Monozygotic twins became increasingly concordant with age and also paralleled each other for the spurts and lags in development. Dizygotic twins became less concordant with age and eventually matched their singleton siblings as closely as one another. The overall results suggested that the course of mental development is guided by the intrinsic scheduling of the genetic program acting in concert with maturational status and environmental influence. (Wilson, Reference Wilson1978, p. 439).
The fact that the heritability of IQ increases with age is now a widely accepted phenomenon (Boomsma et al., Reference Boomsma, Busjahn and Peltonen2002; Deary, Reference Deary2012; Johnson, Reference Johnson2010b). Such acceptance, even recognition, was not always the case. Early studies focused mostly on children and seldom explored the effect of age. The classic review of family studies by Erlenmeyer-Kimling and Jarvick (Reference Erlenmeyer-Kimling and Jarvick1963) does not mention age effects on kin correlations nor (embarrassingly for this author) does the 1981 review by Bouchard and McGue (Reference Bouchard and McGue1981) or the review by Plomin and DeFries (Reference Plomin and DeFries1980). As late as 1997, Devlin et al. (Reference Devlin, Daniels and Roeder1997) asserted there were no age effects on the heritability of IQ.
The purpose of this paper is to provide a striking illustration of this phenomenon and provide it with a name — ‘The Wilson Effect’ — in honor of Ronald Wilson, the developmental behavior geneticist who most clearly articulated its existence and emphasized the importance of putting the findings in both a developmental and evolutionary context (Wilson, Reference Wilson1978, Reference Wilson1983). For those not familiar with Wilson's work, Plomin (Reference Plomin1987) provides a thoughtful summary of his career.
Figure 1 from Wilson (Reference Wilson1983) is a plot of kin correlations across age. The data were gathered by the justly famous Louisville Longitudinal Twin Study. The plot illustrates the changes in kin correlations that mediate the effect; relatively stable monozygotic twin (MZ) correlations from 2 years of age and decreasing same-sex dizygotic twin (DZ) correlations after 4 years of age. The converging of sibling–twin correlations with the DZ correlations at 6 years of age and the increasing age-to-age correlations complement the twin correlations. The effect is well defined by 5–6 years of age when the age-to-age correlations approximate the MZ correlations.

FIGURE 1 Mental development correlations for MZ twins, DZ twins, twin–sibling sets, parent–offspring sets; and for each child with itself, age to age.
Figure 2 puts together a number of key studies (large and representative twin samples and some smaller but statistically powerful adoption designs) that illustrate the generality of the Wilson Effect.
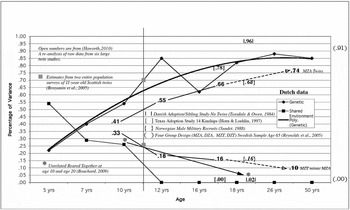
FIGURE 2 Estimates of genetic and shared environmental influence on g by age. The age scale is not linear (see text for details).
The Dutch data are from Boomsma et al. (Reference Boomsma, Busjahn and Peltonen2002) and consist of eight cross-sectional twin studies that provide a frame of reference for the other studies. For illustrative purposes, a polynomial curve (solid black line) has been fit to the estimates of genetic influence (heritabilities) and it reaches an asymptote at about age 20. The estimates of shared environmental influence (common environment) show a linear decrease (black squares) from about 0.55 at age 5 to 0.00 at age 12 and remain at 0.00 through 50 years of age. These findings are from an advanced industrialized society with a sophisticated social welfare system and provide a baseline against which other data can be compared. All genetic estimators in this study and those to be added below are of the broad heritability (hb 2), which includes additive genetic variance, dominance genetic variance, and genetic variance due to assortative mating (Vinkhuyzen et al., Reference Vinkhuyzen, van der Sluis, Maes and Posthuma2012; Visscher et al., Reference Visscher, Hill and Wray2008). Common or shared environmental estimates (c 2) differ slightly depending on the particular design, but the values are all quite low so it would take very large samples to partition any differences. Most twin studies set c 2 to zero if the estimate is not statistically significant. This is an unfortunate practice in our opinion.
How do the Dutch results compare to data sets generated in other settings? Haworth et al. (Reference Haworth, Wright, Luciano, Martin, de Geus, van Beijsterveldt and Plomin2010) combined the raw IQ data from six large twin studies (three from the United States, one from the United Kingdom, one from Australia, and one from the Netherlands). These data were divided into three age groups; mean ages of 9, 12, and 17 years. The findings are shown as numbers with no associated coding in the figure (hb 2 = 0.41, 0.55, and 0.66; c 2 = 0.33, 0.28, and 0.16). The heritabilities go up with age, and the line runs parallel with the Dutch data but lies about 10% below it. There may be some overlap in the Dutch sample in this collection of samples with the Boomsma et al. (Reference Boomsma, Busjahn and Peltonen2002) Dutch data.
What happens if we add data from monozygotic twins reared apart (MZA)? These twin correlations estimate the broad heritability directly (Bouchard, Reference Bouchard2009). The mean of five studies of adults is 0.74. The line from the Haworth data at age 17 is projected (- - ->) to the MZA data and it largely parallels the Dutch data. We can also estimate shared environmental influence on adult IQ by subtracting the adult MZA correlation from the monozygotic twins reared together (MZT) correlation. An estimate of the MZT correlation in advanced industrialized countries (Norway, 1181 pairs, age 18 to 21) is 0.81 (Sundet et al., Reference Sundet, Tambs, Harris, Magnus and Torjussen2005), although an earlier sample suggests 0.84 (Sundet et al., Reference Sundet, Tambs, Magnus and Berg1988). Devlin et al. (Reference Devlin, Daniels and Roeder1997) updated the Bouchard and McGue (Reference Bouchard and McGue1981) estimate of 0.86 and reported a correlation of 0.85. Finkel et al. (Reference Finkel, Pedersen, McGue and McClearn1995) report 0.80 and 0.84 for middle-aged Minnesota and Swedish samples. We use 0.84. This gives an estimate of 0.10 for shared environment and that value has been entered into Figure 2. The results for shared environment are almost a mirror image of the genetic results.
There are a variety of arguments commonly made against twin studies (Bouchard & McGue, Reference Bouchard and McGue2003). One is that they are volunteer samples and do not represent the entire range of environments experienced by the population to which we wish to generalize. Ian Deary's group in Edinburgh, Scotland has addressed this question. They have shown ‘Large, consistent estimates of the heritability of cognitive ability in two entire populations of 11-year-old twins from Scottish Mental Survey of 1932 and 1947’ (Benyamin et al., Reference Benyamin, Wilson, Whalley, Visscher and Deary2005). The estimates are hb 2 = 0.70 and c 2 = 0.21. The large and intentionally population-representative Minnesota Twin Family Study carried out a cognitive ability assessment at age 11 as well. The results were almost identical, h 2 = 0.67 and c 2 = 0.26 (Johnson et al., Reference Johnson, McGue and Iacono2005). These combined data are shown as filled boxes on the line drawn at age 11 years in Figure 2. There is also a Norwegian data set based on military recruits (again a comprehensive sample that includes all males tested in their late teens to early twenties) gathered from 1931 to 1960 (Sundet et al., Reference Sundet, Tambs, Magnus and Berg1988). When we add those values, 0.68 and 0.16 and shown in braces {}, they fall right on the lines connecting the Haworth and MZA data.
The very modest shared environmental influence by mid-childhood revealed by twin studies always puzzles psychologists, as they intuitively expect siblings to become more similar as they spend more time together in the same family and its social and educational environment. Therefore, it is important to subject this counter-intuitive finding to independent tests. The most powerful design for estimating this effect is to study unrelated siblings raised in the same family (URT). When we examine this literature, we find an estimate of 0.26 in childhood (13 studies) and an estimate of 0.04 in adulthood (Bouchard, Reference Bouchard2009, Figure 5). We note here that Devlin et al. (Reference Devlin, Daniels and Roeder1997) found these values to be extremely heterogeneous and dropped them from their analysis. There are only five studies in adulthood; estimates from four cluster are very close to zero and one (a United States study with participants at about age 20 is 0.19). Three of the five studies are longitudinal and all show the decline characteristic of the twin studies in the figure. The childhood and adulthood data points are shown in Figure 2 as two asterisks connected with an arrow pointed to the later age. There are no twins in these studies. Thus, different methods (constructive replication) lead to the same conclusion and this conclusion does not appear to be driven by the assumptions underlying twin studies. A second way to estimate shared environmental influence is by using a comprehensive adoption sample, not just siblings. The Texas Adoption Project (TAP; Horn & Loehlin, Reference Horn and Loehlin2010) used 14 kinships drawn from about 300 families and estimated a shared environmental influence of 0.00 at age 17. As with the URT studies, there are no twins in this project. TAP also provides us with an estimate of heritability. The TAP values are shown in brackets [], 0.78 for heritability and 0.00 for shared environmental influences; these values are very consistent with the Dutch data at the same age.
There is another adoption study that allows us to estimate both heritability and shared environmental influence. It is a small sample study, but because it used Danish military data it is highly representative of the Danish male population (Teasdale & Owen, Reference Teasdale and Owen1984). The study made use of siblings reared apart, maternal and paternal half-siblings reared apart, unrelated reared-together, and full siblings reared together. Four of these five kinships constitute the entire population of such individuals rather than samples; the last group (full siblings reared together) is representative but not a population sample. The estimates are shown in pipes | | in Figure 2 and the values are 0.96 and 0.02. This study provides the highest heritability estimate ever reported and nearly the lowest estimate of shared environmental influence. It did not make use of twins. The unrelated reared together from this study are included in the adult URT sample shown with an asterisk.
Finally, we have estimates of heritability and shared environment from a sample of 65-year-old MZ and DZ twins reared apart and together from Sweden (Reynolds et al., Reference Reynolds, Finkel, McArdle, Gatz, Berg and Pedersen2005). The estimates are 0.91 and 0.00 and shown outside the chart. They are highly consistent with the Dutch data. We show the Reynolds et al. data because these come from the only study of older twins that included both twins reared together and twins reared apart. Lee et al. (Reference Lee, Henry, Trollor and Sachdev2010) have reviewed genetic influence on cognitive abilities in the elderly (over age 65). They concluded that ‘cross-sectional studies seem to suggest a decrease in heritability of g, from 80% at the mean age of 65 to 60% at the mean age of 82’ (p. 10). Figure 2 is consistent with a great deal of other data not included. Examples would be:
1. The Louisville Twin Study, a longitudinal study of twins reared together (Matheny, Reference Matheny, Hahn, Hewitt, Henderson and Benno1990; Wilson, Reference Wilson1983).
2. Other twin studies including one in Russia (Malykh et al., Reference Malykh, Iskoldsky and Gindina2005). For a recent review of twin studies, see Segal and Johnson (Reference Segal, Johnson and Kim2009) and for recent studies, see (Bratko et al., Reference Bratko, Butkovic and Chamorro-Premuzic2010; Davis et al., Reference Davis, Haworth and Plomin2009).
3. Very large studies that include both twin and singleton data (Calvin et al., Reference Calvin, Deary, Webbink, Smith, Fernandes, Lee and Visscher2012).
4. Virtual twin (unrelated reared-together of highly similar childhood ages) studies, both cross-sectionally and longitudinally (Segal et al., Reference Segal, McGuire and Stohs2012).
5. The Colorado Adoption Project (CAP), a longitudinal study of adoptive and biological families (Plomin et al., Reference Plomin, Fulker, Corley and DeFries1997).
6. Other contemporary adoption studies (Petrill & Deater-Deckard, Reference Petrill and Deater-Deckard2004; Plug & Vijverberg, Reference Plug and Vijverberg2003; Scarr, Reference Scarr, Sternberg and Grigorenko1997; Scarr & Weinberg, Reference Scarr and Weinberg1978) as well as much older ones (Burks, Reference Burks1928, Reference Burks1938; Leahy, Reference Leahy1935). For a detailed discussion of these and other earlier studies, see Bouchard (Reference Bouchard and Vernon1993), Bouchard et al. (Reference Bouchard, Lykken, Tellegen, McGue, Benbow and Lubinski1996), and Bouchard and Segal (Reference Bouchard, Segal and Wolman1985).
The observed changes cannot be explained by changes in brain size, as brain volume does not increase over the age period from 8 to 22 years of age (De Bellis et al., Reference De Bellis, Keshavan, Beers, Hall, Frustaci, Masalehdan and Boring2001; Giedd et al., Reference Giedd, Blumenthal, Jeffries, Castellanos, Liu, Zijdenbos and Rapoport1999), although the relative sizes of different parts do change. Cerebral hemisphere size decreases from age 20 to 80 (Raz et al., Reference Raz, Gunning-Dixon, Head, Rodrique, Williamson and Acker2004). Regarding changes in the brain with age and their association with IQ, see also (Karama et al., Reference Karama, Colom, Johnson, Deary, Haier, Waber and Evans2011; Lenroot et al., Reference Lenroot, Schmitt, Ordaz, Wallace, Neale, Lerch and Giedd2009). Brain volume is highly heritable even in older twins (Lessov-Schlaggar et al., Reference Lessov-Schlaggar, Hardin, DeCarli, Krasnow, Reed, Wolf and Carmelli2012).
What does Figure 2 tell us? It tells us three big things, all of which were intuitively implausible to many psychologists and consequently adamantly denied for many years (Gould, Reference Gould1981, Reference Gould1996; Kamin, Reference Kamin1974; Lewontin et al., Reference Lewontin, Rose and Kamin1984).
The first is that IQ is significantly heritable beginning at least at age 7. The second is that by age 10 genetic variance is larger than shared environmental variance. Third, the heritability increases with age until late adulthood when it reaches the mid-seventies and higher for some populations. Contrary to the widespread belief that ‘the slings and arrows of outrageous fortune’ accumulate over time (a version of the spun glass theory of mind; Schofield, Reference Schofield1986), the brain appears to be a robust and resilient mechanism.
The picture does change very late in life, but as one would expect attrition changes the characteristics of the sample — sample selection bias (Johnson et al., in press; Rabbitt & Lunn, Reference Rabbitt and Lunn2008). Nevertheless, heritability is still quite high (0.62) for 80-year-old twins when both individuals are free of major motor, sensory, or cognitive impairment (McClearn et al., Reference McClearn, Johanson, Berg, Pedersen, Ahern, Petrill and Plomin1997).
Currently, much emphasis is being placed on SES by heritability interactions (Nisbet et al., Reference Nisbet, Aronson, Blair, Dickens, Flynn, Halpern and Turkheimer2012). The results of the various studies are mixed, with some failure to replicate and some results in the opposite direction. It may be that the effect is real in childhood and young adulthood but fades in adulthood. As Nisbett et al. pointed out: ‘studies of adults must contend with the low magnitude of shared environmental components overall, and it may be difficult to detect interactions when there are low shared environment effects to work with’ (p. 3).
It may also be that different genes are at work in adulthood and the processes they mediate do not interact with SES (or whatever SES indicates). That is, the SES × heritability interaction may be transient. Wilson's (Reference Wilson1978) work demonstrating spurts and lags in mental development clearly suggests that the development of IQ is strongly influenced by an intrinsic genetic program that expresses itself differently at different ages. In addition, Moffitt et al. (Reference Moffitt, Caspi, Harkness and Silva1993), using a large longitudinal and representative sample (the ‘Dunedin Sample’ measured at ages, 7, 9, 11, and 13), have shown that ‘in a non-trivial minority of children, naturalistic IQ change is marked and real, but this change is variable in its timing, idiosyncratic in its source, and transient in its course’ (p. 455). They argue that while IQ can be thrown off its trajectory, IQ change ‘appears to conform to recovery curves and seems to reflect level-mainlining or even level-seeking phenomena. Intellectual performance that was reliably deflected across time was characterized by a self-righting tendency. IQ appeared to be elastic, rather than plastic’ (p. 496). To our mind, the most compelling finding in the Moffitt et al. study was something they did not find, namely a pattern of change that consisted of a constantly declining IQ. Such a slope would be predicted from the ‘spun glass theory of mind’ (Bouchard, Reference Bouchard, Saklofske and Zeidner1995). Moffitt et al. cite the work of Scarr and McCartney (Reference Scarr and McCartney1983) regarding how genotypes (embedded in individuals) create their own environments as the best theory to explain their results. Figure 2 demonstrates that shared environmental influence is also transient to a considerable extent. Shared environmental influence declines monotonically with age, dropping from about 0.55 at 5 years of age (Dutch data) to 0.10 in adulthood. It is noteworthy that all other estimates after 10 years of age are higher than the zero estimates from the Dutch data. These findings, of course, hold for what might be called the normal range of environments as exemplified by the ‘Dunedin Sample’.
The findings supporting the ‘Wilson Effect’ are highly robust:
1. They do not depend on a single design. Adoption studies with various combinations of kinships, not including twins, yield the same results as twin studies.
2. The findings are much the same across a variety of Western industrialized countries.
3. Many of the samples are quite comprehensive so their results probably apply broadly.
The fundament point, emphasized by Wilson, is that different genes are in play at different times and the manner in which they influence an organism's transactions with the environment also varies with time. This possibility is now recognized (Haworth et al., Reference Haworth, Wright, Luciano, Martin, de Geus, van Beijsterveldt and Plomin2010) but still not widely appreciated, though concrete evidence for it remains scanty. Increasing heritability over time is a quite general phenomenon, having been observed in many phenotypes in both human and non-human species. J. P. Scott (Reference Scott, Hahn, Hewitt, Henderson and Benno1990), who carried out classic work on the behavior genetics of behavior in dogs with John Fuller (Scott & Fuller, Reference Scott and Fuller1965), reported that:
We thought that the best time to study the effects of genetics would be soon after birth, when behavior still had little opportunity to be altered by experience. On the contrary, we found that the different dog breeds were most alike as newborns; that is genetic variation in behavior develops postnatally, in part as a result of the timing of gene action and in part from the interaction of gene action and experience, social, and otherwise. (p. vii)
More generally, a number of human behavioral phenotypes show age-related changes in heritability (Bergen et al., Reference Bergen, Gardner and Kendler2007).
An important limitation of the presentation in Table 2 is that the meaning of the findings can be interpreted in a number of ways as the variance components have been standardized. A more complete picture would flow from the presentation of unstandardized variances. Such an analysis would, however, require a common, well-developed intelligence test be used at each age. Such instruments do exist and have been shown to have the same factor structure from age 6 to 70 (Bickley et al., Reference Bickley, Keith and Wolfe1995). A second limitation and source of variance in the findings is the great variety of instruments, their varying quality, and the number of tests used to estimate IQ. Nevertheless, most of the studies did use reasonably well-developed instruments with a variety of subtests and we know that such batteries do a pretty good job of estimating IQ if not the g factors itself (Johnson et al., Reference Johnson, Nijenhuis and Bouchard2008).
Ronald Wilson ended a number of his papers with a clear statement emphasizing that his findings did not imply that environments are unimportant. He used a variation of the following quote in three important papers (Wilson, Reference Wilson1977, Reference Wilson1978, Reference Wilson1983) and it is still well worth reproducing.
The contribution of the parents, whether natural or adoptive, is in potentiating the child's inherent capabilities, in creating an atmosphere of enthusiasm for learning, and in adapting their expectations to the child's capability. The wide diversity within families emphasizes the importance of giving each child full opportunity for development, and indeed of making sure that the opportunity is taken. The ultimate goal is the maximum realization of each child's intelligence, coupled with a sense of satisfaction and personal accomplishment in its use. There is no better way to foster such development than by a supportive and appropriately stimulating family environment.
We conclude with our own less elegant commentary regarding how these findings should be understood and the role of the environment in the shaping of human psychological traits. It is important to specify the populations to which any results can be generalized and not misinterpret what they mean. The samples were drawn almost exclusively from Western industrial democracies. These settings have characteristic environments. Only a few of the participants were raised in real poverty or by illiterate parents, and all study participants had access to the contemporary educational programs typical of those societies. This is the domain to which we can generalize. The results do not mean that environments are irrelevant or unimportant. The proximate causes of variance in IQ are the ‘cognitively stimulating experiences’ that the individual is provided with and seeks for him/herself. Early in life, those experiences are primarily imposed (or not imposed) on the individual and that shows up as shared environmental influence. As the individual becomes more of an independent agent, the effective experiences are to a large extent self-selected. As Martin et al. (Reference Martin, Eaves, Heath, Jardine, Feingold and Eysenck1986) put it: ‘humans are exploring organisms whose innate abilities and predispositions help them select what is relevant and adaptive from the range of opportunities and stimuli presented in the environment. The effects of mobility and learning, therefore, augment rather than eradicate the effects of the genotype on behavior’ (p. 4368). This idea has been elaborated in some detail by Scarr (Reference Scarr1996) who has argued that people make their own environmentsFootnote 1 and Bouchard (Reference Bouchard1996, Reference Bouchard1997; Johnson, Reference Johnson2010a), who has elaborated the Experience Producing Drive Theory of Keith Hayes (Reference Hayes1962).
Acknowledgments
The author thanks Wendy Johnson for constructive comments on a previous version of the paper.