a similar study conducted in soybean, flumioxazin at 70 g ha−1, sulfentrazone 280 g ha−1, metribuzin 420 g ha−1, or pyroxasulfone 210 g ha−1 applied PRE provided >85% control of HPPD-inhibitor-resistant waterhemp (Hausman et al. Reference Hausman, Tranel, Riechers, Maxwell, Gonzini and Hager2013). This strategy would potentially reduce the number of survivors, thereby delaying the selection of resistance alleles in the population (Wuerffel et al. Reference Wuerffel, Young, Tranel and Young2015).
Relatively fewer POST herbicide options are available to control HPPD-inhibitor-resistant Palmer amaranth and waterhemp in corn and soybean crops (Jhala et al. Reference Jhala, Knezevic, Ganie, Singh, Chauhan and Mahajan2014a). Glufosinate, 2,4-D, or dicamba are among the few POST herbicides that provide more than 80% control; for example, Jhala et al. (Reference Jhala, Sandell, Rana, Kruger and Knezevic2014b) reported that glufosinate (450 g ha−1), 2,4-D ester (560 g ha−1), or dicamba (560 g ha−1) provided >80% control of HPPD-inhibitor-resistant Palmer amaranth 3 WAT. Similarly, 740 g ha−1 of glufosinate or 280 g ha−1 of dicamba provided >90% control of HPPD inhibitor-resistant waterhemp at 3 WAT (Sarangi et al. Reference Sarangi, Stephens, Barker, Patterson, Gaines and Jhala2019). Glufosinate used at 595 g ha−1 applied alone or mixed with dicamba at 280 g ha−1 provided ≥79% control of HPPD-inhibitor-resistant Palmer amaranth 4 WAT in corn (Chahal and Jhala Reference Chahal and Jhala2018a). Additionally, Oliveira et al. (Reference Oliveira, Jhala, Gaines, Irmak, Amundsen, Scott and Knezevic2017) reported that mixing metribuzin (210 g ha−1) to a premix of mesotrione + atrazine (650 g ha−1) applied POST in corn, controlled HPPD-inhibitor-resistant waterhemp by >90% at 3 WAT.
Although herbicides such as metribuzin, pyroxasulfone, glufosinate, or dicamba applied alone can control early- to mid-season cohorts of Palmer amaranth and waterhemp, a season-long control of these weed species is rarely achieved due to their extended period of emergence (Hager et al. Reference Hager, Wax, Simmons and Stoller1997; Keeley et al. Reference Keeley, Carter and Thullen1987). Therefore, multiple herbicide applications (PRE followed by POST), specifically overlapping residual herbicides, are recommended to achieve a season-long control of HPPD-inhibitor-resistant weeds. For example, a premix of acetochlor + clopyralid + flumetsulam (1,190 g ha−1), or saflufenacil + dimethenamid-P (780 g ha−1) applied PRE provided >90% control of HPPD-inhibitor-resistant Palmer amaranth for 3 wk; however, control was reduced to <70% later in the season (Chahal and Jhala Reference Chahal and Jhala2018b). In the same study, glyphosate (870 g ha−1) + dicamba (280 g ha−1) was needed to obtain >96% control. Similarly, overlapping residual herbicide programs, including pyroxasulfone (110 g ha−1) + saflufenacil (75 g ha−1), or saflufenacil + dimethenamid-P (586 g ha−1) applied PRE followed by glyphosate (870 g ha−1) + diflufenzopyr + dicamba (157 g ha−1) + pyroxasulfone (91 g ha−1), or glyphosate + dicamba + diflufenzopyr (157 g ha−1) + pendimethalin (1,060 g ha−1) applied POST controlled HPPD-inhibitor-resistant Palmer amaranth >95% at corn harvest (Chahal et al. Reference Chahal, Ganie and Jhala2018a). In a study conducted in a conventional corn crop, herbicide programs including acetochlor (2,130 g ha−1), or mesotrione + S-metolachlor + atrazine (2,780 g ha−1) applied PRE, followed by dicamba + diflufenzopyr (196 g ha−1) applied POST, controlled HPPD-inhibitor-resistant Palmer amaranth >95% (Chahal et al. Reference Chahal, Irmak, Gaines, Amundsen, Jugulam, Jha, Travlos and Jhala2018b). Similarly, dicamba + thiencarbazone-methyl + atrazine or dicamba + ABMS (acetochlor + bicyclopyrone + mesotrione + S-metolachlor) applied PRE followed by ABMS alone or in a mixture with atrazine, S-metolachlor, or mesotrione applied early POST provided 85% to 6% control of glyphosate and mesotrione-resistant Palmer amaranth at 2 wk after early POST and 2 and 7 wk after late POST in glyphosate/glufosinate-resistant corn in central Kansas (Liu et al. Reference Liu, Kumar, Jhala, Jha and Stahlman2021).
While sequential applications of PRE followed by POST herbicides with multiple sites of action can control HPPD-inhibitor-resistant Palmer amaranth and waterhemp, relying on a single control tactic would potentially enhance selection pressure for the evolution of multiple-herbicide-resistant weeds. Therefore, diversified weed management strategies, including cultural, biological, mechanical, and chemical weed management (with multiple sites of action), are needed to manage herbicide-resistant weed seed banks. More specifically, multi-tactic strategies that target multiple life stages of the weed, including understanding reproductive biology and potential for pollen-mediated gene flow, are required (Jhala et al. Reference Jhala, Beckie, Mallory-Smith, Jasieniuk, Busi, Norsworthy, Bagavathiannan, Tidemann and Geddes2021a, Reference Jhala, Norsworthy, Ganie, Sosnoskie, Beckie, Mallory-Smith, Liu, Wei, Wang and Stoltenberg2021b). This can be accomplished by using an effective multiple-sites-of-action herbicide program, using cover crops, planting corn or soybean in narrow rows, using a harvest weed seed control method, and adopting diversified crop rotations (Mohler et al. Reference Mohler, Teasdale and DiTommaso2021; Striegel and Jhala Reference Striegel and Jhala2022). The increasing use of HPPD-inhibitor herbicides in agronomic crops requires research on herbicide interactions and alternative herbicides or methods for controlling multiple herbicide-resistant weeds.
Interactions of HPPD-Inhibitor Herbicides with Other Herbicides
The HPPD-inhibiting herbicides are commonly mixed with other herbicides, particularly PS II-inhibiting herbicides, to increase weed control and spectrum. The assumption of an herbicide combination is that each herbicide acts independently when applied together (i.e., additive); however, that is not always the case. Weed control from a mixture of two herbicides may be greater (synergistic) or less than (antagonistic) the combined effect of the herbicides applied alone (Colby Reference Colby1967; Hatzios and Penner Reference Hatzios and Penner1985).
Efficacy
The HPPD-inhibiting herbicides applied PRE with a PS II-inhibitor herbicide can have both additive and synergistic effects. In greenhouse studies, atrazine + mesotrione applied PRE were additive for control of velvetleaf (Abutilon theophrasti Medik.) and ivyleaf morningglory (Ipomoea hederacea Jacq.); however, several rate combinations indicated synergistic control (Bollman et al. Reference Bollman, Kells and Penner2006). In field experiments, isoxaflutole + metribuzin applied PRE exhibited additive or synergistic control of Canada fleabane, common lambsquarters, Amaranthus spp., common ragweed, velvetleaf, Setaria spp., barnyardgrass [Echinochloa crus-galli (L.) Beauv.], and fall panicum (Panicum dichotomiflorum Michx.; Ditschun et al. Reference Ditschun, Soltani, Robinson, Tardif, Kaastra and Sikkema2016; Smith et al. Reference Smith, Soltani, Kaastra, Hooker, Robinson and Sikkema2019b). In contrast, control of a population of HPPD-inhibitor-resistant and PS II-inhibitor-resistant Palmer amaranth with mesotrione or topramezone applied PRE with atrazine was additive (Chahal and Jhala Reference Chahal and Jhala2018a).
The literature is replete with observations of additive or synergistic weed control when HPPD-inhibiting herbicides are applied POST with a PS II inhibitor. Mesotrione + atrazine (Abendroth et al. Reference Abendroth, Martin and Roeth2006; Armel et al. Reference Armel, Hall, Wilson and Cullen2005; Creech et al. Reference Creech, Monaco and Evans2004), mesotrione + bromoxynil or metribuzin (Abendroth et al. Reference Abendroth, Martin and Roeth2006) were consistent for controlling several annual weeds as well as Canada thistle [Cirsium arvense (L.) Scop.] compared to mesotrione applied alone. Mixing atrazine with tolpyralate improved control (Metzger et al. Reference Metzger, Soltani, Raeder, Hooker, Robinson and Sikkema2018) or reduced the biologically effective dose of tolpyralate for control of weeds commonly found in corn production fields in Nebraska (Osipitan et al. Reference Osipitan, Scott and Knezevic2018). In research plots throughout North America, mixing atrazine with tembotrione reduced variability in weed control and sweet corn yield variation (Williams et al. Reference Williams, Boydston, Peachey and Robinson2011a, Reference Williams, Boydston, Peachey and Robinson2011b). Similar findings were observed with atrazine + isoxaflutole, mesotrione, topramezone, tembotrione, or tolpyralate for waterhemp control in field corn (Willemse et al. Reference Willemse, Soltani, Benoit, Jhala, Hooker, Robinson and Sikkema2021a). Furthermore, atrazine improved the efficacy of pyrasulfotole + bromoxynil for weed control in grain sorghum (Reddy et al. Reference Reddy, Stahlman, Geier, Thompson, Currie, Schlegel, Olson and Lally2013).
Synergism between an HPPD inhibitor and a PS II inhibitor applied POST can be observed in herbicide-resistant weed populations. Synergistic control with mesotrione + atrazine has been observed in PS II-inhibitor-resistant redroot pigweed (Amaranthus retroflexus L.) (Hugie et al. Reference Hugie, Bollero, Tranel and Riechers2008; Sutton et al. Reference Sutton, Richards, Buren and Glasgow2002) and PS II-inhibitor-resistant wild radish (Walsh et al. Reference Walsh, Stratford, Stone and Powles2012), including temporally separated herbicide applications (e.g., atrazine PRE followed by mesotrione POST; Woodyard et al. Reference Woodyard, Hugie and Riechers2009a). Palmer amaranth, including a PS II-inhibitor-resistant population, exhibited synergistic control with atrazine + mesotrione or tembotrione, but not atrazine with tolpyralate or topramezone (Kohrt and Sprague Reference Kohrt and Sprague2017). Synergistic control of multiple-herbicide-resistant waterhemp was observed with mesotrione + bromoxynil or bentazon; and tolpyralate + bromoxynil (Willemse et al. Reference Willemse, Soltani, David, Jhala, Robinson and Sikkema2021b). In contrast, activity of isoxaflutole or mesotrione applied POST with metribuzin on waterhemp populations varying in herbicide resistance traits was mostly additive (O’Brien et al. Reference O’Brien, Davis and Riechers2018).
Mixing an HPPD inhibitor with a PS II inhibitor does not always result in synergistic weed control. Volunteer potato (Solanum tuberosum L.) control with mesotrione, tembotrione, or topramezone applied POST was not improved when mixed with atrazine, bentazon, or bromoxynil (Koepke-Hill et al. Reference Koepke-Hill, Armel, Wilson, Hines and Vargas2010). Advances have been made in understanding the mechanisms that account for synergistic weed control from mixing HPPD and PS II inhibitors. Armel et al. (Reference Armel, Hall, Wilson and Cullen2005) reported that uptake, translocation, and metabolism of mesotrione did not account for improved control of Canada thistle with mesotrione + atrazine. Mesotrione absorption in Palmer amaranth increased when it was mixed with atrazine, partially accounting for observed synergism (Chahal et al. Reference Chahal, Jugulam and Jhala2019). PS II inhibitors compete with plastoquinones for the D1 protein binding site, disrupting electron transfer in PS II. The inability to transfer electrons creates triplet chlorophyll and singlet oxygen that destroy plant membranes (Hess Reference Hess2000). Armel et al. (Reference Armel, Rardon, McComrick and Ferry2007) showed that carotenoid biosynthesis inhibitor increased the binding efficiency and efficacy of PS II inhibitor by reducing the reformation of the D1 protein following initiation of photo inhibition.
Several factors influence the synergism of an HPPD inhibitor applied in a mixture with a PS II inhibitor. For both PRE and POST applications, the herbicide rate influences the extent of synergistic weed control (Bollman et al. Reference Bollman, Kells and Penner2006; Hugie et al. Reference Hugie, Bollero, Tranel and Riechers2008). In addition to the application rate of the HPPD inhibitor, synergistic weed control was observed more frequently with triketone herbicides (mesotrione and tembotrione) compared to pyrazolone herbicides (topramezone and tolpyralate; Kohrt and Sprague Reference Kohrt and Sprague2017). Adverse environmental conditions (e.g., inadequate rainfall) influence plant response to a mixture of HPPD and PS II inhibitors applied PRE (Smith et al. Reference Smith, Soltani, Kaastra, Hooker, Robinson and Sikkema2019b) and POST (Woodyard et al. Reference Woodyard, Bollero and Riechers2009b). Moreover, the time of POST herbicide application can influence the plant response to HPPD and PS II inhibitors (O’Brien et al. Reference O’Brien, Davis and Riechers2018).
HPPD-inhibitor herbicides can interact with herbicides other than PS II inhibitors. The synthetic auxin triclopyr improved foliar uptake of mesotrione and control of smooth crabgrass (Yu and McCullough Reference Yu and McCullough2016). Conversely, a mixture of an HPPD inhibitor and an ALS inhibitor can be antagonistic. For example, reduced efficacy of sulfonylurea herbicides applied with mesotrione + atrazine for control of Setaria spp. (Schuster et al. Reference Schuster, Al-Khatib and Dille2008) was due to decreased absorption, and in some cases reduced translocation of nicosulfuron (Schuster et al. Reference Schuster, Al-Khatib and Dille2007). Not only can an HPPD inhibitor antagonize an ALS inhibitor for annual grass control, but an ALS inhibitor can also antagonize the HPPD inhibitor (Kaastra et al. Reference Kaastra, Swanton, Tardif and Sikkema2008).
Crop Tolerance
Field corn production systems rely extensively on a mixture of HPPD and PS II inhibitors. Considerable field research demonstrates excellent crop tolerance with their mixtures (Johnson et al. Reference Johnson, Young and Matthews2002; Osipitan et al. Reference Osipitan, Scott and Knezevic2018; Stephenson et al. Reference Stephenson, Bond, Walker, Bararpour and Oliver2004; Whaley et al. Reference Whaley, Armel, Wilson and Hines2006; Willemse et al. Reference Willemse, Soltani, Benoit, Jhala, Hooker, Robinson and Sikkema2021a). Additional research shows that the synergistic effect of HPPD and PS II inhibitors for weed control was not observed on sweet corn response (Choe et al. Reference Choe, Williams, Boydston, Huber, Huber and Pataky2014). Sweet corn injury from tembotrione was influenced by the safener isoxadifen and the genotypic class at a P450 locus (Nsf1; Williams and Pataky Reference Williams and Pataky2010). The extent to which crops other than corn respond to a mixture of HPPD inhibitor and other herbicides has been studied. In an herbicide carryover study, a mixture of atrazine and mesotrione accentuated crop injury and yield losses in broccoli (Brassica oleracea var. italica), carrot (Daucus carota L.), cucumber (Cucumis sativus L.), and onion (Alium cepa L.; Robinson Reference Robinson2008). Grain sorghum was not injured by pyrasulfotole + bromoxynil applied alone or with synthetic auxin; however, the mixing of carfentrazone, a PPO inhibitor, increased phytotoxicity (Besançon et al. Reference Besançon, Riar, Heiniger, Weisz and Everman2016). Isoxaflutole + metribuzin applied PRE on isoxaflutole-resistant soybean injured the crop in environments with the most rainfall, and injury was often synergistic (Smith et al. Reference Smith, Soltani, Kaastra, Hooker, Robinson and Sikkema2019b). Sugarcane displayed transient injury symptoms when topramezone was mixed with ametryn or metribuzin compared with topramezone applied alone (Negrisoli et al. Reference Negrisoli, Odero, MacDonald, Sellers and Laughinghouse2020).
Future of HPPD-Inhibiting Herbicides
HPPD-inhibitor herbicides continue to be researched, patented, and commercialized by agrochemical companies. Benquitrione is currently in development for use in sorghum. A new class of HPPD inhibitor, azole carboxamides, were first disclosed by Bayer Crop Science in 2011 (Koehn et al. Reference Koehn, Tiebes, Van Almsick, Ahrens, Heinemann, Braun, Schmitt, Willms, Feucht and Rosinger2011). While no molecules have yet been commercialized, azole carboxamides have come to dominate the HPPD-inhibitor patent literature, with contributions from Syngenta, BASF, Nissan, KingAgroot, Nippon Soda, and SSARD, in addition to follow-up inventions from Bayer Crop Science. There have been more than 120 international patent applications for azole carboxamides, which represent applications of more than 50% of all HPPD-inhibiting herbicides since 2012. Herbicides from this class are in development that are expected to be available commercially around 2030. Azole carboxamides have different physical properties compared to the previously described classes with a different metabolism, which could potentially overcome non-target-site resistance (Jugulam and Shyam Reference Jugulam and Shyam2019). Patent applications that describe new herbicidal active ingredients will typically include thousands of compounds. However, companies will typically include a low number, often one, of these molecules in additional patent applications for use in mixtures with other active ingredients, for use in herbicide-resistant crops, or for inventions in the synthetic process. These additional patents hint to these specific molecules being of particular interest and likely candidates for further development (Figure 10).

Figure 10. Examples of recently submitted patents for herbicides that inhibit 4-hydroxyphenylpyruvate dioxygenase (HPPD) from Bayer Crop Science, Nissan, and KingAgroot.
Future of HPPD-Inhibitor-Resistant Crops
Research and development of HPPD-inhibitor-resistant crop traits began in the early 2000s. Traits were initially created that were useful in PRE weed control programs. Due to the commercial success of glyphosate-resistant crops, the impact of the early HPPD-inhibitor-resistant crop traits were not as large as anticipated. In the last decade, however, interest in developing HPPD-inhibitor-resistant crop traits has re-emerged and is being driven by the impact of wide-scale occurrence of glyphosate-resistant weeds. This has led to the need for alternatives, and new HPPD-inhibitor crop traits are actively being developed across the crop protection industry, primarily for use in soybean and cotton.
Certain grass crop species such as corn are resistant to most HPPD-inhibitor herbicides (Mitchell et al. Reference Mitchell, Bartlett, Fraser, Hawkes, Holt, Townson and Wichert2001); therefore, HPPD inhibitors such as mesotrione can be applied PRE and POST in corn, POST in oats and sugarcane, but it is labeled only for PRE weed control in sorghum. Nonetheless, HPPD-inhibitor-resistant lines in dicot species [e.g., tobacco (Nicotiana tabacum L.) and soybean that would be otherwise highly sensitive to these herbicides] have been developed. Tobacco transformed with an HPPD gene from wheat showed resistance to mesotrione (Hawkes et al. Reference Hawkes, Holt, Andrews, Thomas, Langford, Hollingworth and Mitchell2001, Reference Hawkes, Langford, Viner, Blain, Callaghan, Mackay, Hogg, Singh and Dale2019). Siehl et al. (Reference Siehl, Tao, Albert, Dong, Heckert, Madrigal, Lincoln-Cabatu, Lu, Fenwick, Bermudez, Sandoval, Horn, Green, Hale, Pagano, Clark, Udranszky, Rizzo, Bourett, Howard, Johnson, Vogt, Akinsola and Castle2014) developed transgenic soybean that is resistant to isoxaflutole, mesotrione, and tembotrione with increased selectivity and a wide spectrum of weed control. In addition, isoxaflutole-resistant soybean has been developed and is available for commercial cultivation in the United States; however, its adoption is limited due to restriction in use of isoxaflutole (Alite 27) in certain counties in states such as Nebraska (Mausbach et al. Reference Mausbach, Irmak, Sarangi, Lindquist and Jhala2021).
Bayer Crop Science has a long history of involvement in the development of HPPD-inhibitor-resistant crop traits, with efforts mainly focused on the expression of an herbicide-insensitive bacterial HPPD enzyme from Pseudomonas fluorescens. A mutated form of the gene that carries a mutation at amino acid position G336W had increased tolerance to isoxaflutole. This gene is used in the FG72 soybean in commercial use (Matringe et al. Reference Matringe, Sailland, Pelissier, Rolland and Zink2005). Work initiated by the former Monsanto business (now part of Bayer Crop Science) also focused on development of an HPPD trait and a planned launch of this trait (HT4) in soybean is expected in the late 2020s. Details of the trait gene used are unknown at the time of writing. This trait is expected to be stacked with 2,4-D, glyphosate, glufosinate, and dicamba. A further development known as HT5 is expected to launch later, adding resistance to PPO-inhibiting herbicides (Reither Reference Reither2021).
BASF acquired much of Bayer Crop Science’s HPPD-inhibitor-resistant crop technology during the crop protection industry consolidation period in the mid-2010s. As such, BASF is now bringing to market products containing the HPPD trait from FG72 soybean into other crops such as cotton (Steadman Reference Steadman2021). A further development is the HPPD trait known as pfHPPD-4, which is expressed in the GMB151 soybean line. This is a Pseudomonas HPPD gene that carries four mutations compared to a single mutation that occurs in the FG72 trait (Olson and Weeks Reference Olson and Weeks2020).
Syngenta’s involvement with HPPD inhibitor-resistant crop traits dates to 2000, when several HPPD target genes, including the wild oat (Avena sativa L.) gene were characterized. The Avena sativa HPPD gene was later used to develop a soybean (SYT-0H2) that is resistant to mesotrione applied PRE (Hawkes et al. Reference Hawkes, Langford, Viner, Blain, Callaghan, Mackay, Hogg, Singh and Dale2019). Syngenta has recently disclosed the invention of a series of further evolved Avena HPPD target site genes that have much enhanced resistance to a broad range of HPPD inhibitors including mesotrione and bicyclopyrone (Hawkes et al. Reference Hawkes, Langford, Viner, Blain, Callaghan, Mackay, Hogg, Singh and Dale2019). These genes are capable of providing resistance to POST applications of these herbicides (Hawkes et al. Reference Hawkes, Langford, Viner, Blain, Callaghan, Mackay, Hogg, Singh and Dale2019). Plant Arc Bio has applied for an exemption for an HPPD-inhibitor trait based on a fungal (Trichoderma harzianum spp.) HPPD gene. The target crops are soybean and cotton (PlantArcBio 2022; Shatlin Reference Shatlin2021), although the spectrum of herbicide resistance of this trait is unknown as of 2022. An alternative technology has been described by the NARO Institute in Japan, which involves the metabolic degradation of certain HPPD-inhibitor formulas such as mesotrione. The His-1 gene was discovered as a part of a project to study the differences in herbicide sensitivity among rice cultivars. The metabolic nature of the gene means that this trait is likely to be narrower in the resistance spectrum compared with target-site-based approaches (Maeda et al. Reference Maeda, Murata, Sakuma, Takei, Yamazaki, Karim, Kawata, Hirose, Kawagishi-Kobayashi, Taniguchi, Suzuki, Sekino, Ohshima, Kato, Yoshida and Tozawa2019). Given the renewed investment in the development of HPPD-inhibitor-resistant crops, it is expected that HPPD-inhibiting herbicides will play an important role in weed control programs in soybean and cotton from the late 2020s onward. If such traits can be combined with the next generation of HPPD-inhibitors, their usefulness will likely be extended into the future. Management of multiple herbicide–resistant crop volunteers might be challenging, and future research should focus on this topic (Jhala et al. Reference Jhala, Beckie, Peters, Culpepper and Norsworthy2021c).
Acknowledgments
This project was partially supported by the Nebraska Agricultural Experiment Station with funding from the Hatch Act through the U.S. Department of Agriculture (USDA) National Institute of Food and Agriculture Project No. NEB-22-396. This project was also supported by the USDA–National Institute of Food and Agriculture Nebraska Extension Implementation Program. No conflicts of interest have been declared.