Background
Lifelong cardiovascular and neurodevelopmental risk may be partially set before birth. A low birth weight, preterm birth and prenatal malnutrition are associated with dyslipidemia, adiposity, type 2 diabetes, cardiovascular parameters and neurodevelopmental disorders (Barker, Reference Barker2006; Gluckman et al., Reference Gluckman, Hanson, Cooper and Thornburg2008; Lumey et al., Reference Lumey, Stein and Susser2011). The common denominator of these factors is that they represent an adverse intrauterine environment, resulting in greater long-term health risk.
Despite research dating back as early as the 1970s, the molecular mechanism underlying these findings remains to be resolved. Epigenetic mechanisms are widely perceived to be a strong candidate (Waterland & Michels, Reference Waterland and Michels2007). Epigenetic marks, such as DNA methylation, are primarily set during intrauterine development (Guo et al., Reference Guo, Zhu, Yan, Li, Hu, Lian and Qiao2014; Slieker et al., Reference Slieker, Roost, van Iperen, Suchiman, Tobi, Carlotti and Chuva de Sousa Lopes2015) and, in conjunction with transcription factors, control the regulation of gene expression. Experimental perturbation of the intrauterine environment in animal models can induce persistent epigenetic changes at the level of DNA methylation (Waterland & Jirtle, Reference Waterland and Jirtle2003). Studies in humans included those on the Dutch Hunger Winter, a severe famine at the end of World War II (Heijmans et al., Reference Heijmans, Tobi, Stein, Putter, Blauw, Susser and Lumey2008; Tobi et al., Reference Tobi, Lumey, Talens, Kremer, Putter, Stein and Heijmans2009). Individuals exposed to prenatal famine were found to have accumulated DNA methylation differences near genes involved in growth and development (Tobi et al., Reference Tobi, Goeman, Monajemi, Gu, Putter, Zhang and Heijmans2014). Specific DNA methylation differences were observed to mediate the association of prenatal famine and adult BMI and blood lipids (Tobi et al., Reference Tobi, Slieker, Luijk, Dekkers, Stein, Xu and Heijmans2018).
However, despite such promising findings, it can be argued that the field still has to deliver robust mechanistic insight and early-life epigenetic biomarkers of long-term health outcomes. This may be achieved by focusing on defined present-day populations experiencing severe intrauterine adversity. Study designs that enable strong inference, tissues other than peripheral blood, and longitudinal analyses starting before birth can be used to enable the testing of epigenetic mediation of prenatal adversity in later health.
Therefore, we established TwinLIFE, a prospective longitudinal investigation of fetal discordance within monochorionic (MC) twin pairs, who are monozygotic twins sharing a single placenta. MC twins can be considered a unique natural experiment as they in origin have the same genetic makeup and share many environmental factors, from the womb, age and sex to a range of maternal and paternal factors. Yet, they are frequently exposed to a vastly discordant prenatal environment due to complications that result from sharing a single placenta. In this study, we will follow the prenatal development of MC twins, store biological samples at birth and follow up the twins into childhood. Our aim is to evaluate whether DNA methylation differences in mesenchymal stromal cells (MSCs) isolated from cord (as a proxy for somawide epigenetic changes) mediate the impact of prenatal adversity ongrowth, cardiovascular and neurodevelopmental outcomes. Moreover, we will collect buccal swabs to evaluate whether the observed epigenetic changes in the MSCs are also represented in peripheral tissue. The results will be of immediate relevance to MC twins, but the impact may extend to the general population of singletons experiencing severe pregnancy complications such as fetal growth restriction. The model of MC twins may help unravel the pathophysiological mechanisms linking an adverse intrauterine environment to lifelong growth patterns, cardiovascular disease (CVD) and neurodevelopmental impairment (NDI) risk.
Hypothesis and Objectives
The overall hypothesis is that MC twin pairs who are discordant for intrauterine adversity display a distinct epigenetic signature in the MSCs at birth which, in turn, explain future interindividual variation in cardiac and vascular measures of CVD, neurodevelopmental outcomes, and growth patterns. We expect that testing this hypothesis will pinpoint the biological pathways that set long-term health risks when epigenetically changed during fetal development.
To test this hypothesis, the objectives of TwinLIFE are:
1. To establish a longitudinal MC twin cohort with prospectively recorded data on growth, cardiovascular and neurodevelopmental parameters measured pre- and perinatally and at 2, 5 and 8 years of follow-up to quantify the discordance in the prenatal environment and health outcomes.
2. To define epigenetic changes induced by an adverse intrauterine environment in the MSCs from umbilical cord by conducting a within-pair comparison of genomewide DNA methylation.
3. To evaluate the link between the observed epigenetic alterations and growth, cardiovascular, and neurodevelopmental health outcomes assessed at birth and 2, 5 and 8 years of follow-up.
Study Procedures
Establishing the MC Twin Cohort
The Leiden University Medical Center (LUMC) is the national referral center for complicated MC twin pregnancies in the Netherlands. The LUMC provides top-referent care that includes close monitoring of development and health of MC twins from early pregnancy to childhood. Therefore, a large proportion of clinical data available for TwinLIFE is routinely collected as part of standard care. In the LUMC, approximately 1 MC twin pair is born per week ensuring a steady enrollment of MC twins into TwinLIFE. We aim to enroll at least 100 MC twin pairs over a period of 2–3 years. Provided that we successfully acquire additional funding, we will increase our sample size beyond 100 pairs. Twins will be followed throughout childhood. This includes the time from inclusion (from week 14 of gestation to birth) to the completion of the follow-up assessments at 2, 5 and 8 years (Figure 1).
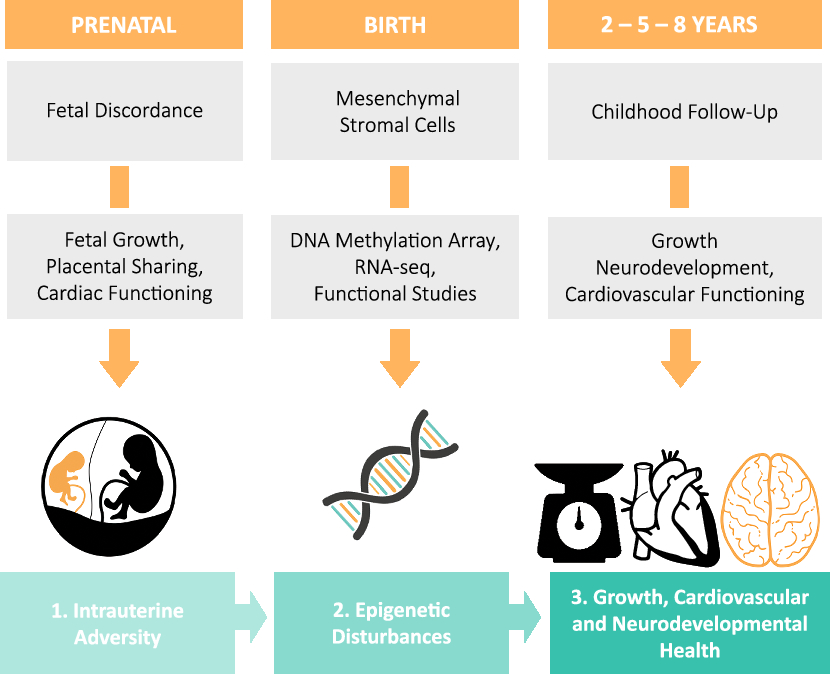
Fig. 1. An overview of the study design and the main procedures that subjects will undergo over the course of the study.
Monochorionicity is diagnosed with ultrasound in the first trimester of gestation, a procedure undergone by all pregnant women in the Netherlands. Women carrying an MC twin are referred to a hospital, either a general hospital or a university medical center, for close monitoring of the pregnancy which consists of an ultrasound every other week for the early detection of amniotic fluid discordance, differences in growth or other complications. If complications are detected, women are referred to a university medical center for specialist care. MC twin pregnancies are at risk of developing numerous complications, regularly in combination, among which selective fetal growth restriction (sFGR), twin–twin transfusion syndrome (TTTS) and twin anemia-polycythemia sequence (TAPS) represent the large majority. sFGR (occurring in up to 25% of MC twins) is characterized by unequal placenta sharing and fetal growth discordance as illustrated in Figure 2 (Inklaar et al., Reference Inklaar, van Klink, Stolk, van Zwet, Oepkes and Lopriore2014). TTTS (occurring in up to 10% of MC twins) results from severe intertwin blood flow imbalances and is diagnosed based on discordancy in amniotic fluid volume (twin oligo-polyhydramnios sequence) (Lewi et al., Reference Lewi, Jani, Blickstein, Huber, Gucciardo, Van Mieghem and Deprest2008). Lastly, TAPS (occurring up to 5% of MC twins) is caused by chronic blood flow imbalances through minuscule vascular anastomoses and diagnosed based on large hemoglobin discordance before or at birth (Slaghekke et al., Reference Slaghekke, Kist, Oepkes, Pasman, Middeldorp, Klumper and Lopriore2010).

Fig. 2. Selective fetal growth restriction: (a) an injected placenta demonstrating an unequal placental share division (79% vs. 21%) and a laser demarcation line following fetoscopic laser surgery for coexistent TTTS; (b) an MC twin pair affected by selective fetal growth restriction treated at the LUMC (gestational age at birth 32 weeks, birth weights 2300 and 1200 g). The larger placental share (a, left) belonged to the larger co-twin (b, left).
Written informed consent from both parents (≥18 years and able to consent) is a prerequisite before entering TwinLIFE. The following cases will be excluded from the study: twins with major anatomical abnormalities (interference with outcomes), triplet or higher order multiple pregnancies (different population, cannot be compared to MC twins), cases with Twin Reversed Arterial Perfusion (TRAP) sequence (acardiac twin) and/or cases with single or double fetal demise (no intertwin comparison possible after birth).
We will conduct a comprehensive series of pre- and perinatal assessments (Tables 1 and 2), which consist of a series of assessments part of standard care supplemented with a fetal cardiac functioning measurement, a postnatal cardiac ultrasound and questionnaires specific for the study. Prenatally, fetal growth and cardiac functioning will be recorded using detailed ultrasound scans every 2 weeks or more frequently on clinical indication. Furthermore, maternal and paternal characteristics will be recorded prenatally using a questionnaire, which collects information on demographics, medication and nutritional supplement use, intoxications and medical history. Also, parental wellbeing will be assessed using the Brief Symptom Inventory (BSI) (Lang, Reference Lang2003) for quantification of somatization, depression and anxiety. After birth, we will assess anthropometric measurements (birth weight, length in supine position and head circumference), brain and cardiac development. Placental characteristics will be recorded after color dye injection by trained staff according to our routine clinical program for placenta analysis in MC twins for in-depth analysis of the placental angioarchitecture (Lopriore et al., Reference Lopriore, Slaghekke, Middeldorp, Klumper, van Lith, Walther and Oepkes2011). Common neonatal morbidities will be recorded from birth and admission to the neonatal intensive care unit until discharge to home by the treating neonatologist. At 6 months (corrected for prematurity), parental wellbeing will be reevaluated using the BSI and child nutrition will be recorded using a standardized questionnaire.
Table 1. Timing and goal of assessments of TwinLIFE
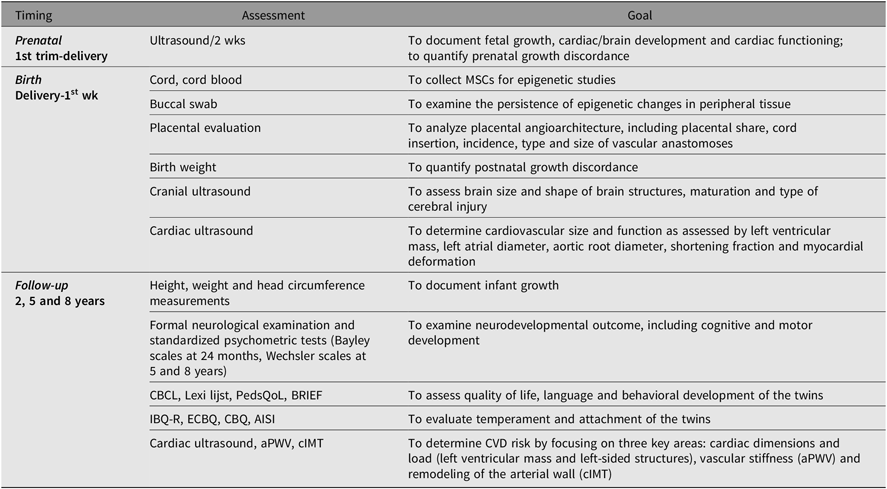
Note: trim = trimester, wks = weeks, MSCs = mesenchymal stromal cells, CBCL = Child Behavior Checklist, PedsQoL = Pediatric Quality of Life Inventory, BRIEF = Behavior Rating Inventory of Executive Functioning, Infant Behavior Questionnaire, ECBQ = Early Childhood Behavior Questionnaire, CBQ = Children’s Behavior Questionnaire, AISI = Attachment Insecurity Screening Inventory, aPWV = aortic pulse wave velocity, cIMT = carotid intima–media thickness, CVD = cardiovascular disease.
Defining Epigenetic Changes at Birth
At birth, we will collect umbilical cord tissue, cord blood and buccal swabs for the TwinLIFE Biobank. Umbilical cords will be collected in a sterile PBS-antibiotic/antimycotic solution on birth as a source of MSCs. MSCs are cells of mesodermal origin with the capacity to differentiate into multiple cell types including osteoblasts, adipocytes and chondroblasts using specific culture conditions (Swamynathan et al., Reference Swamynathan, Venugopal, Kannan, Thej, Kolkundar, Bhagwat and Balasubramanian2014; van der Garde et al., Reference van der Garde, van Pel, Millan Rivero, de Graaf-Dijkstra, Slot, Kleinveld and Zwaginga2015). MSCs can be obtained from umbilical cord without any risk for the donor and expanded to high numbers for experiments. For MSC isolation, a modified explant method operational at the LUMC will be adapted and standardized (van der Garde et al., Reference van der Garde, van Pel, Millan Rivero, de Graaf-Dijkstra, Slot, Kleinveld and Zwaginga2015). After expansion for approximately 10 days, the explants are removed and the MSCs are transferred into culture flasks. The transferred cells will be grown to >70% confluence, frozen and stored until further expansion is required. Subsequent MSC expansions of MSCs obtained from co-twins will be performed simultaneously to minimize batch effects. The uniform classification system for MSCs, introduced by the International Society of Cellular Therapy, will be adopted to ensure unambiguous identification and separation of MSCs (Dominici et al., Reference Dominici, Le Blanc, Mueller, Slaper-Cortenbach, Marini, Krause and Horwitz2006). The number of cells after expansion will be sufficient for the epigenetic profiling, RNA expression analysis, cellular phenotyping, and functional differentiation studies.
Cord blood will be collected in sterile blood collection tubes, containing a citrate phosphate dextrose adenine (CPDA) solution. Subsequently, mononuclear cells and plasma will be isolated using a Ficoll density gradient. The isolated cells and plasma will be stored for further experiments, such as DNA methylation analysis of specific cells types. Buccal swabs will be collected, postnatally for DNA extraction and DNA methylation analysis.
We will perform genomewide DNA methylation profiling of MSCs from the twins, using the Illumina EPIC methylation array (Zhou et al., Reference Zhou, Laird and Shen2017). The platform provides a cost-effective coverage of ~850,000 potentially methylated CG dinucleotides across the genome and is enriched for regions known to be involved in genomic regulation. The EPIC array is the successor of the 450k array that has been widely used to successfully identify epigenetic changes after adverse prenatal exposures, including by ourselves in the context of the Dutch Hunger Winter (Tobi et al., Reference Tobi, Slieker, Stein, Suchiman, Slagboom, van Zwet and Lumey2015). We will use our established work flow for quality control, normalization and analysis methylation array data, which is publicly available (https://github.com/molepi/DNAmArray) and combines our own BioConductor packages with the best practices in the field (van Iterson et al., Reference van Iterson, Tobi, Slieker, den Hollander, Luijk, Slagboom and Heijmans2014, Reference van Iterson, van Zwet, Consortium and Heijmans2017, Reference van Iterson, Cats, Hop, Consortium and Heijmans2018). Co-twins will be measured on the same array and array row and will be randomized with respect to array column.
Uncovering Epigenetic Influences on Growth, CVD and NDI Risk
To study the associations of epigenetic changes at birth with precursors of CVD and NDI risk in childhood, we will initiate a follow-up examination of MC twins at least 2 years of age aimed at a focused growth, cardiac, vascular and neurodevelopmental characterization. Parents and their MC twins will be invited to the LUMC for follow-up examinations at the age of 2, 5 and 8 years (at term equivalent age). Transthoracic echocardiography will be used to assess differences in structural and functional cardiac measures (Toemen et al., Reference Toemen, Gaillard, van Osch-Gevers, Helbing, Hofman and Jaddoe2017). Furthermore, aortic pulse-wave velocity (PWV) reflecting vascular stiffness will be assessed using aortic length regression equation based on the subject’s height and two pulsed Doppler recordings of the aorta (Jo et al., Reference Jo, Lande, Meagher, Wang and Vermilion2010). Measurement of the intima–media thickness (IMT) of the carotid artery, demonstrating the remodeling of the arterial wall, is feasible in small children and will be measured with ultrasonography with a linear array probe of 7.5 MHz (Dilli et al., Reference Dilli, Ozkan, Ozkan, Aydin, Ozyazici, Fettah and Okumus2017).
Long-term follow-up of complicated MC twin pregnancies is part of our standard clinical care at the LUMC and includes a formal neurological examination (Touwen et al., Reference Touwen, Hempel and Westra1992), measurement of relevant growth parameters, measurement of blood pressure and an assessment of cognitive and motor development using standardized psychometric tests (van Klink et al., Reference van Klink, Koopman, Middeldorp, Klumper, Rijken, Oepkes and Lopriore2015, Reference van Klink, Slaghekke, Balestriero, Scelsa, Introvini, Rustico and Lopriore2016). At the (term equivalent) age of 24 months, cognitive and motor development will be assessed using Bayley scales (Bayley, Reference Bayley2006). At 5 and 8 years of follow-up, cognitive development will be assessed using Wechsler scales (Wechsler, Reference Wechsler2002, Reference Wechsler2014). During the visit, we will also collect anthropometric data through our national system of primary care physicians who monitor growth in every Dutch child throughout the first 2 years of life.
To assess quality of life, language and behavioral development of the twins, online questionnaires will be filled in by the parents using a web-based application (Baron, Reference Baron2000; Varni et al., Reference Varni, Seid and Kurtin2001). These questionnaires are part of our standard clinical care. In addition, we will evaluate temperament and attachment using validated questionnaires (Putnam et al., Reference Putnam, Gartstein and Rothbart2006; Spruit et al., Reference Spruit, Wissink, Noom, Colonnesi, Polderman, Willems and Stams2018) (Table 2).
Table 2. Overview of evaluations and measurements within TwinLIFE
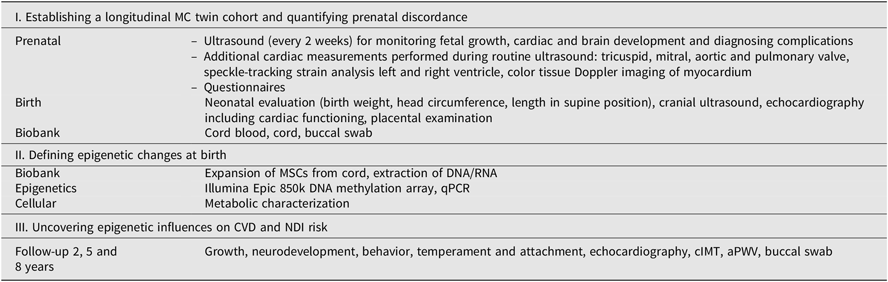
Note: MC = monochorionic, MSCs = mesenchymal stromal cells, qPCR = quantitative polymerase chain reaction, CVD = cardiovascular disease, NDI = neurodevelopmental impairment, cIMT = carotid intima–media thickness, aPWV = aortic pulse-wave velocity
Future Plans
As of January 2019, we have started the inclusion of twin pairs into TwinLIFE. We aim to create a dynamic cohort, where we continuously include new MC twins to increase the sample size beyond 100 pairs and also extend the follow-up beyond 8 years to further deepen our understanding of the effect of an adverse intrauterine environment on growth, CVD and NDI risk at a later ages as mediated by epigenetic alterations. We intend to broaden the scope of our research by adding other disease areas (e.g. lung disease) and analyzing other cell types than cord MSCs (e.g. immune cells and endothelial progenitor cells (EPCs)). Finally, we are committed to collaborate with other MSC-focused cohorts, twin registries and birth cohorts.
Acknowledgments
We wish to thank the mothers and fathers for participating in TwinLIFE with their newborn twins.
Financial support
TwinLIFE is funded by an Established Investigator grant from the Dutch Heart Foundation (2017T075). The funders had no role in study design, data collection, analysis, decision to publish or preparation of the manuscript.
Conflict of interest
None.
Ethical standards
The authors assert that all procedures contributing to this work comply with the ethical standards of the relevant national and institutional committees on human experimentation and with the Helsinki Declaration of 1975, as revised in 2008.