Introduction
The U.S. Great Plains extends from the Canadian border in the north to Texas in the south and is bordered by Mississippi River in the east and the Rocky Mountains in the west (Unger and Baumhardt Reference Unger and Baumhardt2001). This region covers the central portions of the continental United States and accounts for more than 60% of total wheat (Triticum aestivum L.) production in the United States (Paulsen and Shroyer Reference Paulsen and Shroyer2008). The majority of the cropland in this region encompasses a semiarid agroecosystem characterized by hot summer days with cold and dry winters (Lenssen et al. Reference Lenssen, Johnson and Carlson2007). Soil moisture is the most limiting factor for crop production, generally decreasing from east to west with an average annual rainfall of <762 mm in the eastern portions of the Great Plains down to <381 mm in Montana, Wyoming, and far west Texas (Nielsen Reference Nielsen2018; Peterson and Westfall Reference Peterson and Westfall2004; Shafer et al. Reference Shafer, Ojima, Antle, Kluck, McPherson, Peterson, Scanlon and Sherman2014). In addition to limited rainfall, soils are shallow, low in soil organic matter content, and prone to wind erosion. Temporal and spatial climatic variation with extended drought periods further challenge crop production in this region (Hansen et al. Reference Hansen, Allen, Baumhardt and Lyon2012). The Dust Bowl period during the 1930s led to the adoption of soil conservation practices, including fallow and minimum-tillage and no-tillage (NT) crop production across the region (Hansen et al. Reference Hansen, Allen, Baumhardt and Lyon2012; Smika and Wicks Reference Smika and Wicks1968). The transition to NT production was mainly designed to prevent wind and water erosion of the topsoil layer, improve soil organic matter, and conserve soil moisture.
Winter wheat–fallow (W–F) is a major NT dryland crop rotation in the Northern Great Plains (NGP) (Figure 1). Winter wheat–summer crop [corn (Zea mays L.), soybean [Glyicine max (L.) Merr.], grain sorghum [Sorghum bicolor (L.) Moench ssp. bicolor], or sunflower (Helianthus annuus L.)]–fallow (W–S–F) is the most dominant crop rotation in the Southern and Central Great Plains (Figure 1) (Hansen et al. Reference Hansen, Allen, Baumhardt and Lyon2012; Lenssen et al. Reference Lenssen, Johnson and Carlson2007; Nielsen and Vigil Reference Nielsen and Vigil2018; Peterson and Westfall Reference Peterson and Westfall2004). The fallow phase in the rotation was originally included to store and conserve moisture in the soil profile from rainfall or snow events for successful establishment of a subsequent cash crop (Nielsen and Vigil Reference Nielsen and Vigil2010). The fallow period limits the occurrence of crop failure in drier years, stabilizes crop yields across years, and more importantly, prevents soil erosion and soil nutrient depletion, particularly when NT is adopted (Lenssen et al. Reference Lenssen, Johnson and Carlson2007; Nielsen and Vigil, Reference Nielsen and Vigil2018). Depending on location and crop rotation scheme, the fallow period extends from 10 mo (W–S–F) to almost 14 to 15 mo (W–F) after wheat harvest. The adoption of NT practices has increased soil water storage and cropping system intensification across the Great Plains region. More recently, many growers have started intensifying crop rotations to eliminate fallow, and some are eliminating wheat in their rotations for economic reasons. For instance, pulse crops such as field pea (Pisum sativum L.), lentil (Lens culinaris Medik.), and chick pea (Cicer arietinum L.) have replaced a portion of this fallow period in the northern parts (Miller et al. Reference Miller, Gan, Mcconkey and McDonald2003). Similarly, several new crops have been evaluated in 3- or 4-yr rotation schemes by including cereal crops (Nielsen and Vigil Reference Nielsen and Vigil2018; Schlegel et al. Reference Schlegel, Lamm, Assefa and Stone2018), legumes as forage and grain crops (Holman et al. Reference Holman, Arnet, Dille, Maxwell, Obour, Roberts, Roozeboom and Schlegel2018; Lyon et al. Reference Lyon, Blumenthal, Burgener and Harveson2004), and recently oilseed crops (Obour et al. Reference Obour, Chen, Sintim, McVay, Lamb, Obeng, Mohammed, Khan, Afshar and Zheljazkov2018) for potential replacement of fallow in the Central Great Plains (Figure 1). Despite these efforts to increase cropping intensity, crop rotations in the region still include a fallow phase, and most growers practice fallow as a strategy to minimize risk of drought and crop failure. For example, two fallow periods occur in a typical 3-yr (W–S–F) crop rotation. The first fallow period extends from wheat harvest until summer crop planting the next year, and the second fallow period extends from summer crop harvest until winter wheat planting in the following year.
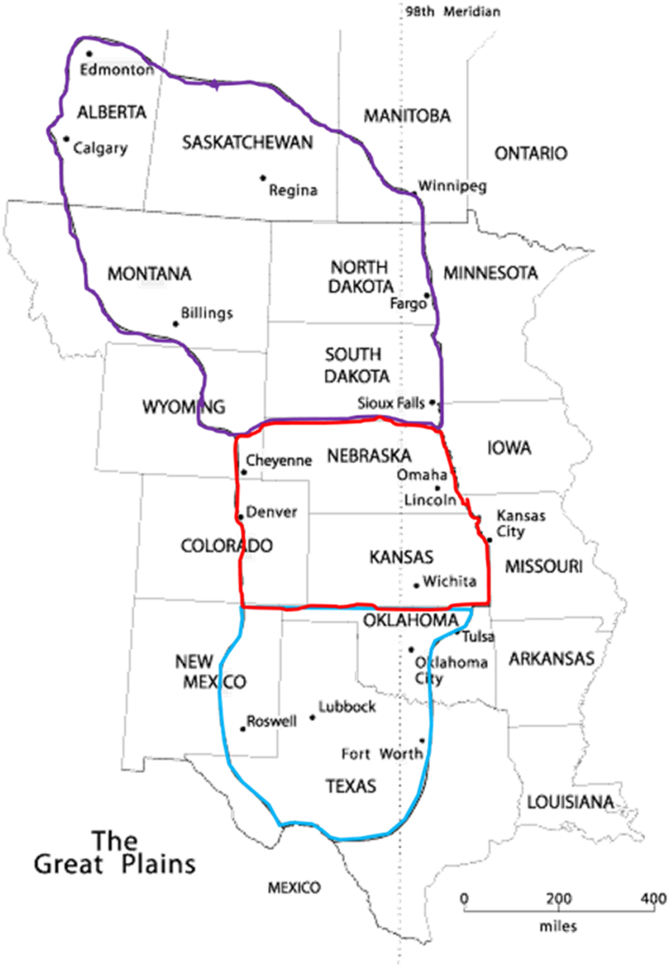
Figure 1. Map of the Great Plains showing three main regions: (1) Northern Great Plains (marked by purple line), (2) Central Great Plains (marked by red line), and (3) Southern Great Plains (marked by light blue line). Adapted from Center for Great Plains Studies, University of Nebraska–Lincoln.
Weed control during fallow is typically accomplished through a combination of various tillage practices and herbicide applications in a minimum-tillage system or with herbicide only in NT. The successful adoption of NT fallow-based crop production in the semiarid Great Plains was possible due to effective weed control with the use of herbicides (Wicks and Smika Reference Wicks and Smika1973; Wicks et al. Reference Wicks, Martin and Mahnken1993). Weed control in the fallow phase of the crop rotation is crucial, as weed infestations can reduce available soil moisture and nutrients, negatively affecting subsequent cash crop yield (Wicks and Smika Reference Wicks and Smika1973). In general, most fallow fields could have three to five applications of herbicides (predominantly glyphosate-based tank mixtures) per year for weed control. The continuous and repetitive use of herbicides with same site(s) of action (SOA) has led to the evolution of herbicide resistance in weed populations across the region (Heap Reference Heap2019). A lack of diversity in weed control programs has further led to the evolution of multiple herbicide resistance in several weed populations in the Great Plains in recent years. Dwindling herbicide options coupled with ever-increasing reports of multiple herbicide-resistant (MHR) weed populations necessitates the development of ecologically based weed management options for the region. With low grain prices and the high cost of controlling MHR weeds, some producers are returning to tillage as a strategic weed management tool. This poses a major threat to gains and investments made in soil conservation practices over the last several decades. Integration of cover crops (CCs) to replace portions of the fallow period in dryland NT crop production may provide effective and sustainable management of HR weed populations while enhancing the productivity and profitability of dominant cropping systems in the region. Growing a CC in place of fallow as part of the crop rotation can suppress weeds and reduce frequency of herbicide use. Several NT dryland producers in the region have also shown interest in growing CCs for controlling HR weeds and gaining soil health benefits. This recent trend has renewed interest among land managers, academic weed scientists, ecologists, agronomists, and soil scientists to improve their understanding of CC management strategies for weed suppression and soil health benefits in the region. In the literature, several research and review articles have documented the effects of CCs on weed suppression, soil health benefits, and subsequent cash crop yields across various regions in the United States. However, there is a paucity of published information on this topic in NT dryland production systems of the Great Plains. Previous review articles on CCs in the semiarid Great Plains have focused on soil health (Ghimire et al. Reference Ghimire, Ghimire, Mesbah, Idowu, O’Neill, Angadi and Shukla2018) or agronomy and water use of CCs (Unger and Vigil Reference Unger and Vigil1998), with little discussion on using CCs for weed suppression in the region. This review article aims to document weed species shifts that have occurred in response to herbicide use patterns in NT dryland production systems and to summarize the literature supporting utilization of CCs for control of these problematic species. This review will discuss opportunities and scenarios for use of CCs to suppress weeds, including agronomic considerations and challenges of adopting CC in this semiarid region. Finally, we will suggest future research directions and extension needs to be able to effectively use CCs as an integrated weed management (IWM) tool in the Great Plains.
Emerging Weed Management Issues in the Great Plains
Weed Control Practices and Weed Shifts
Weed control practices, including tillage, crop rotation, herbicide use patterns, and other production practices are known to influence weed community richness and population dynamics (Johnson et al. Reference Johnson, Davis, Kruger and Weller2009). Two key changes in Great Plains agriculture have occurred over the past few decades: (1) adoption of soil conservation practices (changing from preplant conventional tillage to NT production) (Shaner Reference Shaner2000; Swanton et al. Reference Swanton, Clements and Derksen1993; Wilson et al. Reference Wilson, Miller, Westra, Kniss, Stahlman, Wicks and Kachman2007) and (2) widespread adoption of glyphosate-resistant (GR) crops.
Effective weed control with use of herbicides in the early to mid-1970s facilitated the adoption of NT production practices in the Great Plains (Hansen et al. Reference Hansen, Allen, Baumhardt and Lyon2012; Wicks and Smika Reference Wicks and Smika1973; Wicks et al. Reference Wicks, Martin and Mahnken1993). Weed control strategies shifted away from tillage and became more reliant on herbicides. However, small-seeded broadleaf weeds such as horseweed [Conyza canadensis (L.) Cronquist], kochia [Bassia scoparia (L.) A.J. Scott], Palmer amaranth (Amaranthus palmeri S. Watson), common lambsquarters (Chenopodium album L.), and Russian thistle (Salsola tragus L.) and grass weeds such as downy brome (Bromus tectorum L.), wild oat (Avena fatua L.), foxtail species (Setaria spp.), and tumble windmill grass (Chloris verticillata Nutt.) have become problematic weed species over time in NT production systems of the Great Plains (Buhler and Oplinger Reference Buhler and Oplinger1990; Jha et al. Reference Jha, Kumar and Lim2016; Nichols et al. Reference Nichols, Verhulst, Cox and Govaerts2015). This is mainly because these small-seeded weeds can easily germinate on or near the soil surface under NT fallow conditions. In addition, a majority of these weed species are also prolific seed producers and can quickly replenish the soil seedbank during the fallow period in the absence of crop competition. In contrast, populations of large-seeded broadleaf weeds such as velvetleaf (Abutilon theophrasti Medik.), common cocklebur (Xanthium strumarium L.), and H. annuus have declined over time in this region (Anderson et al. Reference Anderson, Tanaka, Black and Schweizer1998; Buhler et al. Reference Buhler, Mester and Kohler1996). The NT soil surface was probably not a biologically beneficial site for successful germination and emergence of large-seeded compared with small-seeded weed species (Buhler et al. Reference Buhler, Mester and Kohler1996). Large seeds on the soil surface under NT conditions are also exposed to higher mortality risks through predation (insects, diseases, birds, animals, etc.) and weather variability (Baraibar et al. Reference Baraibar, Westerman, Carrión and Recasens2009; Nichols et al. Reference Nichols, Verhulst, Cox and Govaerts2015).
Nevertheless, the extensive and repeated use of glyphosate for weed control in fallow, pre–crop seeding, and postharvest (NT burndown) scenarios has led to the evolution of glyphosate resistance in several weed species in the region (Heap Reference Heap2019; Jha et al. Reference Jha, Kumar and Lim2016; Stahlman Reference Stahlman2016). This was partly due to glyphosate use at lower than recommended rates by growers to minimize cost of weed control in NT fallow. Currently, the reported cases of GR weed species across the Great Plains include C. canadensis, B. scoparia, A. palmeri, and S. tragus (Heap Reference Heap2019). Among all these reported GR weed species, glyphosate resistance is widespread among B. scoparia populations across all the Great Plains states (Kumar et al. Reference Kumar, Jha, Jugulam, Yadav and Stahlman2018a).
GR crops became available in the United States in 1996, allowing producers to use glyphosate for in-season control of broadleaf and grass weeds. By 2005, >90% of total U.S. acreage planted to soybean and cotton (Gossypium hirsutum L.) and nearly 50% of corn had the GR trait (Kniss Reference Kniss2018; Sankula Reference Sankula2006). The GR crops in the U.S. Great Plains occupy a significant portion of the total agricultural land [alfalfa (Medicago sativa L.), canola (Brassica napus L.), corn, cotton, soybean, and sugar beet (Beta vulgaris L.) being the most common GR crops]. The rapid adoption of GR crops, along with the dramatic increase in glyphosate use (almost 8-fold increase between 1996 and 2005), replaced preplant tillage, POST cultivation, and other selective herbicides as a means of weed control (Givens et al. Reference Givens, Shaw, Kruger, Johnson, Weller, Young, Wilson, Owen and Jordan2009; Kniss Reference Kniss2018). This change in glyphosate use pattern contributed to weed species shifts in major agronomic crops in the United States (Johnson et al. Reference Johnson, Davis, Kruger and Weller2009). For instance, Wilson et al. (Reference Wilson, Miller, Westra, Kniss, Stahlman, Wicks and Kachman2007) documented that two continuous in-crop treatments of glyphosate each year at 0.4 or 0.8 kg ae ha−1 shifted B. scoparia and wild proso millet (Panicum miliaceum L.) populations to predominantly C. album populations in a long-term field study conducted in Scottsbluff, NE.Shaner (Reference Shaner2000) concluded that reduced sensitivity in weed seedlings to glyphosate could occur more frequently than evolution of resistant biotypes, further shifting weed populations from susceptible to ones that are more tolerant.
Herbicide-Resistant Weeds
In NT fallow fields, growers primarily rely on multiple applications of herbicides (predominantly glyphosate-based tank mixtures) to achieve season-long weed control (Fenster and Wicks Reference Fenster and Wicks1982; Moyer et al. Reference Moyer, Roman, Lindwall and Blackshaw1994). Repeated use of herbicides with the same SOAs has resulted in the evolution of herbicide resistance in several weed species across the region. This section briefly reviews documented cases of herbicide resistance in common weed species across the U.S. Great Plains.
Bassia scoparia
A well-detailed review on chronology of herbicide resistance, distribution, mechanisms of resistance, seed germination and emergence characteristics, population dynamics, and IWM strategies to manage HR B. scoparia across North America has recently been published (Kumar et al. Reference Kumar, Jha, Jugulam, Yadav and Stahlman2018a). The first case of HR B. scoparia was discovered in 1976, when atrazine-resistant biotypes were reported in cornfields in Kansas and along railroads in Idaho and Iowa (Heap Reference Heap2019; Kumar Reference Kumar, Jha, Jugulam, Yadav and Stahlman2018a; Stahlman Reference Stahlman2016). Bassia scoparia resistant to acetolactate synthase (ALS)-inhibiting herbicides was first identified from a wheat field in Kansas in 1987 (Primiani et al. Reference Primiani, Cotterman and Saari1990). Currently, a majority of B. scoparia populations in the region are believed to be resistant to ALS-inhibiting herbicides (Kumar et al. Reference Kumar, Jha, Jugulam, Yadav and Stahlman2018a). Cross-resistance to dicamba and/or fluroxypyr was identified in B. scoparia populations collected from wheat/fallow fields in Montana and North Dakota in 1995 (Cranston et al. Reference Cranston, Kern, Hackett, Miller, Maxwell and Dyer2001; Nandula and Manthey Reference Nandula and Manthey2002). Currently, B. scoparia resistant to dicamba and fluroxypyr have been reported from six states: Montana, Idaho, North Dakota, Nebraska, Colorado, and Kansas (Heap Reference Heap2019; Kumar et al. Reference Kumar, Jha, Jugulam, Yadav and Stahlman2018a). Resistance to glyphosate was first confirmed in B. scoparia populations from W–S–F fields in western Kansas in 2007 and is currently reported from two-thirds of the U.S. Great Plains states and three Canadian provinces (Beckie et al. Reference Beckie, Blackshaw, Low, Hall, Sauder, Martin, Brandt and Shirriff2013; Godar et al. Reference Godar, Stahlman, Jugulam and Dille2015a; Hall et al. Reference Hall, Beckie, Low, Shirriff, Blackshaw, Kimmel and Neeser2014; Kumar et al. Reference Kumar, Jha and Reichard2014; Waite et al. Reference Waite, Thompson, Peterson, Currie, Olson, Stahlman and Khatib2013; Wiersma et al. Reference Wiersma, Gaines, Preston, Hamilton, Giacomini, Buell, Leach and Westra2015). In addition, several B. scoparia populations across the region have been documented to have multiple resistance to two (glyphosate and ALS inhibitors), three (glyphosate, ALS inhibitors, and dicamba), or four (glyphosate, ALS inhibitors, dicamba, and atrazine) herbicide SOAs (Heap Reference Heap2019; Kumar et al. Reference Kumar, Jha, Giacomini, Westra and Westra2015, Reference Kumar, Currie, Jha and Stahlman2019a; Stahlman Reference Stahlman2016; Varanasi et al. Reference Varanasi, Godar, Currie, Dille, Thompson, Stahlman and Jugulam2015; Westra et al. Reference Westra, Nissen, Getts, Westra and Gaines2019).
Amaranthus palmeri
Historically, A. palmeri is a common weed species in the midsouthern and southeastern United States, A. palmeri has recently become more problematic in the Southern and Central Great Plains (Figure 1) and is moving farther north (Ward et al. Reference Ward, Webster and Steckel2013). Ward et al. (Reference Ward, Webster and Steckel2013) provided a detailed review on various aspects of A. palmeri, including herbicide-resistance evolution in the United States. Amaranthus palmeri populations in Texas were confirmed resistant to atrazine in 1993, and in Kansas in 1995 (Heap Reference Heap2019). Populations of A. palmeri resistant to ALS inhibitors and glyphosate are reported across the Southern and Central Great Plains (Figure 1) (Chahal et al. Reference Chahal, Varanasi, Jugulam and Jhala2017; Garetson et al. Reference Garetson, Singh, Singh, Dotray and Bagavathiannan2019; Horak and Peterson Reference Horak and Peterson1995; Kumar et al. Reference Kumar, Liu, Boyer and Stahlman2019b, Reference Kumar, Liu and Stahlman2020; Nakka et al. Reference Nakka, Thompson, Peterson and Jugulam2017). Amaranthus palmeri resistant to 4-hydroxphenylpyruvate dioxygenase–inhibiting herbicides was first confirmed in Kansas in 2009, and subsequently in Nebraska and Texas (Garetson et al. Reference Garetson, Singh, Singh, Dotray and Bagavathiannan2019; Jhala et al. Reference Jhala, Sandell, Rana, Kruger and Knezevic2014; Singh et al. Reference Singh, Burgos, Singh, Alcober, Salas-Perez and Shivrain2018). Additionally, A. palmeri populations with multiple resistance to two, three, four, or five herbicide SOAs have become an increasing concern in the Southern and Central Great Plains (Figure 1) (Garetson et al. Reference Garetson, Singh, Singh, Dotray and Bagavathiannan2019; Heap Reference Heap2019; Jhala et al. Reference Jhala, Sandell, Rana, Kruger and Knezevic2014; Kumar et al. Reference Kumar, Liu, Boyer and Stahlman2019b, Reference Kumar, Liu and Stahlman2020; Singh et al. Reference Singh, Burgos, Singh, Alcober, Salas-Perez and Shivrain2018). More recently, an A. palmeri population with multiple resistance to 2,4-D, glyphosate, chlorsulfuron, mesotrione, and atrazine and reduced sensitivity to fomesafen has been reported in Kansas (Kumar et al. Reference Kumar, Liu, Boyer and Stahlman2019b).
Salsola tragus
This C4 summer annual is another troublesome broadleaf weed commonly found in semiarid regions of the Great Plains (Leeson et al. Reference Leeson, Thomas, Hall, Brenzil, Andrews, Brown and Van Acker2005). Salsola tragus is highly problematic in summer fallow periods and in spring/winter wheat across the Northern and Central Great Plains (Figure 1) (Schillinger Reference Schillinger2007; Young Reference Young1986). Effective management of this weed species has been complicated by the evolution of herbicide resistance in several field populations (Heap Reference Heap2019). Resistance to ALS inhibitors in S. tragus populations was first confirmed in Washington and Montana in 1987 (Guttieri et al. Reference Guttieri, Eberlein, Mallory-Smith, Thill and Hoffman1992; Heap Reference Heap2019) and was subsequently reported in California, Idaho, Kansas, North Dakota, and Oregon (Heap Reference Heap2019; Peterson Reference Peterson1999). Currently, >75% of the wheat fields in the NGP (Figure 1) are infested with ALS-resistant S. tragus populations (Jha et al. Reference Jha, Kumar and Lim2016; Saari et al. Reference Saari, Cotterman, Smith and Primiani1992; Stallings et al. Reference Stallings, Thill and Mallory-Smith1994). Resistance to glyphosate in S. tragus populations has recently been confirmed from fallow fields of Montana, Washington, and Oregon and is further suspected in populations in the Northern and Central Great Plains (Barroso et al. Reference Barroso, Gourlie, Lutcher, Mingyang and Mallory-Smith2018; Heap Reference Heap2019; Kumar et al. Reference Kumar, Spring, Jha, Lyon and Burk2017c).
Conyza Canadensis
A native species of North America, this winter annual dicot weed species commonly infests agronomic crops, orchards, pastures, roadsides, and industrial/waste areas (Gleason and Cronquist Reference Gleason and Cronquist1963; Miller and Miller Reference Miller and Miller1999). Conyza canadensis favors NT production systems of the U.S. Great Plains, as the seedlings primarily emerge on the soil surface (Nandula et al. Reference Nandula, Eubank, Poston, Koger and Reddy2006). Glyphosate is the most common nonselective herbicide used for its control in NT production systems across the region. Resistance to glyphosate in C. canadensis has been reported in Montana, Nebraska, Kansas, and Oklahoma (Crose et al. Reference Crose, Manuchehri and Baughman2019; Heap Reference Heap2019; Kumar et al. Reference Kumar, Jha and Jhala2017b). In addition to glyphosate resistance, C. canadensis populations with resistance to other herbicide SOAs, including photosystem I (PSI) inhibitors, ALS inhibitors, and PSII inhibitors, have been reported (Gadamski et al. Reference Gadamski, Ciarka, Gressel and Gawronski2000; Heap Reference Heap2019; Mueller et al. Reference Mueller, Massey, Hayes, Main and Stewart2003; Smisek et al. Reference Smisek, Doucet, Jones and Weaver1998).
Weed Management under Changing Climate
Climate change poses a serious concern to the dryland production systems of the U.S. Great Plains. Changing weather trends and extreme conditions in this region can have direct (changes in crop growth, development, and yield) and indirect (strong selective pressures exerted by abiotic stresses and biotic stresses such as pests) effects on agriculture (Hartfield et al. Reference Hartfield, Antle, Garrett, Izaurralde, Mader, Marshall, Nearing, Robertson and Ziska2014). The predicted changes in regional weather conditions (increasing CO2 levels, warmer temperatures, and varying frequency and distribution of annual precipitation) would have a profound effect on invasion, distribution, establishment, composition, demography, fitness, competitiveness, and management of weed species (Bradley et al. Reference Bradley, Blumenthal, Wilcove and Ziska2010; Gritti et al. Reference Gritti, Smith and Sykes2006; Varanasi et al. Reference Varanasi, Prasad and Jugulam2016; Waryszak et al. Reference Waryszak, Lenz, Leishman and Downey2018; Ziska and Dukes Reference Ziska and Dukes2011). The Great Plains region is projected to experience air temperatures increased by 2 to 4 C, doubling of atmospheric CO2 levels, warm winters, and pronounced droughts in some zones within the next half century (Hartfield et al. Reference Hartfield, Antle, Garrett, Izaurralde, Mader, Marshall, Nearing, Robertson and Ziska2014; Rosenburg Reference Rosenberg1982). These climatic changes might influence the growth cycles of predominant weed species in the region, further suggesting the need of innovative and improved weed management practices in future. For instance, rising levels of atmospheric CO2 will likely increase photosynthesis and growth of several weed species, further enhancing the ability of weeds to compete with crop plants (Ziska Reference Ziska2001, Reference Ziska2003). The process of converting CO2 to sugar in C3 plants is less efficient compared with C4 plant species, suggesting that C3 weed species will respond rapidly to increasing atmospheric CO2 concentrations (Ogren and Chollet Reference Ogren and Chollet1982; Ziska Reference Ziska2003). This increased efficiency of C3 weed species under increasing CO2 concentrations may result in aggressive growth, more competitive ability than other weeds or crops, and/or high seed production, indicating potential future weed shifts. Furthermore, changing weather patterns across the region, such as increasing CO2 levels, warm winters, and changing duration and frequency of annual rainfall events, are likely to increase both the risks posed by, and the sources of, invasive weed species (Bradley et al. Reference Bradley, Blumenthal, Early, Grosholz, Lawler, Miller, Sorte, D’Antonio, Diez, Dukes, Ibanez and Olden2012). For instance, the northward spread of invasive weeds such as A. palmeri, which is highly problematic in the Southern and Central Great Plains, could potentially be a concern in the NGP (Figure 1) in near future (Ward et al. Reference Ward, Webster and Steckel2013).
Climate change can also influence the effectiveness of herbicides and make weed management more challenging. Environmental factors such as CO2, light, temperature, and relative humidity can influence the performance of different herbicide SOAs (Varanasi et al. Reference Varanasi, Prasad and Jugulam2016). For instance, glyphosate efficacy was reduced on weeds grown at high CO2 levels (Koleva and Schneider Reference Koleva and Schneider2009). Similarly, reduced efficacy of mesotrione on A. palmeri, glyphosate on B. scoparia and C. album, and dicamba on B. scoparia has been reported at high temperatures (40/30 C) (DeGreeff et al. Reference DeGreeff, Varansai, Dille, Peterson and Jugulam2018; Godar et al. Reference Godar, Varanasi, Nakka, Prasad, Thompson and Mithila2015b; Ou et al. Reference Ou, Thompson, Stahlman, Blowdow and Jugulam2018). These reports demonstrate that more frequent applications and higher rates of herbicides may be needed to obtain adequate weed control in the future, resulting in increased economic and environmental costs associated with herbicide use. Recent literature also suggests that global warming can induce genetic and phenotypic changes within weed populations and will pose a greater risk of evolution of metabolism-based, non–target site herbicide resistance in weed species (Matzrafi et al. Reference Matzrafi, Seiwert, Reemtsma, Rubin and Peleg2016; Ziska et al. Reference Ziska, Blumenthal and Franks2019).
Cover Crops in No-Tillage Regions of the Semiarid Great Plains
Effective weed management is one of the most important agronomic practices for producing a profitable crop (Baucom and Holt Reference Baucom and Holt2009). As fewer and fewer new herbicide modes of action come to market and HR weeds continue to increase in NT dryland production systems of the Great Plains, alternative weed control strategies are needed. The implementation of infrequent tillage, including reduced tillage rather than NT and competitive crops grown in rotations, have contributed to the management of current weed problems; however, these practices alone may not be enough to achieve effective management of HR weeds in dryland production systems. Integration of CCs in dryland crop rotations is gaining popularity not only for weed control but also for other conservation practices. CCs contribute to building soil health and provide supplemental forage for grazing, nitrogen fixation, weed suppression, and habitat for beneficial insects and weed seed predators (Al-Khatib et al. Reference Al-Khatib, Libbye and Boydston1997; Holman et al. Reference Holman, Arnet, Dille, Maxwell, Obour, Roberts, Roozeboom and Schlegel2018; Hoorman Reference Hoorman2009; Kasper et al. Reference Kasper, Jaynes, Parkin and Moorman2007; Teasdale and Daughtry Reference Teasdale and Daughtry1993).
Dryland producers are adopting CCs to suppress weeds and to reduce costs of managing HR weed populations. In the U.S. Great Plains, CCs can be planted in fallow phases of the cropping cycle between cash crops. Theoretically, CCs in place of those fallow phases can help manage weeds in two different ways: (1) through the competitive effect (competition for light, water, nutrients, and space) of CCs for reducing weed growth that would otherwise establish in fallow fields (Osipitan et al. Reference Osipitan, Dille, Assefa and Knezevic2018) and (2) through the suppressive effect of CC residues on weeds after termination of CCs (Pullaro et al. Reference Pullaro, Marino, Jackson, Harrison and Keinath2006). The suppressive effect of CCs can occur in two ways: (1) through physical suppression, with CC residue (mulching) blocking sunlight and altering the soil microclimate (Lemessa and Wakjira Reference Lemessa and Wakjira2015); and (2) through chemical suppression, with CCs releasing allelochemical compounds into the environment (Al-Khatib et al. Reference Al-Khatib, Libbye and Boydston1997; Barnes and Putnam Reference Barnes and Putnam1986; Burgos et al. Reference Burgos, Talbert and Mattice1999; Creamer et al. Reference Creamer, Bennett, Stinner, Cardina and Regnier1996; Dhima et al. Reference Dhima, Vasilakoglou, Eleftherohorinos and Lithourgidis2006; Ercoli et al. Reference Ercoli, Masoni, Pampana and Arduini2007; Teasdale and Daughtry Reference Teasdale and Daughtry1993; White et al. Reference White, Worsham and Blum1989). However, the findings of many allelopathic studies have been confined to research laboratories, greenhouses, and growth chambers (Creamer et al. Reference Creamer, Bennett, Stinner, Cardina and Regnier1996; Sosnoskie et al. Reference Sosnoskie, Grey, Culpepper, Webster, Collins, Li and Shurley2012) and need to be further investigated under field conditions.
In addition to weed suppression, CCs can play a key role in integrated pest management in this semiarid region. CCs are known to influence soil microbial communities, which in turn can affect the viability and persistence of weed seedbanks (Liebman et al. Reference Liebman, Mohler and Staver2001). Kumar et al. (Reference Kumar, Brainard and Bellinder2008) reported that soil fungi contributed to the reduction of corn chamomile (Anthemis arvensis L.) and shepherd’s purse [Capsella bursa-pastoris (L.) Medik.] seedling emergence from soil where a buckwheat CC was grown and incorporated. Similarly, specific soil fungi are known to cause mortality of germinating A. theophrasti seeds (Davis and Renner Reference Davis and Renner2006). However, the long-term impacts of CCs on the soil microbiome are not fully understood and should be investigated under field conditions. There is insufficient research-based information on using CCs for weed suppression in the NT Great Plains, where soil moisture is limited compared with other U.S. regions. The following section reviews some opportunities for inclusion of CCs for weed management in semiarid cropping systems of the U.S. Great Plains.
Ecofriendly Approach and Key Elements of Integrated Pest Management
Growing CCs during fallow periods of NT dryland crop rotations across the Great Plains would serve as an ecofriendly approach for weed management. Effective weed management in NT fallow fields tends to rely on multiple herbicide applications (glyphosate alone or in mixtures) to provide season-long weed control each year. From 1974 to 2014, >1.6 billion kg (about 19% of estimated global use) of glyphosate was applied in U.S. agriculture (Benbrook Reference Benbrook2016). Due to the rapid evolution of GR weed populations, some growers have been replacing glyphosate with paraquat (a restricted-use herbicide due to its high level of acute human toxicity) in their burndown programs for fallow fields (VK and PWS, personal observation). The weed suppression achieved by CCs during fallow periods is likely to reduce the frequent and substantial use of these nonselective herbicides. The resulting decline in use of herbicides such as paraquat, dicamba, and atrazine could help reduce the chemical load on the environment (soil, water, air), further creating a more natural ecosystem.
CCs can also aid in increased weed seed predation and substantial reduction in weed seedbanks by harboring or providing suitable habitat for seed-eating insects, invertebrates, small rodents, and birds (Brust and House, Reference Brust and House1988; Cardina et al. Reference Cardina, Norquay, Stinner and McCartney1996; Maulsby Reference Maulsby2006; Menalled et al. Reference Menalled, Liebman, Renner, Singh, Batish and Kohli2006, Reference Menalled, Smith, Dauer and Fox2007; Westerman et al. Reference Westerman, Liebman, Menalled, Heggenstaller, Hartzler and Dixon2005; White et al. Reference White, Renner, Menalled and Landis2007). Seed predation has been well documented for several weed species such as common ragweed (Ambrosia artemisiifolia L.), A. palmeri, C. album, sicklepod [Senna obtusifolia (L.) Irwin & Barneby]), jimsonweed (Datura stramonium L.), A. theophrasti, fall panicum (Panicum dichotomiflorum Michx.), and giant foxtail (Setaria faberi Herrm.) (Menalled et al. Reference Menalled, Liebman, Renner, Singh, Batish and Kohli2006, Reference Menalled, Smith, Dauer and Fox2007; Westerman et al. Reference Westerman, Liebman, Menalled, Heggenstaller, Hartzler and Dixon2005; White et al. Reference White, Renner, Menalled and Landis2007). Ground beetles (Anisodactylus spp.), field crickets (Gryllus pennsylvanicus Burmeister), ants (Formicidae spp.), earthworms (Lumbricina spp.), slugs (Gastropoda spp.), field mice (Peromyscus maniculatus Wagner), and other small rodents hide below a residue layer on the soil surface for protection (Menalled et al. Reference Menalled, Liebman, Renner, Singh, Batish and Kohli2006; Westerman et al. Reference Westerman, Liebman, Menalled, Heggenstaller, Hartzler and Dixon2005; White et al. Reference White, Renner, Menalled and Landis2007). Therefore, we hypothesize that replacing NT fallow fields with CCs could increase seed predation of problematic weed species in the semiarid regions of the Great Plains and achieve more effective weed control systems.
Cover Crops for Managing Herbicide-Resistant Weeds in the Great Plains
Raising CCs for weed suppression is not a new concept; however, this method of weed control is only recently being tested in NT dryland production systems of the Great Plains. Exploring CCs for weed management in the region will open several new opportunities for weed research. Future CC studies in this dryland environment could investigate how CC management strategies (species selection, seeding rate, time of planting, termination date, livestock grazing, etc.) will affect life-history traits (seed dormancy, germination, emergence, growth, reproduction, seed viability, seed persistence, etc.) of problem weed species. Previous studies have demonstrated that B. scoparia populations in fallow fields from Central Great Plains (Figure 1) had earlier and quicker emergence patterns compared with populations from the NGP (Dille et al. Reference Dille, Stahlman, Du, Geier, Riffel, Currie, Wilson, Sbatella, Westra, Kniss, Moechnig and Cole2017; Kumar et al. Reference Kumar, Jha, Dille and Stahlman2017a). Rapid seed germination has also been observed in B. scoparia resistant to ALS inhibitors, whereas reduced and delayed seed germination has been reported in dicamba-resistant B. scoparia (Dyer et al Reference Dyer, Chee and Fay1993; Kumar and Jha Reference Kumar and Jha2016; Kumar et al. Reference Kumar, Jha, Lim and Stahlman2018b). Differential seed germination characteristics have also been found for GR B. scoparia in the region (Kumar and Jha Reference Kumar and Jha2017; Osipitan and Dille Reference Osipitan and Dille2017). Similarly, differential growth and reproductive characteristics of B. scoparia populations with various herbicide-resistance traits have previously been reported (Kumar and Jha Reference Kumar and Jha2015, Reference Kumar and Jha2016; LeClere et al. Reference LeClere, Wu, Westra and Sammons2018; Martin et al. Reference Martin, Benedict, Sauder, Wei, Da Costa, Hall and Beckie2017; Osipitan and Dille Reference Osipitan and Dille2017). It would be important to understand the fitness attributes of evolved herbicide-resistance traits in problematic weed species (B. scoparia, A. palmeri, C. canadensis, S. tragus, and B. tectorum) under diverse CC management strategies in NT dryland cropping systems. This information will help in exploring the ecological fate of HR alleles in weed populations. For instance, if fall-planted CCs such as winter triticale (×Triticosecale Wittm. ex A. Camus [Secale × Triticum]), cereal rye (Secale cereale L.), and hairy vetch (Vicia villosa Roth) provide competitive effects that might reduce seed germination, emergence, growth, and reproduction of HR B. scoparia and C. canadensis populations, then these CCs could delay or mitigate further spread of HR alleles in these populations. Similarly, spring-planted CCs such as oat (Avena sativa L.), triticale, and pea (Pisum sativum L.) alone or in mixtures can produce enough biomass and sufficient canopy cover to provide competitive effects on growth and reproduction of HR B. scoparia populations (Obour et al. Reference Obour, Holman, Dille and Kumar2019a). However, only those CCs that produced more than 1,000 kg ha−1 were effective at weed suppression (Petrosino et al. Reference Petrosino, Dille, Holman and Roozeboom2015), and greater CC biomass results in less available soil water for the subsequent cash crop (Holman and Obour Reference Holman and Obour2019). The leftover residue upon the termination of these fall- or spring-planted CCs can also provide suppressive effects (physical mulching) on the emergence of A. palmeri populations during early summer in the fallow period of a W–S–F rotation. The suppression of HR B. scoparia, C. canadensis, and A. palmeri populations achieved by these fall- or spring-planted CCs can also reduce the number of POST herbicide applications needed during the fallow phase, which will ultimately reduce selection pressure for further evolution of herbicide-resistance traits in these species.
The long-term impacts of replacing fallow fields with CCs on soil seedbank dynamics of problem weed species (especially HR populations), as well as shifts in weed communities, will be other important research questions to explore. It would be crucial to investigate and identify key competitive traits of growing CCs in the region, including planting dates, mixtures versus single species, seeding rates, and growth attributes (above- and belowground), that can reduce the growth of predominant weed species, thus reducing weed seed production to ultimately deplete the soil seedbanks. Future research could also emphasize understanding the suppressive traits of growing CCs, including residue persistence (especially in this moisture-limited environment) that can help conserve the soil moisture, alter the soil microenvironment (temperature, moisture, microbes), and ultimately, impact weed seedbank life. In a similar context, field and laboratory studies could be designed to identify allelopathic traits of various CCs (single or multiple species) grown in a semiarid environment, including the nature of allelochemicals, timing of release and impact on weed seed germination, weed seed life, and microbes in the soil (Kelton et al. Reference Kelton, Price and Mosjidis2012; Weston Reference Weston1996). Depending upon the goal of growing CCs (competitiveness vs. suppressiveness), multilocation studies should investigate different methods of termination (herbicides, mowing, haying, flailing, grazing, etc.) for different CC species, optimum timing, and their short- and long-term impacts on weed demographics and population dynamics under NT dryland production systems.
Economic and Sustainability Aspects of Using Cover Crops for Weed Management
On average, weed control costs >$11 billion a year for U.S. agriculture, and herbicides are one of the major expenses (Hartfield et al. Reference Hartfield, Antle, Garrett, Izaurralde, Mader, Marshall, Nearing, Robertson and Ziska2014). Weed suppression provided by CCs will potentially reduce herbicide use, which in turn will help reduce herbicide costs. Subsequently, any reductions in herbicide use during fallow periods will reduce the risk of herbicide-resistance evolution in weed populations across the region. In addition, weed seedlings remain smaller and more susceptible to herbicides for longer periods of time under a CC canopy, which could indirectly enhance the effectiveness of chemical control of those small-sized seedlings at the time of CC termination (Wallace et al. Reference Wallace, Curran and Mortensen2019). The replacement of NT fallow periods with CCs that produce high-quality forage can provide additional revenue to growers if properly managed for livestock grazing/haying. A recent study in western Kansas and eastern Colorado found that a spring-planted CC mixture of oat/triticale/pea produced high-quality forage for early summer grazing (Brummer et al. Reference Brummer, Johnson, Obour, Caswell, Moore, Holman, Schipanski and Harmoney2018). In a separate study, the same CC mixture (oat/triticale/pea) provided 90% to 99% suppression of B. scoparia biomass compared with nontreated fallow plots across two different sites in western Kansas (Obour et al. Reference Obour, Holman, Dille and Kumar2019a). However, it is important to note that the CC was not grazed or harvested in that same study, so the overall impact of CC grazing on B. scoparia population is unknown (Obour et al. Reference Obour, Holman, Dille and Kumar2019a). Previous research found that CCs in NT fallow fields could help reduce soil compaction, soil erosion, and nutrient leaching (Blanco-Canqui et al. Reference Blanco-Canqui, Holman, Schlegel, Tatarko and Shaver2013). Legume CCs can also increase nitrogen fixation, improve soil aggregates, protect crop seedlings, and conserve soil moisture by reducing evaporation rates during drought periods through a thick layer of residue after termination (Basche et al. Reference Basche, Kasparb, Archontoulisa, Jaynesb, Sauerb, Parkinb and Miguez2016; Holman et al. Reference Holman, Arnet, Dille, Maxwell, Obour, Roberts, Roozeboom and Schlegel2018; Magdof and Van Es Reference Magdof and Van Es2009; Unger and Vigil Reference Unger and Vigil1998). All of these factors can potentially contribute to enhancing the economic viability and sustainability of semiarid Great Plains cropping systems.
Agronomic Considerations for Integrating Cover Crops in No-Tillage Regions of the Semiarid Great Plains
The intended goal of including CCs in NT cropping systems will influence species selection, planting time, seeding rate, and time of termination. The extent of CC weed-suppressive ability will be greater with more biomass. In NT dryland systems, CC biomass production varies greatly because of variable precipitation amount, crop rotation, CC species, planting time, seeding rate, and soil type. In a recent meta-analysis, Osipitan et al. (Reference Osipitan, Dille, Assefa, Radicetti, Ayeni and Knezevic2019) showed that CC management decisions, including selection of CC species, planting season, planting date, seeding rate, termination date, delay in main crop planting after termination of CCs, and tillage system, all can influence the effective use of CCs for weed suppression. Here, we briefly review major agronomic practices and challenges in growing CCs for weed suppression in NT semiarid regions of the U.S. Great Plains.
Cover Crop Species Selection
Choosing individual CC species or mixtures with vigorous growth and biomass production is critical for effective weed suppression. Previous research documents that several diverse grass and broadleaf species could be planted as single or multiple species CCs in the semiarid Great Plains (Table 1). Cereals are generally considered more weed suppressive than broadleaf plant species (Norsworthy et al. Reference Norsworthy, McClelland, Griffith, Bangarwa and Still2011; Ruffo and Bollero Reference Ruffo and Bollero2003; Ruis et al. Reference Ruis, Blanco, Burr, Olson, Reiman and Rudnick2018). Cereal rye is a popular species chosen by those interested in incorporating a winter-hardy CC, as it can develop a fibrous root system, tolerates low-fertility soils, scavenges for available nitrogen, prevents soil erosion that commonly occurs when no residue or plant material is left on the soil surface, and can suppress weeds (Clark Reference Clark2007). However, cereal rye also can quickly become “weedy,” known as feral rye, and is a weed of dryland agriculture in the western and central United States that causes more than $26 million in annual wheat harvest losses (Pester et al Reference Pester, Westra, Anderson, Lyon, Miller, Stahlman, Northan and Wicks2000; Western Coordinating Committee-077 2019; Whitson et al. Reference Whitson, Burrill, Dewey, Cudney, Nelson, Lee and Parker2000). Barley (Hordeum vulgare L.), wheat, oat, and triticale are also competitive cereals and could possibly fit into some systems better than cereal rye. Although legumes are not considered as competitive as cereals and have a low C:N ratio (which contributes to a faster residue decomposition rate), their use may still offer benefits in the Great Plains (Creamer et al. Reference Creamer, Bennett and Stinner1997).
Table 1. List of grass and broadleaf cover crop species commonly adapted in the Great Plains.
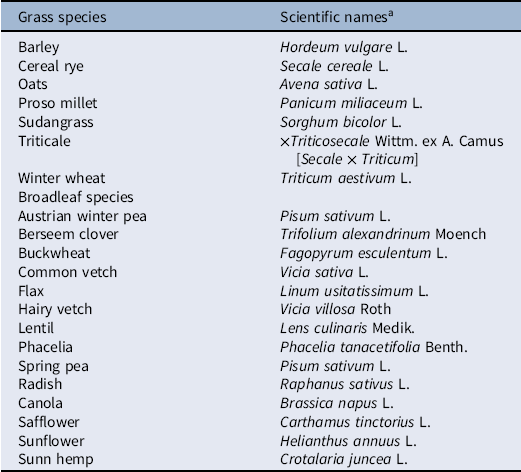
a Reviewed/researched by Calderon et al. Reference Calderon, Nielsen, Acosta-Martínez, Vigil and Lyon2016; Holman et al. Reference Holman, Arnet, Dille, Maxwell, Obour, Roberts, Roozeboom and Schlegel2018; Nielsen et al. Reference Nielsen, Lyon, Hergert, Higgins, Calderon and Vigil2015; Sanderson et al. Reference Sanderson, Johnson and Hendrickson2018.
In field studies conducted in western Kansas, the most productive grass CCs in dryland environments were sorghum or sudangrass (Sorghum bicolor L.) > cereal rye > triticale > wheat > oat > barley (Holman et al. Reference Holman, Arnet, Dille, Maxwell, Obour, Roberts, Roozeboom and Schlegel2018). The broadleaf legume CCs were not competitive and produced little biomass when grown alone or in CC mixes. In southwest Kansas, fall-planted triticale and a triticale–hairy vetch mixture reduced B. scoparia density by 78% and 94%, respectively, and biomass up to 98% when compared with chemical fallow (Petrosino et al. Reference Petrosino, Dille, Holman and Roozeboom2015). In that study, legume CCs such as Austrian winter pea, hairy vetch, spring lentil, and spring pea grown as single-species CCs produced little biomass (600 to 1,000 kg ha−1) and could not provide adequate suppression of B. scoparia growth. This was probably because the emergence timing of these spring-planted CCs coincided with B. scoparia emergence and the CCs could not compete well. This further suggests that weed emergence timing relative to CC establishment is a critical factor in selecting CC species, as previously discussed. Similarly, grass and brassica monocultures or mixtures were more effective at weed suppression than legumes (Baraibar et al. Reference Baraibar, Mortensen, Hunter, Barbercheck, Kaye, Finney, Curran, Bunchek and White2018). Therefore, to be effective, low biomass–producing legumes and other broadleaf CCs may need to be planted in mixtures with productive grass species to improve weed suppression. When weed suppression is the ultimate goal, then selecting a single CC species that produces more biomass can provide a cheaper weed-suppression alternative compared with multispecies mixes that may have a higher seed cost (Holman et al. Reference Holman, Arnet, Dille, Maxwell, Obour, Roberts, Roozeboom and Schlegel2018).
Planting multispecies CCs does not necessarily produce greater biomass or residue compared with a single species or simple CC mixes. Nielsen et al. (Reference Nielsen, Lyon, Hergert, Higgins, Calderon and Vigil2015) reported CC biomass or residue after CC termination with a 10-species mixture was not different compared with biomass produced by single-species CCs across two locations in eastern Colorado and western Nebraska. In North Dakota, weed-suppression benefits with monoculture CCs were not different from mixtures comprising millet [Pennisetum glaucum (L.) R. Br.]/triticale/clover (Trifolium spp.)/radish (Raphanus sativus L.) (Sanderson et al. Reference Sanderson, Johnson and Hendrickson2018). Similarly, Florence et al. (Reference Florence, Higley, Drijber, Francis and Lindquist2019) reported that multispecies CCs mixes did not increase aboveground biomass production or weed suppression when compared with productive single-species CCs across 11 sites in southeastern Nebraska. However, in a 2-yr study conducted in southcentral Montana, Khan and McVay (Reference Khan and McVay2019) concluded that multispecies CCs can provide more stable biomass yield than single-species CCs. In a meta-analysis, Osipitan et al. (Reference Osipitan, Dille, Assefa, Radicetti, Ayeni and Knezevic2019) reported that the decision to use a combination of multispecies mixtures, single species, and grasses or broadleaves was driven by ability of the selected CCs to produce greater biomass and persistent residue to suppress weeds. In recent CC studies in western Kansas, it was found that actively growing CC plants were more effective at suppressing weeds compared with CC residue amounts (Obour et al. Reference Obour, Holman, Dille and Kumar2019a). Hence, extending CC growth over greater portions of the fallow period could provide more weed-suppression benefits, but caution should be taken to avoid depleting plant-available water, which could be detrimental to the subsequent cash crop.
Cover Crop Seeding Rates
This is an important agronomic decision that affects plant stand, growth, water use, competitiveness, and productivity of CCs. Seeding rates of CCs are highly variable, and depend on species and whether planted as single or multiple species (Calderon et al. Reference Calderon, Nielsen, Acosta-Martínez, Vigil and Lyon2016; Holman et al. Reference Holman, Arnet, Dille, Maxwell, Obour, Roberts, Roozeboom and Schlegel2018). Single-species CCs should be planted at seeding rates similar to those used for forage production. For example, the seeding rate for oat and triticale CCs grown in the Great Plains ranged from 45 to 100 kg ha−1 (Calderon et al. Reference Calderon, Nielsen, Acosta-Martínez, Vigil and Lyon2016; Holman et al. Reference Holman, Arnet, Dille, Maxwell, Obour, Roberts, Roozeboom and Schlegel2018). Sudangrass is planted at 13.5 to 16.8 kg ha−1, which is the recommended rate range for forage production. Typical seeding rates for multispecies CCs ranged from 40 to 60 kg ha−1 (Calderon et al. Reference Calderon, Nielsen, Acosta-Martínez, Vigil and Lyon2016; Farney et al. Reference Farney, Sassenrath, Davis and Presley2018); significantly less compared with seeding rates for pea (115 to 134 kg ha−1) or triticale alone (72 to 100 kg ha−1) (Holman et al. Reference Holman, Arnet, Dille, Maxwell, Obour, Roberts, Roozeboom and Schlegel2018). Because of their smaller seed size, brassicas are generally planted at seeding rates of 5.6 to 7.5 kg ha−1 as a single-species CC or reduced to 1.1 to 2.2 kg ha−1 when included in CC mixes (Calderon et al. Reference Calderon, Nielsen, Acosta-Martínez, Vigil and Lyon2016; Farney et al. Reference Farney, Sassenrath, Davis and Presley2018).
There is limited research on CC seeding rate effect on weed suppressiveness in the semiarid Great Plains. However, studies in the mid-Atlantic region (Maryland, Pennsylvania, New York) showed increasing cereal rye seeding rate reduced the amount of weed biomass produced (Ryan et al. Reference Ryan, Curran, Grantham, Hunsberger, Mirsky, Mortensen, Nord and Wilson2011). Weed biomass in that study ranged from 328 g m−2 when rye was seeded at 90 kg seed ha−1 compared with 225 g m−2 when seeding rate was increased to 210 kg seed ha−1. Haramoto (Reference Haramoto2019) reported a greater plant density of cereal rye and winter wheat CCs when seeded at 112 kg ha−1 (184 plants m−2) compared with a lower seeding rate of 34 kg ha−1 (70 to 81 plants m−2); however, CC biomass and weed suppression did not differ between the two seeding rates. Previous research in the Central Great Plains indicated that oat grown for forage could be planted at 25% less than the recommended seeding rate of 72 kg ha−1 with no decrease in total biomass produced (Obour et al. Reference Obour, Holman and Schlegel2019b). This is due to a high tillering ability of cereal grains that compensates for reduced seeding rates. Therefore, a moderate reduction in CC seeding rate may not negatively influence total biomass production. Because of high seed costs associated with increasing seeding rates, CC growers should be moderate in deciding what seeding rates to use. The goal is to provide enough plant density and biomass to provide early-season weed suppression.
Cover Crop Seeding Time
In the semiarid Great Plains, CC growth and productivity varies from year to year because of the variability in precipitation amounts and seeding time. Average CC biomass in southwest Kansas ranged from 780 kg ha−1 when precipitation during the CC growing season was 46% of the 30-yr average to 2,690 kg ha−1 when precipitation was above average (Holman et al. Reference Holman, Arnet, Dille, Maxwell, Obour, Roberts, Roozeboom and Schlegel2018). The CC growing season can affect growth and subsequent impact on weed suppression. Spring- or fall-seeded CCs tend to perform better than a late summer–planted CC in this semiarid environment because of the available moisture at time of seeding. This period also coincides with the emergence of the most problematic weed species of this region: C. canadensis primarily emerges in the fall or early spring; B. scoparia emerges in early spring; whereas A. palmeri emerges in late spring to early summer. Therefore, depending upon soil moisture, fall- or spring-seeded CCs can best compete against and suppress problematic weed species in NT fields that otherwise would remain fallow and allow weed populations to flourish. For instance, fall-seeded cereal rye or winter triticale after wheat harvest can provide enough CC biomass to compete against C. canadensis and B. scoparia, which germinate in early spring. In contrast, spring-seeded CCs (such as oat/triticale/pea) in the fallow phase can help manage A. palmeri and B. scoparia in early summer through competition and late summer through physical suppression from CC residue following termination. Sanderson et al. (Reference Sanderson, Johnson and Hendrickson2018) found that spring-seeded CCs produced greater biomass and provided greater weed suppression compared with when CCs were seeded later in the growing season in North Dakota. This would be expected, because late-summer seedlings of CCs in semiarid regions of the Great Plains face considerable risks due to variable rainfall and a shortened growing season, which can result in reduction of CC biomass and weed-suppression benefits. Irrespective of the CC growing season, optimum planting dates are crucial for adequate plant establishment, increased biomass, and improved weed suppression.
Cover Crop Establishment Challenges
The ability of CCs to suppress weeds is contingent upon achieving good establishment. This can be challenging in semiarid environments where soil moisture at the time of seeding can be limited. Seeding into subsoil moisture will increase seedling emergence, plant vigor, and early establishment. However, CCs are generally seeded in mixtures, and the large number of plant species promoted as CCs exacerbate establishment issues because of differences in seed size within CC mixtures, preventing deep seed placement when smaller-seeded species are included in mixtures. To overcome this challenge, CC seeding should coincide with periods of adequate soil moisture to ensure conditions are favorable for germination and seedling emergence, particularly for small-seeded CC species that need to be seeded at shallow depths. Furthermore, limiting the number of species in CC mixtures to plants with similar seed sizes could ensure the entire CC mixture can be seeded at depths that provide good seed-to-soil contact to increase the chances of emergence and plant establishment.
In general, the CC seeding methods include direct planting into previous crop residue, aerial seeding, interseeding, or broadcasting into a standing crop (Curran et al. Reference Curran, Hoover, Mirsky, Roth, Ryan, Ackroyd, Wallace, Dempsey and Pelzer2018; Holman et al. Reference Holman, Arnet, Dille, Maxwell, Obour, Roberts, Roozeboom and Schlegel2018; Wilson et al. Reference Wilson, Allen and Baker2014). Among these methods, direct seeding with a drill provided better establishment with uniform stands compared with aerial seeding (Holman et al. Reference Holman, Arnet, Dille, Maxwell, Obour, Roberts, Roozeboom and Schlegel2018; Wilson et al. Reference Wilson, Allen and Baker2014). Establishment was reduced with broadcast or aerial seeding because of nonuniform seed distribution, low seed-to-soil contact, lack of moisture, and seed predation (Wilson et al. Reference Wilson, Allen and Baker2014). Establishment of small-seeded CC species could be improved with some tillage to prepare a seedbed; however, such tillage could increase potential for wind erosion and encourage a flush of weeds. Research is needed to determine whether occasional tillage every 3 to 5 yr could be used to establish CCs as part of the crop rotation cycle. This approach can improve CC establishment and control troublesome weeds with minimal impacts on soil properties (Blanco-Canqui and Wortmann Reference Blanco-Canqui and Wortmann2020). One-time strategic tillage in long-term NT fields in western Kansas controlled glyphosate-tolerant red three-awn grass (Aristida purpurea Nutt.) and C. verticillata with no effect on soil water availability, winter wheat yields, and soil properties (Obour et al. Reference Obour, Holman and Schlegel2019c). However, more research is needed to determine the combined effects of strategic tillage and CCs in managing HR weeds and subsequent impacts on soil health and crop yields in this region.
Another major challenge that affects CC establishment is residual herbicide from the previous crop. In dryland W–S–F rotations, producers are currently relying on PRE herbicides to manage HR weeds. Many of these herbicide chemistries are persistent, with residual phytotoxic concentrations in the soil that may affect establishment when CCs are planted in the fallow phase of the rotation. For example, S-metolachlor applied at 1.68 kg ha−1 severely injured and reduced annual ryegrass (Lolium multiflorum Lam.) CC stands regardless of the planting time following herbicide application (Tharp and Kells Reference Tharp and Kells2000). Similarly, biomass of Italian ryegrass [Lolium perenne L. ssp. multiflorum (Lam.) Husnot] and winter oat were significantly reduced (~67%) by pyroxasulfone herbicide carryover from the previous corn crop (Cornelius and Bradley Reference Cornelius and Bradley2017). In general, herbicides with greater persistence will have a long-term residual activity that will affect CC inclusion in the rotation. Herbicide labels typically provide information on restrictions regarding safety of rotational crops, but these are based on cash crops with no or limited information for CC usage. More research information is needed regarding herbicide carryover injury on CCs to prevent risk of establishment failure. In addition, data on the safety of the herbicide with respect to CCs, particularly CCs used as livestock feed, are essential.
Soil Moisture Trade-offs and Impacts on Cash Crop Yields
The fallow phase of the production system is critical for conservation of soil water that stabilizes wheat yields and prevents crop failure in drier years in NT regions of the semiarid Great Plains (Haas et al. Reference Haas, Willis and Bond1974; Nielsen and Vigil Reference Nielsen and Vigil2010). In this water-limited environment, crop yields are directly related to available soil moisture at time of cash crop seeding (Schlegel et al. Reference Schlegel, Lamm, Assefa and Stone2018). Despite the weed-suppression benefits of CCs, previous research demonstrated that replacing fallow with CCs often resulted in decreased soil water content at time of cash crop seeding (Holman et al. Reference Holman, Arnet, Dille, Maxwell, Obour, Roberts, Roozeboom and Schlegel2018; Nielsen and Vigil Reference Nielsen and Vigil2018; Schlegel and Havlin Reference Schlegel and Havlin1997; Unger and Vigil Reference Unger and Vigil1998; Zentner et al. Reference Zentner, Campbell, Biederbeck and Selles1996). Schlegel and Havlin (Reference Schlegel and Havlin1997) reported that a hairy vetch CC reduced soil water content by 178 mm compared with fallow, and the subsequent wheat crop yield was reduced by 42% to 83% across years. In that same study, every millimeter of soil water depleted by hairy vetch resulted in reduction of wheat yields by 15 kg ha−1. Holman et al. (Reference Holman, Arnet, Dille, Maxwell, Obour, Roberts, Roozeboom and Schlegel2018) reported wheat yield reduction of 13 kg ha−1 for every millimeter of soil water depleted by CCs, whether grown as a mixture or as a single species. The impact of CCs on soil water availability will depend on amounts of precipitation and infiltration after termination to replenish water use by CCs. Therefore, growing window and time of CC termination are critical for CC water use and soil water replenishment relative to seeding the next cash crop. Wheat yields following lentil as a CC terminated at full bloom in northeastern Montana was 2,040 kg ha−1 compared with 2,820 kg ha−1 in fallow in a W–F system (Pikul et al. Reference Pikul, Aase and Cochran1997). This corresponds to a more than 25% decrease in wheat yield when lentil replaced fallow in the cropping system. In eastern Colorado, Nielsen and Vigil (Reference Nielsen and Vigil2005) reported that the soil water content in fallow was 320 mm compared with 265 mm when CCs were terminated in early June, 245 mm when terminated in mid-June, or 216 mm when terminated in mid-July. Barker et al. (Reference Barker, Heeren, Koehler-Cole, Shapiro, Blanco-Canqui, Elmore, Proctor, Irmak, Francis, Shaver and Mohammed2018) reported little to no effect of CCs on soil water availability in a 3-yr study across Nebraska.
In general, water use by CCs will vary and may depend on species, biomass production, and precipitation after termination in the growing season. In a 2-yr study conducted at a dryland site in Akron, CO, Nielsen et al. (Reference Nielsen, Lyon, Hergert, Higgins and Holman2016) reported CC water use ranging from 127 mm for pea to 221 mm for canola and 136 mm in the first year (seasonal precipitation of 85 mm) to 202 mm in the second year (seasonal precipitation of 178 mm). The aforementioned studies demonstrate that growing CCs in the semiarid Great Plains uses soil water resources and could affect subsequent cash crop yields regardless of CC species selected, growing window (fall or spring planted), or selection of multispecies CCs. It is therefore imperative that CCs grown in semiarid regions provide some revenue stream to offset seed costs and cash crop yield depressions when CCs are seeded ahead of the main crop (Holman et al. Reference Holman, Arnet, Dille, Maxwell, Obour, Roberts, Roozeboom and Schlegel2018).
Cover Crops Grazing/Haying
An additional revenue stream for farmers is to use CCs for grazing or haying. This approach could provide an opportunity for dryland producers to suppress weeds, improve soil health, and produce harvestable forage for livestock. Recent research in western Kansas demonstrated that most of the species planted as CCs have excellent forage attributes in terms of dry matter production and forage nutritive value (Brummer et al. Reference Brummer, Johnson, Obour, Caswell, Moore, Holman, Schipanski and Harmoney2018; Obour et al. Reference Obour, Holman and Schlegel2019b). Regrowth from hayed or grazed CCs can provide more residue cover compared with fallow. In southwestern Kansas, winter triticale or a winter triticale–legume CC harvested for forage was more profitable than chemical fallow (Holman et al. Reference Holman, Arnet, Dille, Maxwell, Obour, Roberts, Roozeboom and Schlegel2018). Notwithstanding the significant depression in wheat yields following a spring CC, overall system profitability was greater when an oat–pea forage was added to dryland cropping systems compared with fallow (Lyon et al. Reference Lyon, Blumenthal, Burgener and Harveson2004). Therefore, using CCs for forage will provide opportunity for dual-purpose CCs in dryland systems to also provide residue cover to manage HR weeds, reduce erosion, and improve soil health. However, research data on best management practices for grazing CCs in NT dryland systems are limited. More importantly, farmers are asking questions about:
1. CC mixtures to achieve best outcomes;
2. seeding windows for grazing CCs (spring, summer, or fall);
3. amount of CCs biomass that should be grazed relative to residue cover;
4. grazing impact on soil compaction;
5. grazing impacts on weed suppression (weeds like B. scoparia may be palatable); and
6. soil health implications when CC biomass is removed as forage through grazing.
Currently, there is a paucity of research to address these concerns. Ongoing research demonstrates that grazing CCs could increase soil compaction near the soil surface under wet conditions. Wheat grain yields following CCs that were grazed or hayed did not differ from yields when CCs were left standing (Obour et al. Reference Obour, Holman and Schlegel2019b). The grazing potential of CCs in semiarid dryland systems of the Great Plains needs further investigation.
Summary and Future Directions
The conundrum of incorporating CCs in crop rotations in the semiarid U.S. Great Plains is how to take advantage of weed suppression and gain soil health benefits while reducing the negative impacts on available soil water and subsequent crop yields. The potential detrimental effects of CCs on subsequent crop yields have slowed their adoption, because farmers’ decisions are based on the overall economic situations of their farms. For successful adoption of CCs in NT dryland systems, CCs must be managed to increase the amount of biomass at termination for weed suppression and provide an economic value. Replacing NT fallow periods with CCs will potentially reduce the number of herbicide applications. This will hold true when there is adequate moisture for CC establishment to produce adequate biomass.
This review highlights that integration of CCs in NT regions of the semiarid Great Plains can potentially contribute to the development of cost-effective and ecologically based IWM strategies, especially for managing HR weed populations. Multistate studies are needed to investigate the systematic manipulation of specific components of CCs for successful integration into current cropping systems in the region. Research gaps exist in understanding the impacts of various CC management strategies (CC species selection, time of seeding and termination, seeding rates, method of termination, etc.) on population dynamics (seed mortality and longevity; seed dormancy; germination ecology; emergence pattern; and other life-history traits, e.g., fitness) of HR weed populations and subsequent crop yields in the region. Researchers should also investigate other ecosystem services provided by CCs in NT semiarid Great Plains, including allelopathy, attractants for beneficial insects/pollinators, and habitat for weed seed predators, with the ultimate goal of achieving more resilient weed management systems that are more likely to delay evolution of HR weed populations. Long-term field research should also validate the important components of environmental sustainability and economic viability of integrating CCs for weed management in the region.
Acknowledgments
This work was jointly supported by the USDA National Institute of Food and Agriculture (Hatch Projects 1019671 and 1019594) and the USDA North Central Sustainable Agricultural Research and Education Program (grant no. LNC 18-411). No conflicts of interest have been declared. This publication is contribution no. 20-104-J from the Kansas Agricultural Experiment Station, Manhattan, KS.