Introduction
Rice (Oryza sativa L.) is one of the most important food crops in the world. Globally, China is the leading rice producer and consumer (Tian et al. Reference Tian, Niu, Fan, Sun, Ficsher, Zhong, Deng and Tubiello2018). The direct-seeded rice (DSR) and puddled-transplanted rice (PTR) systems are two different rice cultivation systems used in China. The DSR system is gradually replacing the traditional PTR system due to labor shortages and water scarcity (Tao et al. Reference Tao, Chen, Peng, Wang and Nie2016; Wang et al. Reference Wang, Peng, Liu, Tao, Huang, Cui and Nie2017). In the PTR system, transplanted rice seedlings have a competitive advantage over weeds, because flooding inhibits the early germination of a large number of weeds after rice seedlings are transplanted (Phukan et al. Reference Phukan, Kalita and Bora2021; Chhun et al. Reference Chhun, Kumar, Martin, Srean and Hadi2019). Furthermore, standing water in the PTR system can suppress the germination of many weed species. In contrast, in the DSR system, weeds emerge simultaneously with rice and grow more rapidly than rice seedlings, resulting in competition for nutrients, water, and light (Kumar and Ladha Reference Kumar, Ladha and Sparks2011). Therefore, it is easier for weeds to invade the DSR system than the PTR system, which can result in up to 100% rice yield loss in DSR (Chauhan Reference Chauhan2012; Rao et al. Reference Rao, Johnson, Sivaprasad, Ladha and Mortimer2007; Singh et al. Reference Singh, Bhullar and Chauhan2014).
Smallflower umbrella sedge (Cyperus difformis L.), a highly self-pollinating C3-type annual- emerging aquatic species, is widely distributed in both tropical and warm temperate regions of 47 countries (Holm et al. Reference Holm, Plucknett and Pancho1991). Due to rice cropping-pattern changes, C. difformis has become one of the main troublesome sedge weeds infecting the DSR system areas along the lower–middle Yangtze River, China (Rao et al. Reference Rao, Johnson, Sivaprasad, Ladha and Mortimer2007). The biological characteristics of C. difformis include strong tillering capacity, high fecundity, and growth conditions similar to those of the DSR system, resulting in heavy infestations in DSR system fields compared with PTR system fields (Chauhan and Johnson Reference Chauhan and Johnson2009).
Cyperus difformis is mainly controlled by acetolactate synthase (ALS)-inhibiting herbicides such as bensulfuron-methyl, pyrazosulfuron, penoxsulam, and bispyribac-sodium. Other herbicides (e.g., bentazone, MCPA, and propanil) are also used for control. With increasing labor costs for hand weeding, the increase in herbicide use is expected to continue (Huang et al. Reference Huang, Wang and Xiao2017). Consequently, due to overreliance on herbicides, many weed species in rice fields have evolved resistance to common herbicides such as penoxsulam, quinclorac, cyhalofop-butyl, bensulfuron-methyl, and propanil (Liu et al. Reference Liu, Xiang, Zong, Ma, Wu, Liu, Zhou and Bai2019b; Pedroso et al. Reference Pedroso, Al-Khatib and Fischer2016; Peng et al. Reference Peng, Han, Yang, Bai, Yu and Powles2019; Wei et al. Reference Wei, Jingxuan, Jingyun, Yueyang and Yongrui2019; Yang et al. Reference Yang, Han, Cao, Li, Yu and Powles2021; Zhao et al. Reference Zhao, Fu, Yu, Huang, Yan, Li, Shafi, Zhu, Wei and Ji2017). In particular, C. difformis populations have evolved resistance to ALS-inhibiting herbicides such as bensulfuron-methyl, pyrazosulfuron-ethyl, halosulfuron, penoxsulam, and bispyribac-sodium (Kuk et al. Reference Kuk, Kim, Kwon, Lee, Burgos, Jung and Guh2004; Li et al. Reference Li, Li, Chen, Peng, Wang and Cui2020; Loddo et al. Reference Loddo, Kudsk, Costa, Dalla Valle and Sattin2018; Tehranchian et al. Reference Tehranchian, Riar, Norsworthy, Nandula, Mcelroy, Chen and Scott2015b).
Resistance to ALS-inhibiting herbicides can be endowed by either ALS target-gene mutations or non-target enhanced herbicide metabolism, which correspond to target-site resistance (TSR) and non–target site resistance (NTSR) (Powles and Yu Reference Powles and Yu2010). ALS gene mutations have been commonly reported for ALS-inhibitor resistance (Tranel and Wright Reference Tranel and Wright2002). Previous reports have demonstrated amino acid substitutions at eight conserved positions of the ALS gene (Ala-122, Pro-197, Ala-205, Asp-376, Arg-377, Trp-574, Ser-653, and Gly-654) that confer resistance to ALS-inhibiting herbicides (Murphy and Tranel Reference Murphy and Tranel2019). Mutations at Pro-197 and Trp-574 have been reported in Cyperus species with resistance to ALS-inhibiting herbicides (Ntoanidou et al. Reference Ntoanidou, Kaloumenos, Diamantidis, Madesis and Eleftherohorinos2016; Riar et al. Reference Riar, Tehranchian, Norsworthy, Nandula, Mcelroy, Srivastava, Chen, Bond and Scott2015; Tehranchian et al. Reference Tehranchian, Norsworthy, Nandula, Mcelroy, Chen and Scott2015a, 2015b; Yamato et al. Reference Yamato, Sada and Ikeda2013). Amino acid substitutions by His, Ser, and Ala at position Pro-197 have been reported to confer resistance to ALS-inhibiting herbicides in C. difformis populations (Ntoanidou et al. Reference Ntoanidou, Kaloumenos, Diamantidis, Madesis and Eleftherohorinos2016; Tehranchian et al. Reference Tehranchian, Riar, Norsworthy, Nandula, Mcelroy, Chen and Scott2015b). Recently, Pro-197-Ser/Arg/Leu, Asp-376-Glu, and Trp-574-Leu mutations have been reported in resistant C. difformis populations from China (Li et al. Reference Li, Li, Chen, Peng, Wang and Cui2020).
NTSR due to enhanced metabolic resistance to ALS inhibitors occurs widely in weed species (Han et al. Reference Han, Yu, Owen, Cawthray and Powles2016). Resistance mechanisms other than TSR also provide metabolic resistance, which may result in resistance to multiple herbicide modes of action (Laforest et al. Reference Laforest, Soufiane, Patterson, Vargas, Boggess, Houston, Trigiano and Brosnan2021). Metabolic resistance is the most important NTSR, and its related functional genes include cytochrome P450 monooxygenases (P450s), glycosyltransferases, glutathione S-transferases, and ATP-binding cassette transporters (Délye Reference Délye2013; Yu and Powles Reference Yu and Powles2014; Yuan et al. Reference Yuan, Tranel and Stewart2007). P450s play an important metabolic role in plants under herbicide stress (Bak et al. Reference Bak, Beisson, Bishop, Hamberger, Höfer, Paquette and Werck2011). In most cases of ALS-inhibiting herbicide resistance, P450-mediated herbicide metabolism has been verified in various plant species such as wheat (Triticum aestivum L.), rice, blackgrass (Alopecurus myosuroides Huds.), and rigid ryegrass (Lolium rigidum Gaudin) (Dimaano and Iwakami Reference Dimaano and Iwakami2021; Franco-Ortega et al. Reference Franco-Ortega, Goldberg-Cavalleri, Walker, Brazier-Hicks, Onkokesung and Edwards2021; Han et al. Reference Han, Yu, Beffa, González, Maiwald, Wang and Powles2021). In recent years, many reports also demonstrated that TSR and P450-mediated metabolic resistance coexist in weed species resistant to ALS-inhibiting herbicides (Iwakami et al. Reference Iwakami, Endo, Saika, Okuno, Nakamura, Yokoyama, Watanabe, Toki, Uchino and Inamura2014; Yang et al. Reference Yang, Li, Shen, Xu, Liu, Deng, Li and Zheng2018; Yu et al. Reference Yu, Abdallah, Han, Owen and Powles2009). The involvement of P450s in herbicide metabolism has been confirmed by indirect evidence (Busi et al. Reference Busi, Gaines and Powles2017). For example, the P450 inhibitor malathion was used to detect herbicide metabolism indirectly in resistant populations (Guo et al. Reference Guo, Riggins, Hausman, Hager, Riechers, Davis and Tranel2015; Kaspar et al. Reference Kaspar, Grondona, Leon and Zambelli2011; Zhao et al. Reference Zhao, Yan, Ge, Zhu, Liu and Wang2019).
Bensulfuron-methyl, an ALS-inhibiting herbicide, is widely used to control C. difformis in the rice fields of China. In recent years, farmers have complained that field applications of bensulfuron-methyl have been ineffective in controlling C. difformis populations in Hunan Province, well known to have the most DSR production system areas. However, the PTR system is the primary planting system used in Guangxi Province, China. Therefore, C. difformis seeds were harvested from major rice-producing regions of Hunan and Guangxi provinces, China. Following seed harvesting, studies were implemented to compare and investigate [1] C. difformis resistance levels to the ALS-inhibiting herbicide bensulfuron-methyl, [2] cross-resistance to pyrazosulfuron, bispyribac-sodium, penoxsulam, and imazapic, and [3] multiple resistance to bentazone, propanil, and MCPA to understand the potential mechanisms of resistance to ALS-inhibiting herbicides.
Materials and Methods
Plant Material
Cyperus difformis seeds were collected from rice fields of Hunan and Guangxi provinces, where ALS-inhibiting herbicides have been used for at least 15 yr. Susceptible (S) populations (HN-S and GX-S) were harvested from crop regions without herbicide exposure. The sensitivity assays of S populations were performed at half the recommended dose of bensulfuron-methyl in the field. The sample information, including geographic distribution, field coordinates, and cultivation method, were recorded (Figure 1; Table 1). The harvested C. difformis seeds were air-dried and stored at 4 C until they were used in experiments.
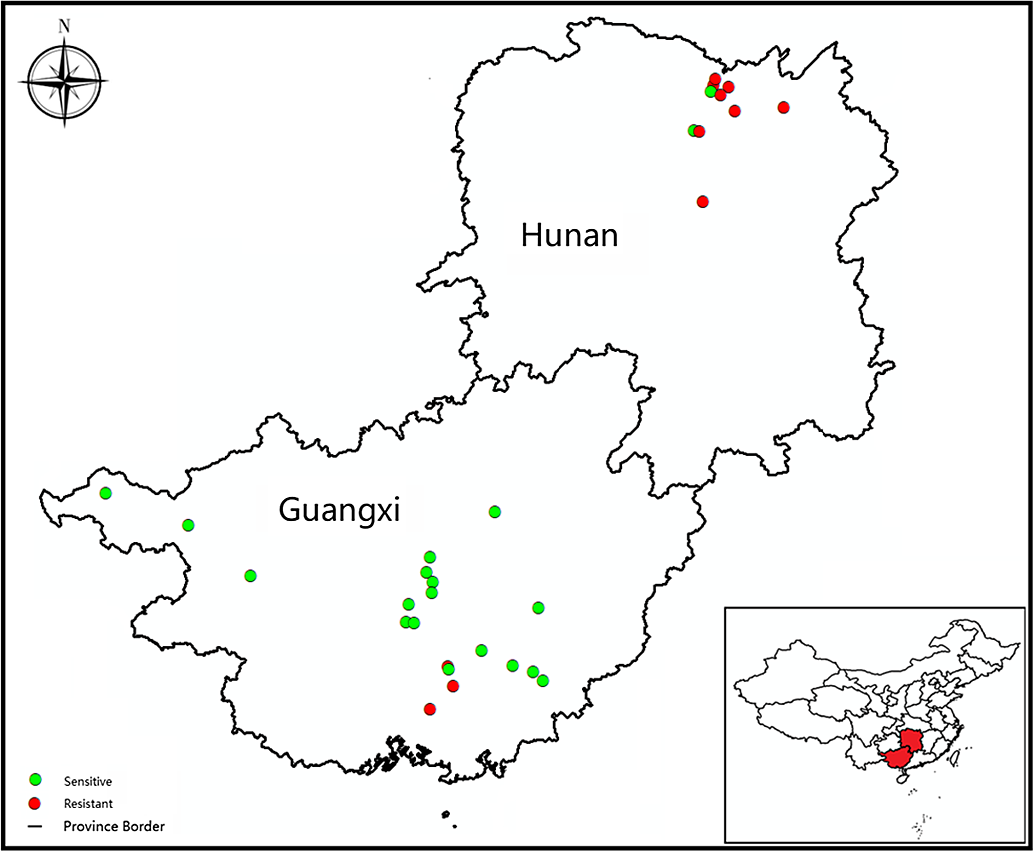
Figure 1. The distribution and resistance profiles to bensulfuron-methyl of Cyperus difformis populations in this study.
Table 1. Recorded Cyperus difformis populations information in this study.
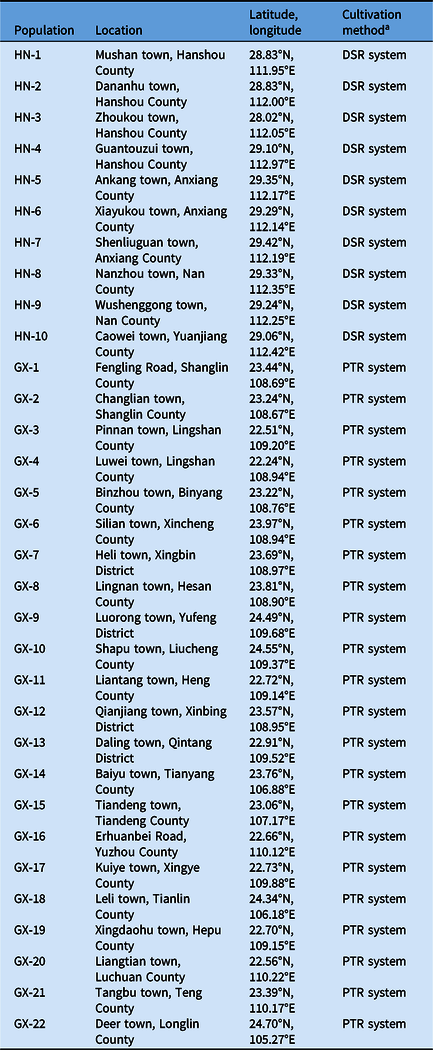
a DSR, direct-seeded rice; PTR. puddled-transplanted rice.
Single-Rate Herbicide Test
The seeds of all populations were germinated in petri plates containing 20 ml of 0.6% agar at room temperature. After emergence, young seedlings were transplanted into 22-cm (length) × 12.5-cm (width) × 7-cm (depth) plastic pots containing loam soil with 40 seedlings per pot. The seedlings were grown in a greenhouse under natural sunlight and were supplemented with instant NPK fertilizer (N ≥ 13.5%, P2O5 ≥ 15%, K2O ≥ 15%) once every 2 wk and watered daily. At the 2- to 3-leaf stage, plants were sprayed with bensulfuron-methyl at 45 g ai ha−1. Herbicide applications were conducted in a moving sprayer equipped with one TPV 9501E flat-fan nozzle (Guangxi Pastoral Biochemistry, Inc., Nanning, Guangxi, China 530000) calibrated to deliver 675 L ha−1 at 0.4 MPa. Plant survival was recorded as a percent at 28 d after treatment (DAT), and the mature seeds of suspected resistant (R) populations were collected to air-dry for screening experiments.
Dose–Response Experiments
Seeds from each population were evenly distributed on the 0.6% agar to germinate (16-h light/8-h dark, 35/25 C, with ∼75% relative humidity) for 2 wk and were then transplanted into 10-cm-diameter plastic pots filled with loam soil (15 seeds per pot) and cultured in a greenhouse as previously described. After 1 wk, seedlings were thinned to 10 uniformly sized seedlings per pot (three repetitions per treatment). At the 2- or the 3-leaf stage, C. difformis populations were treated with varying rates of bensulfuron-methyl at 0, 0.7, 1.4, 2.8, 5.6, and 11.2 g ai ha−1 for S populations; 0, 22.5, 45.0, 90.0, 180.0, and 360.0 g ai ha−1 for HN-8 from Hunan Province and R populations from Guangxi Province; and 0, 45.0, 90.0, 180.0, 360.0, 720.0, 1,440.0, and 2,880.0 g ai ha−1 for R populations from Hunan Province. The experiment was designed as a randomized complete block with three replications per treatment. At 28 DAT, plant survival was recorded, and the aboveground parts of the plant were harvested and then weighed after being oven-dried for 72 h at 80 C.
Susceptibility to Multiple Herbicides
The susceptibility of bensulfuron-methyl R populations, which were the offspring of survivors from bensulfuron-methyl–treated populations, was tested against multiple herbicides with different modes of action (Table 2). Cyperus difformis populations were planted as described earlier. Following germination, 40 seedlings per pot were planted for each R C. difformis population. At the 3- to 4-leaf stage, plants were sprayed with the herbicides at recommended doses (Table 2).
Table 2. Herbicides and applied rates in single-dose experiments.

a IMI, imidazolinone; PTB, pyrimidinyl-thiobenzoate; SU, sulfonylurea; TP, triazolopyrimidine.
b ALS, acetolactate synthase; PSII, photosystem II.
c AS, aqueous solutions; OD, oil dispersion; SC, suspension concentrate; SL, soluble concentrate; WP, wettable powder.
ALS Gene Sequencing
Total RNA from leaf tissue was extracted from untreated S and R plants (10 plants per population) using the UNlQ-10 Column Trizol Total RNA Isolation Kit (Sangon Biotech, Shanghai, China). RNA was quantified using the NanoDrop 2000 spectrometer (Thermo Fisher Scientific, Waltham, MA, USA). The cDNA was synthesized from 1 μg of RNA using the First Strand cDNA Synthesis Kit (Sangon Biotech). To identify the potential mutation in the conserved ALS gene regions, five pairs of primers (Table 3) were designed and synthesized based on the ALS sequence of C. difformis (GenBank EF061294.2). The primers were able to amplify fragments with theoretical sizes of 596, 357, 488, 946, and 1,934 bp, containing seven codons of Ala-122, Pro-197, Ala-205, Asp-376, Trp-574, Pro-653, and Ser-654 (Tehranchian et al. Reference Tehranchian, Riar, Norsworthy, Nandula, Mcelroy, Chen and Scott2015b). Four cDNA sequences amplified using four primer pairs (1, 2, 3, and 4 in Table 3) could be assembled into a 1,673-bp sequence. In addition, the 5th primer pair (allALSF and allALSR) was used to amplify a full-length sequence containing seven known mutation sites.
Table 3. Primer pairs designed for amplification of Cyperus difformis ALS gene.

PCR amplification was conducted in a 25-μl volume containing 1 μl of cDNA (100 ng μl−1), 1 μl of each primer (10 μM), 9.5 μl of double-distilled water (ddH2O), and 12.5 μl of 2×Taq PCR StarMix with Loading Dye (GenStar, Beijing, China). PCR was performed on the thermal cycling system (Applied Biosystems, Foster City, CA, USA) with the following profile: denaturing at 94 C for 4 min; then 40 cycles of 94 C for 30 s, annealing for 30 s at 53, 55, 57, 56, and 56 C for the five primer pairs, respectively (Table 3), and 72 C for 60 s; followed by a final extension step of 10 min at 72 C. Amplified PCR products were sequenced by Sangon Biotech. The sequencing data were visually checked and aligned using multiple sequence comparisons in DNAMAN v. 7.1 software (Edgar Reference Edgar2004).
Metabolic Resistance Screen
The enhanced metabolism of P450s is one of the reasons for metabolic resistance in resistant populations. Malathion (P450 inhibitor) was used to test metabolic resistance to bensulfuron-methyl in R populations of C. difformis. According to our preliminary experiments, 1,000 g ai ha−1 of malathion was applied 2 h before bensulfuron-methyl treatment. Cyperus difformis was cultivated following the same method described earlier. Briefly, 40 seedlings of R offspring (2- to 3-leaf stage) were sprayed with malathion plus bensulfuron-methyl or with bensulfuron-methyl alone in a greenhouse. The rate of surviving plants was recorded as a percentage at 28 DAT.
Statistical Analysis
Data obtained from the dose–response experiments were subjected to a nonlinear regression analysis using a three-parameter equation (Equation 1) in Sigma Plot v. 12.5 (Systat Software, San Jose, CA, USA). The GR50 value (the herbicide dose resulting in 50% growth inhibition) was calculated as follows:

where x is the herbicide concentration, y is the dry biomass percentage of the untreated control, x 0 is GR50, a is a constant, and b is the slope of the curve.
The resistance index (RI) was calculated as the GR50 of the R population divided by the GR50 of the S population.
In the metabolic resistance experiments, data for bensulfuron-methyl metabolism were analyzed by independent-samples t-test (P < 0.05) in SPSS v. 22.0 (IBM, Armonk, NY, USA) to determine whether the use of malathion would affect the populations resistant to herbicides.
Results and Discussion
Herbicide Response Experiments of Bensulfuron-Methyl
A total of 32 C. difformis populations were tested with bensulfuron-methyl at the recommended dose (45 g ai ha−1). The two S populations, HN-S and GX-S, died at this herbicide rate, while populations that survived this rate were considered resistant. In this study, 11 of the 32 populations survived this rate with variable survivorship ranging from 10% to 100% (data not shown), suggesting that these populations had evolved different resistance levels to bensulfuron-methyl. Of the 10 populations from the DSR system of Hunan Province, 8 were resistant to bensulfuron-methyl, and 3 out of those 8 R populations (HN-3, HN-9, and HN-10) had 100% survival at the recommended dose. There were only three R populations from the PTR systems of Guangxi Province with a survival rate of <30%. Based on these findings, 2 S populations (HN-S and GX-S) and 11 putative R populations (HN-2, HN-3, HN-4, HN-5, HN-7, HN-8, HN-9, HN-10, GX-3, GX-4, and GX-11) were studied further in the following experiments.
Compared with the S populations, the putative R populations were confirmed and exhibited various resistance levels to bensulfuron-methyl (Table 4). The GR50 value for R biotypes ranged from 28.0 to >2,880 g ai ha−1 (Table 4), while the two S biotypes showed a similar GR50 value lower than the recommended dose. The RI of Hunan Province populations for bensulfuron-methyl ranged from 31.7- to >1,309-fold. Meanwhile, the RI of Guangxi Province populations showed from 12.7- to 31.2-fold resistance to bensulfuron-methyl compared with the S populations. The highest and lowest resistance levels to bensulfuron-methyl were exhibited by HN-9 and GX-11, respectively (Figure 2). These results reveal that the DSR system’s R populations from Hunan Province show higher resistance levels than those from the Guangxi Province PTR system.
Table 4. Parameter values of dose–response curves to bensulfuron-methyl for Cyperus difformis.
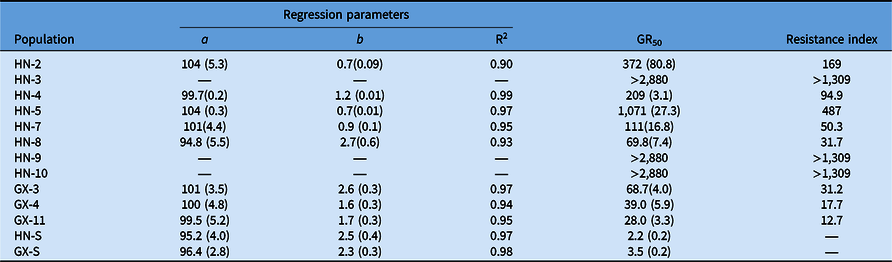

Figure 2. Dose–response curves for bensulfuron-methyl–susceptible (HN-S) and bensulfuron-methyl–resistant (HN-4, HN-9, GX-11) Cyperus difformis populations based on aboveground dry biomass 28 d after treatment.
This is the first study to compare herbicide-resistance evolution in C. difformis from DSR and PTR systems in China. The frequency and level of resistance to bensulfuron-methyl were much higher in C. difformis populations in the DSR system fields of Hunan Province than in the PTR system fields of Guangxi Province. Several factors potentially explain this situation. The transition from the PTR to DSR system in Hunan Province has resulted in overreliance on herbicides. ALS-inhibiting herbicides are applied up to four times annually in the rice fields of Hunan Province (Liu et al. Reference Liu, Xiang, Zong, Ma, Wu, Liu, Zhou and Bai2019b). In contrast, the PTR system is adopted in most areas of Guangxi Province, where the fields are continuously flooded throughout the growing season (Ling et al. Reference Ling, Yuan, Peng and Li2021). The water layer easily controls C. difformis by inhibiting the germination of weed seeds. In addition, paddy fields in Guangxi Province are small and scattered, making it easier to manage weeds with traditional manual labor (Wu et al. Reference Wu, Liang and Tong2020).
The herbicide bensulfuron-methyl had been used for weed control in Hunan Province since the 1990s, much earlier than in Guangxi Province (Li and Chen Reference Li and Chen1991; Wang et al. Reference Wang, Ma, Chen and Zhou1999). The repetitive and continuous use of ALS-inhibiting herbicides for the last 15 yr led to the development of herbicide resistance in weeds of the rice fields in China (Liu et al. Reference Liu, Xiang, Zong, Ma, Wu, Liu, Zhou and Bai2019b; Wu et al. Reference Wu, Cao, Du and Cui2005). In recent years, the resistance of weeds to ALS-inhibiting herbicides has also been reported in a few paddy fields of Hunan Province. (Li et al. Reference Li, Li, Chen, Peng, Wang and Cui2020; Liu et al. Reference Liu, Fang, He, Li and Dong2019a; Peng et al. Reference Peng, Han, Yang, Bai, Yu and Powles2019). In fact, resistant C. difformis populations have been one of the most troublesome weed problems in Hunan Province. These results indicate that resistance evolution in C. difformis is related to both cultural methods and the repeated use of ALS-inhibiting herbicides.
Susceptibility to Multiple Herbicides
In this study, the field-recommended dose of multiple ALS-inhibiting herbicides was used to test the cross-resistance in R populations. The HN-8 and GX-11 populations showed cross-resistance to sulfonylurea (SU, pyrazosulfuron) and imidazolinone (IMI, imazapic), while GX-3 and GX-4 had cross-resistance to SU (pyrazosulfuron), triazolopyrimidine (TP, penoxsulam), and IMI (imazapic). Other R populations exhibited cross-resistance to all herbicides tested (Table 5). Our experiments demonstrated that C. difformis populations in China had different resistance levels to bensulfuron-methyl and cross-resistance to multiple ALS inhibitors. Similar results were also reported in C. difformis and the sedge species bog bulrush [Schoenoplectus mucronatus (L.) Palla], annual sedge (Cyperus compressus L.), and rice flatsedge (Cyperus iria L.) with different target-site mutations (Li et al. Reference Li, Li, Chen, Peng, Wang and Cui2020; McCullough et al. Reference McCullough, Yu, McElroy, Chen, Zhang, Grey and Czarnota2016; Riar et al. Reference Riar, Tehranchian, Norsworthy, Nandula, Mcelroy, Srivastava, Chen, Bond and Scott2015; Yamato et al. Reference Yamato, Sada and Ikeda2013).
Table 5. Percentage of surviving plants from resistant (R) and susceptible (S) Cyperus difformis populations in response to multiple ALS-inhibiting herbicides. a
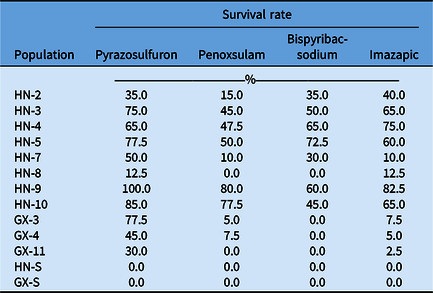
a Forty plants of each population were treated with each herbicide at the field-recommended dose.
Additionally, all populations died when field-recommended doses of other herbicides with non–ALS inhibiting chemistries, such as MCPA, bentazone, and propanil, were applied. This indicates that these populations were still susceptible to MCPA, bentazone, and propanil. Based on these results, we suggest that farmers could also use a mixture of herbicides to control ALS resistance in sedges. For example, the mixture of MCPA and bentazone is an effective way to prevent resistance in C. difformis via foliar application. Previously, herbicide mixtures have been reported as one of the most effective methods for management of resistant weeds (Comont et al. Reference Comont, Lowe, Hull, Crook, Hicks, Onkokesung, Beffa, Childs, Edwards, Freckleton and Neve2020).
Sequencing and Analysis of ALS Gene
Ten individual plants per population were amplified using five pairs of primers, and the sequencing results were compared with the full-length ALS gene of R and S populations. PCR products containing base mutation sequences were purified and transformed into Escherichia coli to select several monoclonal clones for cloning and sequencing to confirm the correctness of the mutation sites. The sequence with point mutations detected by cloning and sequencing was confirmed to endow resistance to ALS-inhibiting herbicides. All assembled sequences from different populations had approximately 99.5% similarity to the previously published ALS gene of C. difformis (GenBank accession EF061294.2).
The alignment of the ALS cDNA sequence showed Pro-197-Leu/Ser mutations in five populations (HN-2, HN-4, HN-8, GX-4, and GX-11), an Asp-376-Glu mutation in three populations (HN-4, HN-7, and HN-10), and a Trp-574-Leu mutation in five populations (HN-2, HN-3, HN-5, HN-9, and HN-10). The Trp-574-Leu mutation was found in more than 70% of individuals in the three populations with higher resistance levels (HN-3, HN-9, and HN-10). That means the frequency of Trp-574-Leu mutations was the highest in the DSR systems from Hunan Province, but this mutation was not identified in the PTR system from Guangxi Province. Pro-197-Ser mutations were identified in two populations (GX-4 and GX-11) from PTR systems. In addition, none of the populations contained any mutation at Pro-653 and Ser-654, and no ALS gene mutation was detected in the R population of GX-3.
Generally, TSR depends on precise mutations, the ALS-inhibiting herbicide’s structure, and the weed species. It has been reported that eight ALS gene mutations conferring resistance to ALS-inhibiting herbicides have been identified in resistant plants (Murphy and Tranel Reference Murphy and Tranel2019). The target-site mutation at codon Pro-197 could have substitutions of Ala, Ser, Arg, and His, which confer cross-resistance to different ALS inhibitors in C. difformis (Li et al. Reference Li, Li, Chen, Peng, Wang and Cui2020). In this study, the populations with Pro-197-Ser were cross-resistant to penoxsulam, imazapic, and bispyribac-sodium. As previously documented, the Pro-197-Ser substitution results in broad-spectrum resistance to SU, TP, PTB (pyrimidinyl-thiobenzoate), or SCT (sulfonyl-aminocarbonyl-triazolinone) herbicides but low or no resistance to IMI herbicides in weeds (Zhao et al. Reference Zhao, Fu, Yu, Huang, Yan, Li, Shafi, Zhu, Wei and Ji2017). Pro-197-Ser/Leu/Arg substitution in C. difformis from Anhui Province in China also conferred cross-resistance to penoxsulam, but plants were sensitive to imazapic and bispyribac-sodium (Li et al. Reference Li, Li, Chen, Peng, Wang and Cui2020).
The Asp-376-Glu mutation was also reported in three R C. difformis populations from China. In this work, five out of eight R populations from Hunan Province were identified as Trp-574-Leu mutants, which revealed the highest resistance level and broad-spectrum resistance to all five classes of ALS-inhibiting herbicides (Table 6). A recent study reported that the Trp-574-Leu mutation was detected in 2 of the 12 resistant populations collected in 2012 to 2015 from Hunan Province (Li et al. Reference Li, Li, Chen, Peng, Wang and Cui2020). These results indicate that resistance of C. difformis populations has evolved in recent years. Thus, resistance evolution to ALS-inhibiting herbicides needs to be focused on in the long term.
Table 6. Frequency of different codons and derived amino acids in the ALS gene in different Cyperus difformis populations.

Metabolic Resistance to Bensulfuron-Methyl
When malathion was applied alone at 1,000 g ai ha−1, no significant influence was observed in the growth of either the R or S populations. As described in Table 7, using malathion 2 h before applying bensulfuron-methyl enhanced the efficacy of bensulfuron-methyl in Hunan (HN-2, HN-3, HN-4, and HN-8) and Guangxi populations (GX-3, GX-4, and GX-11). Moreover, the mortality of these populations showed a significant difference after being treated with malathion (P < 0.05). This finding indicated that P450s likely contribute to bensulfuron-methyl resistance in C. difformis. Other than that, the resistance observed in the HN-5, HN-9, and HN-10 populations was not reversed by malathion. Combined with the changes in mortality, we deduced that the high level of resistance caused by the Trp-574-Leu mutation masked the effect of metabolic resistance. However, these results require further tests to determine whether metabolic resistance works in combination with other NTSR to form herbicide resistance in C. difformis.
Table 7. Mortality rate and significance test of Cyperus difformis populations treated by bensulfuron-methyl in the presence and absence of malathion.

a Data are mean.
* Significant difference at P < 0.05 level by t-test.
The GX-3 population with no mutations was resistant to bensulfuron-methyl for several reasons. First, recent research has demonstrated NTSR (especially P450s) can play an essential role in weed resistance to ALS-inhibiting herbicides (Dimaano and Iwakami Reference Dimaano and Iwakami2021). Our preliminary experiments showed an apparent synergistic effect of the combined application of bensulfuron-methyl plus malathion on R C. difformis populations, which indicated the likely involvement of P450s with resistance in some R populations. Several studies have also demonstrated that P450s were correlated with herbicide resistance in different weed species (Dimaano and Iwakami Reference Dimaano and Iwakami2021; Han et al. Reference Han, Yu, Beffa, González, Maiwald, Wang and Powles2021; Iwakami et al. Reference Iwakami, Kamidate, Yamaguchi, Ishizaka, Endo, Suda, Nagai, Sunohara, Toki and Uchino2019). Second, as some members of Cyperaceae are polyploid, resistance alleles may be homozygous in the genome (Tejavathi and Nijalingappa Reference Tejavathi and Nijalingappa1990). The ploidy and NTSR of C. difformis need further studies to evaluate their relationship with resistance in the future.
Plants metabolize herbicides before they reach the active site, which means that the amount of herbicide metabolization is critical for weed control (Khaledi et al. Reference Khaledi, Fayaz, Kahrizi and Talebi2019). P450s are key metabolic enzymes present in many crops and weeds and perform metabolic functions (Dimaano and Iwakami Reference Dimaano and Iwakami2021; Nandula et al. Reference Nandula, Riechers, Ferhatoglu, Barrett, Duke, Dayan, Goldberg-Cavalleri, Tétard-Jones, Wortley, Onkokesung, Brazier-Hicks, Edwards, Gaines, Iwakami, Jugulam and Ma2019). Many RNA-seq studies have directly demonstrated that P450s are involved in herbicide metabolism, such as in American sloughgrass [Beckmannia syzigachne (Steud.) Fernald], flixweed [Descurainia sophia (L.) Webb ex Prantl], and Echinochloa glabrescens Munro ex Hook. f. (Pan et al. Reference Pan, Gao, Xia, Zhang and Dong2016; Yan et al. Reference Yan, Zhang, Li, Fang, Liu and Dong2019; Yang et al. Reference Yang, Li, Shen, Xu, Liu, Deng, Li and Zheng2018). Therefore, enhanced P450-mediated herbicide metabolism should be further investigated in resistant weed species.
The experiments provide evidence that TSR and P450-mediated metabolic resistance coexist in most R populations. These results are in accordance with previous reports of different weed species’ resistance to ALS-inhibiting herbicides (Bai et al. Reference Bai, Zhang, Li, Wang, Wang, Wang, Liu and Bai2019; Han et al. Reference Han, Yu, Owen, Cawthray and Powles2016; Yang et al. Reference Yang, Deng, Li, Yu, Bai and Zheng2016). Metabolic resistance to ALS-inhibiting herbicides has resulted in rapid evolution (Beckie and Tardif Reference Beckie and Tardif2012; Laforest et al. Reference Laforest, Soufiane, Patterson, Vargas, Boggess, Houston, Trigiano and Brosnan2021). Metabolic herbicide resistance can potentially endow resistance to many herbicides and poses a threat to herbicide sustainability and thus crop production, calling for major research and management efforts. The integrated weed management approach includes the suppression of weeds through nonchemical methods, such as the use of allelopathic rice cultivars, deep flooding, alternation between water- and dry-seeded systems, and crop rotation (Liu et al. Reference Liu, Merchant, Xiang, Zong, Zhou and Bai2021). For example, allelopathic rice inhibited resistant barnyardgrass [Echinochloa crus-galli (L.) P. Beauv.] more than susceptible cultivars (Yang et al. Reference Yang, Kong, Yang and Li2017). Australian grain growers have developed harvest weed seed control (HWSC) practices to reduce the viability of weed seeds by preventing weed seeds from escaping and entering the seedbank (Somerville et al. Reference Somerville, Powles, Walsh and Renton2018; Walsh et al. Reference Walsh, Broster, Norsworthy, Davis, Tidemann, Beckie, Lyon, Soni, Neve and Bagavathiannan2018).
This is the first study to compare C. difformis resistance to bensulfuron-methyl in different planting systems in China. Our findings revealed that Trp-574-Leu mutations in ALS inhibitor–resistant C. difformis are most common in the PTR system of Hunan Province and result in high-level resistance to ALS-inhibiting herbicides. In contrast, Pro-197-Ser and NTSR are the main reason for resistance in the DSR system. The cross-resistance to ALS-inhibiting herbicides was the result of TSR and NTSR. Management practices to control resistant C. difformis populations vary in different cultivation systems. Our study suggests that integrated weed management combined with tillage methods and nonchemical methods to suppress weeds will be critical for delaying the development of ALS inhibitor–resistant weeds in China.
Acknowledgments
The authors are grateful to the anonymous reviewers for their constructive comments and suggestions. This research was supported by the Guangxi Natural Science Foundation (2021GXNSFDA220007), the National Key R&D Program of China (2018YFD0200300), the National Natural Science Foundation of China (31460479 and 31760522), the Research Funding of Guangxi Academy of Agricultural Sciences (2018YM23), and the Guangxi Key Laboratory of Biology for Crop Diseases and Insect Pests (2019-ST-02). The authors have declared that no conflict of interest exists.