Introduction
Weed exposure to diverse environmental stresses is exacerbated in agroecosystems, where weeds compete with crops for nutrients, water, light, and space (Korres Reference Korres2005; Ramegowdaa and Senthil-Kumar Reference Ramegowdaa and Senthil-Kumar2015). At lower environmental stress, acquisition of these resources by the weeds intensifies, resulting in vigorous growth, increased seed production, and ease of weed population establishment, all of which have direct consequences on field operations, crop husbandry, and final crop yield (Korres Reference Korres2005). This is particularly true for Palmer amaranth (Amaranthus palmeri S. Watson), one of the most troublesome weeds in southern U.S. crops (Riar et al. Reference Riar, Norsworthy, Steckel, Stephenson, Eubank and Scott2013; Webster and Nichols Reference Webster and Nichols2012) and ranked as the most agronomically problematic species in the United States, the presence of which causes significant yield losses (Bensch et al. Reference Bensch, Horak and Peterson2003; Massinga et al. Reference Massinga, Currie, Horak and Boyer2001) when not adequately controlled.
The success of future weed management strategies targeting this weed will rely on an improved understanding of its biological, phenological, and reproductive characteristics and population dynamics (Puricelli et al. Reference Puricelli, Orioli and Sabbatini2002; Sellers et al. Reference Sellers, Smeda, Johnson and Ellersieck2003). Knowledge of weed biology can be used for the development of fundamental principles necessary for the improvement of current weed control practices (Wyse Reference Wyse1992). An understanding of a weed’s emergence pattern or the weed establishment time within a particular cropping system will enable timely implementation of control strategies (Korres et al. Reference Korres, Norsworthy, Brye, Skinner and Mauromoustakos2017a, Reference Korres, Norsworthy, FitzSimons, Trent and Oosterhuisb). Amaranthus palmeri, for example, succeeds because its emergence pattern coincides with the production systems of major row crops (Bell et al. Reference Bell, Norsworthy and Scott2015; Jha and Norsworthy Reference Jha and Norsworthy2009); thus, awareness of the timing of and critical period for implementation of A. palmeri control strategies is vital.
Among the abiotic factors regulating a habitat’s suitability for a species, shade is considered one of the most pertinent. Characterizing the response of A. palmeri to crop canopy shade is important for improved understanding of crop–weed interference and weed population dynamics (Jha et al. Reference Jha, Norsworthy, Bridges and Riley2008; Korres and Norsworthy Reference Korres and Norsworthy2017; Korres et al. Reference Korres, Norsworthy, FitzSimons, Trent and Oosterhuis2017b). Light regime affects A. palmeri biomass production, leaf number, partitioning of dry weight to stem tissue, stem elongation, specific leaf area, and photosynthesis (Korres et al. Reference Korres, Norsworthy, FitzSimons, Trent and Oosterhuis2017b). It has been reported that shading by the soybean [Glycine max (L.) Merr.] canopy is directly related to row spacing, an important factor to determine not only soybean yield (Bradley Reference Bradley2006), but also A. palmeri performance and cultural weed management methods (Jha et al. Reference Jha, Norsworthy, Bridges and Riley2008; Yelverton and Coble Reference Yelverton and Coble1991). The cosmopolitan nature of this species (EPPO 2018), the threat imposed by this species (Korres et al. Reference Korres, Norsworthy, Brye, Skinner and Mauromoustakos2017a), and its ability to develop herbicide resistance to various herbicide mechanisms of action in many countries (Heap Reference Heap2018) justify the present work. Development of efficient weed control strategies and cropping systems that enhance soybean competitiveness against A. palmeri need to be exploited more aggressively and under various crop–weed interference scenarios. In addition, results of this work can be used in population dynamic models that depend on data involving weed biological characteristics such as height, biomass production, and fecundity.
Research was conducted, therefore, to investigate whether various A. palmeri establishment times and distances from soybean affect the performance of the weed by evaluating: (1) A. palmeri biological characteristics (i.e., height, dry matter production, seed production); (2) A. palmeri phenology (flowering); and (3) the effects these variations in A. palmeri plantings have on soybean yield.
Materials and Methods
Experimental Setup
Field experiments were conducted in a randomized complete block design at the University of Arkansas Agriculture Research and Extension Center, Fayetteville, AR (36.094464, −94.172074), during the summers of 2014 and 2015 to investigate the effects of A. palmeri establishment time and distance from the soybean wide-row (i.e., ∼96-cm row spacing; henceforth distance from the crop) on crop yield and on the biological characteristics and phenology of the weed. Establishment time, in weeks after crop emergence (WAE), was composed of six treatment levels (0, 1, 2, 4, 6, and 8 WAE), whereas the distance from the crop consisted of three treatment levels (0, 24, and 48 cm) (Figure 1). The experiments were conducted in a silt-loam soil (fine, mixed, active, thermic Typic Albaquults) containing 34% sand, 53% silt, 13% clay, and 1.5% organic matter with a pH between 6.5 and 6.9. A field cultivator (Kongskilde Industries, Hudson, IL) was used after disking for the preparation of the seedbed before crop planting. A glufosinate-resistant soybean cultivar (i.e., Pioneer® 95L01, maturity group 4.6, DuPont, Leland, MS) was planted in 96-cm-wide, 4-row plots at a rate of 320,000 seeds ha−1 on June 24, 2014, and June 25, 2015. Plots were 9- and 6-m long for the 2014 and 2015 experiments, respectively, and approximately 3.7-m wide.
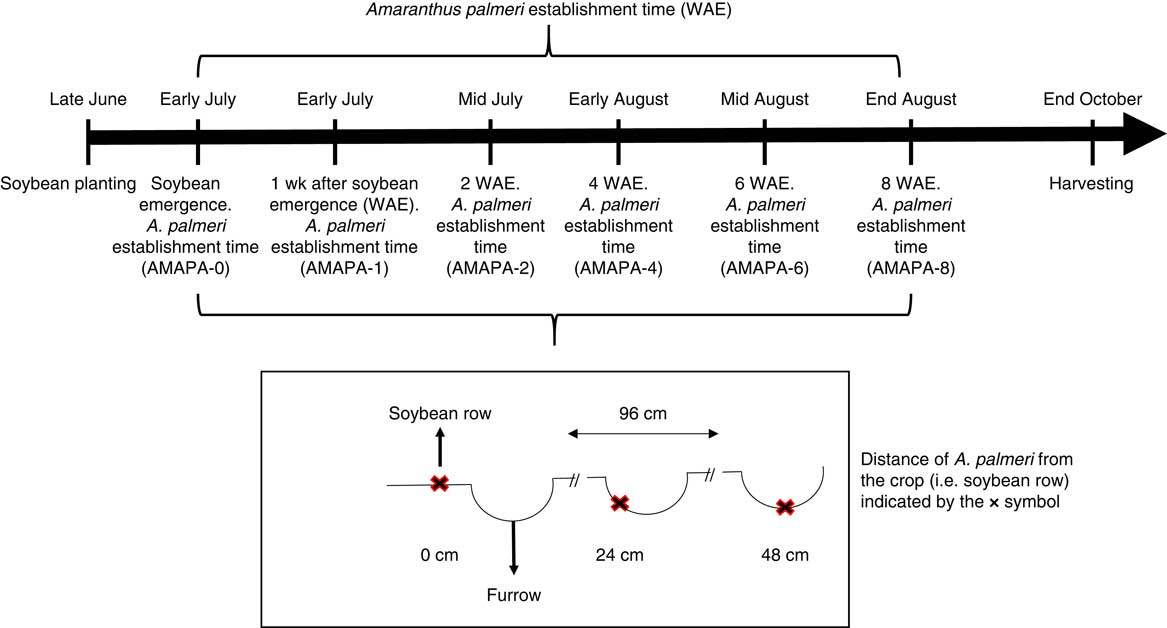
Figure 1 Schematic representation of the experimental setup depicting the distance of Amaranthus palmeri (AMAPA) from the crop (i.e., 0, 24, and 48 cm from the soybean row) and the sequence of A. palmeri establishment time (i.e., 0, 1, 2, 4, 6, and 8 wk after soybean emergence [WAE] or AMAPA-0, AMAPA-1, AMAPA-2, AMAPA-4, AMAPA-6, and AMAPA-8, respectively). Each treatment combination (i.e., establishment time×distance from the crop) was applied only to one randomly selected experimental plot per replication.
Experimental plots were routinely hand weeded during the entire experimental period to remove unwanted weeds and were irrigated using a Valley remote irrigation system (Valmont Industries, Valley, NE) that delivered 12.5 mm of water per irrigation run when significant rainfall did not occur for a 7-d period.
Plant Material and Experimental Treatments
Amaranthus palmeri seeds were collected in the 2013 to 2014 growing season at the Agriculture Research and Extension Center, Fayetteville, AR, and were stored in sealed vials at 5 C. At the initiation of the experiment, seeds of A. palmeri were sown in plastic trays (52.5 by 25.5 by 5.5 cm) containing a commercial potting mix (Sunshine® LC1 potting mix, Sun Gro Horticulture, Agawam, MA). Amaranthus palmeri germination was planned to coincide with each establishment time treatment as it was defined by soybean emergence; for example, AMAPA-0 coincided with soybean emergence in the field or at 0 WAE weed establishment time (approximately 7 to 10 d after planting for both crop and weed). Trays for A. palmeri seed germination were placed in a greenhouse with a 35/23 C day/night temperature and an approximately 14-h photoperiod with light intensity between 800 to 1200 μmol m−2 s−1 as measured at midday with an AccuPAR/LAI Sunfleck Ceptometer Model LP-80 (Meter Group, Pullman, WA). The same process was repeated for each weed establishment time treatment.
Approximately 500 vigorous A. palmeri seedlings, for each establishment time, were transplanted into Jiffy-7® peat pellets (HummertTM International, Earth City, MO) and placed in 72-plug plastic trays for a few days before being transplanted in the field. These A. palmeri seedlings were transplanted into the corresponding plots at three distances from the top of the crop row, that is, 0, 24, or 48 cm at 0, 1, 2, 4, 6, and 8 WAE establishment time (Figure 1). At each time point, 12 randomly selected plots (i.e., 3 distances from the crop by 4 replications) were used for transplanting until all experimental treatments were applied. The two center rows of each randomly designated plot (the border rows were maintained weed-free) were selected, and A. palmeri plants at the 2-leaf stage and approximately 3-cm tall were transplanted at 0-, 24-, or 48-cm distance from the crop (Figure 1) every 1 m along the crop row, targeting a density of approximately 1 A. palmeri plant m−2. Klingaman and Oliver (Reference Klingaman and Oliver1994) reported that A. palmeri densities greater than 1 A. palmeri plant m−1 of soybean row initiates intraspecific interference between adjacent A. palmeri plants.
This seedling production and transplanting method allowed the establishment of plants of uniform size and equal distribution along the row for each experimental treatment. The young A. palmeri plants were watered every 2 d for a 2-wk period (Burke et al. Reference Burke, Schroeder, Thomas and Wilcut2007) to minimize possible stress during the acclimatization period. The methodology used, although relatively common (Bond and Oliver Reference Bond and Oliver2006; Moore et al. Reference Moore, Murray and Westerman2004), might have affected the actual growth rate of A. palmeri seedlings, particularly the early established seedlings as opposed to seedlings that emerge from the natural seedbank. Nevertheless, closed crop canopies might have impaired the germination, emergence, and survival of late-emerging A. palmeri plants (Bradley Reference Bradley2006; Hartzler and Battles Reference Hartzler and Battles2004; Steckel and Sprague Reference Steckel and Sprague2004) if direct seeding was used instead of transplanting. Ultimately, this method allowed the simulation of interference by an established A. palmeri population within the soybean crop at 0, 1, 2, 4, 6, and 8 WAE.
Sampling and Data Collection
Soybean crop establishment was evaluated at first trifoliate (V1) growth stage, and it was recorded as >95% for both years. In addition, for 20 randomly selected plants from the middle 2 rows plot−1, soybean height (from ground level to the apical meristem) was recorded six and eight times (i.e., sampling occasions) starting at 2.4 and 1.8 WAE for 2014 and 2015, respectively. In parallel to soybean height recordings, height for each A. palmeri plant (18 and 14 plants plot−1 for 2014 and 2015, respectively, to adjust for plot size changes between years) from ground level to the apical meristem was also recorded, along with the number of A. palmeri flowering plants (i.e., flowering initiation and cumulative number of flowering plants thereafter).
Soybean yield from the two middle plot rows was harvested with a small-plot combine at crop maturity, and yields were adjusted to 13% moisture content. Before soybean harvesting, A. palmeri plants, both male and female, were harvested by cutting the stems at the soil level. The height of each A. palmeri plant in the plot was recorded before harvesting, and plants were placed in paper bags and dried at 60 C for 4 d. Dry weight for each A. palmeri plant was recorded, and female plants were threshed to evaluate seed production. Seeds from each female plant were separated from plant tissue using a series of sieves, with the bracts and other plant debris removed by gently blowing air over the seeds as they were transferred between sieves. A minimum of five 100-seed subsamples from each female plant was weighed, and the total number of seeds produced per plant was extrapolated based on Equation 1 (Sellers et al. Reference Sellers, Smeda, Johnson and Ellersieck2003).

where W represents the total weight of all seeds for a particular plant, S the average weight of the five 100-seed subsamples, and T the total number of seeds produced for a plant.
Ground Cover, Leaf Area Index, and Light-Interception Measurements
A digital camera (Sony DSC-W570, 16.1 megapixels, 25-mm wide-angle lens, 2.7 LCD screen Sony, New York, NY) was used to obtain photographs of crop canopy from two predetermined marked positions in each experimental plot as described in Bell et al. (Reference Bell, Norsworthy and Scott2015). Photographs from each plot were analyzed individually using SigmaScan Pro v. 5.0 (Systat Software, San Jose, CA) and Turf Analyzer (https://www.turfanalyzer.com) for the determination of canopy closure, described as “ground cover” (Purcell Reference Purcell2000; Richardson et al. Reference Richardson, Karcher and Purcell2001), immediately before each A. palmeri transplanting treatment.
The efficiency of light interception by the crop canopy was estimated by measurements of light transmittance through the crop canopy using an AccuPAR/LAI Sunfleck Ceptometer Model LP-80 (Meter Group). Light-transmittance recordings were taken above and below the crop canopy from the same two predetermined points used for canopy closure photographs, under uniform sky conditions between 1100 and 1400 hours. Two measurements perpendicular to the crop row were taken from each plot; these were averaged, and the extinction coefficient, based on light transmittance, was estimated (Equation 2) (Wolf et al. Reference Wolf, Carson and Brown1972).

where k is the extinction coefficient; I 0 is the light intensity above the crop canopy; I is the light intensity below crop canopy; and L is the LAI (leaf area index) of leaves causing the light attenuation. Soybean LAI was estimated based on leaf area measurements (adjusted for 1 m2) on 5 soybean plants from each of the same two predetermined points used for canopy closure photographs using a Li-Cor 3000 portable area meter (Li-Cor, Lincoln, NE).
Statistical Analysis
ANOVA for soybean height and yield along with A. palmeri biological characteristics (i.e., height, aboveground biomass, seed production, and flowering) was performed using JMP Pro v. 13.1.0 software (SAS Institute, Cary, NC). ANOVA for the height and flowering of A. palmeri throughout the growing season was performed separately for each sampling date. No differences in crop stand were found between treatments by year (unpublished data). Nevertheless, due to interactions between A. palmeri establishment time and year for soybean yield, the statistical analysis was done separately by year. Amaranthus palmeri biological and phenological characteristics were analyzed using weed establishment time and distance from the crop as fixed effects.
Rectangular hyperbolas were fit for soybean yield in relation to the initial A. palmeri establishment time (i.e., 0 WAE) relative to soybean for 2014 and 2015 using SigmaPlot v. 13.0 (Systat Software). Values from SigmaScan Pro were exported to SigmaPlot v. 13.0 to examine the correlation between ground cover and extinction coefficient.
Results and Discussion
Amaranthus palmeri biological characteristics
Amaranthus palmeri established at soybean emergence was taller (P<0.001) than the crop at 7.3 and 10.7 WAE for 2014 and 2015, respectively (Figure 2). The second A. palmeri cohort, which established 1 wk later than the crop (AMAPA-1 WAE), overgrew the crop at 17 WAE in 2014 (Figure 2). In 2015, however, soybean was significantly taller (P<0.001) than A. palmeri established at 2 WAE onward.
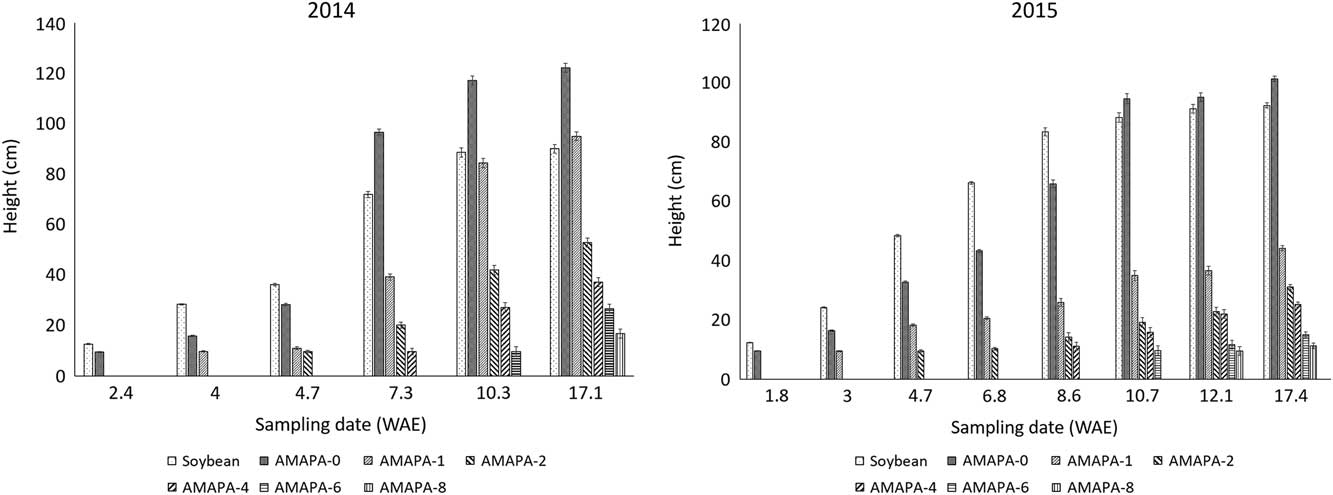
Figure 2 Soybean and Amaranthus palmeri (AMAPA) height (averaged across distance from the crop) at 0, 1, 2, 4, 6, and 8 wk after soybean emergence (WAE) (i.e., AMAPA-0, AMAPA-1, AMAPA-2, AMAPA-4, AMAPA-6, and AMAPA-8, respectively). Vertical bars represent±standard error of the mean from the analysis for comparisons within each sampling date (i.e., n=12 for 0 WAE, 24 for 1 WAE, etc.).
A significant interaction between weed establishment time and effect of distance from soybean crop on A. palmeri performance was observed at harvesting for both 2014 and 2015. More specifically, shorter establishment timings in combination with the farthest distance from the crop resulted in fewer effects on A. palmeri biological characteristics, including height (Figure 3), biomass (i.e., aboveground dry weight; Figure 4), and seed production (Figure 5) compared with late establishment times (i.e., 2, 4, 6, and 8 WAE) and shorter distances to the crop (i.e., 0 and 23 cm).
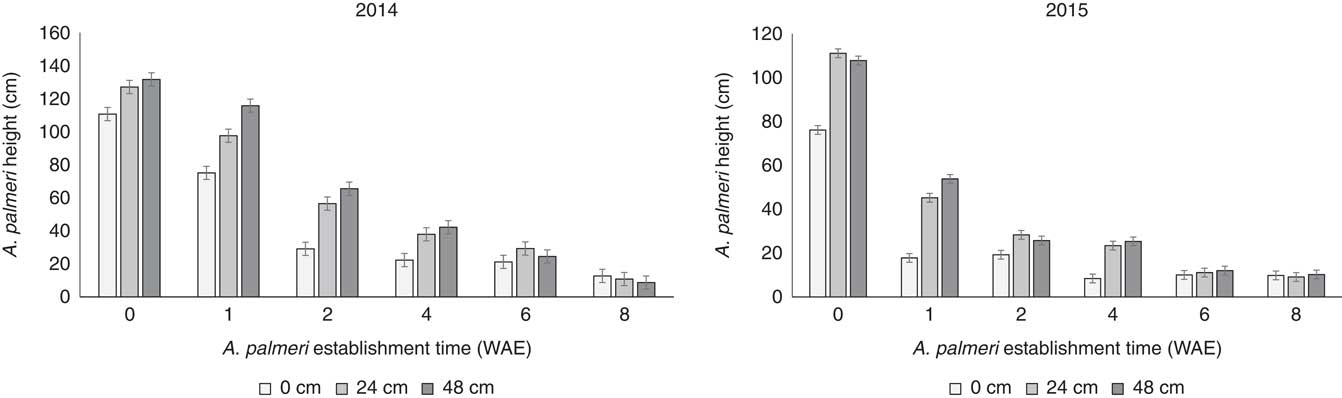
Figure 3 Effects of the interaction of weed establishment time and distance from the crop on Amaranthus palmeri plant height at harvest. Vertical bars represent±standard error of the mean (SE2014=4.68; SE2015=3.14) from the analysis for comparisons between weed establishment times with sample size n=72. WAE, weeks after soybean emergence.
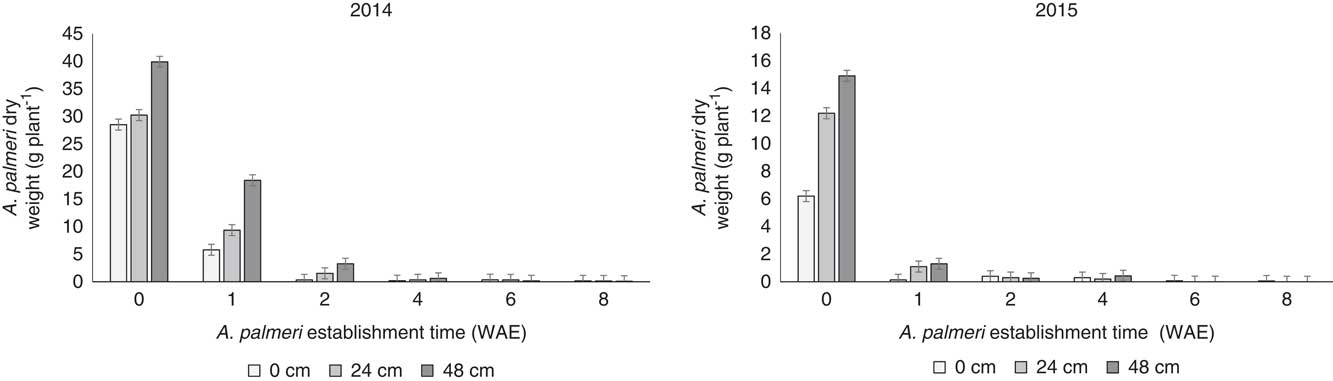
Figure 4 Effects of the interaction of weed establishment time and distance from the crop row on Amaranthus palmeri dry weight before soybean harvest. Vertical bars represent±standard error of the mean (SE2014=1.27; SE2015=0.74) from the analysis for comparisons between weed establishment times with sample size n=72. WAE, weeks after soybean emergence.
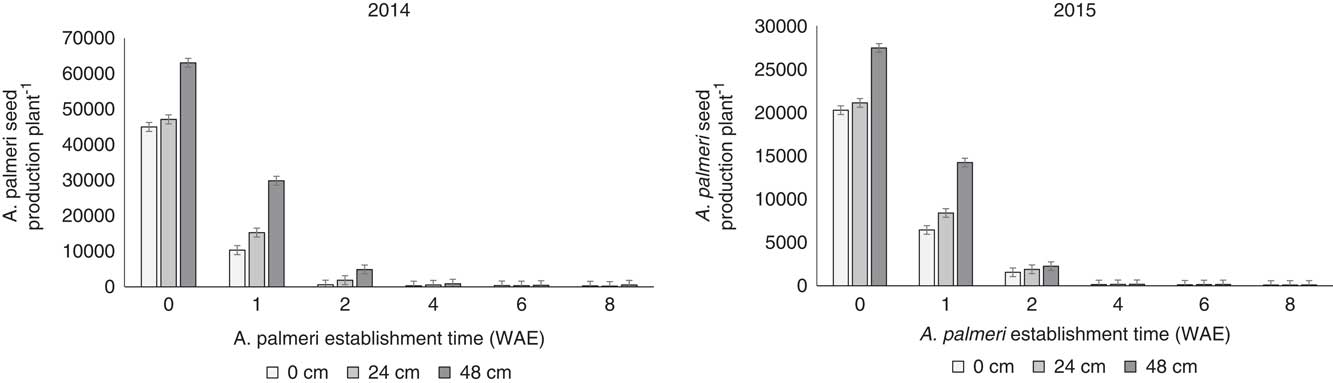
Figure 5 Effects of the interaction of weed establishment time and distance from the crop row on Amaranthus palmeri seed production before soybean harvest. Vertical bars represent±standard error of the mean (SE2014=2,530.27; SE2015=1,008.30) from the analysis for comparisons between weed establishment times with sample size n=72. WAE, weeks after soybean emergence.
Amaranthus palmeri plants at 48 and 24 cm from the crop at 0, 1, 2, and 4 WAE were significantly taller (P<0.001 for 2014 and 2015) than A. palmeri plants growing adjacent to the soybean crop (Figure 3). These results were similar for both years. Similarly, the interaction of weed establishment time and distance from the crop exerted significant effects (P<0.001 for 2014 and 2015) on A. palmeri plant biomass (Figure 4) and seed production (P<0.001 and P=0.0021 for 2014 and 2015, respectively) (Figure 5). Amaranthus palmeri biomass production per plant was greater for A. palmeri plants established with the crop or at 1 WAE at a 48-cm distance from the crop compared with the biomass produced by those established at 2 WAE or later regardless the distance from the crop (Figure 4). In 2015, biomass production for A. palmeri that was established at 1 WAE was not different between 24 and 48 cm (Figure 4).
The greater the biomass produced, the greater the seed production, especially for A. palmeri plants established at crop emergence and at a 48-cm distance from the crop row (Figure 5). Amaranthus palmeri plants established at 1 WAE at a 48-cm distance produced 50% and 70% fewer seeds for 2014 and 2015, respectively, compared with A. palmeri plants in the soybean row at 0 WAE. These trends were observed for the A. palmeri cohorts established at 0 and 1 WAE. Amaranthus palmeri seed production at the other establishment times was significantly reduced irrespective of distance from the crop (Figure 5).
Amaranthus species are among the most troublesome weeds in many crop production systems (Korres and Norsworthy Reference Korres and Norsworthy2017; Webster and Grey Reference Webster and Grey2015). Effective control of these species, given their highly competitive ability and tendency to evolve resistance to various herbicides (Korres et al. Reference Korres, Norsworthy, FitzSimons, Trent and Oosterhuis2017b), often begins with understanding their biological and reproductive characteristics (Korres et al. Reference Korres, Norsworthy, FitzSimons, Trent and Oosterhuis2017b; Sellers et al. Reference Sellers, Smeda, Johnson and Ellersieck2003). Differences in plant biomass production between early-season and late-season establishment of A. palmeri (Figure 4) were due to changes in plant height (Figures 2 and 3). Taller A. palmeri plants accumulated greater dry weight (Fig. 4), hence higher seed production (Figure 5). These parameters confirm the highly competitive ability of A. palmeri (Trucco and Tranel Reference Trucco and Tranel2011). Toler et al. (Reference Toler, Guice and Murdock1996) reported that the ability of Amaranthus plants to grow taller than soybean is one of the success factors of these weed species in competition with soybean. Studies by Nassiri Mahallati and Kropff (Reference Nassiri Mahallati and Kropff1997) on crop and weed competition for light indicated the important role of increased weed height. This is particularly illustrated by data at 7.3 WAE and 10.7 WAE, when A. palmeri overgrew the crop in 2014 and 2015, respectively (Figure 2).
Amaranthus palmeri flowering
The effects of the distance from the crop on A. palmeri flowering time were not significant in both years, although the greater the distance of A. palmeri from the crop, the greater the number of plants that flowered (unpublished data). Flowering was significantly (P<0.001) affected by establishment time independent of distance from the crop (Figure 6). The shorter the weed establishment time, the greater the number of A. palmeri plants that flowered. More particularly, flowering for A. palmeri plants established at 8 WAE was 33% and 59% lower than for plants established at 0 WAE for 2014 and 2015, respectively (Figure 6). Not surprisingly, the earliest established A. palmeri plants flowered within 7 WAE in 2014 (Figure 6). Likewise, the earliest established A. palmeri reached full flowering between 9.8 and 11.1 WAE (Figure 6) for 2014 and 2015, respectively, probably facilitated by taller A. palmeri plants (Sosnoskie et al. Reference Sosnoskie, Culpepper, Grey and Webster2012). Taller A. palmeri plants were able to avoid shading caused by crop canopy due to dense ground cover as indicated by the relatively strong relationship between ground cover and extinction coefficient around these dates, particularly at 10.6 and 8.4 WAE for 2014 and 2015, respectively (Figures 7 and 8). The greater the ground cover, the higher the (absolute) value of the extinction coefficient, indicating a high light interception.
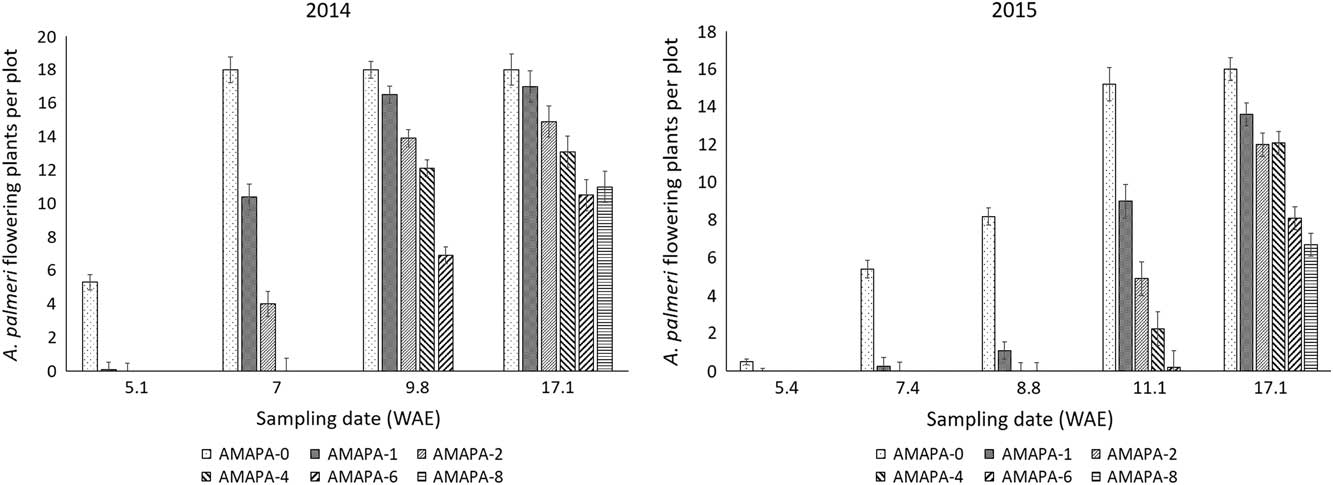
Figure 6 Effects of weed establishment time on Amaranthus palmeri (AMAPA) flowering (averaged across distance from the crop) at various sampling occasions for 0, 1, 2, 4, 6, and 8 wk after soybean emergence (WAE) (i.e., AMAPA-0, AMAPA-1, AMAPA-2, AMAPA-4, AMAPA-6, and AMAPA-8 respectively) in 2014 and 2015. Vertical bars represent±standard error of the mean (i.e., flowering of the entire A. palmeri population was evaluated at each sampling occasion) from the analysis for comparisons within each sampling date (i.e., n=12 plots for 0 WAE, 24 plots for 1 WAE, 36 plots for 2 WAE, etc.).

Figure 7 Relationship between ground cover and extinction coefficient for each sampling date (n=12 plots) throughout the 2014 growing season. WAE, weeks after soybean emergence.
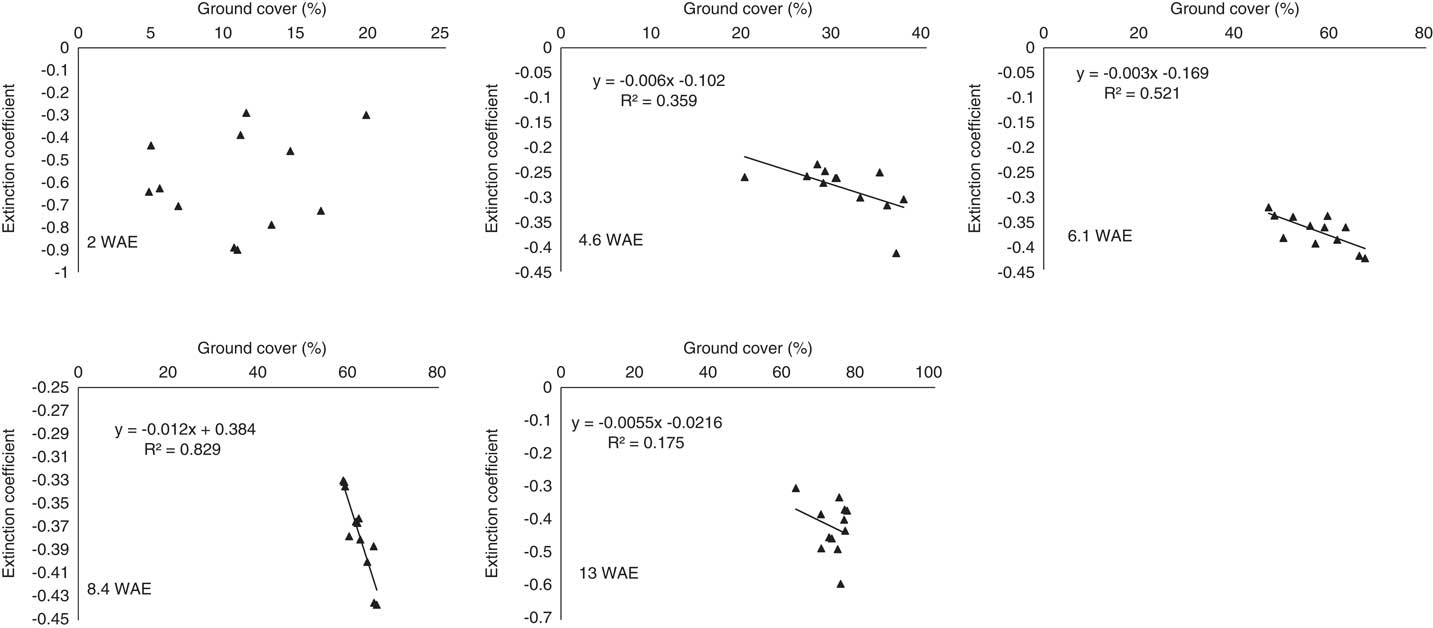
Figure 8 Relationship between ground cover and extinction coefficient for each sampling date (n=12 plots) throughout the 2015 growing season. WAE, weeks after soybean emergence
Such “shade-avoidance” response, which among other parameters involves stem elongation (Morgan and Smith Reference Morgan and Smith1976), may improve plant fitness by increasing capture of the most limiting resource, in this case light, under stressful conditions (Bradshaw Reference Bradshaw1965; Sultan Reference Sultan1987, Reference Sultan2000). Under a crop canopy, both red:far-red (R:FR) ratio and irradiance level along with the impact of these parameters on flowering timing is difficult to predict. In most cases, competition results in either no change or a delay in the onset of reproduction (Weiner Reference Weiner1988). Smith and Whitelam (Reference Smith and Whitelam1997) suggested that reductions in flowering in response to reduced R:FR ratios may be adaptive, because the probability of seed production increases due to crop competition. Alternatively, changes in flowering time may be the indirect consequence of physiological trade-offs and may be unstable if adjustments in flowering initiation result in premature partitioning away from light-capturing tissue (Cohen Reference Cohen1976).
Weed fecundity and biomass are highly dependent upon time of emergence in the crop and proximity of the weed to the crop (Clay et al. Reference Clay, Kleinjan, Clay, Forcella and Batchelor2005; Knezevic and Horak Reference Knezevic and Horak1998). Nevertheless, A. palmeri continued flowering until later in the season (Figure 6) and produced seeds even when it emerged 6 and 8 wk after soybean emergence (Figure 5), despite the increased light interception by crop canopy (Figures 7 and 8).
Brown and Blaser (Reference Brown and Blaser1968) emphasized that plant stands with low k values indicate inefficient light interception by leaves. Nevertheless, Wolf et al. (Reference Wolf, Carson and Brown1972) stated that the extinction coefficient varies when leaf area can no longer be represented by LAI, although other non-leaf structures (e.g., stems) intercept light. In our experiments, soybean was entering into the maturing stage (R5 to R7) at 12.3 to 13 WAE, with consequent initiation of leaf senescence (Setiyono et al. Reference Setiyono, Weiss, Specht, Cassman and Dobermann2008, Reference Setiyono, Cassman, Specht, Dobermann, Weiss, Yang, Conley, Robinson, Pedersen and De Bruin2010).
In addition, it would have been expected that A. palmeri, a species with a C4 photosynthetic pathway (Wang et al. Reference Wang, Klessig and Berry1992), would be sensitive to shade, particularly for plants emerging late in the growing season (Buehring et al. Reference Buehring, Nice and Shaw2002), because this species exhibits prolific growth at high light intensities (Keeley et al. Reference Keeley, Carter and Thullen1987; Massinga et al. Reference Massinga, Currie and Trooien2003). Despite that, it appears that A. palmeri can tolerate shade and grow under crop canopies (Ward et al. Reference Ward, Webster and Steckel2013) and evolve traits that increase its potential to grow and reproduce in various agroecosystems and environmental conditions (Bravo et al. Reference Bravo, Leon, Ferrell, Mulvaney and Wood2017; Korres et al. Reference Korres, Norsworthy, Brye, Skinner and Mauromoustakos2017a, Reference Korres, Norsworthy, FitzSimons, Trent and Oosterhuisb). Patterson (Reference Patterson1985) reported that A. palmeri under reduced light intensity exhibited a noticeable plasticity in acclimation that enabled the plant to survive and produce viable seeds (Jha et al. Reference Jha, Norsworthy, Riley and Bridges2010). Avoiding the development of more aggressive A. palmeri biotypes and considering the consequences of evolutionary change is important in designing cropping systems and weed management strategies (Bravo et al. Reference Bravo, Leon, Ferrell, Mulvaney and Wood2017). The reduced A. palmeri plant biomass at the later soybean stages reflects the greater competitiveness of the crop compared with the earlier A. palmeri establishment dates, when soybean was smaller, and supports the differences in soybean yield loss among these treatments as is discussed in the following section.
Soybean Yield Losses
A significant response between soybean yield and A. palmeri establishment date was found. The shorter the establishment time of A. palmeri in relation to the crop, the greater the soybean yield reduction (P<0.001) (Figure 9). In 2014, soybean yields were reduced by 19%, 15%, and 8.2% when A. palmeri establishment time was 0, 1, and 2 WAE, respectively, compared with yield when establishment time was 8 WAE. Similarly, in 2015, soybean yield was reduced by 23%, 19%, 10%, and 12% when weed establishment time was 0, 1, 2, and 4 WAE, respectively, compared with the yield obtained when weed establishment time was 8 WAE. It should be noted that late weed infestations (i.e., an 8-wk weed establishment time) rarely cause significant soybean yield losses (Bensch et al. Reference Bensch, Horak and Peterson2003; Keramati et al. Reference Keramati, Pirdashti, Esmaili, Abbasian and Habibi2008; Suryanto et al. Reference Suryanto, Tohari Sulistyaningsih, Susila Putra, Kastono and Alam2017; Van Acker et al. Reference Van Acker, Swanton and Weise1993). Highly significant relationships (R2=0.925 and 0.937 for 2014 and 2015, respectively) were revealed when rectangular hyperbolic relationships were fit between soybean yield and weed establishment time in relation to crop emergence (Table 1; Figure 9).
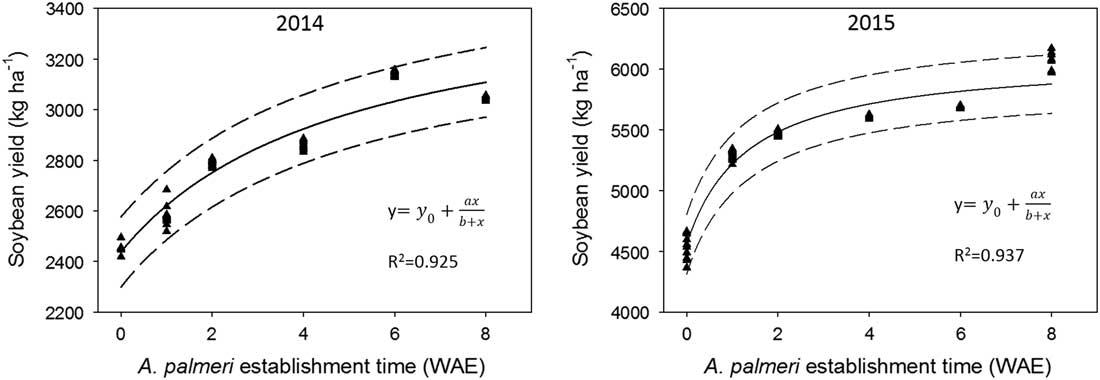
Figure 9 Effects of weed establishment time on soybean yield averaged across Amaranthus palmeri distances from the crop row. Dashed lines indicate the confidence intervals at 95% confidence level (sample size n=72). WAE, weeks after soybean emergence.
Table 1 Parameters of the hyperbolic relationship between soybean yield and Amaranthus palmeri establishment time in weeks after soybean emergence (WAE).
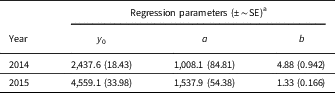
a y 0, minimum theoretical yield value (in kg ha−1); a, range of theoretical kg ha−1 point; b, inflection point (WAE).
Soybean yield reductions in relation to distances from the crop in each year were not significantly different (P=0.846 and P=0.678 for 2014 and 2015, respectively; Figure 10). Amaranthus palmeri is widely considered one of the most frequently occurring and damaging agricultural weeds (Korres et al. Reference Korres, Norsworthy, Bagavathiannan and Mauromoustakos2015; Webster and Nichols Reference Webster and Nichols2012). Soybean yield reductions due to A. palmeri competition were recorded at 78% when 8 A. palmeri plants m−2 emerged and competed with the crop for the entire growing season (Bensch et al. Reference Bensch, Horak and Peterson2003).
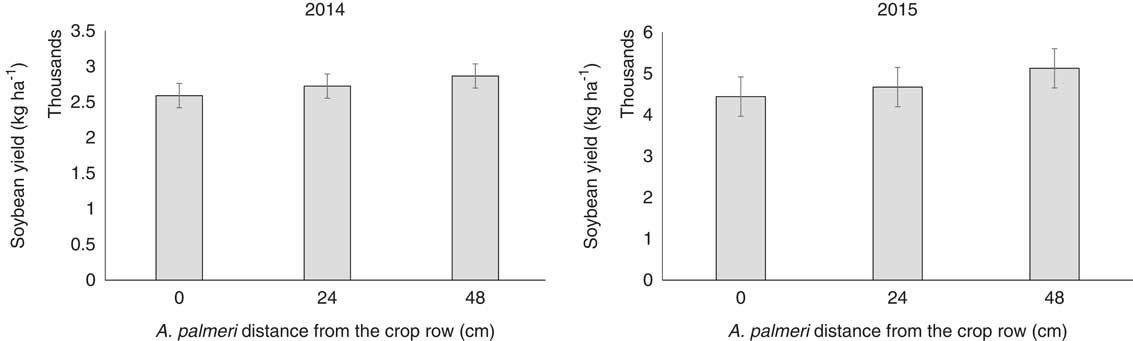
Figure 10 Effects of Amaranthus palmeri distance from the soybean row on crop yield averaged across A. palmeri establishment times. Vertical bars represent±standard error of the mean (SE2014=337.45; SE2015=207.14) from the analysis for comparisons between A. palmeri distances from the crop with sample size n=72.
Establishment time of amaranths regulates the extent of competition with crops but also affects plant size before shorter days convert plants from vegetative to reproductive growth (Goyne and Schneiter Reference Goyne and Schneiter1988). When A. palmeri emerged at 19 to 38 d after planting, no detectable effects of weed competition on soybean yield loss were observed (Bensch et al. Reference Bensch, Horak and Peterson2003).
Bensch et al. (Reference Bensch, Horak and Peterson2003) reported that early-emerging A. palmeri plants also cause greater soybean grain yield reduction compared with later-emerging plants. Similarly, in our study, soybean yield reductions from interference by the early-established A. palmeri plants were found to be higher (i.e., yields reductions were recorded at 23%, 19%, and 12% in 2014 and 24%, 12%, and 9% in 2015 when A. palmeri established at 0, 1, and 2 WAE, respectively, compared with soybean yields when A. palmeri established at 8 WAE). The shorter the weed establishment time, the greater the yield reduction (Figure 9). Amaranthus palmeri biomass was reduced at late weed establishment times (Figure 4), which reflects the greater competitive ability of soybean with late-established A. palmeri plants compared with plants established earlier (i.e., 0 WAE) when soybean is smaller.
Planned programs of weed management depend upon knowledge of the effects of weed competition on crop yield. Action thresholds, which can be used to decide whether or not to spray, for example, can be projected from crop yield–weed density curves (Cousens Reference Cousens1987), assuming an average response and an applicability to the current crop (Cousens et al. Reference Cousens, Firbank, Mortimer and Smith1988). A significant rectangular hyperbolic response between soybean yield and A. palmeri establishment dates was detected for both 2014 and 2015 (Figure 9), indicating the necessity for A. palmeri control within the first 2 wk after its establishment. The parameter estimates of the rectangular hyperbola were relatively stable for both years (Table 1). Care should be taken when interpreting the hyperbolic parameter estimates when they are considered individually. The economic weed control threshold, that is, the level at which control becomes economically worthwhile, can be derived from the yield equations (Table 1; Figure 9) and can be used for weed management decisions.
Conclusions and Future Research
For soybean producers, a weed-free interval within 2 to 3 WAE prevents soybean yield losses in wide-row soybean cropping systems; later-established A. palmeri plants may not affect soybean yields, but will replenish the soil seedbank. The available information to date indicates that under most conditions, narrow-row crop spacing will interfere with soil seedbank replenishments of weeds, hence weed population dynamics, a response that might be related to reduction in light interception at the soil surface. Nevertheless, Esbenshade et al. (Reference Esbenshade, Curran, Roth, Hartwig and Orzolek2001) reported that row spacing had little effect on burcucumber (Sicyos angulatus L.) emergence and control and appears to have little impact on S. angulatus management in corn (Zea mays L.); hence, manipulation of row spacing might depend on crop and weed species.
In addition, results of this research can be used in population dynamics models that depend on estimates of weed biological characteristics such as height, biomass production, and fecundity (Cousens and Mortimer Reference Cousens and Mortimer1995), providing much-needed empirical information for the parameterization of these models under different competitive environments (Bussan and Boerboom Reference Bussan and Boerboom2001).
The competitive abilities of different soybean cultivars against different weed species are not consistent (Datta et al. Reference Datta, Ullah, Tursun, Pornprom, Knezevic and Chauhan2017). It would be of special interest to define and improve the weed-free period (i.e., various weed establishment times) for different soybean cultivars. This could be an invaluable tool for enhancing crop competitiveness with and suppression of highly competitive weeds such as A. palmeri, particularly in conservation systems where soybean yield depends mostly on the timely implementation of weed management (Carkner and Entz Reference Carkner and Entz2017).
Acknowledgments
Funding for this research was provided by the Arkansas Soybean Research and Promotion Board. No conflicts of interest have been declared. The authors would like to thank Ramon Leon (associate editor) and the two anonymous reviewers for their constructive criticism.