Introduction
Dust-like seeds represent a derived state that has evolved separately in at least 12 families, including Burmanniaceae, Gesneriaceae Orobanchaceae and Orchidaceae (Eriksson and Kainulainen, Reference Eriksson and Kainulainen2011). Purportedly, a reverse evolutionary trajectory resulted in dwarf seeds of these families undergoing endosperm reduction and the production of an undifferentiated embryo. One ecological consequence of these physical changes to orchid seeds is a trade-off between plant competition and colonization. Miniaturization of the seed increased hugely the potential seed yield per plant. For example, about 2000 seeds per capsule in the temperate species Dactylorrhiza fuchsia (Druce) Soó (Marks et al., Reference Marks, Seaton and Pritchard2014) and about 4 million seeds in capsules of the tropical epiphyte Cycnoches ventricosum Bateman (Arditti and Ghani, Reference Arditti and Ghani2000). Such seed yields are only possible as seed weights are reduced to micrograms; for example, 1.9 and 3.6 μg for D. fuchsii and C. ventricosum, respectively (Arditti and Ghani, Reference Arditti and Ghani2000; Marks et al., Reference Marks, Seaton and Pritchard2014). In addition to being flimsy, a lack of endosperm has resulted in the seeds of many species having an air space between the embryo and the testa. There is considerable inter-species variation in this balloon-like space as a proportion of seed volume, for example, 92% air space in Epipactis palustris (L.) Crantz (Arditti and Ghani, Reference Arditti and Ghani2000), and 13–36% (Prasongsom et al., Reference Prasongsom, Thammasiri, Pritchard and Thammasiri2017) and 32–92% (Diantina et al., Reference Diantina, McGill, Millner, Nadarajan, Pritchard and McCormick2020a) among a range of Dendrobium species. Geographical differences (tropical vs temperate) and life traits (epiphyte vs terrestrial) are critical factors affecting seed traits (Arditti and Ghani, Reference Arditti and Ghani2000; Diantina et al., Reference Diantina, Kartikaningrum, McCormick, Millner, McGill, Pritchard and Nadarajan2020b). The lighter (less mass) and more buoyant (more air space) the seed is the greater the potential dispersal distance from metres to kilometres. Thus, miniaturization of the seed affects the likelihood of the progeny reaching favourable environments for germination and thus colonization (Mitra, Reference Mitra1971; Arditti and Ghani, Reference Arditti and Ghani2000; Hashimoto et al., Reference Hashimoto, Fukukawa, Kunishi, Suga, Richard, Sauve and Selosse2012).
Orchid seeds with a very small embryo and no endosperm have a limited internal energy supply. Consequently, in the natural environment, the seed embryo may imbibe but fail to develop further unless an exogenous supply of carbohydrate is provided or mycorrhizal association occurs (Smith and Read, Reference Smith and Read1997; Chugh et al., Reference Chugh, Guha and Rao2009). In the laboratory, orchid seed germination is possible in vitro on a medium that varies in complexity depending on the species but generally includes macro- and micro-elements and a source of carbon (Arditti et al., Reference Arditti, Clements, Fast, Hadley, Nishimura, Ernst and Arditti1982; Deb and Pongener, Reference Deb and Pongener2011). Norstog medium, with amino acids, may be effective with epiphytic species, while Knudson C, with activated charcoal, can support the germination of tropical terrestrial species (Nadarajan et al., Reference Nadarajan, Woods, Marks, Seaton and Pritchard2011). Alternatively, the seed can be sown on a simple oat-yeast medium in the presence of a symbiotic fungus (Muir, Reference Muir and Pritchard1989), such symbiosis reflecting so-called mycovitalism in nature (Vujanovic and Vujanovic, Reference Vujanovic and Vujanovic2007). These means of germinating orchid seeds are relatively complicated compared to the sowing of seeds of other species on an inert, moistened substrate (e.g. filter paper, sand, agar) to which stimulants can be added, if appropriate (Baskin and Baskin, Reference Baskin and Baskin2014).
An understanding of the type of stimulant required for seed germination (e.g. nitrate, gibberellic acid), the combinations of temperatures needed (warm, cold, warm followed by cold, etc.) and insights on seed morphology (especially embryo size and form) have all contributed to the development of a classification system for seed dormancy that has been applied to thousands of species (Baskin and Baskin, Reference Baskin and Baskin2004, Reference Baskin and Baskin2021). This system identifies key classes of seed dormancy: physiological (PD), morphological (MD), morphophysiological (MPD), physical (PY) and combinational (PY + PD). Because of their dust-like form, undifferentiated embryo and other features, orchids are proposed to have a special kind of MD or MPD (Baskin and Baskin, Reference Baskin and Baskin2004, Reference Baskin and Baskin2014), and most recently, the ‘dust seeds’ of mycoheterotrophs, including orchids, have been placed within the seven subclasses of MD (Baskin and Baskin, Reference Baskin and Baskin2021). Of the relatively few orchid species studied for seed dormancy and germination out of the >25,000 species in the family, the most detailed studies have been undertaken with temperate species. One of the main pieces of evidence for seed dormancy in orchids is less germination in mature compared with immature seeds (Rasmussen, Reference Rasmussen1995). For example, Phaius tankervilleae (Banks) Blume seed maturation was accompanied by a decrease in water content and a concomitant increase in ABA content and PtNCED1 mRNA level along with a marked decrease in germination percentage (Lee et al., Reference Lee, Chan, Lin, Chung and Leu2018). Other evidences for orchid seed dormancy include a delay of several months before embryo growth occurs; restriction of growth by the testa; a requirement for cold stratification or after-ripening and responsiveness to gibberellic acid (Baskin and Baskin, Reference Baskin and Baskin2014). While physical dormancy is not a general feature of orchid seeds (Baskin and Baskin, Reference Baskin and Baskin2014), ultra-low temperature treatment of dry seeds can improve germination in Anacamptis morio (L.) R.M.Bateman, Pridgeon & M.W.Chase (Pritchard, Reference Pritchard1984) and laccase enzyme scarification facilitates lignin degradation, water uptake and germination in the same species (Pierce et al., Reference Pierce, Spada, Caporali, Ceriani and Buffa2018). Surface disinfection with bleach can also partially scarify orchid seeds (Magrini and De Vitis, Reference Magrini and De Vitis2017; Pierce et al., Reference Pierce, Spada, Caporali, Ceriani and Buffa2018) and facilitate the permeation of the vital stain triphenyl tetrazolium chloride (Custódio et al., Reference Custódio, Marks, Pritchard, Hosomi and Machado-Neto2016).
Disinfection with bleach is applied as a standard, and necessary, treatment for orchid seeds from mature capsules prior to sowing on in vitro medium, primarily as a means of ensuring clean cultures. However, the application of pro-oxidants to seeds may also affect a physiological block to germination. For example, germination of tea seeds increased with H2O2 treatment (Chen et al., Reference Chen, Pritchard, Seal, Nadarajan, Li, Yang and Kranner2012) and exposure of mature seeds of the orchid P. tankervilleae to NaOCl solution lowers ABA content and improves seed germination (Lee et al., Reference Lee, Chan, Lin, Chung and Leu2018). In addition, nutrient media for orchid seed germination incorporate relatively high levels of nitrate, for example, ammonium nitrate and potassium nitrate in Murashige and Skoog medium, although rarely have the potential dose effects of nitrate on orchid seed germination been explored (Ponert et al., Reference Ponert, Figura, Vosolsobe, Lipavska, Vohnik and Jersakova2013). This is important, as nitrate is known to facilitate both endosperm and testa rupture in Sisymbrium officinale (L.) Scop. seeds (Toorop, Reference Toorop2015), and to act as a signal, in a wide range of species, to stimulate seed germination, particularly in association with light and alternating temperatures (Probert et al., Reference Probert, Gajjar and Haslam1987; Baskin and Baskin, Reference Baskin and Baskin2014; Toorop, Reference Toorop2015; Duermeyer et al., Reference Duermeyer, Khhodapanahi, Yan, Krapp, Rothstein and Nambara2018). On occasions, Dendrobium species seed has been successfully germinated on nutrient-poor medium containing oat meal agar (OMA), usually in the presence of potato dextrose agar (PDA), following disinfection of the capsule or seed directly and conditions of light (16 h d−1) following dark (Swangmaneecharern et al., Reference Swangmaneecharern, Serivichyaswat and Nontachaiyapoom2012; Mala et al., Reference Mala, Kuegkong, Sa-ngiaemsri and Nontachaiyapoom2017). As seeds of terrestrial and epiphytic species tend to prefer dark and light conditions, respectively, for germination (Rasmussen, Reference Rasmussen1995), determining the nature of seed dormancy in orchids requires an assessment of the effects of a range of factors (including disinfection, nitrate, and light and temperature alternation) on the germination response.
Both the evolution of seed dormancy (Nikolaeva, Reference Nikolaeva2004; Willis et al., Reference Willis, Baskin, Baskin, Auld, Venable, Cavender-Bares, Donohue and de Casas2014) and seed embryos (Finch-Savage and Leubner-Metzger, Reference Finch-Savage and Leubner-Metzger2006) have received considerable attention, indicating key evolutional transitions between dormancy classes, including the class ‘DUST’ seeds. DUST seeds may have arisen from MPD seeds but not from MD seeds, and the non-dormant (ND) state primarily evolved from PD but rarely from MPD (Willis et al., Reference Willis, Baskin, Baskin, Auld, Venable, Cavender-Bares, Donohue and de Casas2014). The evolution of PD was also associated with increased speciation rates. Thus, physiologically regulated environmental cueing of dormancy appears to have influenced major evolutionary patterns in the seed plants (Willis et al., Reference Willis, Baskin, Baskin, Auld, Venable, Cavender-Bares, Donohue and de Casas2014).
The genus Dendrobium in the Orchidaceae has undergone one of the greatest levels of speciation in the plant kingdom. Around 800–1500 species, which are mainly epiphytic (occasionally lithophytic), have considerable morphological diversity and occur across a wide range of microenvironments (Xiang et al., Reference Xiang, Schuiteman, Li, Huang, Chung, Li, Zhou, Jin, Lai, Li and Jin2013). Thus, this genus could be an excellent model in which to consider the evolution of dormancy in orchids (Prasongsom et al., Reference Prasongsom, Thammasiri, Pritchard and Thammasiri2017). Seeds of some Dendrobium species can germinate ex vitro on plain agar-water in the absence of soluble sugars, with the enlarging embryo turning green, as in several other tropical orchids, forming a spherical protocorm and breaking through the testa. Thus, spherical protocorm development ex vitro, the first stage of germination in orchids, has been reported for nine species of the genus: D. chrysotoxum, D. draconis, D. fimbriatum, D. findlayanum, D. hercoglossum, D. lituiflorum, D. parishii, D. pulchellum and D. schildhaueri (synonym of D. kontumense) (Prasongsom et al., Reference Prasongsom, Thammasiri, Pritchard and Thammasiri2017). In these species, the air space is relatively small from 13 to 35% of seed volume, suggesting that variation in embryo size might contribute to germination performance in DUST seeds of orchids. However, micromorphometric studies on orchid seeds are often limited to the external dimensions and appearance of the seeds in relation to taxonomy. In contrast, far less attention has been given to seed micromorphometry and germination in orchids (Arditti and Ghani, Reference Arditti and Ghani2000).
The objective of this study is to explore the possible presence and type of dormancy in Dendrobium cruentum Rchb. f. seeds. This epiphytic orchid originates from southwest Thailand, growing on small trees in open forests at low elevations, and flowers all year (Vaddhanaphuti, Reference Vaddhanaphuti2005). It is facing extinction due to climate change and loss of natural habitats and is listed in Appendix I of CITES. We assessed the effects of various factors (disinfection, nitrate, light/dark, constant and alternating temperatures) on the dormancy release or germination response and characterized embryo growth both in vitro and ex vitro. Finally, we consider for fresh and stored seed whether the DUST seeds of some orchids can be non-dormant.
Materials and methods
Plant and seed materials
Wild plants of D. cruentum were collected from their natural environments and then cultivated in a greenhouse (Fig. 1A) at the Institute of Science and Technology for Research and Development, Salaya Campus, Mahidol University, Thailand. Flowers (Fig. 1B) were hand pollinated and mature, just dehiscing capsules harvested (Fig. 1C). Seeds were extracted from single capsules (containing many thousands of seeds) maturing in two different years. Seed lot 1 was held in the laboratory briefly (approximately 80% relative humidity, RH) until dispatch to the UK; thereafter, the seeds were dried for about 3 weeks in a room operating at 15% RH and 15°C and then stored in vials at −20°C for 7 months until experimentation. Seed lot 2 was not subjected to artificial drying, with the freshly isolated seeds committed to experimentation soon after extraction. The collected capsule was cleaned under running tap water followed by dipping briefly in 95% alcohol. The seeds were removed from the capsule and used immediately in the experimentation to reduce any likelihood of after-ripening. During this handling procedure, the seeds may have equilibrated to ambient conditions (approximately 80% RH).
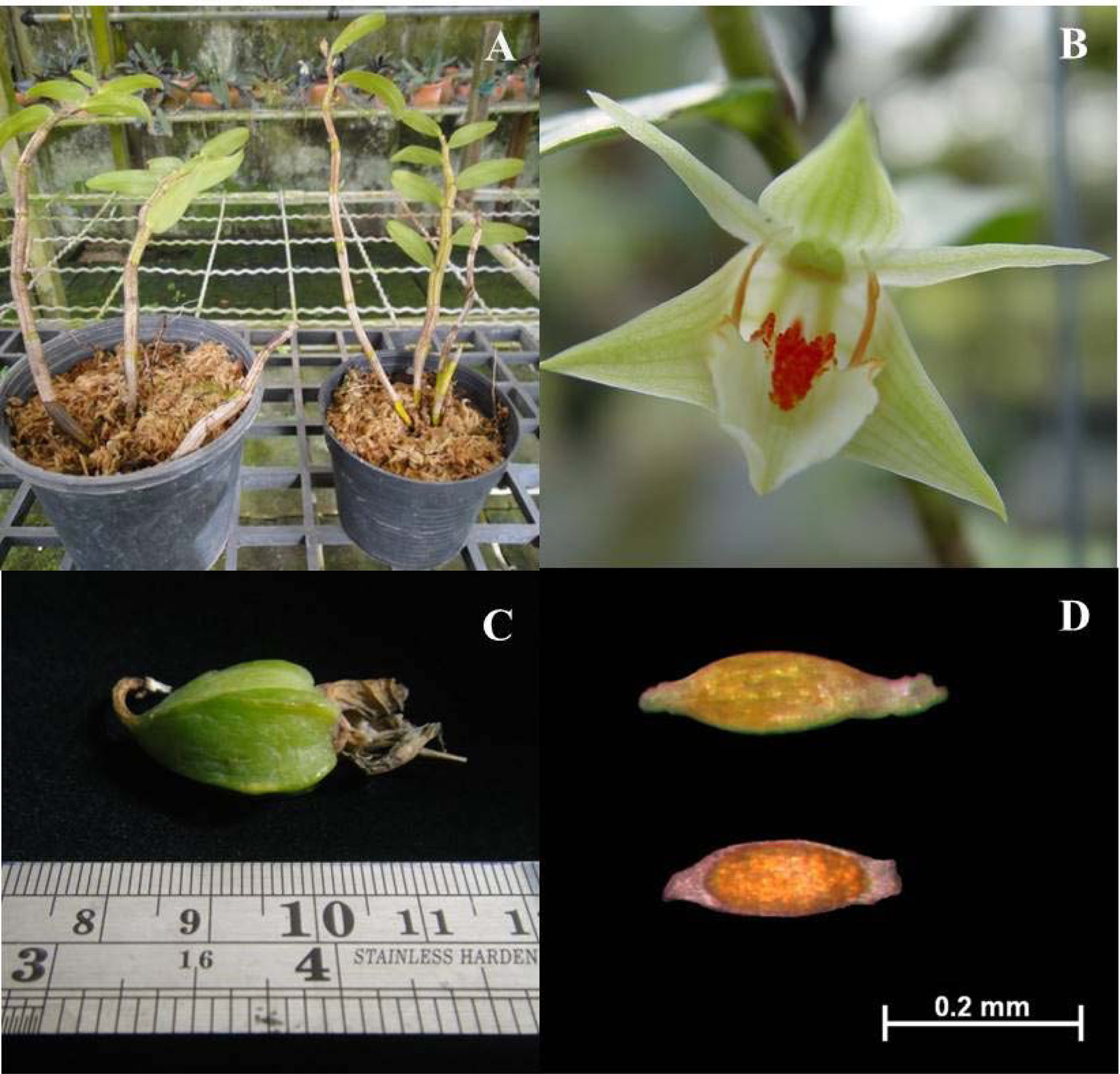
Fig. 1. Plant, fruit and seed characteristics of D. cruentum. (A) Plants cultivated in the green house. (B) Flowering stage. (C) Maturing capsule. (D) Dry-stored seeds (lot 1) before (upper) and after vital staining with tetrazolium chloride, showing orange-red stained embryo (lower).
An initial seed viability of the seed lots was assessed using a 1% (w/v) solution of 2,3,5-triphenyl tetrazolium chloride (TTC) in phosphate buffer (Custódio et al., Reference Custódio, Marks, Pritchard, Hosomi and Machado-Neto2016). Seeds were added to 20 ml of TTC solution in a screw cap tube and kept in the dark at 30°C for 24 h. While viable embryos appeared to turn yellow-red from yellowish, as judged under a light microscope (Fig. 1D), the colour change was not always strong.
Micromorphology analyses
The seeds were placed on stubs using double adhesive tape and coated with gold palladium alloy for 5 min. Thereafter, the material was examined in a Scanning Electron Microscope (EDS model 6610LV), and the macromorphology of the seed coat (testa cell) surface was photographed.
The micromorphology of the seed was examined at the start of, and during, germination (i.e. emergence of the spherical protocorm through the testa). For each determination, 30 seeds and embryos were measured under a light microscope using AxioVision software (Carl Zeiss: AxioCam). The descriptors included seed and embryo length and width, seed shape and colour, and embryo/protocorm colour. The percentage air space in ungerminated seed was calculated from determinations of the seed and embryo volumes using the following two equations (adapted from Arditti et al. (Reference Arditti, Michaud and Healey1980)):

where EL is embryo length and EW is embryo width.

where SL is seed length and SW is seed width and 1.047 = π/3.
Seed sowing
For ex vitro germination, fresh (seed lot 2) and dry-stored seeds (lot 1) were sown without disinfection on non-sterile agar-water. For in vitro (sterile culture) germination, fresh and dry-stored seeds were placed in filter paper packets and disinfected for 20 min with 1% sodium hypochlorite solution, followed by washing four times in sterile distilled water for 5 min each. Disinfected seeds were transferred to sterile, half-strength Murashige and Skoog (MS) medium (Murashige and Skoog, Reference Murashige and Skoog1962) in Petri dishes. Both ex vitro and in vitro media contained 1% agar (w/v).
Light and temperature treatments
The effect of constant temperature in the light (12 h d−1 light; white fluorescent tubes at approximately 15–20 μmol m−2 s−1) on seed germination was assessed in eight environmental chambers from 5 to 40°C at 5°C intervals. Fresh and dry-stored seeds were sown ex vitro and in vitro, as described above.
Fresh and dry-stored seeds were also sown ex vitro and germinated at constant 25°C and alternating 30/20°C under different light conditions. Seeds were incubated in the light (12 h photoperiod; applied during the warm temperature phase for the alternating treatment), in dark (aluminium foil wrapped, but opened weekly, and briefly, for germination scoring) and double dark (only unwrapped at the end of the test).
In all instances, three replicates of 100–150 full seeds were used per treatment and germination was assessed, as spherical protocorm production, after 28 d.
Nitrate and light treatments
Fresh and dry-stored seeds were sown ex vitro on plain agar with different concentrations (0, 1, 3, 10 and 30 mmol) of the dormancy-breaking chemical KNO3. Seeds were incubated in the light and dark, as described above, at 25°C, the optimum temperature for germination. Three replicates of 100–150 full seeds per nitrate concentration were assessed for germination (spherical protocorm formation) after 28 d.
Statistical analysis
Changes in mean embryo volume (n = 30 seeds) during the germination process were subjected to a t-test (P ≤ 0.05). For the germination experiments, data were arc-sine transformed to normalize the distribution and subjected to ANOVA and all pairs of means compared using Duncan's Multiple Range Test (SPSS Statistic 21 software).
Results
D. cruentum plants produced capsules around 2 cm long (Fig. 1). The seeds were fusiform in shape with a relatively large central embryo (Fig. 1). The micropylar and the chalazal cells of the testa appeared shorter than the medial cells, and the raised testa cell walls had frilled edges (Fig. 2), described by Chaudhary et al. (Reference Chaudhary, Chattopadhyay and Banerjee2014), as ‘cottony-white substance’. The seeds were extremely small, measuring around 0.285 mm long and 0.060 mm wide (n = 30), with some variations in dimensions between seed lots. In particular, fresh seeds (lot 2) were slightly, but significantly (P < 0.05) narrower, and had estimated seed and embryo volumes about 15% less than dry-stored seeds (lot 1) (Table 1). The embryo dimension varied from 162 to 172 μm long and 48 to 54 μm wide (Table 1). Based on the seed:embryo volume ratios, the air space did not vary between seed lots (P > 0.05), being around 15–17%; meaning that the embryo occupied about 83–85% of the seed volume (Table 1). The frequency distribution for seed air space across 30 Dendrobium species, including D. cruentum (this study), indicated that two-thirds had embryo volumes that were >60% of the seed volume (Fig. 3).
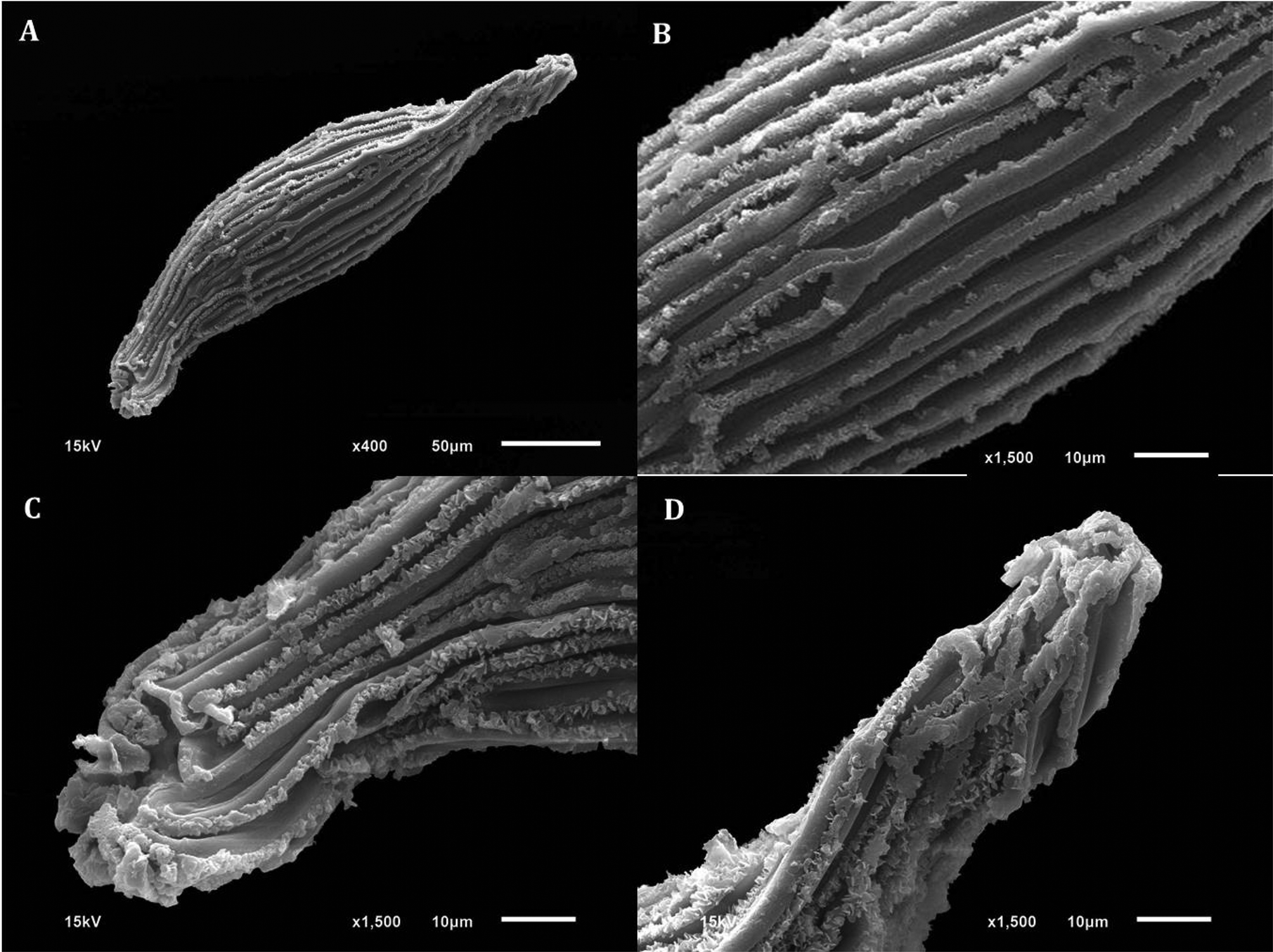
Fig. 2. Scanning electron microscopy (SEM) of D. cruentum seeds. (A) Whole seed. (B) Elongated testa cells with thickened walls. (C) Close-up of micropyle opening. (D) Close-up of chalazal end and cell wall thickenings with frilled edges.

Fig. 3. Frequency distribution for seed air space in 30 Dendrobium species. Species names follow the original publications and are thought to be synonyms for three species based on plants of the World Online (https://powo.science.kew.org/). Species: D. chrysanthum, D. chrysotoxum, D. clavatum (synonym of D. denneanum), D. crepidatum, D. cruentum, D. cunninghamii, D. densiflorum, D. devonianum, D. draconis, D. farmeri, D. fimbriatum, D. findlayanum, D. formosum, D. hercoglossum, D. heterocarpum, D. hookerianum, D. insigne, D. lineale, D. lituiflorum, D. moschatum, D. nobile, D. ochriatum, D. parishii, D. primulinum (synonym of D. polyanthum), D. pulchellum, D. schildhaueri (synonym of D. kontumense), D. strebloceras, D. transparens, D. wardianum, D. williamsonii. Information sources: Arditti and Ghani (Reference Arditti and Ghani2000); Chaudhary et al. (Reference Chaudhary, Chattopadhyay and Banerjee2014); Prasongsom et al. (Reference Prasongsom, Thammasiri, Pritchard and Thammasiri2017); Diantina et al. (Reference Diantina, McGill, Millner, Nadarajan, Pritchard and McCormick2020a) and Tongbram et al. (Reference Tongbram, Nageshwara Rao and Vij2012).
Table 1. Micromorphometric data of the seed and embryo (n = 30) of D. cruentum

Data for fresh and stored seeds are significantly different (P < 0.05) when followed by different letters.
Seed embryos were orange-yellow in colour, and viable embryos were turned orange-red when exposed to tetrazolium chloride (Fig. 1). When seeds were incubated ex vitro (i.e. without disinfection and when sown on plain agar), the embryos doubled in volume in the first 7 d. This applied to both seed embryos that were alive and turning green or dead and turning brown (Fig. 4; Table 2). For germination to the spherical protocorm stage, there was a sevenfold increase in embryo volume by 28 d. In contrast, seeds disinfected and sown in vitro, in the presence of macroelements and sucrose, achieved a 28-fold increase in embryo volume by 28 d and formed a top-shaped protocorm (Fig. 4; Table 2). The volume increases through each stage of germination (imbibition, spherical and top-shaped protocorm formation) were significantly different (P < 0.05).

Fig. 4. Stages of D. cruentum Rchb. f. seed embryo growth ex vitro (a–e) and in vitro (f). (a) Embryoless seed. (b) Full seed, with the embryo occupying about 87% of the seed volume. (c) Seed turning green after 7 d imbibition at the start of embryo growth. (d) Full, dead seed, in which the embryo failed to achieve germination and shows signs of oxidative stress (browning). (e) Spherical embryos (protocorm) of germinating seeds after 28 d ex vitro. (f) Top-shaped protocorm formation from germination seeds after 28 d in vitro.
Table 2. Changes in embryo dimensions of D. cruentum dry-stored seed (lot 1) during germination ex vitro and in vitro
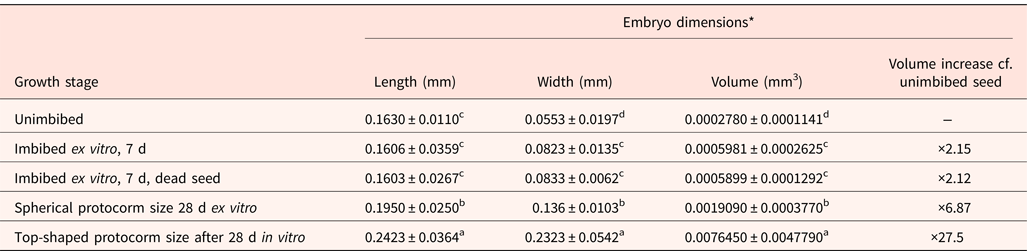
* 30 embryos assessed per growth stage. Mean values within columns followed by different letters are significantly different (P < 0.05, t-test).
In the light, temperature (F = 54.8; P < 0.001) had a significant effect on germination (spherical protocorm formation), but medium (ex vitro or in vitro) did not (F = 3.4; P > 0.07) for fresh seeds (Fig. 5). Both temperature (F = 30.5; P < 0.001) and the medium used (F = 21.9; P < 0.001) had treatment effects in dry-stored seeds, with ex vitro germination consistently higher than in vitro germination (Fig. 5). The range of temperature over which germination was recorded was slightly wider ex vitro (around 15–30°C) than in vitro (around 20–25°C), with 25°C clearly effective for both seed lots (Fig. 5). Total germination was, however, about twice as high for fresh seeds, lot 2, compared to dry-stored seeds, lot 1 (around 60 vs 30% maximum).
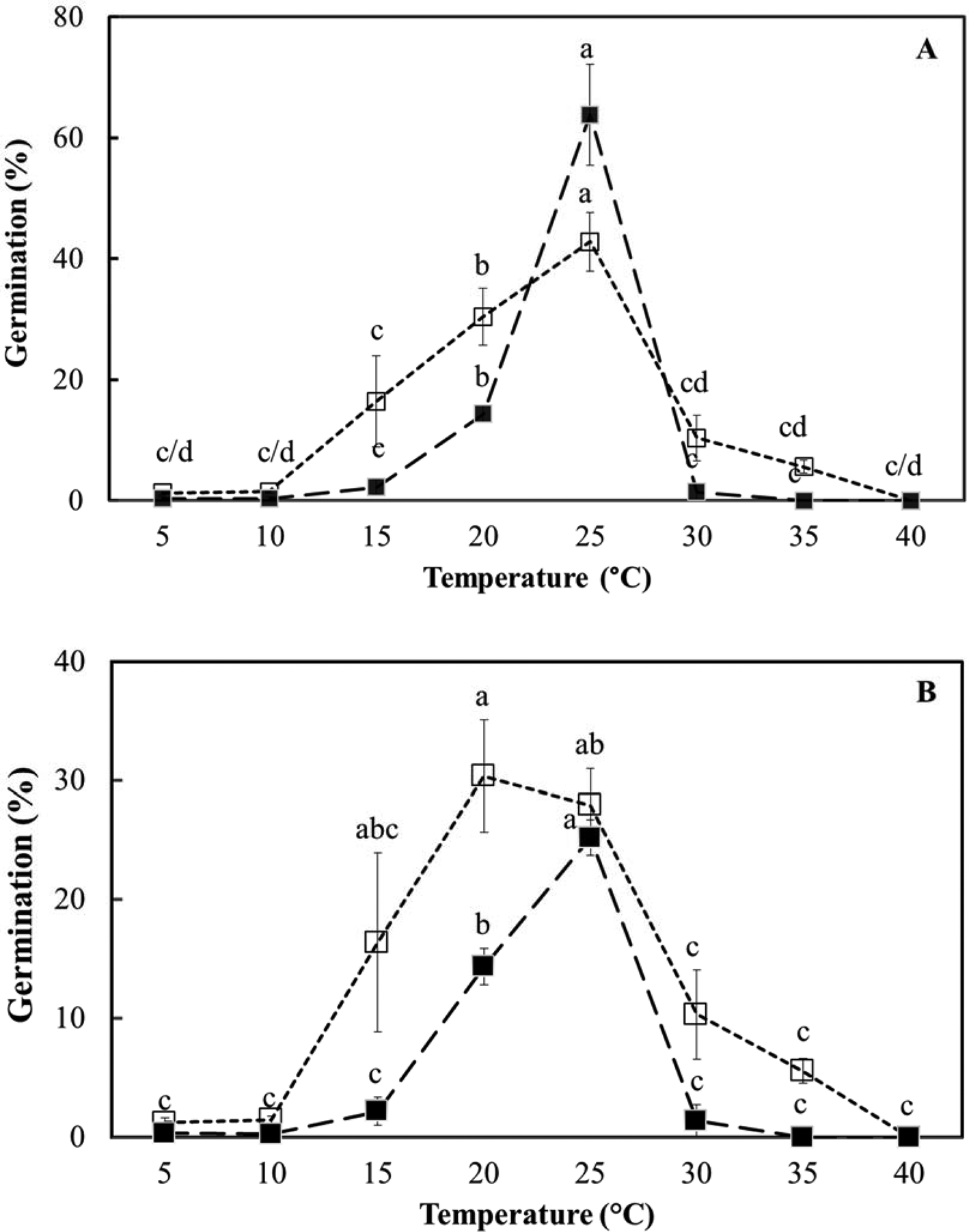
Fig. 5. Effect of temperature on D. cruentum seed germination (spherical protoccorm production) ex vitro, on plain agar without disinfection (□) and in vitro, on ½ MS medium with disinfection (■). (A) Fresh seeds (lot 2) and (B) dry-stored seed (lot 1) were assessed for germination after 28 d under a 12 h photoperiod. For each temperature, significant germination differences (P < 0.05) between sowing methods are indicated by different letters based on triplicate sowings of 100–150 full seeds.
The possible promoting or inhibitory effect of light on ex vitro seed germination was assessed at one constant and one alternating temperature. Light level had no significant (P > 0.05) effect on germination of fresh seeds (lot 2) at 25°C when comparing light (12 h d−1) versus dark (weekly unwrapping dishes for scoring) versus double dark (unwrapped after 28 d) (Table 3). For dry-stored seed, the effect of light was variable, with germination in double dark > light > dark at 25°C (Table 3). However, among temperature treatments (constant vs alternating) for dry-stored seeds, the effect of alternating temperature (30/20°C) was to reduce germination significantly (P < 0.05) at comparable light levels, except for the dark treatment (Table 3).
Table 3. Effect of temperature (25 and 30/20°C) and light (12/12 h light, dark and double dark condition) on the ex vitro germination (spherical protocorm formation) of fresh (lot 2) and dry-stored (lot 1) D. cruentum seeds after 28 d

* Within a single temperature treatment, the results are significantly different with the lighting condition when the data are followed by a different lower case letter. For dry-stored seed only, data are significantly different for specific light conditions across temperature treatments when followed by difference upper case letters.
Finally, the effect of nitrate concentration on the ex vitro germination of the two seed lots was assessed at 25°C in the light and dark (Fig. 6). At each KNO3 concentration, there was no effect of light conditions on the germination of fresh (F = 0.007; P = 0.93) or dry-stored seed (F = 0.109; P = 0.75). The effect of nitrate on germination was just significant in fresh seeds (F = 2.941; P = 0.05) and highly significant in dry-stored seeds (F = 4.32; P = 0.01), mainly as a result of an inhibitory effect on germination in the light and the dark at 30 mmol KNO3 (Fig. 6). There was no interaction between the factors of light and nitrate (F = 0.386; P = 0.82 for fresh seed and F = 1.17; P = 0.36 for dry-stored seed).
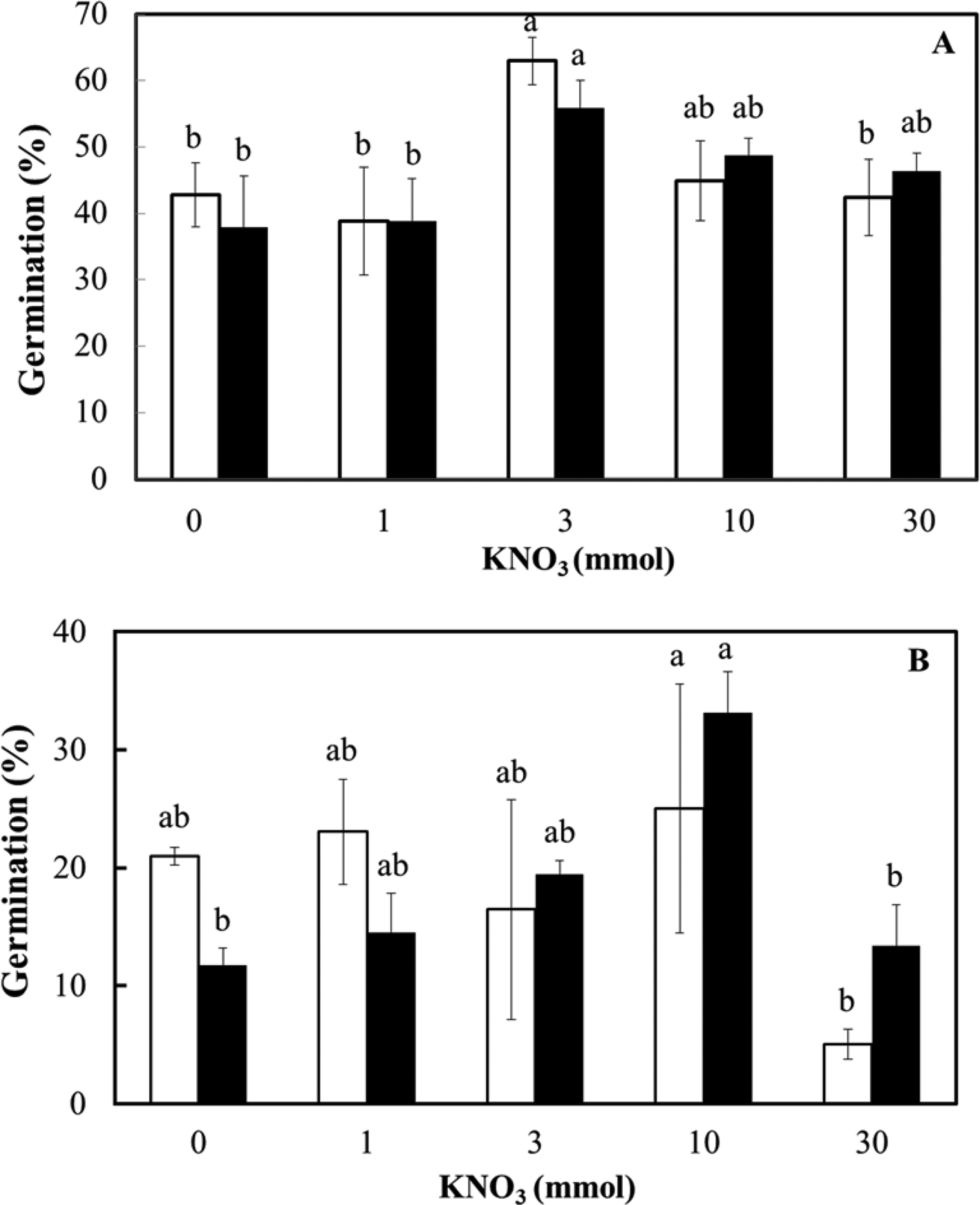
Fig. 6. Ex vitro germination of D. cruentum seeds in response to KNO3 concentration (0, 1, 3, 10 and 30 mmol). Seeds were sown without disinfection on plain agar in the light (□) and dark (■) at 25°C. (A) Fresh seeds (lot 2) and (B) dry-stored seeds (lot 1) were assessed for germination after 28 d in the light (12 h photoperiod) or dark (occasional opening of wrapped dishes). For each light condition, significant germination differences (P < 0.05) between KNO3 concentrations are indicated by different letters based on triplicate sowings of 100–150 full seeds.
Discussion
Seed micromorphology
Orchid seed micromorphometric characteristics are important in elucidating taxonomic, phylogenetic and phytogeographic relationships (Verma et al., Reference Verma, Sharma, Thakur, Sembi and Vij2014), including in Dendrobium (Chattopadhyay et al., Reference Chattopadhyay, Banerjee and Chaudhury2010). While orchid seeds are often <1 mm long, and can be considered to be DUST-like, they are of different shapes, including ellipsoid, oblongoid, ovoid, globose, trigonous, tetragonous and fusiform in the Epidendreae (Dressler, Reference Dressler1993; Molvary and Kores, Reference Molvary and Kores1995; Molvary and Chase, Reference Molvary, Chase, Pridgeon, Cribb, Chase and Rasmussen1999). In the case of D. cruentum, the seeds are fusiform, with thickened testa cell walls (Figs 1–3), quite typical of Dendrobium species (Chaudhary et al., Reference Chaudhary, Chattopadhyay and Banerjee2014). Moreover, D. cruentum testa cell thickenings have a cottony-white substance, previously observed in sub-tropical D. parishii Rchb.f. and D. williamsonii Day & Rchb.f. (Chaudhary et al., Reference Chaudhary, Chattopadhyay and Banerjee2014).
Comparative studies on 32 threatened Western Himalayan orchids reveal that most of the seed space is air, with only 10 species having embryos that exceeded 50% of the seed volume (Verma et al., Reference Verma, Sharma, Thakur, Sembi and Vij2014). In contrast, in Dendrobium species studied thus far, the seed embryo is relatively large and often (two-thirds of cases) occupies >60% of the seed volume (Fig. 3). Our previous comparative study on nine Dendrobium species revealed that the embryo occupied 64–87% of the seed volume (Prasongsom et al., Reference Prasongsom, Thammasiri, Pritchard and Thammasiri2017). Moreover, these ‘large’ embryo seeds generally germinated as well ex vitro as in vitro (Prasongsom et al., Reference Prasongsom, Thammasiri, Pritchard and Thammasiri2017). Similarly, the air space in D. cruentum seeds is only around 13–15% (Table 2) and germination ex vitro is possible (Figs 4–6).
The determination of embryo size, including relative to the seed or endosperm, is a valuable way of morphologically categorizing seeds (Martin, Reference Martin1946; Baskin and Baskin, Reference Baskin and Baskin2014), and embryo size data have been used to study the evolution of dormancy (Forbis et al., Reference Forbis, Floyd and de Queiroz2002; Finch-Savage and Leubner-Metzger, Reference Finch-Savage and Leubner-Metzger2006; Linkis et al., Reference Linkis, Graeber, Knight and Leubner-Metzger2010). For example, reconstructions for embryo to seed ratio (E:S) using family means for 179 families show that E:S increases in derived angiosperms compared with ancestral angiosperms (Forbis et al., Reference Forbis, Floyd and de Queiroz2002). Moreover, morphological (MD) and morphophysiological (MPD) dormancy is often present in seeds with differentiated embryos and a low E:S ratio. In Ribes (Grossulariaceae) species, E:S needs to increase from approximately 0.2–0.3 to 0.6–0.8 before germination can be completed, and among species, the greater the initial embryo length the lower the dormancy level, which is also co-associated with warmer mean annual temperatures (Mattana et al., Reference Mattana, Stuppy, Fraser, Waller and Pritchard2013). Habitat is also an evolutionary driver for embryo size in Apiaceae, with a large relative embryo length associated with more rapid germination of species in dry and open habitats (Vandelook et al., Reference Vandelook, Janssens and Probert2012). Similarly, in Dendrobium species, the seed air space is more dependent on climatic regions than phylogeny. For example in 20 species of eastern North India, average embryo volumes increase from 62 to 76% of the seed volume in species adapted to temperate versus tropical habitats (Chaudhary et al., Reference Chaudhary, Chattopadhyay and Banerjee2014). In tropical D. cruentum, the DUST seeds have a relatively large embryo (approximately 84% of the seed volume; Table 1) that does not need to extend in length before germination occurs. Such a strategy in this orchid potentially reduces the distribution of germination times and improves competitiveness in its ephiphytic niche.
Taken with our earlier studies on 12 Dendrobium species (Prasongsom et al., Reference Prasongsom, Thammasiri, Pritchard and Thammasiri2017; Diantina et al., Reference Diantina, McGill, Millner, Nadarajan, Pritchard and McCormick2020a), the results for D. cruentum emphasize the importance of not limiting considerations of the ecology, evolutionary and phylogenetic trajectory of orchids to the seeds’ external (testa) morphology (Parthibhan et al., Reference Parthibhan, Benjamin, Muthukumar, Ahamed Sherif, Senthil Kumar and Rao2012; Barthlott et al., Reference Barthlott, Große-Veldmann and Korotkova2014). Rather, embryo size (and volume) determinations in relation to the seed appear to provide new perspectives on the ecophysiology and evolution of orchid seed germination and dormancy in the way that the E:S ratio has done for angiosperms as a whole (Linkis et al., Reference Linkis, Graeber, Knight and Leubner-Metzger2010; Vandelook et al., Reference Vandelook, Janssens and Probert2012; Willis et al., Reference Willis, Baskin, Baskin, Auld, Venable, Cavender-Bares, Donohue and de Casas2014).
Nitrate, temperature, light and seed germination
Many orchid seed culture media include two forms of nitrate (KNO3 and NH4NO3), and a concentration of about 10 mmol is known to increase seed germination and seedling development in Dendrobium aqueum Lindl. (Parthibhan et al., Reference Parthibhan, Benjamin, Muthukumar, Ahamed Sherif, Senthil Kumar and Rao2012). In contrast, increasing the concentration of NO3− ions to approximately 5 mmol slightly decreases dark germination in the temperate terrestrial species Dactylorhiza majalis (Rchb.) P.F.Hunt & Summerh. (Rasmussen, Reference Rasmussen1995), indicating the potential for a species-specific effect of nitrate on germination. We exposed seeds of D. cruentum seeds to a 30-fold range of KNO3 (1–30 mmol), within the known range of physiological activity for nitrate when applied as KNO3, that is, generally 0.1–50 mmol (Duermeyer et al., Reference Duermeyer, Khhodapanahi, Yan, Krapp, Rothstein and Nambara2018). To seeds, nitrate has a dual function; as a nutrient and as a signal, discrimination between the two is not often attempted. To complicate matters, nitrate as a signal appears to contribute to the dormancy level, including through seed development, and to stimulating germination (Toorop, Reference Toorop2015; Duermeyer et al., Reference Duermeyer, Khhodapanahi, Yan, Krapp, Rothstein and Nambara2018). The efficacy of nitrate can relate to an increase in population sensitivity to alternating temperature, for example, Ranunculus sceleratus L. (Probert et al., Reference Probert, Gajjar and Haslam1987), to a decrease in ABA, for example, in Arabidopsis (Ali-rachedi et al., Reference Ali-rachedi, Bouineot, Wagner, Bedu, Meyer and Truong2004), or to light responsiveness via the active form of phytochrome (Pfr) (Probert et al., Reference Probert, Gajjar and Haslam1987; Baskin and Baskin, Reference Baskin and Baskin2014). In reviewing evidence for 20 species, it was found that half of the nitrate-related responses in seeds were associated with light; for example, nitrate reduces the light requirement for germination (three species) or improved germination in the dark (five species) (Duermeyer et al., Reference Duermeyer, Khhodapanahi, Yan, Krapp, Rothstein and Nambara2018). In contrast to seeds of the orchid Bletilla striata (Thunb.) Rchb.f. that are stimulated to germinate by low concentrations of nitrate (Ichihashi and Yamashita, Reference Ichihashi and Yamashita1977), nitrate seems to promote germination in D. cruentum seed only slightly (around 20% increase) at 3 mmol for fresh (lot 2) seed and at 10 mmol for dry-stored (lot 1) seed (Fig. 6). This response is not dependent on light conditions (light vs dark), however. More typically, seeds receptive to nitrate are much more sensitive, for example, 20 mmol KNO3 increases S. officinale germination by around 60% by controlling testa rupture and seed water content (Toorop, Reference Toorop2015). We conclude that the promotive effect of nitrate on D. cruentum seed germination was modest and might relate more to its nutrient rather than signalling effect. In any case, there is no evidence of nitrate reducing any light requirement for D. cruentum seed germination ex vitro, as germination is similar in the dark. It is possible that this response is confounded by the possible saturation of any light requirement during the dark treatment, as the dishes were opened briefly for germination scoring once a week for the first 3 weeks. However, comparable germination levels in the double dark treatment (Table 3) indicate this is not the case. Overall, this is not surprising as unlike small seeds (<0.1 mg) of many angiosperms that are light requiring (Baskin and Baskin, Reference Baskin and Baskin2014), microseeds of epiphytic orchids are able generally to germinate in both light and dark (Arditti, Reference Arditti1979); however, long periods in the dark will ensure that the developing protocorms remain white, as seen for D. aqueum (Parthibhan et al., Reference Parthibhan, Benjamin, Muthukumar, Ahamed Sherif, Senthil Kumar and Rao2012). Nitrate does, though, have the potential to reduce germination in orchids, for example, at 2 mg NO3− dm−3 on mature seeds of Pseudorchis albida (L.) Á.Löve & D.Löve (Ponert et al., Reference Ponert, Figura, Vosolsobe, Lipavska, Vohnik and Jersakova2013). Similarly, D. cruentum seeds exposed to 30 mmol KNO3 had reduced germination ex vitro in the light and the dark (Fig. 6). Nitrate sensitivity during germination can affect orchid species’ habitat preferences and distribution; orchids from oligotrophic habitats are much more sensitive than those of eutrophic habitats, which can be almost insensitive (Figura et al., Reference Figura, Weiser and Ponert2020). Sensitivity to nitrate was also associated with soil parameters that indicated a higher nitrification rate.
Constant temperatures are clearly sufficient for D. cruentum seed germination (spherical protocorm formation; Fig. 3) in vitro and ex vitro. However, the temperature range for maximum germination (Fig. 5; Table 3) is quite narrow compared to many other species (Baskin and Baskin, Reference Baskin and Baskin2014; Durr et al., Reference Durr, Dickie, Yang and Pritchard2015), although well adapted to the tropical conditions in Thailand. Apart from fresh seed (lot 2) at 25°C, the level of germination in vitro is lower than that observed ex vitro. This could arise if the concentration of nitrate in the medium (52.6 mmol KNO3 plus ammonium nitrate) is supraoptimal and inhibitory. Regarding a possible interdependency of germination on light and alternating temperature, as in seeds of R. sceleratus (Probert et al., Reference Probert, Gajjar and Haslam1987), D. cruentum seeds tend to germinate better under various light conditions when temperature is constant rather than alternating, with the same average temperature (Table 3). However, it is clear that the warm temperature phase (30°C) applied here is supraoptimal for germination (Fig. 5). While this has the potential to obscure temperature × light effects, it appears that D. cruentum seed germination does not need a complex and interacting set of environmental factors for effective germination. In fact, the evidence suggests that these DUST seeds are non-dormant.
Dormancy concepts in orchids
Seed quality varied between the two seed lots of D. cruentum, with the dry-stored seed (lot 1) having lower germination than the fresh seed (lot 2) (Fig. 5). Undoubtedly, the processing time for the dry-stored versus fresh seed was longer, possibly resulting in seed ageing prior to cold storage. Orchid seed is reputed to be short-lived, with half lives of only about 2 weeks in humid conditions at about 40°C (Pritchard et al., Reference Pritchard, Poynter and Seaton1999; Hay et al., Reference Hay, Merritt, Soanes and Dixon2010; Fileti et al., Reference Fileti, Hengling, Gianeti, Hosomi, Pritchard, Machado-Neto and Custódio2021). To counteract this risk, many of the experiments were also run on fresh seed. In this instance, the seed came from a capsule that was just about to dehisce and used in the experiments soon after extraction. Such seed material might not have reached full maturity, thereby reducing the likelihood of the induction of maturation-related physiological dormancy. But this seems unlikely. Although the fresh seed (lot 2) dimensions are slightly narrower, the seed is not shorter than the dry-stored seed (Table 1). Moreover, the embryo occupancy of the seed space is not different between two seed lots (Table 1), indicating similar maturity.
Coat-imposed physical dormancy – a hindrance to water uptake through the testa, reinforced through high phenolic content – has been tentatively proposed for summer endemic members of the orchid genus Disa that inhabit high altitude grasslands with severe winter conditions to which the seeds are exposed (Thompson et al., Reference Thompson, Edwards and van Staden2001). Prolonged (4 h) chemical scarification with 1.75% NaOCl solution ensures maximum permeability of the testa and inner carapace, facilitating tetrazolium chloride permeation and conversion to formazan by the embryo. Similarly, surface disinfection with NaOCl bleach with 5% active chlorine both disinfects and scarifies seeds of Limodorum trabutianum Batt., an European terrestrial orchid, leading to much improved germination (Magrini and De Vitis, Reference Magrini and De Vitis2017). In contrast, seeds of the tropical epiphyte D. cruentum germinate (spherical protocorm) in 28 d on plain agar-water substrate without disinfection (Figs 4–6), indicating high permeability to water, no delay to the onset of germination and a lack of physical dormancy.
Undoubtedly physiological dormancy is present in the seeds of many orchid species from temperate regions, with germination benefiting from cold stratification and sowing in vitro on media composed of various elements and a carbon source (Rasmussen, Reference Rasmussen1995; Baskin and Baskin, Reference Baskin and Baskin2014). When the seeds’ dust-like form and undifferentiated embryo is factored in, it is not surprising that orchids are proposed to have a special kind of MD or MPD (Baskin and Baskin, Reference Baskin and Baskin2014). But viewing orchid seed as an uniform propagule in form and function does not account for observed heterogeneities. In relation to form, tropical Dendrobium species tend to have relatively large embryos, occupying a considerable proportion of the seed volume (Figs 1, 3, 4, Table 1; Prasongsom et al., Reference Prasongsom, Thammasiri, Pritchard and Thammasiri2017; Diantina et al., Reference Diantina, McGill, Millner, Nadarajan, Pritchard and McCormick2020a). In contrast, the mean embryo and seed air space of ten temperate species in Cephalanthera, Corallorhiza, Cypripedium, Epipactis, Paphiopedilum and Platanthera is 12 and 88%, respectively (Arditti and Ghani, Reference Arditti and Ghani2000). As noted earlier, ‘dust’ has no internal air spaces (Arditti and Ghani, Reference Arditti and Ghani2000) and the term gives no hint of the variation in orchid seed internal micromorphology.
Furthermore, there are also reasons to reassess the wholesale assignment of orchid seeds to a DUST class of dormancy (Willis et al., Reference Willis, Baskin, Baskin, Auld, Venable, Cavender-Bares, Donohue and de Casas2014). Beyond the traditional five kinds of dormancy, dust seeds (DUST) are small in size (mostly ≤1.0 mm in length) and have undifferentiated embryos (Eriksson and Kainulainen, Reference Eriksson and Kainulainen2011). In the case of D. cruentum and other species assessed (Prasongsom et al., Reference Prasongsom, Thammasiri, Pritchard and Thammasiri2017), the embryos may be undifferentiated with few cells, but they are also relatively large compared to the size of the seed, and are able to germinate under a wide range of conditions immediately after dispersal without any dormancy-breaking treatments, that is, ex vitro (Figs 4–6). This type of response actually defines the dormancy class of ND (Baskin and Baskin, Reference Baskin and Baskin2004, Reference Baskin and Baskin2014). It seems, therefore, that seeds of some orchid species can be both DUST and ND. We concur with the view that for small embryos in dwarf seeds, the ‘assignment of seeds to a dormancy class requires that studies be done to determine if embryos grow inside the seed before germination can occur’ (Baskin and Baskin, Reference Baskin and Baskin2005). In the case of D. cruentum, the large embryo does not need to grow substantially lengthwise within the seed before germination; rather spherical protocorm formation progresses and the testa splits (Fig. 4, Table 2).
Finally, it is thought that DUST seeds may have arisen from MPD seeds but not from MD seeds, and the ND state primarily evolved from PD but rarely from MPD (Willis et al., Reference Willis, Baskin, Baskin, Auld, Venable, Cavender-Bares, Donohue and de Casas2014). Analysis of the evolution of seed dormancy revealed no examples of a transition from DUST seeds to ND (Willis et al., Reference Willis, Baskin, Baskin, Auld, Venable, Cavender-Bares, Donohue and de Casas2014). Nonetheless, it seems that some Dendrobium species may disperse ND seeds.
Acknowledgements
S.P. acknowledges receipt of a Science Achievement Scholarship of Thailand (SAST). The Royal Botanic Gardens, Kew receives grant-in-aid from DEFRA.
Conflicts of interest
None declared.