Introduction
Lewis flax (Linum lewisii Pursh) is a perennial semi-evergreen subshrub native to North America (Ogle et al., Reference Ogle, St. John, Peterson and Tilley2006). The geographic range of Lewis flax in the United States extends from California to Eastern Minnesota and Michigan (Ogle et al., Reference Ogle, St. John, Peterson and Tilley2006; Reeves, Reference Reeves2006; Tork et al., Reference Tork, Anderson, Wyse and Betts2019). Lewis flax thrives in a variety of habitats throughout its range including mixed grass prairie, alpine brush, aspen (Populus tremuloides Michx.) communities, and coniferous forest openings (Ogle et al., Reference Ogle, St. John, Peterson and Tilley2006). Grown as a perennial oilseed crop, Lewis flax could potentially offer farmers sustainability and agroecosystem resiliency benefits typically associated with perennial crops. Increased root biomass associated with perennial grain crops can improve soil aggregate stability, reduce erosion and nutrient runoff risk, sequester atmospheric carbon, help withstand drought, and increase soil organic matter (Glover and Reganold, Reference Glover and Reganold2010; Marquardt et al., Reference Marquardt, Vico, Glynn, Weih, Eksvärd and Björkman2016; Baker, Reference Baker2017; Vico and Brunsell, Reference Vico and Brunsell2018; Crews, Carton and Olsson, Reference Crews, Carton and Olsson2018; DeHaan and Ismail, Reference DeHaan and Ismail2017; Ledo et al., Reference Ledo, Smith, Zerihun, Whitaker, Vicente-Vicente, Qin, McNamara, Zinn, Llorente, Liebig, Kuhnert, Dondini, Don, Diaz-Pines, Datta, Bakka, Aguilera and Hillier2020). Perennials often use nutrients more efficiently than annuals, potentially sparing input expenses to producers (Cox et al., Reference Cox, Glover, Van Tassel, Cox and DeHaan2006; Vico and Brunsell, Reference Vico and Brunsell2018; DeHaan et al., Reference DeHaan, Larson, López-Marqués, Wenkel, Gao and Palmgren2020). Producers could also save time by growing Lewis flax, as perennial crops do not require replanting each spring (DeHaan et al., Reference DeHaan, Larson, López-Marqués, Wenkel, Gao and Palmgren2020; Ryan et al., Reference Ryan, Crews, Culman, DeHann, Hayes, Jungers and Bakker2018; Lanker, Bell and Picasso, Reference Lanker, Bell and Picasso2019). Perennial crops can also be intercropped with annual grain or legume crops to provide greater productivity and additional revenue streams (Mckenna et al., Reference Mckenna, Crews, Kemp and Sikes2020; Ryan et al., Reference Ryan, Crews, Culman, DeHann, Hayes, Jungers and Bakker2018).
Additionally, Lewis flax benefits several biotic ecosystem components as a symbiotic partner to a diversity of pollinators and rhizome microbiome habitat. The showy blooms of perennial flax are visited by at least 12 genera of native pollinators and honeybees (Cane and Love, Reference Cane and Love2016). Additionally, Lewis flax is known to form symbiotic mutualistic relationships with arbuscular mycorrhizal fungi (AMF). Analyses of native plant species' associations with AMF by Jordan et al. (Reference Jordan, Aldrich-Wolfe, Huerd, Larson and Muehlbauer2012) found that Lewis flax root rhizosphere samples were associated with greater AMF species richness compared to several other species. Relationships with AMF may improve the ability of Lewis flax to uptake essential nutrients like phosphorus and nitrogen (Baker, Reference Baker2017) and soil water. Improved nutrient uptake facilitated by AMF relationships is due to extensive hyphal networks that act as an extension of the root system, increasing effective root extent and absorptive area (Hart and Trevors, Reference Hart and Trevors2005). Mutualistic relationships between Lewis flax and AMF could also improve soil structure and carbon sequestration (Gujre et al., Reference Gujre, Soni, Rangan, Tsang and Mitra2021).
In addition to potential ecosystem and agricultural system resiliency benefits, Lewis flax seed is rich in omega-3 fatty acids, which are indistinguishable from those produced by cultivated annual flax (Linum usitatissimum) varieties (Innes et al., Reference Innes, Gossweiler, Jensen, Tilley, St. John, Jones, Kitchen and Hulke2022). The human health benefits of omega-3s are well-documented; therefore, Lewis flax seeds could potentially be marketed similarly to annual flax (Singh et al., Reference Singh, Mridula, Rehal and Barnwal2011; Tork et al., Reference Tork, Anderson, Wyse and Betts2019). Large corporations and small-scale bakeries alike have expressed interest in using perennial crops for their products to meet sustainability goals (Baker, Reference Baker2017; Lanker, Bell and Picasso, Reference Lanker, Bell and Picasso2019). For example, Kernza© has been used for brewing beer by Patagonia Provisions and making cereal by General Mills (Lanker, Bell and Picasso, Reference Lanker, Bell and Picasso2019). Similar interest has been expressed for perennial flax by food processing companies interested in promoting agroecosystem sustainability or regenerative production practices. Finally, Lewis flax could also provide fiber for textile use, similar to annual flax, providing an additional revenue stream.
Lewis flax may have a promising future as an alternative oilseed and fiber crop; however, much work remains to adapt this wild species to row-crop production systems. Compared to annual flax, Lewis flax is a larger plant than annual flax when mature, producing several branches that originate from a woody base (Ogle et al., Reference Ogle, St. John, Peterson and Tilley2006; Reeves, Reference Reeves2006). This morphological difference suggests that producers may need to use different seeding rates, row spacings, and production practices when cultivating Lewis flax vs annual flax. Optimal seeding date also likely differs between the two species. Annual flax is typically planted in late April through early May (Kandel and Keene, Reference Kandel and Keene2020; Ogle et al., Reference Ogle, St. John, Peterson and Tilley2006; Reeves, Reference Reeves2006), but optimal planting timing for perennial flax is unknown. Optimal harvesting approaches for perennial flax will also likely differ from approaches used to harvest annual flax, because perennial flax will be green at harvest, not senescing as annual flax.
Furthermore, like annual flax, Lewis flax is likely a weak competitor against weeds, and both seed quality and yield may be reduced because of competition from weeds (Bilalis et al., Reference Bilalis, Karkanis, Pantelia, Patsiali, Konstantas and Efthimiadou2012; Ehrensing, Reference Ehrensing2008; Flax Council of Canada, 2022; McCollough, Gallandt and Molloy, Reference McCollough, Gallandt and Molloy2020). A meta-analysis of the organic:conventional yield gap by de Ponti et al. (Reference de Ponti, Rijk and Van Ittersum2012) found that average organic flax yields are only 65% of typical conventional yields. Lewis flax may struggle even more to compete against weeds than annual flax in the establishment year, as it requires up to 30 days to germinate, providing annual weeds a substantial time advantage for establishing (Reeves, Reference Reeves2006). Annual flax growers can compensate for weak flax competition with herbicides, but no herbicides are currently labeled for use in perennial flax systems where cultural control methods would initially prevail. Also, markets for perennial flax seed may favor organic production practices.
Although growing Lewis flax as an oilseed crop may have a promising future, significant agronomic and genetic work remains in order to develop it into an economically viable crop. Due to the novel nature of growing Lewis flax in a row-cropping system, we approached the task of developing its agronomic best management practices through the lens of adaptive strategies. We established multiple experiments in Eastern and Central North Dakota and Western Minnesota to assess planting timing, seeding depth, row spacing, plant population density, weed management, intercropping strategies, and harvesting techniques within Lewis flax stands grown as an agronomic oilseed crop. Determining agronomic best management practices for Lewis flax will benefit agricultural producers hoping to produce perennial flax.
Methods
Plant materials
Seeds of Lewis flax (L. lewisii Pursh.) cultivar ‘Maple Grove’ were used for all experiments. Flax seed was sourced from Southwest Seed Inc. Dolores, CO. For some experiments, intercrop or cover crop species were also included. These included oat (Avena sativa L. cv. ‘Paul’), winter wheat (Triticum aestivum L. cv. ‘Noreen’), crested wheatgrass (Agropyron cristatum [L.] Gaertn.), prairie Junegrass (Koeleria macrantha [Ledeb.] Schult.), field pea (Pisum sativum L.), red clover (Trifolium pratense L.), white clover (Trifolium repens L.), berseem clover (Trifolium alexandrinum L.), and faba bean (Vicia faba L.).
Field design and management
Experiment one consisted of a flax plant spacing study and an intercropping study. Both studies were planted near Comstock, MN (lat. 46.623927, long. −96.752881, elev. 281.33 m) on 27 May 2020 and the North Dakota State University Carrington Research Extension Center in Carrington, ND (lat. 47.506380, long. −99.123652, elev. 475.5 m), on 29 May 2020, using an Almaco belt cone seeder. The primary environmental difference between these two sites was soil type, with the Comstock site dominated by poorly drained Fargo series fine, smectitic, frigid typic epiaquerts and the Carrington site dominated by well-drained Heimdal-Emrick series coarse-loamy, mixed, superactive, frigid calcic hapludolls. The Lewis flax plant spacing study used a randomized complete block design with four replications. Plot dimensions were 3.7 m×6.1 m. Treatments were factorial combinations of row spacing (30.5 or 76.2 cm) and seeding density (896,610, 1,793,220, and 3,586,440 pure live seeds ha−1 [i.e., 1×, 2×, and 4× the recommended seeding rate]) (Kitchen, Reference Kitchen1994) (USDA-Natural Resource Conservation Service, 2004). Seeds were planted between 1.3 and 1.9 cm deep on soil prepared with a sweep shovel cultivator with harrow-type smoothing (DMI, CNH Industrial, London, UK). Plots were over-sown with an oat and pea companion crop at 304,918 and 130,679 pure live seeds ha−1, respectively. The intercropping study, by contrast, included Lewis flax planted using 61 cm row spacing at the 2× rate, with the inter-row spaces planted to one of the following cover crop treatments: red clover, white clover, berseem clover, crested wheatgrass, prairie Junegrass, faba bean, or no cover crop. An additional control plot was planted in each rep with 76.2 cm row spacing at the 2× rate with no cover crop. Experiment one was planted a second time at Comstock, MN, on 24 June 2020, with the only change in protocol being the use of packing wheels (Brillion Landoll, Marysville, KS, USA) to firm the soil prior to planting.
Experiment two was designed to explore optimal plant spacings and planting dates for Lewis flax. This experiment was established at two sites. The Comstock, MN site was used once again for experiment two; however, the Carrington, ND site was replaced with a new site at the Dale E. Herman Research Arboretum near Absaraka, ND (lat. 46.987708, long. −97.352090, elev. 314.55 m). The primary environmental difference between these two sites is soil type. Soils at the Absaraka site were dominated by a Warsing sandy loam complex (fine-loamy over sandy or sandy-skeletal, mixed, superactive, frigid Oxyaquic Hapludolls) (United States Department of Agriculture—Natural Resource Conservation Service). Experiment 2 was planted on 5 September 2020 (fall seeded) and 5 November 2020 (dormant seeded) into shallowly roto-tilled soil, using a different Almaco belt cone seeder with four CaseIH 1200 series row openers in 76.2 cm wide row spacing. This experiment used a randomized complete block design with four replications, with 3.0 m×6.1 m plots. Seeds were planted approximately 0.3 cm deep into moist soil. ‘Planting approach’ treatments applied at each site were factorial combinations of row spacing (38.1 or 76.2 cm wide), seeding population density at 4× and 6× rates (3,586,440 and 5,379,660 pure live seed ha−1, respectively), and planting timing (early fall vs late-fall-dormant). This study included three additional replicates of the dormant seeded 4× density at 76.2 cm row spacing, which we intended to, but subsequently did not, manage differently. These replicate treatments are labeled as ‘A’, ‘B’, and ‘C’ for results presentation purposes.
Agronomic management for experiment two consisted of inter-row cultivation (for plots with 76.2 cm flax row spacing only) utilizing an Alloway four-row cultivator (Alloway Manufacturing, Fargo, ND, USA) with sweep shovels set at 76.2 cm row spacing and a depth of 7 cm and mowing entire plots periodically with a rotary blade deck mower or a John Deere 370 flail mower (John Deere, Moline, IL, USA).
To quantify soil fertility at the sites where experiment two was established, a Giddings probe was used 15 May 2021 to quantify soil nitrate (NO3), phosphorus (P), potassium (K), pH, electrical conductivity (EC), ammonium (NH4), and total carbon (C) at two depths (0–20 and 20–40 cm). Total nitrogen (N) and organic matter (OM) were quantified at these depths as well, but also at 40–60 and 60–80 cm. At Comstock, one 80 cm core was extracted because the field was relatively homogeneous. At Absaraka, however, the studies were planted across a patchwork of previous perennial crops. One patch had been planted to strawberry (Fragaria × ananassa spp. Duchesne) for five years (ABS-A), a second patch was occupied by a study that included perennial ryegrass (Lolium perenne L.), white clover, and red clover (ABS-B), and a third patch was in alfalfa (Medicago sativa L.) for five years (ABS-C). We extracted one 100 cm core from each of these three patch areas, and each core was divided into four 20 cm sections for analysis. Analysis was performed by the North Dakota State University Soil Testing Lab. Results are reported in Table 1. Fertilizer recommendations for Lewis flax do not exist, however previous research about annual flax indicated that the optimal balance between yield and N use efficiency was found between 20 and 30 kg ha−1 N (Kakabouki et al., Reference Kakabouki, Mavroeidis, Tataridas, Roussis, Katsenios, Efthimiadou, Tigka, Karydogianni, Zisi, Folina and Bilalis2021), or approximately the amount of N residual our fields contained. Therefore, we did not add supplemental fertility to either field site during data collection.
Table 1. Soil nitrate (NO3), phosphorus (P), potassium (K), pH, organic matter (OM), ammonium (NH4), total nitrogen (N), and total carbon (C) quantified from 80 cm deep soil cores extracted at Comstock MN (COM) and Absaraka ND (ABS) on 14 May 2021
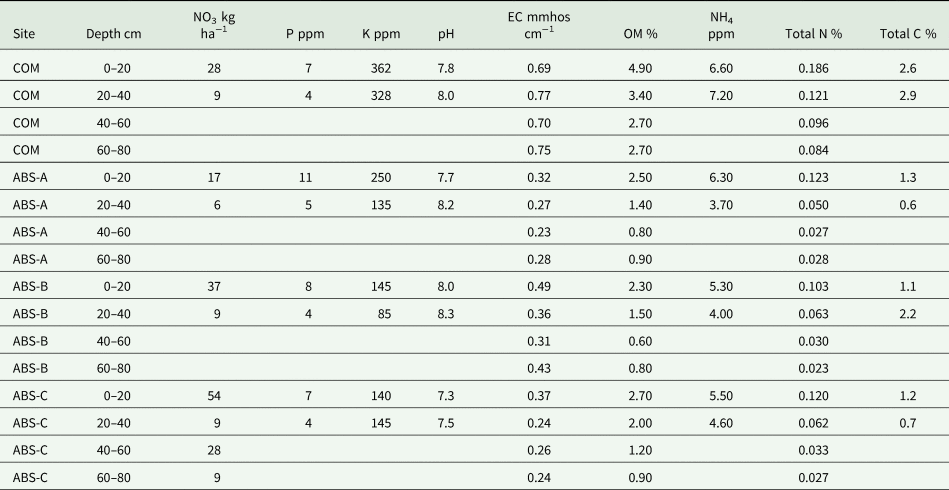
ABS-A area was previously planted to strawberry, ABS-B area was previously planted to red clover, white clover, and perennial ryegrass, and ABS-C area was previously planted to alfalfa.
An additional intercropping demonstration experiment was planted 24 September 2021 at Comstock, MN, and Absaraka, ND. Hard red winter wheat cv. ‘Noreen’ was planted using a 1.5 m wide Great Plains 3P600 grain drill with rows spaced 19 cm (7.5 in). Two winter wheat population densities were planted: 76 kg ha−1 (68 lbs ac−1) and 121 kg ha−1 (108 lbs ac−1) along with a control with no wheat planted, using a randomized complete block design in a split plot arrangement with two replications. Plots measured 3 m×3 m. On the same days wheat was planted, Lewis flax was planted perpendicular to the winter wheat using an Almaco belt cone seeder with four Case IH 1200 series row openers and 76.2 cm wide rows. The left two row units planted Lewis flax at the 1× rate, and the right two row units at the 2× rate. A single pass of the planter was made at each environment, with seeds planted approximately 0.3 cm deep into moist soil.
Data collection
Approximately two months after Lewis flax had fully emerged, initial flax stand counts were conducted at the Comstock site in all the ‘planting approach’ treatments (experiment two). During mid-July 2021 and again during mid-May 2022, stand counts were conducted in a subset of plots (4× seeding rate/76.2 row spacing/dormant seeding) at both sites. The same methodology was used for all flax stand counts. Quadrats measuring ¼ m2 were placed at evenly spaced positions along one inner flax row per plot, and all flax plants were counted, taking care to count individual plants, not branches. For the flax/winter wheat intercropping demonstration study, we counted all the flax plants in each planted row to determine stand density.
Prior to harvest, Lewis flax was cut at 17–20 cm (7–8 inches) height using a sickle mower (Maschio Gaspardo NA, Dewitt, IA, USA) when the earliest seed bolls had fully dried, flax seed had turned dark brown, and bolls were beginning to shatter, at both locations on 7 July 2022. Flax plants were left in windrows and later picked up and threshed using a Hege 125 small plot combine with a draper header (Wintersteiger Seedmech, Austria) at the Comstock, MN site. The Absaraka, ND site was not combined due to excessive boll shatter and windrow decomposition caused by damp conditions. Harvested seeds for each plot were gathered in cloth bags, cleaned with sieves, and weighed to determine yield. Winter wheat in the intercropping demonstration experiment was directly cut with the Hege 125 combine at optimal grain moisture. Grain was captured in cloth bags, dried additionally at 32°C for a week, then cleaned and weighed.
Statistical analyses
Analysis of variance was performed using SAS PROC GLIMMIX (SAS release 9.4, SAS Institute Inc., Cary, NC, USA) to test fixed treatment effects (planting date, row spacing, planting density, environment) on flax stand counts and flax yield for experiment two. For the winter wheat intercropping demonstration study, a similar approach was used to test the fixed effects of winter wheat seeding rate and flax seeding rate on flax stand count. Simple main treatment effects and interactions for all tests were considered significant at α = 0.05. Distributional assumptions were adjusted to optimize model fit statistics. In general, a negative binomial distribution optimized the model fit for count data, whereas a lognormal distribution was optimal for continuous data such as yield. Replication was considered as a random effect for all models. Following significant F-tests, post-hoc mean separations were performed using Tukey's honest significant difference correction for multiple comparisons. The study design and analysis plan was not pre-registered.
Results and discussion
2020: experiment one, spring-seeded perennial flax
At the Comstock, MN, site, spring-planted Lewis flax emerged, but soon after emergence was buried by a torrential rain that washed soil from the top of planting ridges into the planting furrows, thus burying and killing the newly emerged seedlings. Subsequently, an attempt at re-establishment on 24 June 2020, in which we used packing wheels to reduce the soil ridging from the planter, also failed, because more early summer rains induced formation of an impenetrable crust on the clayey soil, thereby suppressing emergence. At the Carrington site, establishment of spring-planted Lewis flax and small-seeded intercrop species failed as well, likely due to a combination of drought and improper seeding depth related to a loose seedbed prepared on the morning of planting. Only the large-seeded faba bean intercrop emerged reliably at Carrington, followed by a substantial flush of summer annual weeds dominated by redroot pigweed (Amaranthus retroflexus L.).
Lewis flax seeds are smaller than domesticated annual flax, with an observed range in 1000 seed mass from 1.5 to 3.4 g, compared to 5.77 g for annual flax (Pradhan et al., Reference Pradhan, Meda, Naik and Tabil2010; Innes et al., Reference Innes, Gossweiler, Jensen, Tilley, St. John, Jones, Kitchen and Hulke2022). Planting depth recommendations for growing Lewis flax in an agronomic context suggest planting at a depth of 0.6–1.3 cm (¼–½ inch; USDA-Natural Resources Conservation Service, 2004). For experiment one, Lewis flax seeds were planted no less than 1.3 cm deep, due to limitations of the planting equipment, but this may have been too deep. Seedbed preparation may also have played some role in poor flax and intercrop emergence at the Carrington site. For seeding annual flax, rolling to create a firmer seedbed on lighter-textured soils can improve annual flax establishment (Couture et al., Reference Couture, DiTommaso, Asbil and Watson2004). The Carrington soil is lighter-textured, whereas soil at the Comstock site is heavier clay, which may explain why seeding proved more difficult at the Carrington site, as the lighter soil texture made shallow seeding difficult to achieve.
Like annual flax, Lewis flax has a mucilaginous seed coat that helps the seed adhere to soil when dispersed (Chambers, Reference Chambers2000). Presumably, this feature should help stabilize shallow seedings of Lewis flax in the soil. However, even with this adaptation, we observed that the fragile newly emerged seedlings were vulnerable to soil erosion and crusting caused by substantial precipitation events. Due to numerous failures of spring- or summer-seeded perennial flax, we decided to test seeding during early and late fall (i.e., dormant seeding) for establishment of a second set of experiments, because heavy precipitation events are less common during fall.
2021–2022: experiment two, fall-seeded perennial flax
Both early and late fall (dormant) seeding of Lewis flax produced even stands of flax that started to emerge in late March, while heavy frosts were still occurring. Based on stand counts in all planting approach treatments conducted at Comstock 13 July 2021, the fall seeding timing was associated with greater initial mean flax stand density compared to the later fall dormant timing (P = 0.0055, F = 9.46, 20 ± 7 vs 13 ± 4 flax plants m−1, respectively). Row spacing (i.e., 76.2 vs 38.1 cm) did not affect the mean number of flax plants that emerged and established (P = 0.1518, F = 2.2, 5 ± 5 vs 18 ± 6 plants m−1, respectively). Planting density (i.e., 4× vs 6×) also did not influence mean flax stand density (P = 0.8966, F = 0.02, 16 ± 6 vs 16 ± 5 plants m−1, respectively). Based on counts made in a subset of ‘planting approach’ treatments (the A, B, and C replications of the dormant seeded 4× rate with 76.2 cm row treatment) conducted at both sites during mid-July 2021, flax counts differed between sites, with 100 ± 15 plants m−1 at Absaraka and 14 ± 3 plants m−1 at Comstock (P < 0.0001, F = 74.95). Flax counts did not differ among the A, B, and C plots (Fig. 1). These results indicate that equal seeding rates at each site resulted in substantially divergent stand counts between sites when assessed shortly after flax establishment. However, stand counts determined during mid-May 2022 at both sites in the same plots indicated that flax stand counts did not differ between sites (P = 0.1085, F = 3.54, data not shown), with a mean of 23 ± 7 plants m−1 at Comstock vs 10 ± 3 plants m−1 at Absaraka (data not shown). As with the 2021 count, flax stand counts did not differ among the A, B, and C replications (Fig. 2). These results show that flax stands in the first year were highly variable; however, during the second year of establishment, plants tended to self-thin to a more standard density. Self-thinning occurred in response to an extreme heatwave during which air temperatures exceeded 37°C (100°F) accompanied by high winds in June 2021. Increased thinning occurred in plots that were least protected by neighboring vegetation (observation). No other research exists documenting details about stand persistence or thinning in field-grown Lewis flax. Research conducted about another perennial crop, Kernza© intermediate wheatgrass (Thinopyrum intermedium) found that seeding the crop at 10.6 kg ha−1 and a row spacing of 0.19 m resulted in stand counts of 12–18 plants m−1 (Pinto et al., Reference Pinto, De Haan and Picasso2021), a crop density similar to that achieved in our experiments by the second year of establishment, 2022.

Figure 1. Mean + S.E. flax stand counts (plants m−1) for dormant seeded (‘Dorm’) flax planted with 76.2 cm row and a 4× seeding rate, pooled across both sites. Counts from 2021 were conducted in mid-July and counts from 2022 were conducted on 23 May. Different lowercase letters denote means that differed according to post-hoc means comparison tests with P-values adjusted via Tukey's honest significant difference (α = 0.05).

Figure 2. Mean flax seed yield + S.E. (kg ha−1) harvested during July 2022 at Comstock, MN from dormant (‘Dorm’) or fall planted flax planted at either 4× or 6× rates with either 38.1 or 76.2 cm wide rows. Different lowercase letters denote means that differed according to post-hoc means comparison tests with P-values adjusted via Tukey's honest significant difference (α = 0.05).
Lewis flax seed yield, which was successfully harvested at Comstock only, did not differ between fall and dormant seeding timings (P = 0.5590, F = 0.35, 62 ± 6 vs 60 ± 6 kg ha−1, respectively). Flax yield did not differ between the 38.1 vs 76.2 row spacings (P = 0.2599, F = 1.33, 57 ± 6 vs 64 ± 6 kg ha−1, respectively). Flax yield also was not influenced by seeding density (P = 0.3635, F = 0.86, 58 ± 6 vs 63 ± 6 kg ha−1 for 4× and 6× rates, respectively). Mean flax yield for all combinations of planting timing, row spacing, and seeding density is shown in Figure 2. The average yield across all treatments was approximately 59 kg ha−1, which is substantially below the average yield for annual flax which ranged from 753 to 1788 kg ha−1 in North Dakota in 2020 (USDA National Agricultural Statistics Service, 2020). Observationally, a large proportion of the seed yield was lost due to flax boll shattering that left the seed on the soil surface before combining occurred. Breeding efforts to improve Lewis flax as an agronomic crop plant must focus on driving variation toward more determinant flowering and reduction of boll shattering to increase harvestable yield (Innes et al., Reference Innes, Gossweiler, Jensen, Tilley, St. John, Jones, Kitchen and Hulke2022). Another factor possibly involved in low yield was lack of fertility. No supplemental fertility was added to either site; therefore, low fertility could have contributed to reduced seed yield, although according to soil tests, residual N should have been adequate. Substantial weed pressure at both sites likely reduced yield as well.
At Absaraka, the flax was swathed, but instead of drying, the windrows decomposed under wet conditions, making combining the seed impossible. Observationally, the flax yield at Absaraka was lower than the yield at Comstock, probably due to several factors. One, the field site at Absaraka was established hastily when logistics related to the global pandemic made using a site at Carrington ND (as originally planned) infeasible. We had little time to prepare the Absaraka site, which harbored perennial plant remnants from past studies (white clover [T. repens L.], red clover [T. pratense L.], perennial ryegrass [L. perenne L.], and strawberry [Fragaria × ananassa]), numerous entrenched deeply rooted perennial weed species (dandelion [Taraxacum officianale L.], Canada thistle [Cirsium arvense (L.) Scop.], curly dock [Rumex crispus L.], and perennial sowthistle [Sonchus arvensis L.]), as well as a newly planted winter rye (Secale cereale L.) cover crop. Inadequate time for site preparation left many of these plants in place, which caused much heavier perennial weed pressure at Absaraka compared to Comstock. The perennial weeds were not harmed much by mowing or tillage, especially the plants within the flax rows. Therefore, weed management attempts were less successful in terms of protecting yield at Absaraka compared to Comstock. We did not quantify weed density in this experiment, but weed counts conducted in other perennial flax plots produced total weed densities ranging from 300 to 600 plants m−2, constituting substantial weed pressure. Observationally, weed densities in the current study at Absaraka were similar.
Another challenging issue was wet soil at Absaraka, especially during 2022, which prevented timely weed management operations such as mowing and inter-row tillage. Wet weather also likely contributed to rust infection, which was observed affecting many plants at Absaraka. We assume that this infection was flax rust (Melampsora lini), a pathogen known to infect domesticated annual flax, or a closely related rust species that infects Lewis flax (Innes et al., Reference Innes, Gossweiler, Jensen, Tilley, St. John, Jones, Kitchen and Hulke2022).
Flax/winter wheat intercropping demonstration
At both sites, winter wheat established and formed a competitive canopy that nearly eliminated annual weed pressure from emergence to harvest. At Absaraka, winter wheat did not suppress Canada thistle, but only one of the plots was heavily infested with this weed species. Winter wheat mean yield differed between sites (P = 0.0021, F = 99.9), with 2476 kg ha−1 (36.8 bu ac−1) at Comstock and 6016 kg ha−1 (89.5 bu ac−1) at Absaraka. Mean winter wheat yield did not differ between the full vs 2/3 wheat seeding rates (P = 0.7539, F = 0.12). Flax stand density did not differ between sites (P = 0.0725, F = 11.1) or winter wheat rates (P = 0.3314, F = 1.07), but the 1× flax seeding rate produced less dense flax stands than the 2× rate (2.3 vs 4.9 flax plants m−1, respectively; P = 0.0103, F = 11.14).
At Absaraka, most of the established Lewis flax was found at the beginnings and ends of the planting rows. Edge effects of competition (the edges were maintained as alleys free of vegetation) could explain why flax did not establish well at Absaraka. The winter wheat grown at Absaraka yielded more than double the yield at Comstock, indicating that the wheat may have been more vigorous at Absaraka, and thus more competitive. At Comstock, both rates (i.e., 1× and 2× rates) emerged and established more uniform rows of Lewis flax (i.e., no edge effect) but the density was relatively low. Reducing the winter wheat seeding rate further, e.g., to a half-rate, would probably facilitate improved flax establishment but could also increase the risk of competitive annual weed establishment. More work remains to determine the best intercropping practices for perennial flax establishment; however, this approach has already shown clear benefits of providing a grain crop during the perennial flax establishment year while reducing annual weed pressure, even in organic field with sizable annual weed seed banks. Annual grains like winter rye or winter wheat could be successfully intercropped with Lewis flax, but intercropping with legumes would also be an avenue to explore due to soil fertility and pollinator resource benefits. Previous research demonstrated that Kernza© intercropped with white clover, sweet clover (Melilotus officinalis [L.] Pall.), or alfalfa did not suffer seed yield losses (Dick, Cattani and Entz, Reference Dick, Cattani and Entz2018). These perennial legume intercrops contribute to soil N and provide pollinator resource, but do not provide a cash grain crop like small grains.
Lessons learned and future directions
The main objective of this study was to test basic agronomic approaches for seeding, establishing, managing, and harvesting Lewis perennial flax grown as an agronomic oilseed crop. One key insight gained from the study is Lewis flax's readiness to germinate and emerge when planted during either early spring or fall; however, fall planting is considerably more likely to lead to an established stand. This is because forceful precipitation events can easily damage fragile Lewis flax seedlings which are vulnerable while emerging or newly emerged. In the upper Midwest, such precipitation events are more common in the late spring and throughout summer than in the fall. Consequently, planting either in early or late fall is more likely to provide an opportunity for flax seedlings to emerge and establish successfully, free of these damaging storms. Our observations and results strongly suggest an advantage for early fall planting over dormant seeding for increasing stand densities, a surprising result.
Another key insight to Lewis flax gained from our experiments is the need for seeds to be planted quite shallowly, preferably no deeper than 1 cm. Depending on the soil type and seedling equipment, one could consider preplant seedbed preparation operations to create a firmer seedbed. This is probably more important on lighter-textured soils and will also depend on the type of seeding equipment used. Shallow seeding will also benefit from occurring away from forceful rainstorms that could dislodge the tiny seeds and is another reason why the fall planting timing is preferable.
Flax yield was not influenced by seeding density or row spacing, however because inter-row cultivation is an important tool for weed management in perennial flax, 76.2 row spacing may be preferable. This row spacing will also be best for growing intercrops with perennial flax. For experiment two, we used high seeding densities (i.e., 4× and 6× the recommended rate). We observed relatively even and complete emergence, resulting in high flax densities, as many as 100 plants m−1. Though the ‘Maple Grove’ variety of Lewis flax we grew does not have notable cold tolerance for the species (unpublished data, 2023), we did not observe winter kill, despite air temperatures that occasionally fell as low as −38°C during the winter of 2021–2022. Consequently, we suggest that a rate of 1× to 2× of the USDA-NRCS (2004) recommendation is likely sufficient for establishing Lewis flax. These seeding densities, when fall planted with winter wheat, led to sufficient stand establishment at the Comstock site. Because seed is expensive, seeding the minimal rate needed to produce a stand is critical, but seed costs should decrease as breeding increases yield and reduces seed losses. Using the correct equipment to achieve consistent shallow planting depth is also essential. Lanker, Bell and Picasso (Reference Lanker, Bell and Picasso2019) reported that farmers growing Kernza© struggled substantially with basic field operations needed to produce the crop, because the small and light Kernza© seed presented challenges to seeding and harvesting. Growers hoping to grow Lewis flax will undoubtedly face similar issues, but our research suggests equipment that improves seed–soil contact at shallow depths is preferred.
Yield was negatively impacted by boll shattering that occurred between swathing and combining windrows. Our harvest method involved a two-pass operation whereby the flax was first windrowed with a sickle mower then picked up with a small plot combine with a draper head and conventional threshing cylinder after drying. A two-pass operation is required because Lewis flax foliage and stems stay green while the bolls mature. A better choice would be a plot combine equipped with a pickup header with a rotary threshing design, like most modern combines. This design allows the combine to pick up the windrow with less disturbance, and allows threshing at higher straw moisture, thus reducing seed lost to shattering.
Breeding efforts to improve Lewis flax characteristics, which are currently underway, will also be critical to the development of this potential new crop. In addition to increasing yield, another critical improvement will be the development of Lewis flax varieties with more determinant flowering and reduced shattering. Additional research to refine weed management approaches and fertility recommendations will also help to improve Lewis flax yield. Intercropping Lewis flax with annual grains or legumes will likely be an approach that will improve weed management and add economic benefits for producers. However, additional research will be required to fully explore intercropping approaches.
Future research should also focus on assessing agroecosystem services provided by growing perennial flax, including quantifying impacts on soil carbon sequestration, soil water infiltration, soil aggregation, soil microbiology, and pollinator benefits. Quantifying perennial flax contributions to these ecosystem services is critical to drive adoption because the market value of a perennial grain or oilseeds is often partly based on the value of these services. Determining optimal soil fertility management practices to achieve a balance between flax productivity and annual weed growth will also be important. Continuing to refine weed management approaches is another important short-term goal. Other research topics could be assessing Lewis flax stand longevity and determining best management practices to renovate waning stands and manage transitions out of flax to create logical cropping sequences. Lewis flax production could also be tested across a range of soil types and climates to determine optimal growing conditions for the crop in relation to economic returns. Like Kernza, perennial flax production may be more suited to marginal cropland than prime cropland until more productive perennial flax genetics become available.
Data availability statement
The data that support the findings of this study are available from author G. G. upon reasonable request.
Acknowledgements
The authors wish to acknowledge funding from the USDA Sustainable Agriculture Research and Education program (SARE grant #548236), as well as USDA-Hatch funding for project #ND01516. A special thank you to Darin Eisinger, Steve Zwinger, Keith Biggers, and Kristin Boll for their technical support. We would also like to thank Brady Koehler, Hailee Meiners, Zach Tarble, and the interns of the USDA-ARS Hulke Lab for equipment and seed counting assistance.
Author contributions
Burton Johnson, Greta Gramig, and Brent S. Hulke developed the grant proposal for this research. All authors contributed to research question formulation, adaptive management design, and implementation. Greta Gramig led data analysis for this project. All authors contributed to the interpretation of findings and the writing of this manuscript.
Competing interests
We the authors declare no competing interests. Mention of trade names or commercial products in this publication is solely for the purpose of providing specific information and does not imply recommendation or endorsement by the USDA. USDA is an equal opportunity provider and employer.